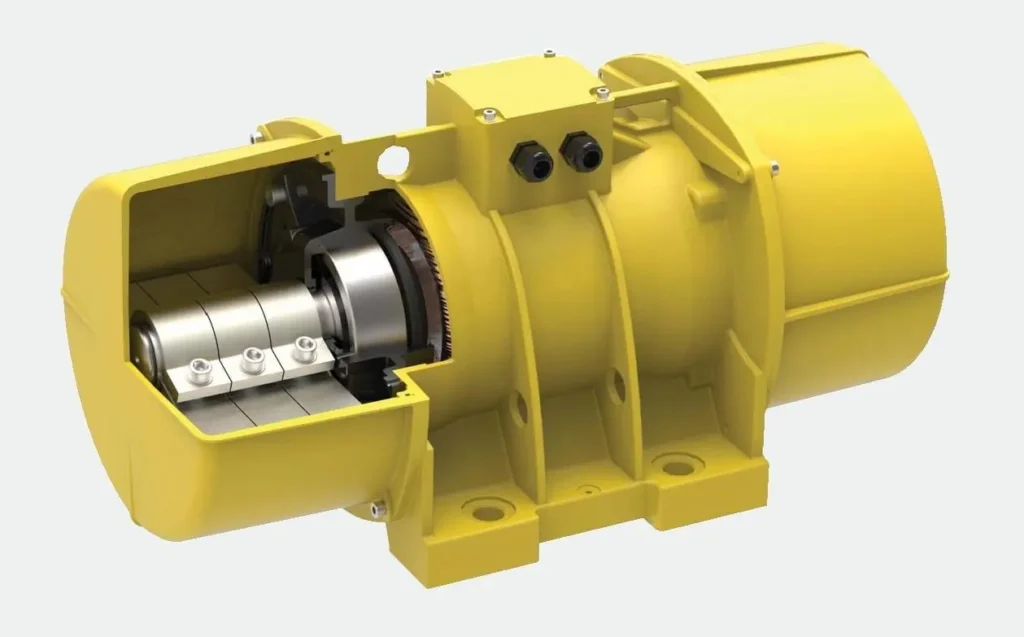
As a Vibration Generator Manufacturer, we design and manufacture AC and DC vibration motors for the industry. Small and Big sizes are available
Vibration Generator
Diving into the world of Vibration Generators, our exploration begins with the foundational term, “Vibration Generator.” A Vibration Generator is a dynamic device that serves as the architectural cornerstone in the orchestration of controlled mechanical oscillations. Functioning as the impetus for vibratory motion, this device finds its applications spanning a spectrum from industrial processes to scientific research and testing.
At its core, the Vibration Generator converts various forms of energy, such as electrical or mechanical, into the rhythmic and controlled vibrations required for a multitude of purposes. This versatility is exemplified in its role as a Mechanical Vibration Generator, where the emphasis lies on harnessing physical forces to generate oscillations. Similarly, as an Electric Vibration Generator, it taps into the efficiency of electrical energy to power its vibratory functions, showcasing the adaptability of this foundational device.
Portability is a defining feature of the Portable Vibration Generator. This variation of the device caters to applications where mobility and flexibility are paramount, allowing for controlled vibrations in diverse settings. Whether in field studies, on-site testing, or temporary setups, the Portable Vibration Generator stands as a testament to the device’s adaptability to dynamic operational landscapes.
The spectrum extends to the specialized realms of High-Frequency Vibration Generators, designed to deliver rapid oscillations. These generators find their niche in applications demanding precision and speed, such as in the testing of electronic components or the calibration of high-frequency systems. Conversely, Low-Frequency Vibration Generators cater to scenarios where slower, deliberate vibrations are essential, emphasizing the device’s adaptability across a range of industrial processes.
The Industrial Vibration Generator represents the robust variant of this foundational device. Engineered for heavy-duty applications, it exhibits durability and reliability in scenarios demanding powerful vibratory forces. This could manifest in tasks such as material compaction in construction or the efficient conveyance of goods in industrial production lines, showcasing the Industrial Vibration Generator’s prowess in shaping the efficiency of large-scale operations.
In laboratory settings, the Lab Vibration Generator becomes a critical tool for scientific experimentation. Its controlled and precise vibratory capabilities find application in modal analysis, fatigue testing, and other research endeavors where understanding and manipulating mechanical vibrations are essential. The Lab Vibration Generator exemplifies the device’s role as an indispensable tool in advancing scientific understanding and technological innovation.
The Variable Frequency Vibration Generator introduces an additional layer of adaptability. With the ability to manipulate the frequency of vibrations, this variant caters to diverse applications where varying vibratory frequencies are required. It becomes an essential component in scenarios ranging from structural analysis to material testing, showcasing the device’s flexibility in addressing the dynamic needs of different industries.
Compactness takes center stage with the Compact Vibration Generator. In applications where space is at a premium, such as in small-scale machinery or portable devices, the Compact Vibration Generator provides a solution without compromising the efficiency of controlled vibrations. This compact variant stands as a testament to the device’s ability to adapt to spatial constraints without sacrificing performance.
Digital advancements usher in the era of Digital Vibration Generators. These generators leverage digital technologies to precisely control and manipulate vibratory patterns. In industries where intricate vibratory sequences are required, such as in vibration testing or simulation, the Digital Vibration Generator becomes an invaluable asset, showcasing the device’s evolution in tandem with technological progress.
The realm of piezoelectric materials introduces the Piezoelectric Vibration Generator. Harnessing the unique properties of piezoelectricity, this variant becomes a beacon of efficiency in applications where compact size, rapid response, and precision are paramount. Piezoelectric Vibration Generators find their place in cutting-edge technologies, including medical devices, where controlled vibrations are employed in procedures requiring utmost precision.
In conclusion, the Vibration Generator stands as the linchpin in the world of controlled mechanical oscillations. From its foundational role as a Mechanical Vibration Generator to its adaptability in various forms, frequencies, and sizes, this device is an instrumental force shaping the landscape of industrial processes, scientific research, and technological innovation. Whether in the form of Portable, High-Frequency, or Piezoelectric variants, the Vibration Generator is a dynamic force that resonates across diverse industries, contributing to the vibrancy and precision of modern applications.
Continuing our exploration into the diverse realm of Vibration Generators, our focus now turns to the second set of keywords, honing in on the concept of the “Mechanical Vibration Generator.” As the second keyword in our journey, the Mechanical Vibration Generator embodies the essence of harnessing physical forces to generate controlled oscillations, serving as a cornerstone in various industrial, scientific, and testing applications.
The core principle of a Mechanical Vibration Generator lies in its ability to convert mechanical energy into rhythmic motion. This process often involves an unbalanced mass, eccentrically attached to a rotating shaft, creating an imbalance that results in vibrations when the shaft is set into motion. This fundamental design renders the Mechanical Vibration Generator as a versatile tool, adaptable to a multitude of scenarios where controlled vibrations are essential.
One of the defining features of the Mechanical Vibration Generator is its broad applicability across different industries. In manufacturing, it plays a pivotal role in the operation of material handling conveyors, facilitating the smooth transport of goods along production lines. The controlled vibratory motion generated by these devices contributes to the precision and efficiency of material conveyance, showcasing their significance in streamlining industrial processes.
In the realm of scientific research, the Mechanical Vibration Generator becomes a cornerstone in modal analysis and vibration testing. Laboratories utilize these generators to understand the natural frequencies and vibrational characteristics of structures and materials. Researchers and engineers leverage the controlled vibrations to assess structural integrity, identify potential weaknesses, and optimize designs in fields ranging from civil engineering to aerospace.
The versatility of the Mechanical Vibration Generator extends to its application in vibration simulation. In industries such as automotive and aerospace, where products must endure various environmental conditions and operational stresses, these generators are employed to simulate real-world vibrations. This ensures that products are tested rigorously under controlled conditions, leading to the development of more robust and reliable structures.
As a pivotal tool in fatigue testing, the Mechanical Vibration Generator aids in evaluating the endurance and durability of materials and components subjected to repetitive vibrational forces. This is particularly crucial in industries such as transportation and infrastructure, where the longevity of materials and structures is paramount to safety and reliability.
The robust construction of Mechanical Vibration Generators is a testament to their ability to withstand demanding industrial environments. Typically housed in sturdy casings crafted from durable materials, these generators exhibit resilience against external factors such as dust, moisture, and mechanical stress. This robustness ensures their longevity and sustained performance in challenging operational conditions.
The Mechanical Vibration Generator is also integral to the realm of seismic testing, where its controlled vibrations simulate seismic events to assess the structural response of buildings and infrastructure. This application is instrumental in earthquake engineering, contributing to the development of structures that can withstand and mitigate the impact of seismic forces.
In conclusion, the Mechanical Vibration Generator emerges as a stalwart in the landscape of controlled mechanical oscillations. From its foundational role in industrial material handling to its sophisticated applications in scientific research and testing, this device epitomizes adaptability and efficiency. As industries evolve and technologies advance, the Mechanical Vibration Generator remains a timeless and indispensable tool, shaping the vibrational landscape of the modern industrial and scientific spheres.
The vibration Generator gives mechanical oscillations when fed by signals from a power signal generator capable of driving a low resistance load, such as the Power Signal Generator (G85793). A special high-temperature coil system enables the unit to be operated at its maximum rating for a
prolonged period without damage due to overheating. The frequency response encompasses the whole of the audio spectrum and beyond. Electrical input is made via two 4mm sockets and the mechanical output is provided by a shaft terminating in a threaded rod with a pair of clamping nuts.
- Maximum peak-to-peak displacement: 8 mm at 1 Hz, decreasing with an increase in frequency.
- Total frequency range: D.C. to 10kHz.
- Coil impedance: 3.5Ω at 50 Hz.
- Dimensions: 100 x 90 x 95mm high.
- The vibrator can be used in any position, free-standing or clamped.
Vibration Generator for Sale
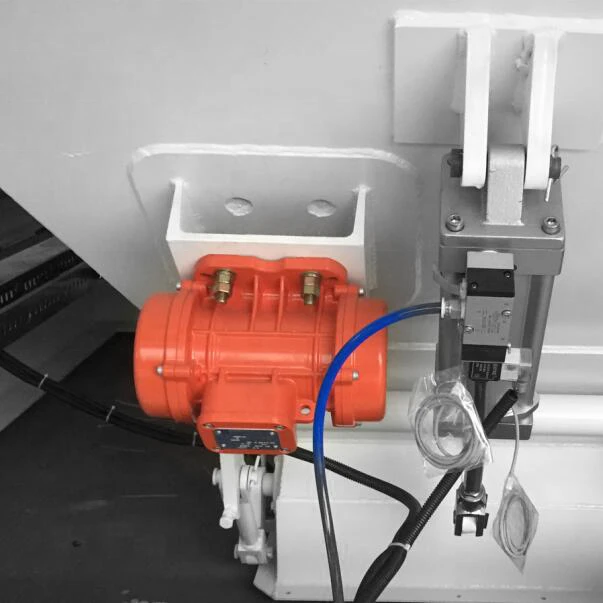
Venturing further into the realm of Vibration Generators, our exploration turns its focus to the third set of keywords, emphasizing the significance of the “Electric Vibration Generator.” As the third keyword in our journey, the Electric Vibration Generator represents a pivot towards efficiency, adaptability, and precision by harnessing the power of electrical energy to propel controlled mechanical oscillations.
The Electric Vibration Generator stands at the nexus of innovation, merging electrical engineering finesse with the demand for precise vibratory motion across diverse applications. Unlike its mechanical counterparts, this variant leverages the efficiency of electrical power, providing a reliable means to generate controlled vibrations in a myriad of industrial, scientific, and testing scenarios.
At its core, the Electric Vibration Generator transforms electrical energy into the rhythmic dance of controlled vibrations, offering a versatile solution across various industries. This adaptability finds expression in its role within material handling conveyors, where the seamless transport of goods along manufacturing lines demands precision. The controlled vibratory forces imparted by Electric Vibration Generators contribute to the streamlined efficiency of material conveyance, embodying their pivotal role in the industrial landscape.
Laboratory settings witness the Electric Vibration Generator as a key player in the realm of scientific experimentation. With precise control over vibratory patterns, this variant becomes an invaluable tool for modal analysis, fatigue testing, and various research endeavors where manipulating mechanical vibrations is essential. The controlled and reliable nature of electrically generated vibrations ensures accuracy in scientific assessments and testing protocols.
A distinctive feature of Electric Vibration Generators lies in their adaptability to variable frequencies. This trait is especially valuable in applications requiring a range of vibratory frequencies. In structural analysis or material testing, for instance, the ability to manipulate the frequency of vibrations becomes crucial, and Electric Vibration Generators emerge as reliable assets in addressing the dynamic needs of different industries.
The Electric Vibration Generator also finds its place in the realm of vibration simulation, particularly in industries such as automotive and aerospace. Here, these generators play a pivotal role in replicating real-world vibrational scenarios, allowing engineers to test and optimize products under controlled conditions. The precise and consistent vibratory forces generated electrically contribute to the development of more resilient and reliable structures and materials.
Beyond industrial applications, Electric Vibration Generators are integral components in the domain of haptic feedback technology. In consumer electronics, particularly smartphones and wearable devices, these generators provide users with tactile responses, enhancing the overall user experience. The vibratory alerts and responses, generated efficiently through electrical means, contribute to the immersive and interactive nature of modern gadgets.
The reliability and adaptability of Electric Vibration Generators extend to their application in vibration testing. Industries ranging from electronics to aerospace leverage these generators to subject products and components to controlled vibrational forces, ensuring that they can withstand operational stresses and environmental conditions. This is particularly crucial in the development of products that adhere to stringent quality and safety standards.
In conclusion, the Electric Vibration Generator emerges as a dynamic force in the landscape of controlled mechanical oscillations. From its role in industrial material handling to its precision applications in scientific research and testing, this variant embodies the evolution and adaptability of vibration generation technology. As industries continue to advance, the Electric Vibration Generator stands as a testament to the seamless integration of electrical efficiency with vibratory precision, shaping the vibrational landscape of modern industrial processes and technological innovations.
