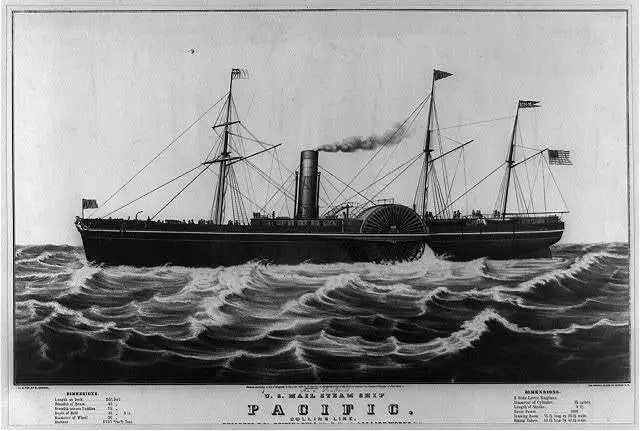
Vessels with Steam Turbine Propulsion: Steam turbine propulsion has been a cornerstone of marine engineering for much of the 20th century, playing a pivotal role in the evolution of naval and commercial shipping. This propulsion method leverages the thermodynamic principles of steam to convert thermal energy into mechanical work, which drives the vessel’s propellers. The steam turbine system typically begins with a boiler, where water is heated to produce high-pressure steam. This steam is then channeled into turbines, which consist of a series of blades attached to a rotor. As the steam passes through the turbine blades, it expands and cools, transferring its energy to the blades and causing the rotor to spin.
This spinning rotor is connected to the ship’s propeller shaft, and the rotational energy generated by the turbine is transmitted to the propeller, propelling the ship forward. One of the key advantages of steam turbine propulsion is its ability to generate substantial power output, making it suitable for large vessels such as ocean liners, warships, and large cargo ships. The propulsion system is also relatively smooth and efficient, with fewer moving parts compared to reciprocating engines, leading to lower maintenance requirements and higher reliability.
However, steam turbine propulsion is not without its drawbacks. The system requires significant space and weight for the boilers, turbines, condensers, and associated piping. Moreover, the process of generating steam and maintaining the required pressure and temperature levels demands a considerable amount of fuel, leading to high operational costs. Despite these challenges, the robustness and power output of steam turbines made them the propulsion method of choice for many naval vessels and commercial ships, particularly in the first half of the 20th century.
Over time, advancements in technology and the advent of more efficient propulsion systems, such as diesel engines and gas turbines, have led to a decline in the use of steam turbines in modern shipping. These newer systems offer higher fuel efficiency, lower emissions, and more compact designs, making them more suitable for contemporary maritime needs. Nevertheless, steam turbines still hold a significant place in maritime history and continue to be used in certain applications, such as nuclear-powered ships, where the heat generated by nuclear reactors is used to produce steam.
The legacy of steam turbine propulsion is evident in its impact on ship design, engineering practices, and the evolution of marine technology. The principles of thermodynamics, fluid dynamics, and materials science that underpin steam turbine technology continue to influence modern engineering disciplines, highlighting the enduring importance of this propulsion system in the history of maritime transportation.
Vessels with Steam Turbine Propulsion
Despite the decline in their prevalence, steam turbines remain relevant in specific contexts, notably in naval vessels and certain large commercial ships where their unique advantages are still valuable. In the military domain, steam turbines are often used in aircraft carriers and submarines, particularly those with nuclear propulsion. The reason for this is the inherent compatibility between nuclear reactors and steam turbines. Nuclear reactors generate heat through nuclear fission, and this heat is used to produce steam, which then drives the turbines. This combination allows for extended periods of operation without refueling, a critical requirement for naval operations.
In commercial shipping, steam turbines are sometimes employed in liquefied natural gas (LNG) carriers. These ships often utilize boil-off gas from the LNG cargo as a fuel source to generate steam, which then drives the turbines. This approach provides a practical solution for managing boil-off gas while also powering the ship, demonstrating the versatility of steam turbine systems in specialized applications.