Carefully unpack the product, ensuring that you retain the small attachment which is pushed into the polystyrene lid. On observing the unit you will note that there is a slider labeled Unlock/Lock. Ensure this is set to Unlock before using the unit. Always set to Lock after use. You may need to manually adjust the post height to achieve this
Connection to the Power Signal Generator is through standard 4mm plug cables, with one cable from the yellow low-impedance socket and one from the black socket immediately to its right. Set the signal generator to sine wave output at about 3-5Hz and turn up the amplitude. You should see that the armature rises and falls at the set frequency. If you see nothing, check that the fuse holder is tightened properly and also check that the fuse is conducting.
The replacement fuse is a 1A quick blow 20mm glass fuse, which is readily available. The frequency, amplitude, and waveform can be chosen and altered as desired depending upon the vibration you wish to generate. The vibration generator performs best over the frequency range of 3Hz – 10kHz, which can be adjusted as desired by altering the frequency range dial on the Signal Generator.
Vibration Motor
Continuing our journey into the diverse landscape of Vibration Generators, we now shift our focus to the fourth set of keywords, spotlighting the concept of the “Portable Vibration Generator.” As the fourth keyword in our exploration, the Portable Vibration Generator introduces a dimension of mobility and flexibility, showcasing its adaptability to dynamic operational landscapes across various industries.
The Portable Vibration Generator stands as a testament to the demand for controlled mechanical oscillations in scenarios where mobility is paramount. Unlike its stationary counterparts, this variant is designed to be compact, lightweight, and easily transportable, making it a valuable tool in applications ranging from on-site testing to field studies and temporary setups.
At its core, the Portable Vibration Generator exemplifies the marriage of efficiency and portability. This device allows for the generation of controlled vibrations in diverse settings, providing a solution for scenarios where stationary generators might be impractical or impossible to deploy. The controlled vibratory motion facilitated by the Portable Vibration Generator finds application in various industrial, research, and testing contexts.
In field studies, especially in geophysics and environmental monitoring, the Portable Vibration Generator becomes an indispensable tool. Its ability to produce controlled vibrations aids researchers in studying the composition of the Earth’s subsurface or assessing the structural integrity of buildings and infrastructure. The mobility of this generator allows for flexibility in choosing testing locations, contributing to the versatility of field-based research endeavors.
The construction industry also benefits significantly from the Portable Vibration Generator. In on-site scenarios where construction materials need to be compacted or settled, this generator provides a reliable means of imparting controlled vibratory forces. Its portability allows construction crews to efficiently address specific areas that might be challenging to reach with larger, stationary equipment.
The adaptability of Portable Vibration Generators extends to the realm of structural health monitoring. In assessing the integrity of bridges, dams, or other critical infrastructure, these generators can be strategically placed to induce vibrations that reveal potential weaknesses or structural anomalies. This application is vital in ensuring the safety and reliability of essential infrastructure elements.
Research expeditions, whether in archaeology or environmental science, benefit from the versatility of Portable Vibration Generators. In archaeological digs, for instance, these generators can be employed to gently vibrate soil layers, aiding researchers in the discovery and preservation of delicate artifacts. The portability of the generator enhances its utility in remote or challenging terrains.
The entertainment industry also embraces the Portable Vibration Generator in immersive experiences such as virtual reality. By integrating these generators into haptic feedback systems, virtual environments can be enriched with tactile sensations, enhancing the realism of virtual interactions. This application underscores the diverse and evolving uses of Portable Vibration Generators beyond traditional industrial and research settings.
The robust construction of Portable Vibration Generators is pivotal in ensuring their resilience in various environments. Typically housed in durable casings designed to withstand the rigors of transportation and field conditions, these generators exhibit a blend of ruggedness and efficiency. This durability ensures their reliability and performance even in challenging operational settings.
In conclusion, the Portable Vibration Generator emerges as a dynamic and adaptable tool in the vibrational landscape. From on-site construction applications to field-based research endeavors, its mobility and efficiency make it a versatile asset across industries. The Portable Vibration Generator epitomizes the evolving nature of vibration generation technology, catering to the demands of a world where flexibility and mobility are increasingly valued in the pursuit of controlled mechanical oscillations.
Delving further into the intricate world of Vibration Generators, our exploration centers on the fifth set of keywords, shedding light on the concept of the “High-Frequency Vibration Generator.” As the fifth keyword in our journey, the High-Frequency Vibration Generator unveils a specialized variant that operates at frequencies beyond the conventional range, catering to applications where rapid oscillations and precision are paramount.
At its essence, the High-Frequency Vibration Generator represents a technological leap, pushing the boundaries of vibrational capabilities. Unlike its counterparts, this variant is engineered to produce oscillations at frequencies that exceed the standard spectrum. This distinction positions it as a pivotal tool in industries and fields where the demand for swift and precise vibrations is essential.
The High-Frequency Vibration Generator’s primary strength lies in its ability to generate rapid oscillations, often beyond the audible range for humans. This trait makes it invaluable in applications where speed, accuracy, and intricacy are critical. Industries such as electronics, materials testing, and medical research benefit significantly from the precision afforded by high-frequency vibrations.
In the realm of materials testing, particularly in the electronics industry, High-Frequency Vibration Generators play a crucial role. These generators are employed to subject electronic components and devices to accelerated testing conditions. Rapid vibrations simulate the stressors that components might experience during their operational lifespan, allowing manufacturers to identify potential weaknesses and improve the reliability of electronic products.
Medical research and diagnostics also embrace the High-Frequency Vibration Generator. In applications such as ultrasonography, where controlled vibrations are used to produce detailed images of internal structures, the high-frequency variant becomes indispensable. The precise oscillations generated by these devices contribute to the clarity and accuracy of medical imaging, enhancing diagnostic capabilities.
The precision of High-Frequency Vibration Generators finds a niche in the field of nanotechnology. As researchers delve into the manipulation and study of materials at the nanoscale, these generators provide the controlled vibrational forces needed for delicate procedures. The ability to operate at high frequencies ensures that the interactions at the smallest scales can be finely tuned and observed.
Beyond industrial and scientific applications, the entertainment industry leverages the capabilities of High-Frequency Vibration Generators to enhance virtual reality experiences. By incorporating these generators into haptic feedback systems, developers can simulate subtle sensations and detailed interactions, creating a more immersive and realistic virtual environment for users.
The efficiency and precision of High-Frequency Vibration Generators are particularly beneficial in tasks that involve sorting and separation. Industries such as pharmaceuticals and fine materials processing rely on these generators to achieve meticulous separation of particles based on size or density. The high-frequency oscillations ensure a level of accuracy that is crucial in these precision-oriented processes.
The adaptability of High-Frequency Vibration Generators extends to the aerospace industry. In testing scenarios where components need to withstand high-frequency vibrations, these generators contribute to the development of resilient materials and structures. The ability to subject aerospace components to rigorous conditions ensures their reliability and safety in actual operational environments.
The construction industry also benefits from the precision of High-Frequency Vibration Generators, particularly in scenarios where the consolidation of fine materials is required. These generators contribute to the densification of concrete or other construction materials, ensuring uniformity and strength in the final product.
In conclusion, the High-Frequency Vibration Generator emerges as a specialized and powerful tool within the vibrational landscape. Its ability to operate at frequencies beyond the conventional spectrum opens doors to a myriad of applications in industries where precision, speed, and intricacy are paramount. From electronics testing to medical diagnostics and nanotechnology, the High-Frequency Vibration Generator stands as a testament to the evolving nature of vibration generation technology, propelling advancements in various fields with its capacity for rapid and controlled mechanical oscillations.
Push the vibrating armature into the post and fit a thin string into the top, securing it with the screw. Run the string over a fixed pulley and hang a 100g mass hanger from it. Now increase the frequency of the sine wave until a vibration is seen on the string. Finely adjust the frequency until you achieve a standing wave. Measure the wavelength using a meter rule and make a note of the frequency. Calculate the velocity of the wave for that particular string tension. Now add a 100g mass to the hanger and repeat. Repeat for additional 100g masses. Please note that you should compare like with like i.e. the same number of nodes on the string.
Vibration motors are a type of motor that, as the name suggests, create a vibrating motion. They have become integral components in many modern devices and systems, providing feedback, alerts, and haptic responses that enhance user experience and functionality. These motors are designed to convert electrical energy into mechanical vibration, and they are used across various industries, including consumer electronics, automotive, medical devices, and industrial applications.
Historical Development
The history of vibration motors dates back to the early 20th century when basic mechanisms for generating vibration were first conceptualized. Over the years, technological advancements have led to more sophisticated designs, allowing for a wide range of applications. Early vibration motors were primarily used in industrial settings for tasks such as sorting and conveying materials. However, with the miniaturization of components and improvements in precision and control, they have become essential in everyday consumer electronics, providing tactile feedback and alerts in mobile devices and wearables.
Overview of Different Types of Vibration Motors
Vibration motors come in various types, each with unique characteristics and applications. The most common types include:
- Eccentric Rotating Mass (ERM) Motors: These motors generate vibration through the rotation of an off-center mass attached to the motor shaft. They are widely used in consumer electronics for simple haptic feedback.
- Linear Resonant Actuators (LRA): Unlike ERM motors, LRAs use a magnetic field to move a mass linearly, creating a more refined and precise vibration. This type is favored in applications requiring specific frequency responses and quick reaction times.
- Piezoelectric Vibration Motors: These utilize piezoelectric materials that expand or contract when an electrical voltage is applied. They offer high precision and are often used in applications requiring minimal size and power consumption.
This introduction sets the stage for a deeper exploration of the technical aspects, applications, and future developments of vibration motors.
Types of Vibration Motors
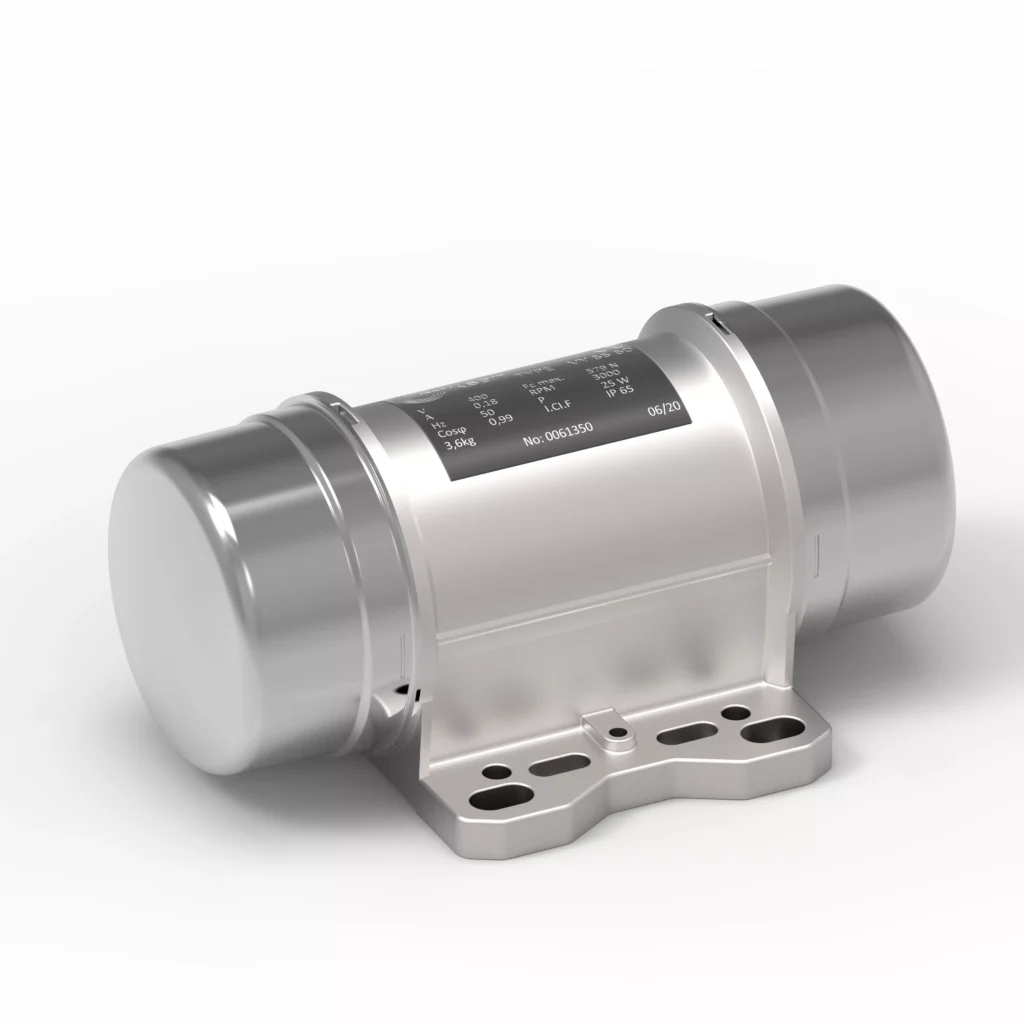
Understanding the different types of vibration motors is crucial for selecting the right one for a specific application. Each type has distinct characteristics that make it suitable for particular uses. This section explores the main types of vibration motors, their design, operation, and typical applications.
Eccentric Rotating Mass (ERM) Motors
Design and Operation
Eccentric Rotating Mass (ERM) motors are among the most widely used vibration motors. They operate by rotating an unbalanced mass, which generates centrifugal force, causing the motor to vibrate. The frequency and amplitude of the vibration are determined by the speed of rotation and the size of the mass.
The basic components of an ERM motor include:
- Motor Shaft: The central axis on which the unbalanced mass is mounted.
- Unbalanced Mass: The key component responsible for generating vibration. It is usually attached off-center on the motor shaft.
- Housing: Encloses the motor components, protecting them from environmental factors and mechanical damage.
ERM motors are relatively simple in design, making them cost-effective and easy to produce. They are typically used in applications where basic haptic feedback is needed, such as in mobile phones and handheld gaming devices.
Common Uses
- Mobile Phones and Tablets: ERM motors provide tactile feedback for touchscreen interactions and notifications.
- Wearable Devices: Used in smartwatches and fitness trackers to alert users with vibrations.
- Remote Controls: Enhance user experience by providing feedback during button presses.