One of the key engineering challenges in steam turbine propulsion systems is the management of condensate. After steam has passed through the turbine and transferred its energy, it condenses back into water in the condenser. Efficiently recovering and recycling this condensate is crucial for maintaining system efficiency and preventing water loss. Advanced condensate management systems have been developed to maximize the recovery of water and ensure that it is returned to the boiler for reuse, thereby improving the overall efficiency of the propulsion system.
Another aspect of steam turbine propulsion is the design and material selection for turbine blades. These blades operate under extreme conditions of high temperature and pressure, and they must withstand significant mechanical stress. Materials used in turbine blade manufacturing are often specially engineered alloys designed to resist thermal creep and corrosion. The design of the blades is also critical, with aerodynamic profiles optimized to maximize the efficiency of steam expansion and energy transfer.
In the context of environmental impact, steam turbine propulsion systems have traditionally been less efficient than modern alternatives, leading to higher fuel consumption and greater emissions. However, advancements in boiler technology, combined with the development of more efficient turbines and better insulation materials, have mitigated some of these concerns. Additionally, regulatory pressures have driven the adoption of cleaner fuels and more stringent emissions controls in maritime operations, further influencing the evolution of steam turbine technology.
The historical significance of steam turbines in marine propulsion cannot be overstated. They represented a significant technological leap from the earlier era of sail and reciprocating steam engines, enabling faster, larger, and more powerful ships. This technological advancement facilitated global trade, naval supremacy, and the expansion of maritime transportation networks, shaping the modern world in profound ways.
In summary, while steam turbine propulsion may no longer be the dominant force in maritime propulsion, its legacy endures. The systems that once powered the largest and most formidable vessels on the seas continue to inform and inspire contemporary engineering practices. The story of steam turbine propulsion is one of innovation, adaptation, and enduring impact, reflecting the broader narrative of human ingenuity in harnessing the power of steam to conquer the oceans.
Steam Turbine Propulsion
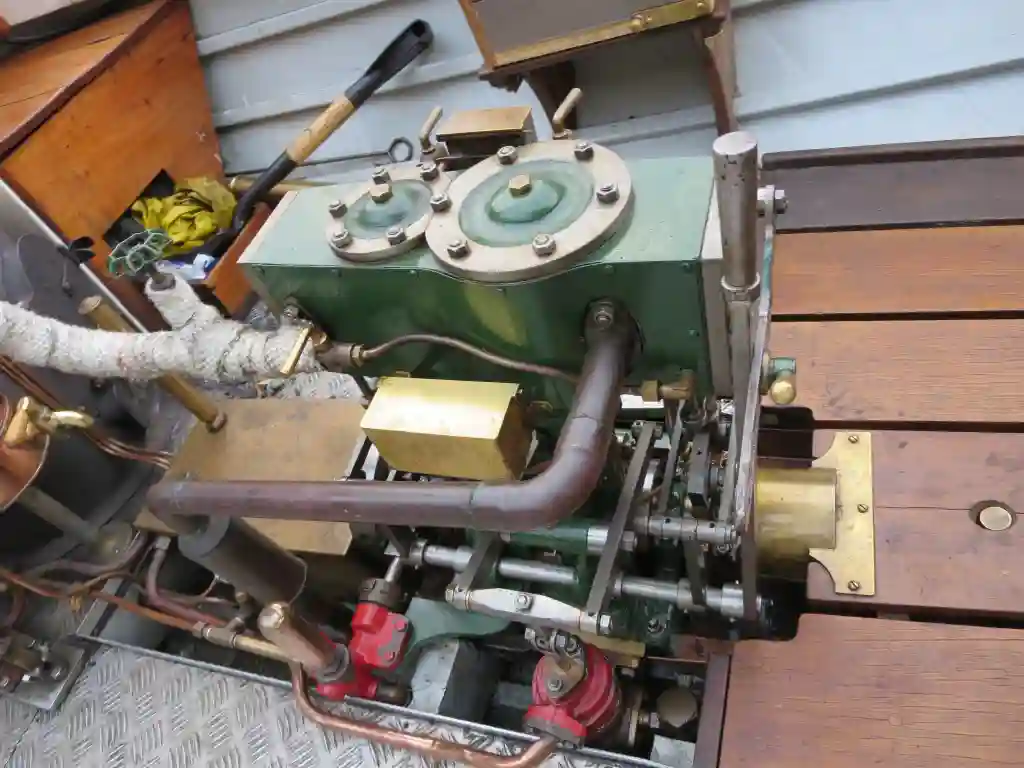
The influence of steam turbine propulsion extends beyond its direct application in ships to its role in advancing broader engineering and industrial practices. The development and refinement of steam turbines for marine use have contributed significantly to the fields of thermodynamics, fluid mechanics, and materials science. These advancements have had a ripple effect, influencing the design and operation of land-based power plants, industrial machinery, and even aerospace technology.