Linear Resonant Actuators (LRA)
Design and Operation
Linear Resonant Actuators (LRA) are more advanced than ERM motors, offering improved precision and control. They consist of a movable mass attached to a spring and a magnetic field. When an AC voltage is applied, the mass oscillates linearly, creating a vibration.
Key components of LRA include:
- Movable Mass: Suspended by springs, this component moves back and forth to generate vibration.
- Electromagnetic Coil: Creates a magnetic field that interacts with the movable mass.
- Spring System: Ensures the mass returns to its original position after displacement.
LRA motors are known for their high-frequency response and efficiency, making them suitable for applications requiring precise control and fast response times.
Common Uses
- Haptic Feedback in Mobile Devices: Provides more nuanced and responsive feedback compared to ERM motors.
- Gaming Controllers: Enhances immersion by delivering realistic vibrations based on in-game events.
- Automotive Touchscreens: Improves user interaction with infotainment systems through tactile feedback.
Piezoelectric Vibration Motors
Design and Operation
Piezoelectric vibration motors leverage the piezoelectric effect, where certain materials generate an electric charge in response to mechanical stress. These motors consist of piezoelectric elements that deform when an electric field is applied, producing vibration.
Key components of piezoelectric motors include:
- Piezoelectric Elements: The core components that expand or contract to create vibration.
- Metal Plate: Acts as a diaphragm that vibrates in response to the deformation of the piezoelectric elements.
- Drive Circuit: Controls the frequency and amplitude of the applied voltage, affecting the vibration characteristics.
Piezoelectric motors are prized for their small size, low power consumption, and high precision. They are often used in applications where space is limited, and precise control is required.
Common Uses
- Medical Devices: Provide haptic feedback in surgical tools and diagnostic equipment.
- Consumer Electronics: Used in compact devices such as smartwatches and portable audio players.
- Industrial Sensors: Enhance sensitivity and accuracy in measurement instruments.
Comparison of Different Types
Each type of vibration motor has its advantages and disadvantages, influencing the selection process for specific applications.
Type | Advantages | Disadvantages |
---|---|---|
ERM | – Simple design<br>- Cost-effective<br>- Easy to produce | – Limited frequency range<br>- Slower response time |
LRA | – Precise control<br>- Fast response<br>- Efficient | – Higher cost<br>- More complex design |
Piezoelectric | – Small size<br>- Low power consumption<br>- High precision | – Limited force output<br>- Requires specialized materials |
Selection Criteria for Different Applications
When selecting a vibration motor, several factors should be considered:
- Application Requirements: Determine the desired frequency, amplitude, and response time.
- Size and Weight: Consider the available space and weight constraints in the device or system.
- Power Consumption: Evaluate the power requirements, especially for battery-powered devices.
- Cost: Balance performance needs with budgetary constraints.
In summary, the choice of vibration motor depends on the specific application requirements, balancing factors such as performance, size, cost, and power consumption.
Technical Aspects of Vibration Motors
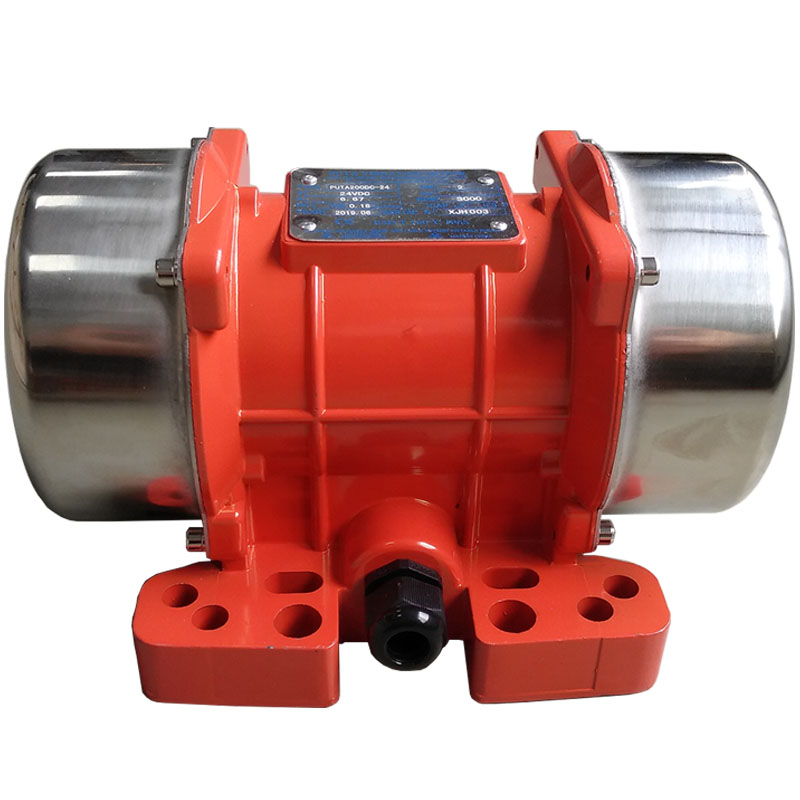
A comprehensive understanding of the technical aspects of vibration motors is essential for designing and integrating them into various applications. This section explores the construction, key specifications, performance characteristics, and design considerations associated with vibration motors.
Construction and Materials
Vibration motors are constructed using materials and components that ensure durability, efficiency, and performance. The choice of materials can significantly impact the motor’s lifespan and functionality.
Key Components
- Motor Housing: Typically made from metal or high-strength plastic, the housing protects the internal components from mechanical damage and environmental factors. It also serves as a mounting structure.
- Rotor and Stator: The rotor is the rotating part of the motor, while the stator is the stationary component. These are usually made from magnetic materials to facilitate electromagnetic interactions.
- Bearings: Bearings reduce friction between moving parts and support the motor shaft. They are often made from materials like stainless steel or ceramic for durability and reliability.
- Wiring and Connectors: Electrical connections are made using insulated copper wires and connectors that provide reliable electrical conductivity.
- Unbalanced Mass or Piezoelectric Elements: Depending on the type of motor, these components generate the vibrations. The unbalanced mass is typically made of metal, while piezoelectric elements are crafted from specialized ceramic materials.
Key Specifications
When selecting a vibration motor, several key specifications must be considered to ensure optimal performance for the intended application.
Size and Dimensions
- Length, Width, and Height: The overall dimensions of the motor must fit within the available space in the device or system.
- Weight: The motor’s weight can affect the balance and handling of the device, particularly in portable applications.
Power and Voltage
- Operating Voltage: The voltage required to power the motor, which can range from a few volts in small devices to several hundred volts in industrial applications.
- Power Consumption: The amount of electrical power consumed by the motor during operation. Lower power consumption is preferred for battery-powered devices.
Frequency and Amplitude
- Frequency Range: The range of vibration frequencies the motor can produce, typically measured in Hertz (Hz).
- Amplitude: The magnitude of the vibration, often expressed in millimeters (mm) or inches.
Performance Characteristics
Understanding the performance characteristics of vibration motors is crucial for achieving the desired vibration effects in various applications.
- Vibration Strength: The intensity of the vibration produced by the motor, influenced by factors such as motor speed, mass, and power input.
- Response Time: The time it takes for the motor to reach its desired vibration state after power is applied. Faster response times are preferred for applications requiring immediate feedback.
- Efficiency: The ratio of mechanical output power to electrical input power, indicating how effectively the motor converts electrical energy into vibration.
- Durability and Lifespan: The expected operational lifespan of the motor, affected by factors such as material quality, construction, and operating conditions.
Design Considerations and Challenges
Designing and integrating vibration motors into devices and systems involves several challenges and considerations to ensure optimal performance and reliability.
Heat Dissipation
Vibration motors generate heat during operation, which can affect their performance and lifespan. Effective heat dissipation methods, such as using heat sinks or conductive materials, are essential to prevent overheating.
Noise and Vibration Isolation
Unwanted noise and vibrations can interfere with the operation of devices and systems. Implementing noise and vibration isolation techniques, such as using damping materials or mounting systems, can minimize these issues.
Environmental Factors
Vibration motors may be exposed to various environmental factors, such as temperature extremes, moisture, and dust. Designing motors with protective features, such as seals or coatings, can enhance their resilience to environmental challenges.
Integration with Control Systems
Integrating vibration motors with control systems requires careful consideration of factors such as signal processing, power management, and feedback mechanisms. This ensures precise control and synchronization with other components in the system.
In conclusion, the technical aspects of vibration motors encompass a wide range of considerations, from construction and materials to performance characteristics and design challenges. Understanding these aspects is essential for selecting and implementing the right vibration motor for specific applications.
Applications of Vibration Motors
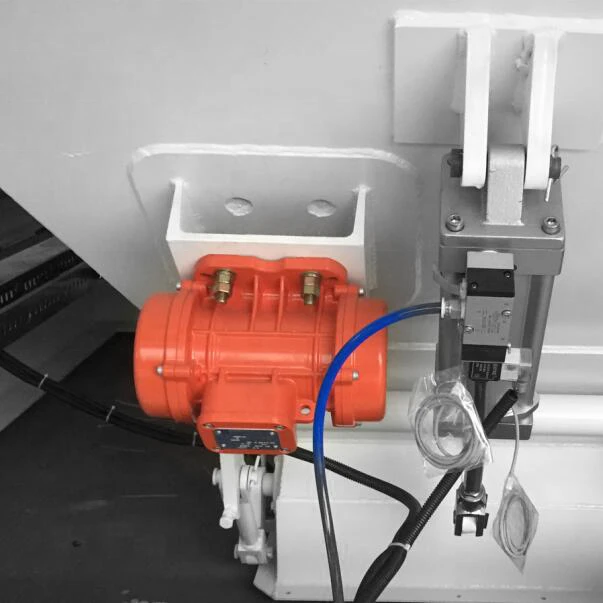
Vibration motors play a crucial role in various industries, enhancing functionality and user experience through tactile feedback, alerts, and precise control. This section explores the diverse applications of vibration motors across different sectors.
Consumer Electronics
Mobile Phones and Tablets
Vibration motors are integral components in mobile phones and tablets, providing haptic feedback for touchscreen interactions and notifications. They enhance the user experience by delivering tactile responses to touch inputs, alerts, and messages.
- Touchscreen Feedback: Vibration motors simulate the sensation of pressing physical buttons, improving user interaction with touchscreen interfaces.
- Notifications and Alerts: Vibration motors provide discreet alerts for incoming calls, messages, and notifications, allowing users to stay informed without relying on audible alerts.
Wearables and Smart Devices
In wearable technology, vibration motors are used to deliver notifications and alerts directly to the user’s wrist or body. This enhances the functionality and convenience of devices such as smartwatches and fitness trackers.
- Fitness Tracking: Vibration motors provide feedback for activity goals, heart rate monitoring, and other fitness metrics.
- Health Monitoring: Vibration alerts can signal important health-related notifications, such as medication reminders or emergency alerts.
Automotive Industry
Haptic Feedback in Touchscreens
Vibration motors are used in automotive touchscreens to enhance user interaction with infotainment and navigation systems. Haptic feedback provides tactile responses to touch inputs, improving usability and reducing driver distraction.
- Infotainment Systems: Vibration motors simulate button presses, enhancing the user experience and making interactions more intuitive.
- Navigation Controls: Haptic feedback assists drivers in navigating menus and settings, ensuring safer and more efficient operation.
Alert Systems
Vibration motors are employed in automotive alert systems to provide non-intrusive warnings and notifications to drivers. These systems enhance safety by delivering tactile alerts for various vehicle conditions and hazards.
- Collision Warning: Vibration alerts can warn drivers of potential collisions or obstacles, improving reaction times and safety.
- Lane Departure Warning: Vibration motors provide feedback when a vehicle deviates from its lane, helping drivers maintain proper lane positioning.
Industrial Applications
Equipment and Machinery Monitoring
In industrial settings, vibration motors are used for equipment and machinery monitoring. They provide feedback on the operational status and performance of machinery, helping to identify issues and prevent failures.
- Condition Monitoring: Vibration sensors detect changes in equipment performance, allowing for timely maintenance and repairs.
- Fault Detection: Vibration analysis helps identify mechanical faults, such as imbalances or misalignments, improving equipment reliability and efficiency.
Material Handling and Processing
Vibration motors are employed in material handling and processing applications, facilitating the movement and sorting of materials. They improve efficiency and accuracy in various industrial processes.
- Vibratory Conveyors: Vibration motors drive conveyors that transport materials, enhancing the speed and precision of material handling.
- Sorting and Screening: Vibration motors assist in sorting and screening materials based on size, weight, or other characteristics, optimizing production processes.
Medical Devices
Feedback Systems in Prosthetics
In the medical field, vibration motors are used in prosthetic devices to provide sensory feedback to users. This enhances the functionality and user experience of prosthetics, improving mobility and control.
- Tactile Feedback: Vibration motors simulate sensations such as touch and pressure, helping users interact with their environment.
- Control Feedback: Vibration alerts assist users in controlling prosthetic movements, enhancing precision and coordination.
Patient Monitoring Equipment
Vibration motors are employed in patient monitoring equipment to deliver alerts and notifications for various medical conditions. They improve patient care by providing timely and discreet notifications to healthcare professionals and patients.
- Vital Sign Monitoring: Vibration alerts signal changes in vital signs, such as heart rate or blood pressure, allowing for prompt medical intervention.
- Medication Reminders: Vibration motors provide reminders for medication administration, ensuring adherence to treatment plans.
Other Emerging Applications
Virtual Reality and Gaming
In virtual reality (VR) and gaming, vibration motors enhance immersion by delivering realistic tactile feedback based on in-game events and interactions. This improves the overall gaming experience and realism.
- Haptic Feedback in VR: Vibration motors simulate various sensations, such as impacts, textures, and environmental effects, enhancing the sense of presence in virtual environments.
- Gaming Controllers: Vibration motors provide feedback for in-game actions, such as explosions, collisions, and character movements, improving player engagement and satisfaction.
Robotics and Automation
Vibration motors are used in robotics and automation to enhance precision, control, and feedback in various applications. They improve the performance and functionality of robotic systems.
- Tactile Sensors: Vibration motors provide feedback for robotic grippers and manipulators, improving object handling and manipulation.
- Precision Control: Vibration alerts assist in fine-tuning robotic movements, enhancing accuracy and coordination in automated processes.
In conclusion, vibration motors have diverse applications across various industries, enhancing functionality, user experience, and performance. Their versatility and adaptability make them essential components in modern technology and systems.
Future Trends and Innovations
As technology continues to evolve, vibration motors are expected to undergo significant advancements, leading to new applications and improved performance. This section explores the future trends and innovations in vibration motor technology.
Technological Advancements
- Miniaturization: Advances in manufacturing and materials will enable the development of smaller and more compact vibration motors, expanding their use in miniaturized devices and systems.
- Increased Efficiency: Innovations in motor design and materials will lead to more efficient vibration motors with lower power consumption and higher performance.
- Improved Control and Precision: Enhanced control algorithms and sensor integration will enable more precise and customizable vibration effects, improving user experience and application performance.
Integration with IoT and Smart Technologies
The integration of vibration motors with the Internet of Things (IoT) and smart technologies will open up new possibilities for applications and functionality.
- Smart Home Devices: Vibration motors will provide tactile feedback and alerts in smart home devices, enhancing user interaction and control.
- Wearable Health Monitors: Integration with IoT will enable real-time monitoring and feedback for health and fitness applications, improving user outcomes and experiences.
Potential New Applications
- Augmented Reality (AR): Vibration motors will enhance AR experiences by providing tactile feedback for virtual interactions, improving immersion and realism.
- Advanced Automotive Systems: Vibration motors will play a role in advanced driver assistance systems (ADAS) and autonomous vehicles, providing feedback and alerts for various driving conditions and scenarios.
- Next-Generation Consumer Electronics: Vibration motors will continue to enhance user experience in emerging consumer electronics, such as foldable devices and flexible displays.
In summary, the future of vibration motors is promising, with advancements in technology and integration with smart systems leading to new applications and improved performance. These innovations will continue to enhance functionality and user experience across various industries.
Conclusion
Vibration motors are essential components in modern technology, providing tactile feedback, alerts, and precise control in a wide range of applications. This article has explored the various types of vibration motors, their technical aspects, and their diverse applications across industries.
Recap of Key Points
- Types of Vibration Motors: We examined the three main types of vibration motors—Eccentric Rotating Mass (ERM), Linear Resonant Actuators (LRA), and Piezoelectric vibration motors—each with unique characteristics and applications.
- Technical Aspects: We discussed the construction, key specifications, performance characteristics, and design considerations associated with vibration motors, highlighting the factors that influence their selection and integration.
- Applications: We explored the diverse applications of vibration motors in consumer electronics, automotive, industrial, medical, and other emerging fields, showcasing their versatility and adaptability.
- Future Trends and Innovations: We examined the future trends and innovations in vibration motor technology, including miniaturization, increased efficiency, and integration with IoT and smart technologies.
Importance of Vibration Motors in Modern Technology
Vibration motors play a crucial role in enhancing functionality and user experience across various industries. Their ability to provide tactile feedback, alerts, and precise control makes them indispensable components in modern devices and systems.
- Consumer Electronics: Vibration motors improve user interaction and experience in mobile phones, wearables, and other consumer devices.
- Automotive Industry: Vibration motors enhance safety and usability in automotive systems, providing feedback and alerts for various conditions.
- Industrial and Medical Applications: Vibration motors improve efficiency and performance in industrial processes and medical devices, providing valuable feedback and control.
Final Thoughts on Future Developments
As technology continues to advance, vibration motors will play an increasingly important role in emerging applications and industries. Innovations in design, materials, and integration will lead to new possibilities and improved performance, enhancing functionality and user experience.
In conclusion, vibration motors are vital components in modern technology, providing essential functionality and enhancing user experience across a wide range of applications. Their versatility, adaptability, and potential for future advancements make them indispensable in the ever-evolving technological landscape.
Introduction to Industrial Vibration Motors
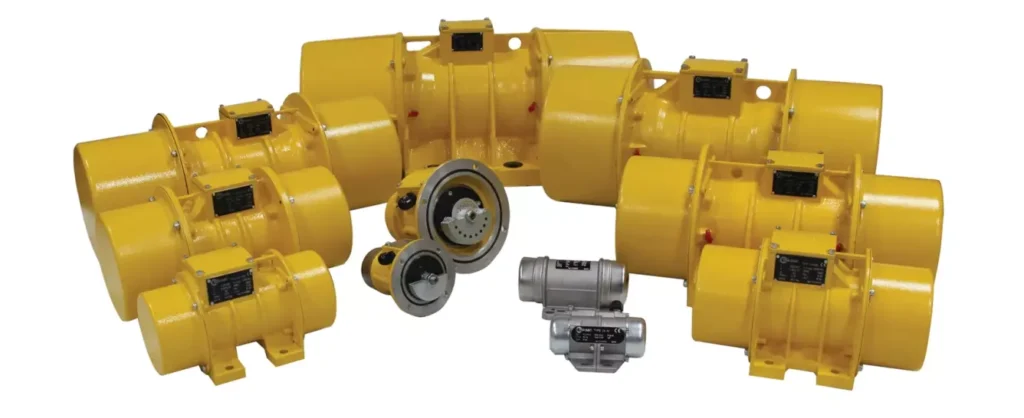
Industrial vibration motors are specialized devices designed to generate mechanical vibrations for various industrial applications. They are integral to processes that require the movement, sorting, and monitoring of materials and equipment. By converting electrical energy into mechanical vibrations, these motors facilitate efficient material handling, quality control, and operational monitoring in industrial settings.
Key functions of industrial vibration motors include:
- Material Movement: Vibration motors aid in the transportation and handling of bulk materials, ensuring smooth and efficient processes.
- Sorting and Separation: They enhance the sorting and separation of materials based on size, weight, or other characteristics, optimizing production efficiency.
- Equipment Monitoring: Vibration motors provide feedback on the operational status of machinery, helping to identify potential issues before they lead to failures.
Historical Context
The use of vibration in industrial applications can be traced back to the early 20th century when basic vibrating mechanisms were employed for tasks such as material sorting and handling. The development of dedicated vibration motors, however, marked a significant advancement in technology.
- Early Innovations: Initial applications were primarily mechanical, relying on simple mechanisms to generate vibrations. These early systems were limited in precision and control.
- Technological Advancements: With the advent of electric motors and advancements in materials science, vibration motors became more sophisticated, offering improved control, efficiency, and versatility.
- Modern Applications: Today, industrial vibration motors are used across a wide range of industries, including mining, construction, food processing, and pharmaceuticals, highlighting their essential role in modern industrial processes.
Key Features
Industrial vibration motors are characterized by several key features that make them suitable for demanding applications:
- Robust Construction: Designed to withstand harsh industrial environments, vibration motors are built with durable materials and components.
- High Efficiency: They are engineered for optimal energy conversion, ensuring efficient performance and minimal energy loss.
- Versatility: Available in various sizes and configurations, vibration motors can be tailored to specific industrial needs and applications.
- Precision Control: Advanced control systems allow for precise adjustment of vibration frequency and amplitude, enabling customized solutions for diverse industrial tasks.
Key takeaways:
- Essential for Industrial Processes: Vibration motors are vital for efficient and effective material handling, sorting, and monitoring in industrial settings.
- Technological Evolution: From early mechanical systems to modern electric motors, technological advancements have significantly enhanced their capabilities.
- Diverse Applications: Used across multiple industries, vibration motors are adaptable to various tasks, showcasing their versatility and importance in modern industry.
Types of Industrial Vibration Motors
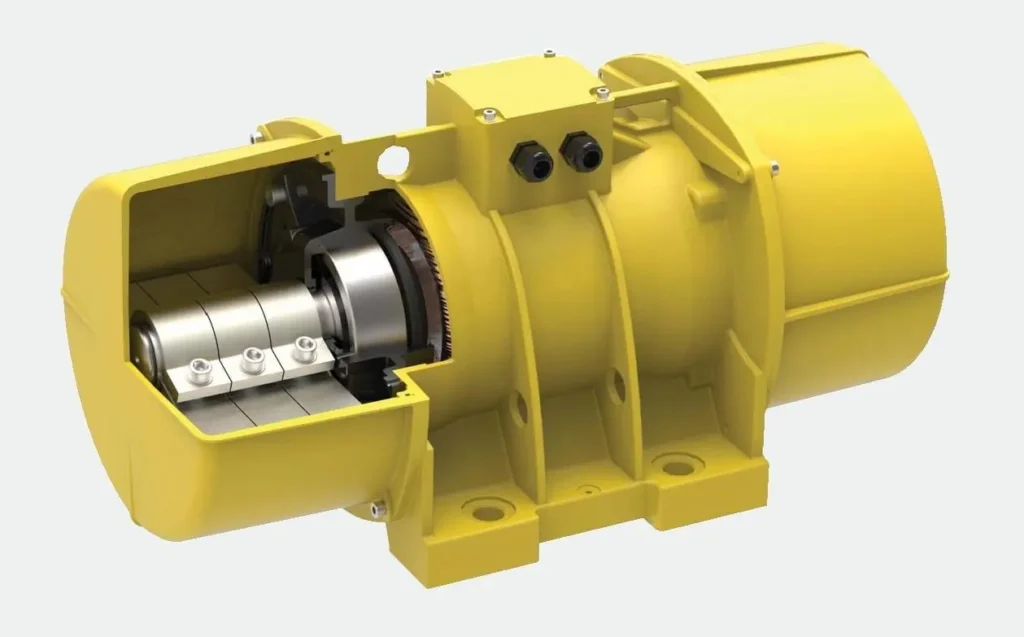
Understanding the different types of industrial vibration motors is crucial for selecting the appropriate motor for specific applications. Each type offers unique characteristics and advantages, making them suitable for various industrial tasks.
Eccentric Rotating Mass (ERM) Motors
Design and Operation
Eccentric Rotating Mass (ERM) motors are among the most commonly used vibration motors in industrial applications. They operate by rotating an unbalanced mass attached to the motor shaft, generating centrifugal force that produces vibration. The frequency and amplitude of the vibration are determined by the speed of rotation and the size of the unbalanced mass.
Key components of ERM motors include:
- Motor Shaft: The central axis on which the unbalanced mass is mounted.
- Unbalanced Mass: The component responsible for generating vibration. It is usually attached off-center on the motor shaft.
- Housing: Encloses the motor components, protecting them from environmental factors and mechanical damage.
Advantages of ERM motors:
- Simple Design: The straightforward design makes them easy to manufacture and maintain.
- Cost-Effective: They are relatively inexpensive compared to other types of vibration motors.
- Versatile Applications: Suitable for a wide range of industrial tasks, including material handling and sorting.
Common Applications
- Material Conveying: ERM motors drive vibratory conveyors, enhancing the movement of bulk materials.
- Screening and Sorting: They facilitate the sorting and screening of materials based on size and weight.
- Compact Equipment: ERM motors are used in applications where space is limited, providing efficient vibration solutions.
Linear Resonant Actuators (LRA)
Design and Operation
Linear Resonant Actuators (LRA) differ from ERM motors in that they generate vibration through the linear movement of a mass rather than rotational motion. An electromagnetic field is used to move the mass back and forth, producing vibrations with high precision and control.
Key components of LRA motors include:
- Movable Mass: Suspended by springs, the mass moves linearly to generate vibration.
- Electromagnetic Coil: Creates a magnetic field that interacts with the movable mass.
- Spring System: Ensures the mass returns to its original position after displacement.
Advantages of LRA motors:
- Precise Control: Offer more precise control over vibration frequency and amplitude.
- Efficient Performance: Consume less power compared to ERM motors, making them suitable for energy-sensitive applications.
- High-Frequency Response: Capable of producing high-frequency vibrations with minimal delay.
Common Applications
- Precision Manufacturing: Used in processes requiring exact vibration control, such as semiconductor manufacturing.
- Laboratory Equipment: Enhance the precision and accuracy of laboratory instruments and devices.
- Quality Control: Facilitate quality control processes by providing precise vibration feedback.
Electromagnetic Vibration Motors
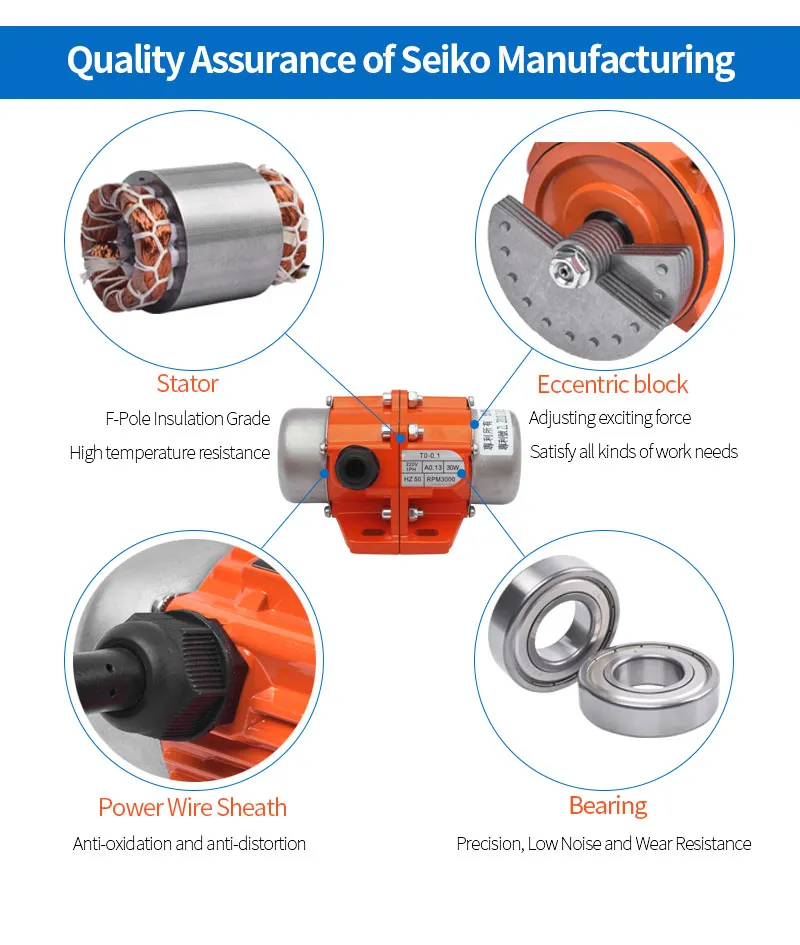
Design and Operation
Electromagnetic vibration motors generate vibrations through the interaction of magnetic fields. These motors consist of an electromagnetic coil and a vibrating armature, which is set into motion by the magnetic field generated when current flows through the coil.