In the maritime sector, the evolution of steam turbine propulsion has been closely tied to the changing demands and priorities of the industry. During the early to mid-20th century, the need for larger, faster, and more powerful ships drove the adoption of steam turbines. The technology was seen as a solution to the limitations of reciprocating engines, which were bulky, complex, and less efficient at converting steam energy into mechanical work. Steam turbines offered a more compact and efficient means of propulsion, enabling the construction of vessels that could carry more cargo or passengers over greater distances at higher speeds.
One of the key milestones in the history of steam turbine propulsion was the launch of the RMS Mauretania in 1906, which, along with her sister ship RMS Lusitania, was among the first major ocean liners to be powered by steam turbines. These ships set new standards for speed and efficiency, capturing the Blue Riband for the fastest transatlantic crossing and demonstrating the potential of steam turbine technology in commercial shipping. The success of these vessels spurred further investment and innovation in steam turbine propulsion, leading to its widespread adoption in both the commercial and military sectors.
As steam turbines became more prevalent, engineers and designers faced the challenge of optimizing their performance and reliability. This led to significant advancements in turbine blade design, thermal insulation, and lubrication systems. The development of multi-stage turbines, where steam is expanded and energy extracted in multiple stages, further improved the efficiency of steam turbine systems. These technological advancements not only enhanced the performance of ships but also reduced operating costs and extended the lifespan of the propulsion systems.
In the naval domain, steam turbines played a crucial role in the modernization of fleets. During World War II, many of the world’s most powerful warships, including aircraft carriers and battleships, were equipped with steam turbine propulsion. The high power output and reliability of steam turbines were critical in enabling these vessels to perform a wide range of combat and support operations. The strategic importance of steam turbine-powered ships underscored the need for continuous improvement in turbine technology, driving research and development in areas such as fuel efficiency, thermal management, and noise reduction.
The post-war period saw a gradual shift in maritime propulsion technology, with diesel engines and gas turbines gaining prominence due to their superior fuel efficiency and lower emissions. However, steam turbines remained in use in specific applications where their unique advantages were still relevant. The advent of nuclear propulsion in the 1950s and 1960s provided a new lease of life for steam turbines, as the heat generated by nuclear reactors could be efficiently converted into steam to drive turbines. This development was particularly significant in the context of submarines and aircraft carriers, where the ability to operate for extended periods without refueling was a major strategic advantage.
In the modern era, the use of steam turbines in marine propulsion is limited but still important in certain niches. For example, some LNG carriers continue to use steam turbines, taking advantage of the availability of boil-off gas as a fuel source. Additionally, the lessons learned from decades of operating and maintaining steam turbine systems have informed the design and development of more advanced propulsion technologies, ensuring that the legacy of steam turbines continues to influence the future of maritime engineering.
Overall, the story of steam turbine propulsion is one of technological evolution, adaptation, and enduring impact. From its heyday in the early 20th century to its specialized applications today, steam turbine technology has left an indelible mark on the maritime industry and beyond. Its contributions to the fields of engineering, transportation, and energy have helped shape the modern world, highlighting the transformative power of human innovation in harnessing the energy of steam to propel ships across the seas.