Key components of electromagnetic motors include:
- Electromagnetic Coil: Generates a magnetic field when energized.
- Vibrating Armature: Responds to the magnetic field, creating vibrations.
- Damping System: Reduces noise and unwanted vibrations, enhancing motor performance.
Advantages of electromagnetic motors:
- Smooth Operation: Provide smooth and continuous vibrations, reducing mechanical stress on components.
- Adjustable Frequency: Allow for precise control of vibration frequency and amplitude.
- Low Noise Levels: Operate with minimal noise, making them suitable for noise-sensitive environments.
Common Applications
- Conveying Systems: Used in conveyor systems for smooth and efficient material transportation.
- Vibratory Feeders: Enhance the performance of vibratory feeders used in packaging and assembly lines.
- Textile Industry: Improve the efficiency of textile processing equipment by providing consistent vibrations.
Piezoelectric Vibration Motors
Design and Operation
Piezoelectric vibration motors leverage the piezoelectric effect, where certain materials generate an electric charge in response to mechanical stress. These motors consist of piezoelectric elements that expand or contract when an electric field is applied, producing vibration.
Key components of piezoelectric motors include:
- Piezoelectric Elements: The core components that expand or contract to create vibration.
- Metal Plate: Acts as a diaphragm that vibrates in response to the deformation of the piezoelectric elements.
- Drive Circuit: Controls the frequency and amplitude of the applied voltage, affecting the vibration characteristics.
Advantages of piezoelectric motors:
- High Precision: Offer precise control over vibration characteristics, making them suitable for sensitive applications.
- Compact Design: Small and lightweight, ideal for applications with space constraints.
- Low Power Consumption: Require minimal power, making them energy-efficient.
Common Applications
- Medical Devices: Provide precise vibration feedback in medical diagnostic equipment.
- Microelectronics: Enhance the performance of microelectronic devices by providing controlled vibrations.
- Research and Development: Used in experimental setups requiring precise vibration control.
Comparison of Types
Each type of industrial vibration motor has its own set of advantages and disadvantages, influencing their suitability for specific applications.
Type | Advantages | Disadvantages |
---|---|---|
ERM | – Simple design<br>- Cost-effective<br>- Versatile | – Limited frequency range<br>- Slower response time |
LRA | – Precise control<br>- High efficiency<br>- Fast response | – Higher cost<br>- More complex design |
Electromagnetic | – Smooth operation<br>- Adjustable frequency<br>- Low noise | – Complex construction<br>- Requires precise alignment |
Piezoelectric | – High precision<br>- Compact design<br>- Low power consumption | – Limited force output<br>- Specialized materials required |
Selection Criteria:
- Application Requirements: Determine the desired frequency, amplitude, and response time.
- Size and Weight: Consider the available space and weight constraints in the application.
- Power Consumption: Evaluate the power requirements, especially for energy-sensitive applications.
- Cost: Balance performance needs with budgetary constraints.
Summary:
- Diverse Options: A variety of vibration motors are available, each with unique characteristics and advantages.
- Application-Specific Selection: The choice of motor depends on the specific requirements and constraints of the application.
Technical Aspects of Industrial Vibration Motors
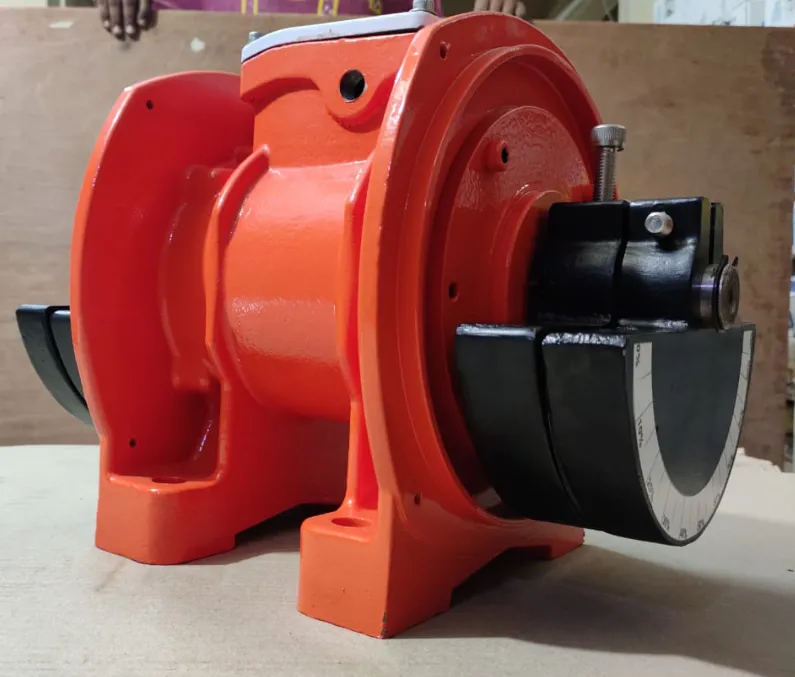
A comprehensive understanding of the technical aspects of industrial vibration motors is essential for designing and integrating them into various applications. This section explores the construction, key specifications, performance characteristics, and design considerations associated with vibration motors.
Construction and Materials
Industrial vibration motors are constructed using materials and components that ensure durability, efficiency, and performance. The choice of materials can significantly impact the motor’s lifespan and functionality.
Key Components
- Motor Housing: Typically made from metal or high-strength plastic, the housing protects the internal components from mechanical damage and environmental factors. It also serves as a mounting structure.
- Rotor and Stator: The rotor is the rotating part of the motor, while the stator is the stationary component. These are usually made from magnetic materials to facilitate electromagnetic interactions.
- Bearings: Bearings reduce friction between moving parts and support the motor shaft. They are often made from materials like stainless steel or ceramic for durability and reliability.
- Wiring and Connectors: Electrical connections are made using insulated copper wires and connectors that provide reliable electrical conductivity.
- Unbalanced Mass or Piezoelectric Elements: Depending on the type of motor, these components generate the vibrations. The unbalanced mass is typically made of metal, while piezoelectric elements are crafted from specialized ceramic materials.
Key Specifications
When selecting a vibration motor, several key specifications must be considered to ensure optimal performance for the intended application.
Size and Dimensions
- Length, Width, and Height: The overall dimensions of the motor must fit within the available space in the device or system.
- Weight: The motor’s weight can affect the balance and handling of the device, particularly in portable applications.
Power and Voltage
- Operating Voltage: The voltage required to power the motor, which can range from a few volts in small devices to several hundred volts in industrial applications.
- Power Consumption: The amount of electrical power consumed by the motor during operation. Lower power consumption is preferred for battery-powered devices.
Frequency and Amplitude
- Frequency Range: The range of vibration frequencies the motor can produce, typically measured in Hertz (Hz).
- Amplitude: The magnitude of the vibration, often expressed in millimeters (mm) or inches.
Performance Characteristics
Understanding the performance characteristics of vibration motors is crucial for achieving the desired vibration effects in various applications.
- Vibration Strength: The intensity of the vibration produced by the motor, influenced by factors such as motor speed, mass, and power input.
- Response Time: The time it takes for the motor to reach its desired vibration state after power is applied. Faster response times are preferred for applications requiring immediate feedback.
- Efficiency: The ratio of mechanical output power to electrical input power, indicating how effectively the motor converts electrical energy into vibration.
- Durability and Lifespan: The expected operational lifespan of the motor, affected by factors such as material quality, construction, and operating conditions.
Design Considerations and Challenges
Designing and integrating vibration motors into devices and systems involves several challenges and considerations to ensure optimal performance and reliability.
Heat Dissipation
Vibration motors generate heat during operation, which can affect their performance and lifespan. Effective heat dissipation methods, such as using heat sinks or conductive materials, are essential to prevent overheating.
Noise and Vibration Isolation
Unwanted noise and vibrations can interfere with the operation of devices and systems. Implementing noise and vibration isolation techniques, such as using damping materials or mounting systems, can minimize these issues.
Environmental Factors
Vibration motors may be exposed to various environmental factors, such as temperature extremes, moisture, and dust. Designing motors with protective features, such as seals or coatings, can enhance their resilience to environmental challenges.
Integration with Control Systems
Integrating vibration motors with control systems requires careful consideration of factors such as signal processing, power management, and feedback mechanisms. This ensures precise control and synchronization with other components in the system.
In conclusion, the technical aspects of vibration motors encompass a wide range of considerations, from construction and materials to performance characteristics and design challenges. Understanding these aspects is essential for selecting and implementing the right vibration motor for specific applications.
Applications of Industrial Vibration Motors
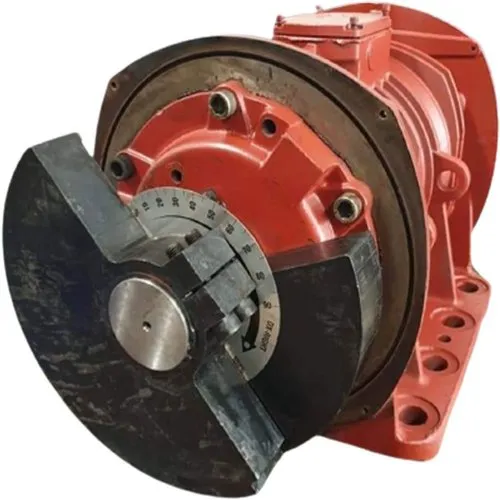
Industrial vibration motors play a crucial role in enhancing efficiency, productivity, and quality across various industries. This section explores the diverse applications of vibration motors in industrial settings.
Material Handling and Processing
Vibratory Conveyors
Vibration motors are integral components of vibratory conveyors, which are used to transport bulk materials efficiently. By generating controlled vibrations, these motors facilitate the smooth movement of materials, reducing friction and wear on conveyor surfaces.
- Efficient Transportation: Vibration motors enable the continuous flow of materials, minimizing downtime and increasing throughput.
- Versatile Applications: Vibratory conveyors are used in industries such as food processing, pharmaceuticals, and mining, where precise material handling is essential.
Screening and Sorting
In screening and sorting applications, vibration motors enhance the separation of materials based on size, weight, or other characteristics. They drive vibratory screens and separators, ensuring accurate and efficient sorting processes.
- Improved Accuracy: Vibration motors provide consistent and controlled vibrations, improving the accuracy of sorting and screening operations.
- Customizable Solutions: Adjustable frequency and amplitude settings allow for tailored solutions to meet specific sorting requirements.
Feeding and Dosing
Vibration motors are used in feeding and dosing applications to deliver precise amounts of materials to processing equipment. They drive vibratory feeders, ensuring accurate and consistent material flow.
- Precise Control: Vibration motors enable precise control over material flow rates, enhancing the efficiency of production processes.
- Reduced Waste: Accurate dosing reduces material waste, improving overall production efficiency and sustainability.
Machinery and Equipment Monitoring
Condition Monitoring
Vibration motors are employed in condition monitoring systems to assess the operational status of machinery and equipment. They provide feedback on vibration levels, helping to identify potential issues before they lead to failures.
- Early Fault Detection: Vibration analysis can detect mechanical faults, such as imbalances, misalignments, and bearing wear, allowing for timely maintenance and repairs.
- Improved Equipment Reliability: Regular monitoring of vibration levels enhances equipment reliability and longevity, reducing downtime and maintenance costs.
Predictive Maintenance
In predictive maintenance applications, vibration motors play a key role in monitoring the health of machinery and equipment. They provide real-time data on vibration patterns, enabling proactive maintenance strategies.
- Reduced Downtime: Predictive maintenance minimizes unexpected equipment failures, reducing downtime and production disruptions.
- Cost Savings: By addressing issues before they escalate, predictive maintenance reduces maintenance costs and extends equipment lifespan.
Mining and Quarrying
Material Transportation
In the mining and quarrying industry, vibration motors are used to transport bulk materials, such as ore and aggregates, over long distances. They drive vibratory feeders and conveyors, ensuring efficient material handling.
- High-Capacity Transportation: Vibration motors enable the transportation of large volumes of materials, increasing production efficiency.
- Durable Solutions: Designed for harsh environments, vibration motors provide reliable performance in demanding mining and quarrying applications.
Screening and Classification
Vibration motors are employed in screening and classification processes to separate materials based on size and composition. They drive vibratory screens and separators, ensuring accurate classification of materials.
- Accurate Classification: Vibration motors provide consistent and controlled vibrations, improving the accuracy of screening and classification operations.
- Adaptable Solutions: Adjustable frequency and amplitude settings allow for tailored solutions to meet specific classification requirements.
Construction Industry
Concrete Compaction
In the construction industry, vibration motors are used to compact concrete, ensuring uniform density and strength. They drive vibratory compactors and tampers, improving the quality of concrete structures.
- Improved Concrete Quality: Vibration motors enhance concrete compaction, reducing voids and increasing structural integrity.
- Efficient Construction Processes: Vibration motors streamline concrete compaction, reducing labor costs and construction time.
Soil Compaction
Vibration motors are employed in soil compaction applications to improve soil stability and load-bearing capacity. They drive vibratory rollers and compactors, enhancing the performance of construction equipment.
- Enhanced Soil Stability: Vibration motors improve soil compaction, increasing stability and load-bearing capacity.
- Versatile Applications: Used in road construction, foundation preparation, and landscaping, vibration motors provide adaptable solutions for various soil compaction needs.
Food and Beverage Industry
Food Processing
In the food and beverage industry, vibration motors are used to enhance food processing operations. They drive vibratory conveyors and feeders, ensuring efficient and hygienic material handling.
- Efficient Material Handling: Vibration motors enable the smooth and consistent flow of food products, reducing processing time and waste.
- Hygienic Solutions: Designed for easy cleaning and maintenance, vibration motors ensure hygienic food processing operations.
Packaging and Sorting
Vibration motors are employed in packaging and sorting applications to enhance efficiency and accuracy. They drive vibratory sorters and packers, ensuring precise material handling.
- Improved Sorting Accuracy: Vibration motors provide controlled vibrations, improving the accuracy of packaging and sorting operations.
- Customizable Solutions: Adjustable frequency and amplitude settings allow for tailored solutions to meet specific packaging and sorting requirements.
Pharmaceutical Industry
Tablet Coating
In the pharmaceutical industry, vibration motors are used to enhance tablet coating processes. They drive vibratory coaters, ensuring uniform coating application.
- Improved Coating Quality: Vibration motors ensure uniform coating thickness, improving the quality and efficacy of pharmaceutical products.
- Efficient Production Processes: Vibration motors streamline tablet coating, reducing production time and costs.
Capsule Filling
Vibration motors are employed in capsule filling applications to enhance accuracy and efficiency. They drive vibratory fillers, ensuring precise material dosing.
- Accurate Dosing: Vibration motors enable precise control over material flow rates, improving the accuracy of capsule filling operations.
- Reduced Waste: Accurate dosing reduces material waste, improving overall production efficiency and sustainability.
In conclusion, industrial vibration motors have diverse applications across various industries, enhancing efficiency, productivity, and quality. Their versatility and adaptability make them essential components in modern industrial processes.
Challenges and Solutions in Industrial Applications
Industrial vibration motors are subject to various challenges that can impact their performance and reliability. This section explores common challenges in industrial applications and potential solutions to address them.
Environmental Considerations
Harsh Operating Conditions
Industrial environments can expose vibration motors to harsh conditions, such as extreme temperatures, moisture, dust, and chemicals. These factors can affect motor performance and longevity.
Solutions:
- Protective Coatings: Apply protective coatings to motor components to resist corrosion and wear.
- Sealed Enclosures: Use sealed enclosures to protect internal components from environmental factors.
- Temperature Management: Implement cooling systems or heat sinks to manage temperature fluctuations.
Vibration-Induced Fatigue
Continuous vibration exposure can lead to fatigue and wear on motor components, reducing their lifespan and reliability.
Solutions:
- Durable Materials: Use high-strength materials and components designed to withstand vibration-induced fatigue.
- Regular Maintenance: Implement routine maintenance schedules to identify and address wear and fatigue early.
- Vibration Dampening: Incorporate vibration dampening techniques, such as shock absorbers or isolators, to reduce stress on components.
Maintenance and Longevity
Wear and Tear
Vibration motors are subject to wear and tear due to constant operation, which can impact performance and reliability.
Solutions:
- High-Quality Components: Use high-quality bearings, seals, and other components to minimize wear and tear.
- Lubrication: Implement proper lubrication practices to reduce friction and extend component lifespan.
- Preventive Maintenance: Conduct regular inspections and maintenance to identify and address potential issues before they escalate.
Unexpected Failures
Unexpected motor failures can lead to downtime and production disruptions, impacting productivity and profitability.
Solutions:
- Condition Monitoring: Implement condition monitoring systems to assess motor health and detect potential issues early.
- Predictive Maintenance: Use predictive maintenance strategies to anticipate and address issues before they result in failures.
- Redundancy: Incorporate redundancy in critical applications to minimize the impact of motor failures.
Noise and Vibration Control
Unwanted Noise
Vibration motors can generate unwanted noise, impacting the working environment and operator comfort.
Solutions:
- Noise Reduction Techniques: Implement noise reduction techniques, such as damping materials or enclosures, to minimize noise levels.
- Precision Engineering: Use precision-engineered components and designs to reduce mechanical noise.
- Isolation: Isolate motors from other components to prevent noise transmission.
Vibration Transmission
Uncontrolled vibration transmission can affect the performance of other equipment and systems, leading to inefficiencies and failures.
Solutions:
- Vibration Isolation: Use vibration isolation techniques, such as mounts or shock absorbers, to reduce vibration transmission.
- Balancing: Ensure motors and components are properly balanced to minimize vibration transmission.
- Control Systems: Implement advanced control systems to manage and adjust vibration characteristics.
In conclusion, industrial vibration motors face various challenges in harsh operating environments. By implementing appropriate solutions, such as protective measures, maintenance strategies, and noise control techniques, these challenges can be effectively addressed, ensuring reliable and efficient motor performance.
Future Trends and Innovations
As technology continues to evolve, industrial vibration motors are expected to undergo significant advancements, leading to new applications and improved performance. This section explores future trends and innovations in vibration motor technology.
Technological Advancements
- Miniaturization: Advances in manufacturing and materials will enable the development of smaller and more compact vibration motors, expanding their use in miniaturized devices and systems.
- Increased Efficiency: Innovations in motor design and materials will lead to more efficient vibration motors with lower power consumption and higher performance.
- Improved Control and Precision: Enhanced control algorithms and sensor integration will enable more precise and customizable vibration effects, improving user experience and application performance.
Integration with Smart Technologies
The integration of vibration motors with the Internet of Things (IoT) and smart technologies will open up new possibilities for applications and functionality.
- Smart Manufacturing: Vibration motors will play a key role in smart manufacturing processes, enabling real-time monitoring and control of production systems.
- Automated Systems: Integration with smart technologies will enhance the performance and efficiency of automated systems, improving productivity and quality.
Emerging Applications
- Advanced Robotics: Vibration motors will enhance the performance of advanced robotic systems, providing precise control and feedback for complex tasks.
- Augmented Reality (AR): Vibration motors will enhance AR experiences by providing tactile feedback for virtual interactions, improving immersion and realism.
- Next-Generation Industrial Equipment: Vibration motors will continue to enhance the performance of next-generation industrial equipment, such as 3D printers and advanced manufacturing systems.
In summary, the future of industrial vibration motors is promising, with advancements in technology and integration with smart systems leading to new applications and improved performance. These innovations will continue to enhance functionality and efficiency across various industries.
Conclusion
Industrial vibration motors are essential components in modern technology, providing crucial functionality and enhancing efficiency across a wide range of applications. This article has explored the various types of vibration motors, their technical aspects, and their diverse applications in industrial settings.
Recap of Key Points
- Types of Vibration Motors: We examined the different types of industrial vibration motors, each with unique characteristics and advantages.
- Technical Aspects: We discussed the construction, key specifications, performance characteristics, and design considerations associated with vibration motors, highlighting the factors that influence their selection and integration.
- Applications: We explored the diverse applications of vibration motors in industries such as material handling, mining, construction, and pharmaceuticals, showcasing their versatility and importance in modern industry.
- Challenges and Solutions: We identified common challenges in industrial applications and provided solutions to address them, ensuring reliable and efficient motor performance.
- Future Trends and Innovations: We examined the future trends and innovations in vibration motor technology, including miniaturization, increased efficiency, and integration with smart technologies.
Importance in Modern Industry
Industrial vibration motors play a crucial role in enhancing efficiency, productivity, and quality across various industries. Their ability to provide precise control, feedback, and motion makes them indispensable components in modern industrial processes.
- Material Handling: Vibration motors improve the efficiency of material handling operations, enhancing productivity and reducing costs.
- Machinery Monitoring: Vibration motors enable real-time monitoring and maintenance of machinery, improving equipment reliability and longevity.
- Diverse Applications: From mining to pharmaceuticals, vibration motors are used in a wide range of applications, highlighting their versatility and adaptability.
Future Outlook
As technology continues to advance, industrial vibration motors will play an increasingly important role in emerging applications and industries. Innovations in design, materials, and integration will lead to new possibilities and improved performance, enhancing functionality and efficiency across various sectors.
In conclusion, industrial vibration motors are vital components in modern technology, providing essential functionality and enhancing efficiency across a wide range of applications. Their versatility, adaptability, and potential for future advancements make them indispensable in the ever-evolving industrial landscape.
Introduction to Energy Consumption
Creating an informative article on energy consumption tips can help individuals and businesses save money, reduce their environmental impact, and improve overall energy efficiency. Below is a comprehensive 5000-word article suitable for a professional website, covering various aspects of energy consumption tips:
Energy consumption refers to the total amount of energy used by individuals, households, businesses, and industries. It encompasses various forms of energy, including electricity, natural gas, oil, and renewable energy sources. Understanding energy consumption is crucial for identifying areas where efficiency can be improved, leading to cost savings and environmental benefits.
Key aspects of energy consumption include:
- Types of Energy: Energy consumption can be categorized into different types, such as electrical, thermal, and mechanical energy, each serving specific purposes in daily activities and industrial processes.
- Energy Sources: Energy can be derived from various sources, including fossil fuels (coal, oil, natural gas) and renewable sources (solar, wind, geothermal, biomass, hydroelectric).
- Energy Demand: The demand for energy varies based on factors such as population, economic activity, climate, and technological advancements.
Importance of Energy Efficiency
Energy efficiency involves using less energy to perform the same tasks, reducing energy waste, and minimizing the environmental impact of energy use. It is a critical component of sustainable development and offers numerous benefits:
- Cost Savings: Improving energy efficiency can lead to significant reductions in energy bills, benefiting households, businesses, and industries.
- Environmental Benefits: Reducing energy consumption decreases greenhouse gas emissions and reduces reliance on fossil fuels, mitigating the impact of climate change.
- Resource Conservation: Energy efficiency helps conserve natural resources by reducing the demand for energy production and consumption.
- Energy Security: Efficient energy use reduces dependence on imported energy sources, enhancing energy security and resilience.
Benefits of Reducing Energy Consumption
Reducing energy consumption offers a wide range of benefits, including:
- Financial Savings: Lower energy bills result in financial savings for individuals, businesses, and governments, freeing up resources for other investments and initiatives.
- Environmental Protection: Reduced energy consumption leads to lower emissions of pollutants and greenhouse gases, contributing to cleaner air and a healthier environment.
- Improved Comfort and Quality of Life: Energy-efficient homes and buildings provide enhanced comfort, improved indoor air quality, and better thermal regulation.
- Economic Growth: Energy efficiency can drive economic growth by creating jobs in energy-efficient technologies, renewable energy, and sustainable practices.
Key takeaways:
- Essential for Sustainability: Energy consumption is a critical aspect of modern life, and improving energy efficiency is essential for achieving sustainable development.
- Diverse Benefits: Reducing energy consumption offers financial, environmental, and social benefits, making it a valuable goal for individuals, businesses, and governments.
Energy Consumption in Residential Settings
Improving energy efficiency in residential settings can lead to significant cost savings and environmental benefits. This section explores various strategies for reducing energy consumption in homes.
Heating and Cooling
Heating and cooling account for a significant portion of residential energy consumption. Implementing energy-efficient practices can lead to substantial savings:
- Programmable Thermostats: Use programmable thermostats to set specific temperature schedules, reducing energy use when heating or cooling is not needed.
- Example: Set the thermostat to lower the temperature during the winter when you’re asleep or away from home and raise it during the summer.
- Benefits: Reduces energy consumption by adjusting heating and cooling based on occupancy patterns.
- Regular Maintenance: Ensure heating and cooling systems are regularly maintained, including cleaning filters, checking ducts, and servicing equipment.
- Example: Schedule annual maintenance for HVAC systems to ensure they operate efficiently.
- Benefits: Increases the efficiency and lifespan of heating and cooling equipment.
- Insulation and Sealing: Improve insulation in walls, attics, and basements, and seal gaps and cracks to prevent air leaks.
- Example: Use weatherstripping to seal doors and windows, and add insulation to attics.
- Benefits: Reduces heat loss in winter and heat gain in summer, improving comfort and reducing energy use.
- Energy-Efficient HVAC Systems: Consider upgrading to energy-efficient heating and cooling systems, such as heat pumps and high-efficiency furnaces.
- Example: Replace an older furnace with an ENERGY STAR-certified model that offers improved efficiency.
- Benefits: Reduces energy consumption and provides consistent heating and cooling performance.
Lighting
Lighting is another significant contributor to residential energy consumption. Implementing energy-efficient lighting solutions can lead to substantial savings:
- LED Lighting: Replace incandescent and fluorescent bulbs with LED lighting, which uses less energy and lasts longer.
- Example: Install LED bulbs in all light fixtures, including lamps, overhead lights, and outdoor lighting.
- Benefits: Reduces energy consumption, lowers electricity bills, and reduces the need for frequent bulb replacements.
- Motion Sensors and Timers: Use motion sensors and timers to automatically turn off lights when not in use, reducing energy waste.
- Example: Install motion sensors in areas with infrequent use, such as hallways and closets, to automatically turn off lights.
- Benefits: Eliminates energy waste by ensuring lights are only on when needed.
- Natural Lighting: Maximize natural lighting by using windows, skylights, and light-colored interior finishes to reduce the need for artificial lighting.
- Example: Use sheer curtains to allow natural light to enter while maintaining privacy.
- Benefits: Reduces the need for artificial lighting, improving energy efficiency and enhancing indoor ambiance.
- Lighting Controls: Implement lighting controls, such as dimmers and smart switches, to adjust lighting levels based on needs and preferences.
- Example: Install dimmer switches in living areas to control lighting intensity and create desired atmospheres.
- Benefits: Allows for customized lighting levels, reducing energy consumption and enhancing comfort.
Appliances and Electronics
Appliances and electronics account for a significant portion of residential energy use. Implementing energy-efficient practices can lead to significant savings:
- ENERGY STAR Appliances: Choose ENERGY STAR-certified appliances, which meet strict energy efficiency standards and consume less energy.
- Example: Replace an old refrigerator with an ENERGY STAR-certified model that offers improved efficiency.
- Benefits: Reduces energy consumption and lowers electricity bills.
- Unplugging Devices: Unplug devices and chargers when not in use to eliminate standby power consumption, also known as “phantom load.”
- Example: Use power strips to easily turn off multiple devices and chargers when not in use.
- Benefits: Reduces energy waste and lowers electricity bills.
- Efficient Use of Appliances: Use appliances efficiently, such as running full loads in dishwashers and washing machines and using cold water for laundry.