- Historical Development and Adoption
Steam turbine propulsion emerged in the late 19th and early 20th centuries as a revolutionary technology in maritime engineering. The shift from reciprocating steam engines to steam turbines marked a significant advancement in ship propulsion. Early adopters, such as the RMS Mauretania and RMS Lusitania, demonstrated the advantages of turbines, including higher speeds and greater efficiency, prompting widespread adoption in both commercial and naval vessels. These ships set new benchmarks for performance, influencing the design of future maritime vessels and establishing steam turbines as the preferred propulsion method for large ships. - Engineering Principles and System Components
The core of steam turbine propulsion lies in the conversion of thermal energy from steam into mechanical work. The system comprises a boiler to generate high-pressure steam, turbines with precision-engineered blades to extract energy, and a condenser to recycle water. The turbines operate on thermodynamic cycles, typically the Rankine cycle, and involve multiple stages to maximize energy extraction. Materials science plays a crucial role, with advanced alloys used for turbine blades to withstand high temperatures and pressures. This engineering complexity ensures efficient and reliable propulsion for large ships. - Applications and Advantages in Maritime Operations
Steam turbine propulsion proved invaluable for a range of maritime applications, from luxury liners to military vessels. Its ability to generate substantial power made it ideal for large ships requiring high speeds and extended operational ranges. The smooth and continuous power delivery of turbines also contributed to their preference in naval vessels, where reliability was paramount. In commercial shipping, turbines enabled the transportation of goods and passengers at unprecedented speeds, fostering global trade and connectivity. - Challenges and Evolution
Despite its advantages, steam turbine propulsion faced challenges, including high fuel consumption and significant space requirements. The complexity of the system demanded rigorous maintenance and operational expertise. The mid-20th century saw a shift towards more fuel-efficient propulsion methods, such as diesel engines and gas turbines. However, the advent of nuclear propulsion in naval vessels offered a new application for steam turbines, utilizing reactor-generated heat to produce steam. This development highlighted the adaptability of steam turbines and their continued relevance in specific contexts. - Legacy and Modern Relevance
While steam turbines have largely been replaced by more modern propulsion systems, their legacy endures in maritime history. They represent a critical phase in the evolution of marine engineering, influencing contemporary propulsion technologies. Steam turbines remain in use in specialized applications, such as LNG carriers and nuclear-powered ships, where their unique benefits are still advantageous. The engineering principles and innovations derived from steam turbine development continue to impact modern technologies, underscoring their lasting significance in the field of maritime propulsion.
Historical Development and Adoption
The historical development and adoption of steam turbine propulsion represent a significant chapter in the evolution of maritime engineering. This transition from traditional reciprocating steam engines to the more advanced steam turbines marked a pivotal moment in the history of naval and commercial shipping. The initial foray into steam turbine technology can be traced back to the late 19th century when engineers began to explore ways to improve the efficiency and performance of ship propulsion systems. The limitations of reciprocating steam engines, which were bulky, complex, and less efficient, spurred the search for alternative propulsion methods that could offer higher speeds and greater power.
The breakthrough came with the invention of the steam turbine by Sir Charles Parsons in 1884. Parsons’ design featured a continuous rotary motion that was more efficient than the back-and-forth motion of reciprocating engines. His demonstration of a small steam turbine-powered vessel, Turbinia, at the Royal Navy Fleet Review in 1897, showcased the potential of this new technology. Turbinia achieved unprecedented speeds, far surpassing those of contemporary ships, and this performance caught the attention of the maritime industry.
The success of Turbinia led to the adoption of steam turbines in larger vessels, with the RMS Mauretania and RMS Lusitania being among the first major ocean liners to be equipped with this technology. Launched in the early 20th century, these ships set new records for transatlantic crossings, capturing the Blue Riband and demonstrating the superior performance of steam turbines. Their ability to maintain higher speeds and carry more passengers and cargo than their reciprocating engine-powered counterparts made them icons of a new era in maritime travel.
The adoption of steam turbines was not limited to commercial shipping. Naval fleets quickly recognized the strategic advantages of turbine propulsion, particularly in terms of speed and maneuverability. The Royal Navy and other major naval powers began to incorporate steam turbines into their warships, leading to the development of faster and more powerful vessels. During World War I and World War II, steam turbine-powered ships played crucial roles in naval operations, with aircraft carriers, battleships, and cruisers leveraging the technology for enhanced combat capabilities.
The widespread adoption of steam turbines in both commercial and military vessels was driven by several factors. The higher efficiency and smoother operation of turbines compared to reciprocating engines reduced fuel consumption and maintenance costs, making them economically advantageous. Additionally, the compact design of turbines allowed for more efficient use of space within the ship, providing room for additional cargo or armament.