- Example: Wash clothes in cold water and air dry when possible to save energy.
- Benefits: Reduces energy consumption and extends the lifespan of appliances.
- Smart Home Technology: Implement smart home technology to monitor and control appliance energy use, allowing for optimized energy management.
- Example: Use a smart thermostat to control heating and cooling remotely and adjust settings based on occupancy patterns.
- Benefits: Provides greater control over energy use, reducing waste and lowering energy bills.
Water Heating
Water heating is another significant contributor to residential energy consumption. Implementing energy-efficient practices can lead to substantial savings:
- Tankless Water Heaters: Consider installing tankless water heaters, which provide hot water on demand and eliminate standby energy loss.
- Example: Replace a traditional tank water heater with a tankless model that heats water as needed.
- Benefits: Reduces energy consumption and provides continuous hot water supply.
- Water Heater Insulation: Insulate water heater tanks and pipes to reduce heat loss and improve efficiency.
- Example: Install a water heater blanket and insulate hot water pipes to minimize heat loss.
- Benefits: Reduces energy consumption and improves water heating efficiency.
- Lower Water Heater Temperature: Set the water heater temperature to 120°F (49°C) to reduce energy use while maintaining comfort.
- Example: Adjust the thermostat on the water heater to maintain a temperature of 120°F.
- Benefits: Reduces energy consumption and lowers the risk of scalding.
- Efficient Water Use: Implement water-saving practices, such as shorter showers and using low-flow fixtures, to reduce hot water demand.
- Example: Install low-flow showerheads and faucets to reduce water use without sacrificing comfort.
- Benefits: Reduces hot water demand and lowers energy consumption.
Insulation and Sealing
Improving insulation and sealing in residential settings can lead to significant energy savings:
- Wall and Attic Insulation: Improve insulation in walls, attics, and basements to reduce heat loss and gain.
- Example: Add insulation to attics and walls to improve thermal performance and comfort.
- Benefits: Reduces heating and cooling energy consumption and improves indoor comfort.
- Window and Door Sealing: Seal gaps and cracks around windows and doors to prevent air leaks and improve energy efficiency.
- Example: Use weatherstripping and caulking to seal gaps around windows and doors.
- Benefits: Reduces heat loss and gain, improving energy efficiency and comfort.
- Energy-Efficient Windows: Consider upgrading to energy-efficient windows with low-emissivity (Low-E) coatings and double glazing.
- Example: Replace single-pane windows with ENERGY STAR-certified double-pane windows.
- Benefits: Reduces heat transfer and improves energy efficiency.
- Roof and Floor Insulation: Improve insulation in roofs and floors to reduce heat loss and gain, enhancing energy efficiency.
- Example: Install reflective roof coatings and floor insulation to improve thermal performance.
- Benefits: Reduces heating and cooling energy consumption and improves comfort.
In summary, improving energy efficiency in residential settings involves a combination of technology, practices, and behavioral changes. By implementing energy-efficient solutions for heating, cooling, lighting, appliances, and insulation, homeowners can achieve significant cost savings and environmental benefits.
Energy Consumption in Commercial and Industrial Settings
Improving energy efficiency in commercial and industrial settings can lead to significant cost savings, enhanced productivity, and environmental benefits. This section explores various strategies for reducing energy consumption in businesses and industries.
Energy Audits and Monitoring
Conducting energy audits and implementing energy monitoring systems are essential steps in identifying areas for improvement and optimizing energy use.
- Energy Audits: Conduct comprehensive energy audits to assess energy use, identify inefficiencies, and recommend improvements.
- Example: Hire a professional energy auditor to evaluate energy use in a commercial building and provide recommendations for efficiency improvements.
- Benefits: Identifies areas for improvement and provides actionable insights for reducing energy consumption.
- Energy Monitoring Systems: Implement energy monitoring systems to track energy use in real-time, identify trends, and optimize energy management.
- Example: Use energy monitoring software to track electricity use in an industrial facility and identify peak demand periods.
- Benefits: Provides real-time data for informed decision-making and energy optimization.
- Benchmarking: Compare energy use against industry benchmarks and standards to identify areas for improvement and set energy efficiency goals.
- Example: Use energy benchmarking tools to compare energy use in a commercial building against similar facilities.
- Benefits: Identifies opportunities for improvement and sets achievable energy efficiency goals.
- Continuous Improvement: Implement a continuous improvement process for energy management, regularly reviewing energy use and implementing efficiency measures.
- Example: Establish an energy management team to review energy use, set goals, and implement efficiency measures.
- Benefits: Ensures ongoing optimization of energy use and continuous improvement.
Lighting Solutions
Implementing energy-efficient lighting solutions can lead to substantial savings in commercial and industrial settings.
- LED Lighting: Replace traditional lighting with LED lighting, which uses less energy and lasts longer.
- Example: Install LED lighting in offices, warehouses, and manufacturing facilities to reduce energy consumption.
- Benefits: Reduces energy consumption, lowers electricity bills, and reduces maintenance costs.
- Daylighting: Maximize natural daylighting through the use of windows, skylights, and light tubes, reducing the need for artificial lighting.
- Example: Install skylights in warehouses and manufacturing facilities to maximize natural lighting.
- Benefits: Reduces the need for artificial lighting, improving energy efficiency and enhancing indoor ambiance.
- Lighting Controls: Implement lighting controls, such as motion sensors, timers, and dimmers, to optimize lighting use based on occupancy and needs.
- Example: Install motion sensors in restrooms and storage areas to automatically turn off lights when not in use.
- Benefits: Eliminates energy waste and reduces electricity bills.
- Task Lighting: Use task lighting in workspaces to provide focused illumination, reducing the need for overhead lighting.
- Example: Install task lighting at individual workstations to provide focused illumination for tasks.
- Benefits: Reduces overall lighting energy consumption and enhances comfort.
HVAC Systems
Heating, ventilation, and air conditioning (HVAC) systems are major contributors to energy consumption in commercial and industrial settings. Implementing energy-efficient HVAC solutions can lead to substantial savings:
- Regular Maintenance: Ensure HVAC systems are regularly maintained, including cleaning filters, checking ducts, and servicing equipment.
- Example: Schedule annual maintenance for HVAC systems to ensure they operate efficiently.
- Benefits: Increases the efficiency and lifespan of HVAC equipment.
- Energy-Efficient HVAC Systems: Upgrade to energy-efficient HVAC systems, such as high-efficiency heat pumps, chillers, and boilers.
- Example: Replace an older HVAC system with an ENERGY STAR-certified model that offers improved efficiency.
- Benefits: Reduces energy consumption and provides consistent heating and cooling performance.
- Zoning Systems: Implement zoning systems to control heating and cooling in specific areas, reducing energy use in unoccupied or low-traffic areas.
- Example: Use zoning controls to manage temperature settings in different areas of a building based on occupancy patterns.
- Benefits: Reduces energy consumption and enhances comfort.
- Building Automation Systems: Use building automation systems to monitor and control HVAC systems, optimizing energy use based on occupancy and needs.
- Example: Implement a building automation system to adjust HVAC settings based on occupancy patterns and external conditions.
- Benefits: Provides greater control over energy use, reducing waste and lowering energy bills.
Industrial Equipment and Machinery
Improving the energy efficiency of industrial equipment and machinery can lead to significant cost savings and environmental benefits.
- Variable Frequency Drives (VFDs): Install variable frequency drives on motors to optimize speed and reduce energy consumption.
- Example: Install VFDs on pumps and fans to adjust motor speed based on demand.
- Benefits: Reduces energy consumption and extends the lifespan of motors.
- Energy-Efficient Motors: Upgrade to energy-efficient motors that consume less energy and provide improved performance.
- Example: Replace older motors with high-efficiency models that meet or exceed industry standards.
- Benefits: Reduces energy consumption and lowers maintenance costs.
- Compressed Air Systems: Optimize compressed air systems to reduce energy consumption and improve efficiency.
- Example: Conduct regular maintenance, repair leaks, and adjust pressure settings in compressed air systems.
- Benefits: Reduces energy waste and improves system performance.
- Efficient Use of Equipment: Implement practices to optimize the use of industrial equipment, such as scheduling maintenance during off-peak hours and reducing idle time.
- Example: Schedule equipment maintenance during off-peak hours to reduce energy demand.
- Benefits: Reduces energy consumption and enhances equipment performance.
Office Equipment and Electronics
Improving the energy efficiency of office equipment and electronics can lead to significant savings in commercial settings.
- ENERGY STAR Office Equipment: Choose ENERGY STAR-certified office equipment, which meets strict energy efficiency standards and consumes less energy.
- Example: Replace older printers and copiers with ENERGY STAR-certified models that offer improved efficiency.
- Benefits: Reduces energy consumption and lowers electricity bills.
- Power Management Features: Use power management features on computers, monitors, and other electronics to reduce energy consumption during periods of inactivity.
- Example: Enable sleep mode on computers and monitors to reduce energy use during periods of inactivity.
- Benefits: Reduces energy waste and extends the lifespan of electronics.
- Unplugging Devices: Unplug devices and chargers when not in use to eliminate standby power consumption, also known as “phantom load.”
- Example: Use power strips to easily turn off multiple devices and chargers when not in use.
- Benefits: Reduces energy waste and lowers electricity bills.
- Efficient Use of Equipment: Implement practices to optimize the use of office equipment, such as using digital documents instead of printing and turning off equipment when not in use.
- Example: Use digital documents and cloud storage to reduce paper use and energy consumption.
- Benefits: Reduces energy consumption and lowers operating costs.
In summary, improving energy efficiency in commercial and industrial settings involves a combination of technology, practices, and behavioral changes. By implementing energy-efficient solutions for lighting, HVAC, equipment, and electronics, businesses can achieve significant cost savings and environmental benefits.
Renewable Energy Solutions
Incorporating renewable energy solutions can significantly reduce energy consumption, lower carbon emissions, and promote sustainability. This section explores various renewable energy solutions and their benefits.
Solar Power
Solar power is a clean and renewable energy source that harnesses the sun’s energy to generate electricity and heat. It is a versatile solution that can be used in residential, commercial, and industrial settings.
Solar Photovoltaic (PV) Systems
Solar PV systems convert sunlight into electricity using solar panels. They can be installed on rooftops, ground-mounted systems, or integrated into building designs.
Benefits of Solar PV Systems:
- Clean Energy: Solar PV systems produce electricity without emitting greenhouse gases or pollutants.
- Cost Savings: Solar PV systems can reduce or eliminate electricity bills, leading to significant cost savings over time.
- Energy Independence: Solar PV systems provide energy independence by reducing reliance on grid electricity.
- Scalability: Solar PV systems can be scaled to meet the energy needs of various applications, from small residential systems to large commercial installations.
Solar Thermal Systems
Solar thermal systems use sunlight to heat water or air for residential, commercial, and industrial applications. They are commonly used for water heating, space heating, and industrial processes.
Benefits of Solar Thermal Systems:
- Energy Efficiency: Solar thermal systems are highly efficient, converting a large portion of sunlight into usable heat.
- Cost Savings: Solar thermal systems can reduce or eliminate heating bills, leading to significant cost savings over time.
- Environmental Benefits: Solar thermal systems produce heat without emitting greenhouse gases or pollutants.
- Versatility: Solar thermal systems can be used for various applications, including domestic hot water, space heating, and industrial processes.
Wind Energy
Wind energy is a clean and renewable energy source that harnesses the power of the wind to generate electricity. It is a cost-effective solution for reducing energy consumption and promoting sustainability.
Wind Turbines
Wind turbines convert the kinetic energy of the wind into electricity. They can be installed in various settings, including onshore and offshore wind farms.
Benefits of Wind Energy:
- Clean Energy: Wind turbines produce electricity without emitting greenhouse gases or pollutants.
- Cost-Effective: Wind energy is one of the most cost-effective renewable energy sources, offering competitive electricity prices.
- Energy Independence: Wind energy provides energy independence by reducing reliance on fossil fuels and grid electricity.
- Scalability: Wind energy can be scaled to meet the energy needs of various applications, from small residential systems to large utility-scale installations.
Geothermal Heating and Cooling
Geothermal heating and cooling systems use the stable temperature of the earth to provide efficient heating and cooling for residential, commercial, and industrial applications.
Ground Source Heat Pumps (GSHPs)
Ground source heat pumps use the earth’s temperature to transfer heat to or from a building, providing efficient heating and cooling.
Benefits of Geothermal Heating and Cooling:
- Energy Efficiency: Geothermal systems are highly efficient, reducing energy consumption and operating costs.
- Environmental Benefits: Geothermal systems produce heating and cooling without emitting greenhouse gases or pollutants.
- Versatility: Geothermal systems can be used for various applications, including residential heating and cooling, commercial HVAC systems, and industrial processes.
- Longevity: Geothermal systems have a long lifespan, providing reliable performance for decades.
Biomass Energy
Biomass energy is a renewable energy source that uses organic materials, such as wood, agricultural residues, and waste, to generate heat and electricity.
Biomass Boilers
Biomass boilers use organic materials as fuel to produce heat for residential, commercial, and industrial applications.
Benefits of Biomass Energy:
- Renewable Resource: Biomass energy uses renewable organic materials, reducing reliance on fossil fuels.
- Carbon Neutral: Biomass energy is considered carbon neutral, as the carbon dioxide released during combustion is offset by the carbon dioxide absorbed by plants during growth.
- Waste Reduction: Biomass energy can use waste materials as fuel, reducing waste and promoting sustainability.
- Versatility: Biomass energy can be used for various applications, including residential heating, industrial processes, and electricity generation.
Hydroelectric Power
Hydroelectric power is a renewable energy source that uses the flow of water to generate electricity. It is a reliable and cost-effective solution for reducing energy consumption and promoting sustainability.
Hydroelectric Dams
Hydroelectric dams use the flow of water from rivers or reservoirs to generate electricity. They are commonly used for large-scale electricity generation.
Benefits of Hydroelectric Power:
- Clean Energy: Hydroelectric power produces electricity without emitting greenhouse gases or pollutants.
- Reliable Source: Hydroelectric power is a reliable and consistent source of electricity, providing stable power generation.