As the 20th century progressed, the technological advancements in steam turbine design continued to evolve. The development of multi-stage turbines, which allowed for greater energy extraction from steam, further improved their efficiency and performance. These advancements, combined with improvements in boiler technology and materials science, enhanced the reliability and durability of steam turbine systems, solidifying their place in maritime propulsion.
However, the dominance of steam turbines began to wane in the mid-20th century with the rise of diesel engines and gas turbines. These newer propulsion systems offered better fuel efficiency and lower emissions, aligning with the changing priorities of the maritime industry. Despite this shift, steam turbines found a new application in the emerging field of nuclear propulsion. The heat generated by nuclear reactors was effectively used to produce steam, which then powered turbines, making them a critical component of nuclear-powered submarines and aircraft carriers.
In conclusion, the historical development and adoption of steam turbine propulsion marked a transformative period in maritime history. The transition from reciprocating engines to steam turbines enabled the creation of faster, more efficient, and more powerful ships, revolutionizing both commercial and military maritime operations. While newer technologies have since taken precedence, the legacy of steam turbines continues to influence modern engineering and propulsion systems, underscoring their enduring impact on the maritime industry.
The adoption of steam turbine propulsion had a profound impact on shipbuilding and maritime operations, shaping the future of global transportation and naval strategy. The transition to steam turbines brought about significant changes in ship design, as the compact and efficient nature of turbines allowed for more streamlined hulls and improved overall vessel performance. This period saw the emergence of iconic ships that exemplified the advantages of steam turbine propulsion, not only in terms of speed but also in reliability and operational efficiency.
One of the most notable effects of this technological shift was the enhancement of global trade and commerce. Steam turbine-powered vessels could traverse long distances more quickly and with greater reliability than their predecessors, which was particularly advantageous for transoceanic routes. The reduced travel times and increased cargo capacity enabled shipping companies to expand their networks, facilitating the growth of international trade and contributing to the globalization of markets. Passenger liners also benefited from these advancements, offering faster and more comfortable journeys, which helped to popularize transatlantic travel among the general public.
In the naval sphere, the strategic advantages of steam turbine propulsion were quickly realized. The increased speed and maneuverability of turbine-powered warships provided naval forces with a critical edge in both offensive and defensive operations. The ability to outpace enemy ships and reposition rapidly was a game-changer in naval warfare, influencing tactics and fleet compositions. The Royal Navy, for instance, leveraged steam turbine technology to maintain its dominance on the high seas, particularly during the early 20th century when naval power was a cornerstone of global influence.
The introduction of steam turbines also spurred a wave of innovation and competition within the maritime industry. Shipyards and engine manufacturers invested heavily in research and development to optimize turbine designs and improve their efficiency. This period of intense innovation led to numerous advancements, such as better turbine blade aerodynamics, enhanced thermal efficiency, and more robust materials capable of withstanding the harsh marine environment. These improvements not only extended the operational lifespan of turbines but also made them more cost-effective and reliable.
Despite the eventual decline in the use of steam turbines for conventional ships, their legacy persisted through their continued use in specialized applications and the lessons they provided for future technologies. The experience gained in designing and operating steam turbines laid the groundwork for advancements in other areas of engineering, including power generation and aerospace. The principles of thermodynamics and fluid dynamics honed through turbine development have been applied to a wide range of industries, illustrating the far-reaching impact of this maritime innovation.
In the modern era, the influence of steam turbine propulsion can still be seen in the context of nuclear-powered vessels. The synergy between nuclear reactors and steam turbines remains a key aspect of naval engineering, with many of today’s most advanced submarines and aircraft carriers utilizing this combination to achieve unparalleled endurance and operational capability. Furthermore, the expertise in managing high-pressure steam systems and optimizing thermal cycles continues to inform contemporary engineering practices, ensuring that the knowledge gained from the era of steam turbine dominance is not lost.
In retrospect, the historical development and adoption of steam turbine propulsion represent a landmark in maritime history, one that not only transformed the shipping industry but also set the stage for future technological advancements. The legacy of steam turbines is evident in the way they reshaped global trade, military strategy, and engineering practices, leaving an indelible mark on the world and highlighting the enduring significance of this remarkable technology.