- Cost-Effective: Hydroelectric power offers competitive electricity prices, making it one of the most cost-effective renewable energy sources.
- Energy Independence: Hydroelectric power provides energy independence by reducing reliance on fossil fuels and grid electricity.
In summary, incorporating renewable energy solutions can significantly reduce energy consumption, lower carbon emissions, and promote sustainability. By utilizing solar power, wind energy, geothermal heating and cooling, biomass energy, and hydroelectric power, individuals and businesses can achieve significant environmental and economic benefits.
Smart Technology and Energy Management
Smart technology and energy management solutions can significantly improve energy efficiency, reduce energy consumption, and enhance control over energy use. This section explores various smart technologies and their benefits.
Smart Meters
Smart meters are advanced metering devices that provide real-time data on energy use, allowing for better monitoring and control of energy consumption.
Benefits of Smart Meters:
- Real-Time Data: Smart meters provide real-time data on energy use, allowing for informed decision-making and energy optimization.
- Energy Savings: Smart meters enable consumers to identify energy waste and implement efficiency measures, leading to cost savings.
- Enhanced Control: Smart meters provide greater control over energy use, allowing consumers to adjust consumption based on real-time data.
- Demand Response: Smart meters facilitate demand response programs, allowing utilities to manage peak demand and reduce energy costs.
Home Automation Systems
Home automation systems integrate smart devices and appliances, allowing for centralized control and monitoring of energy use.
Benefits of Home Automation Systems:
- Convenience: Home automation systems provide centralized control over lighting, heating, cooling, and appliances, enhancing convenience and comfort.
- Energy Efficiency: Home automation systems optimize energy use by adjusting settings based on occupancy patterns and preferences.
- Remote Monitoring: Home automation systems provide remote monitoring and control, allowing consumers to manage energy use from anywhere.
- Security: Home automation systems enhance security by integrating smart locks, cameras, and alarms, providing peace of mind.
Energy Management Software
Energy management software provides tools for monitoring, analyzing, and optimizing energy use in residential, commercial, and industrial settings.
Benefits of Energy Management Software:
- Data Analysis: Energy management software provides data analysis tools for identifying trends, inefficiencies, and opportunities for improvement.
- Customizable Solutions: Energy management software offers customizable solutions to meet specific energy management needs and goals.
- Real-Time Monitoring: Energy management software provides real-time monitoring and alerts, enabling proactive energy management.
- Cost Savings: Energy management software helps identify and implement efficiency measures, leading to significant cost savings.
IoT Devices for Energy Efficiency
Internet of Things (IoT) devices provide advanced monitoring and control of energy use, enhancing efficiency and sustainability.
Benefits of IoT Devices:
- Enhanced Monitoring: IoT devices provide real-time data on energy use, enabling informed decision-making and optimization.
- Remote Control: IoT devices provide remote control and monitoring, allowing consumers to manage energy use from anywhere.
- Integration: IoT devices integrate with smart home systems, enhancing convenience and control.
- Energy Savings: IoT devices enable consumers to identify and eliminate energy waste, leading to significant cost savings.
Demand Response Systems
Demand response systems enable utilities and consumers to manage energy use during peak demand periods, reducing energy costs and improving grid reliability.
Benefits of Demand Response Systems:
- Cost Savings: Demand response systems reduce energy costs by shifting consumption to off-peak periods.
- Grid Reliability: Demand response systems improve grid reliability by reducing peak demand and preventing outages.
- Environmental Benefits: Demand response systems reduce the need for additional power generation, lowering emissions and environmental impact.
- Enhanced Control: Demand response systems provide consumers with greater control over energy use, allowing for more efficient consumption.
In summary, smart technology and energy management solutions provide advanced tools for improving energy efficiency, reducing energy consumption, and enhancing control over energy use. By implementing smart meters, home automation systems, energy management software, IoT devices, and demand response systems, individuals and businesses can achieve significant cost savings and environmental benefits.
Behavioral Changes for Energy Savings

Behavioral changes can have a significant impact on energy consumption, leading to cost savings and environmental benefits. This section explores various behavioral changes that can reduce energy use.
Energy-Saving Habits
Adopting energy-saving habits can lead to significant reductions in energy consumption:
- Turning Off Lights: Turn off lights when leaving a room to reduce energy waste.
- Example: Develop a habit of turning off lights when exiting a room, even for short periods.
- Benefits: Reduces energy consumption and lowers electricity bills.
- Unplugging Devices: Unplug devices and chargers when not in use to eliminate standby power consumption, also known as “phantom load.”
- Example: Use power strips to easily turn off multiple devices and chargers when not in use.
- Benefits: Reduces energy waste and lowers electricity bills.
- Adjusting Thermostat Settings: Adjust thermostat settings based on occupancy patterns and preferences to reduce energy use.
- Example: Set the thermostat to lower temperatures during the winter and higher temperatures during the summer when the home is unoccupied.
- Benefits: Reduces energy consumption and lowers heating and cooling bills.
- Using Energy-Efficient Transportation: Choose energy-efficient transportation options, such as walking, biking, carpooling, or using public transit.
- Example: Use public transit for commuting to work instead of driving a personal vehicle.
- Benefits: Reduces energy consumption and lowers transportation costs.
Encouraging Energy Awareness
Promoting energy awareness can lead to increased energy efficiency and sustainability:
- Educating Employees: Educate employees about energy efficiency and encourage energy-saving practices in the workplace.
- Example: Conduct energy efficiency workshops and training sessions for employees.
- Benefits: Increases energy awareness and promotes energy-saving practices.
- Setting Energy Goals: Set energy efficiency goals and track progress to encourage energy-saving practices.
- Example: Set a goal to reduce energy consumption by a specific percentage over a set period and track progress regularly.
- Benefits: Encourages energy-saving practices and promotes continuous improvement.
- Incentivizing Energy Efficiency: Provide incentives for energy-saving practices, such as rewards for meeting energy efficiency goals.
- Example: Offer rewards or recognition for employees or departments that achieve energy efficiency goals.
- Benefits: Motivates individuals and teams to adopt energy-saving practices.
- Promoting Energy Conservation: Promote energy conservation through awareness campaigns, workshops, and educational materials.
- Example: Develop educational materials and campaigns to promote energy conservation in the community.
- Benefits: Raises awareness about energy efficiency and encourages energy-saving practices.
Reducing Energy Waste
Reducing energy waste can lead to significant cost savings and environmental benefits:
- Fixing Leaks: Fix leaks in pipes, faucets, and ducts to prevent energy waste.
- Example: Regularly inspect and repair leaks in plumbing and HVAC systems.
- Benefits: Reduces energy waste and lowers energy bills.
- Optimizing Equipment Use: Use equipment efficiently, such as running full loads in dishwashers and washing machines and using cold water for laundry.
- Example: Wash clothes in cold water and air dry when possible to save energy.
- Benefits: Reduces energy consumption and extends the lifespan of equipment.
- Regular Maintenance: Ensure equipment and systems are regularly maintained to optimize performance and efficiency.
- Example: Schedule regular maintenance for HVAC systems, appliances, and vehicles.
- Benefits: Improves energy efficiency and extends the lifespan of equipment.
- Efficient Use of Resources: Implement practices to optimize the use of resources, such as using digital documents instead of printing and turning off equipment when not in use.
- Example: Use digital documents and cloud storage to reduce paper use and energy consumption.
- Benefits: Reduces energy consumption and lowers operating costs.
In summary, behavioral changes can have a significant impact on energy consumption, leading to cost savings and environmental benefits. By adopting energy-saving habits, promoting energy awareness, and reducing energy waste, individuals and businesses can achieve significant energy efficiency and sustainability.
Conclusion
Energy consumption is a critical aspect of modern life, and improving energy efficiency is essential for achieving sustainable development. This article has explored various strategies for reducing energy consumption in residential, commercial, and industrial settings.
Recap of Key Points
- Residential Energy Efficiency: We explored energy-efficient solutions for heating, cooling, lighting, appliances, and insulation, highlighting the importance of technology, practices, and behavioral changes.
- Commercial and Industrial Energy Efficiency: We discussed energy-efficient solutions for lighting, HVAC, equipment, and electronics, emphasizing the importance of energy audits, monitoring, and management.
- Renewable Energy Solutions: We examined the benefits of incorporating renewable energy solutions, such as solar power, wind energy, geothermal heating and cooling, biomass energy, and hydroelectric power.
- Smart Technology and Energy Management: We explored the benefits of smart technology and energy management solutions, including smart meters, home automation systems, energy management software, IoT devices, and demand response systems.
- Behavioral Changes for Energy Savings: We discussed the importance of adopting energy-saving habits, promoting energy awareness, and reducing energy waste to achieve significant energy efficiency and sustainability.
The Role of Individuals and Businesses
Individuals and businesses play a crucial role in reducing energy consumption and promoting sustainability. By implementing energy-efficient solutions and adopting energy-saving practices, individuals and businesses can achieve significant cost savings, reduce environmental impact, and contribute to a more sustainable future.
Key actions for individuals and businesses:
- Invest in Energy Efficiency: Invest in energy-efficient technologies and solutions to reduce energy consumption and lower operating costs.
- Promote Energy Awareness: Promote energy awareness and education to encourage energy-saving practices and behaviors.
- Set Energy Efficiency Goals: Set energy efficiency goals and track progress to drive continuous improvement and sustainability.
Future Outlook
As technology continues to evolve, new opportunities for improving energy efficiency and sustainability will emerge. Advancements in renewable energy, smart technology, and energy management will drive further improvements in energy efficiency and provide new solutions for reducing energy consumption.
Future trends in energy efficiency:
- Renewable Energy Integration: The integration of renewable energy solutions will continue to expand, providing clean and sustainable energy for various applications.
- Smart Technology Advancements: Advancements in smart technology and energy management will provide new tools and solutions for optimizing energy use and reducing energy waste.
- Sustainable Practices: The adoption of sustainable practices and behaviors will continue to grow, driving further improvements in energy efficiency and sustainability.
In conclusion, energy consumption is a critical aspect of modern life, and improving energy efficiency is essential for achieving sustainable development. By implementing energy-efficient solutions, adopting energy-saving practices, and promoting sustainability, individuals and businesses can achieve significant energy efficiency and environmental benefits, contributing to a more sustainable future.
EMS Power Machines
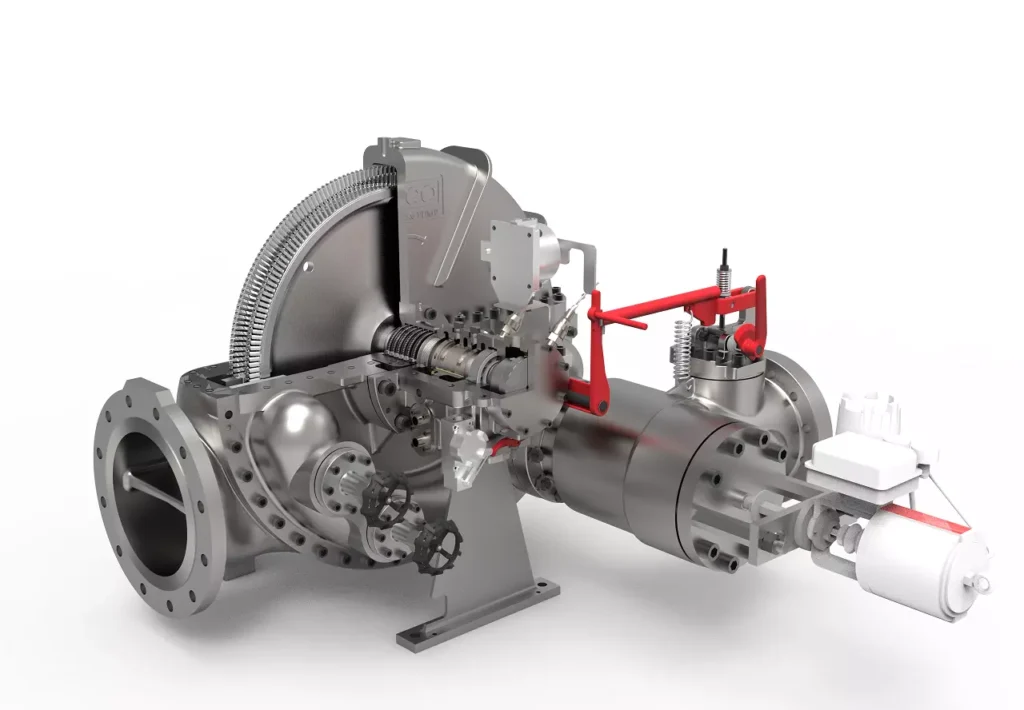
We design, manufacture and assembly Power Machines such as – diesel generators, electric motors, vibration motors, pumps, steam engines and steam turbines
EMS Power Machines is a global power engineering company, one of the five world leaders in the industry in terms of installed equipment. The companies included in the company have been operating in the energy market for more than 60 years.
EMS Power Machines manufactures steam turbines, gas turbines, hydroelectric turbines, generators, and other power equipment for thermal, nuclear, and hydroelectric power plants, as well as for various industries, transport, and marine energy.
EMS Power Machines is a major player in the global power industry, and its equipment is used in power plants all over the world. The company has a strong track record of innovation, and it is constantly developing new and improved technologies.
Here are some examples of Power Machines’ products and services:
- Steam turbines for thermal and nuclear power plants
- Gas turbines for combined cycle power plants and industrial applications
- Hydroelectric turbines for hydroelectric power plants
- Generators for all types of power plants
- Boilers for thermal power plants
- Condensers for thermal power plants
- Reheaters for thermal power plants
- Air preheaters for thermal power plants
- Feedwater pumps for thermal power plants
- Control systems for power plants
- Maintenance and repair services for power plants
EMS Power Machines is committed to providing its customers with high-quality products and services. The company has a strong reputation for reliability and innovation. Power Machines is a leading provider of power equipment and services, and it plays a vital role in the global power industry.
EMS Power Machines, which began in 1961 as a small factory of electric motors, has become a leading global supplier of electronic products for different segments. The search for excellence has resulted in the diversification of the business, adding to the electric motors products which provide from power generation to more efficient means of use.