Engineering Principles and System Components
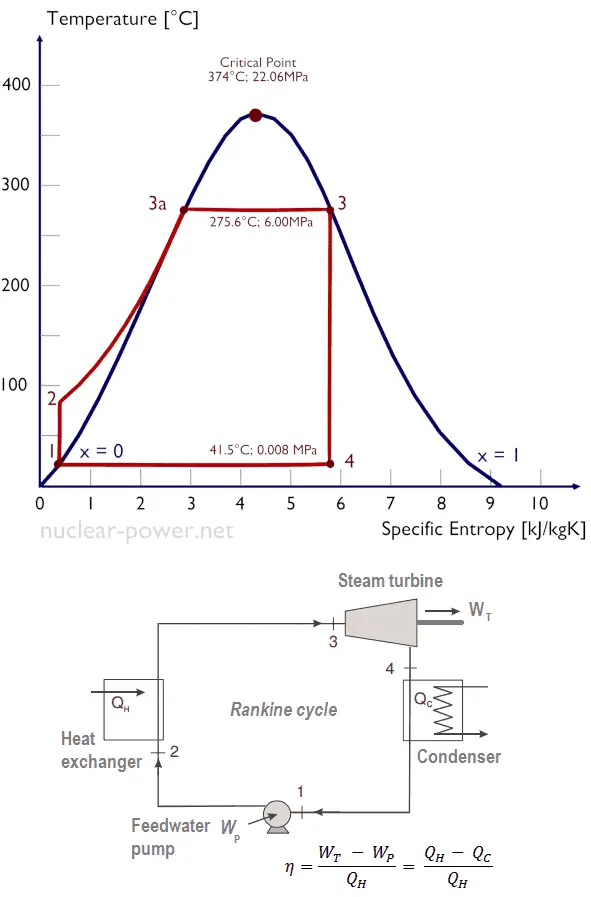
The engineering principles and system components of steam turbine propulsion form a complex yet elegant system designed to efficiently convert thermal energy from steam into mechanical work for propelling ships. Central to this technology is the thermodynamic cycle, typically the Rankine cycle, which describes the processes through which water is converted into steam and then condensed back into water for reuse. This cycle involves several key components: the boiler, the steam turbine, the condenser, and the feedwater pump, each playing a crucial role in the overall operation and efficiency of the propulsion system.
At the heart of the system is the boiler, where water is heated to produce high-pressure steam. The boiler must maintain precise temperature and pressure conditions to ensure the efficient generation of steam. This steam, at a high temperature and pressure, is then directed to the steam turbine. The steam turbine itself is a marvel of engineering, consisting of a series of blades arranged in multiple stages on a rotor. These blades are designed to extract energy from the steam as it expands and cools through the turbine. The arrangement of blades is crucial, with the first set of blades (nozzles) accelerating the steam and directing it onto the rotor blades, where the actual energy transfer occurs. This setup allows for the efficient conversion of the steam’s thermal and kinetic energy into mechanical work.
The turbine operates on the principle of converting the energy of steam into rotational energy. As steam enters the turbine, it strikes the blades at high velocity, causing the rotor to spin. This spinning rotor is connected to the ship’s propeller shaft, and the mechanical energy generated by the turbine is transmitted to the propeller, propelling the ship through the water. The design of the turbine blades is critical; they must be aerodynamically optimized to maximize energy extraction while withstanding the high temperatures and pressures of the steam. Materials used in these blades are typically advanced alloys that offer high strength and resistance to thermal creep and corrosion.
Once the steam has passed through the turbine, it enters the condenser, where it is cooled and converted back into water. The condenser plays a vital role in the system by ensuring that the steam is efficiently condensed into water for reuse in the boiler. This closed-loop system minimizes water loss and enhances the overall efficiency of the propulsion system. The cooling process in the condenser is typically achieved using seawater, which absorbs the heat from the steam. Efficient condensate management is essential to maintain the system’s efficiency and to prevent the buildup of air or non-condensable gases, which can reduce the effectiveness of the condensation process.
After condensation, the water is pumped back into the boiler using feedwater pumps. These pumps must be capable of handling high pressures to ensure the continuous and efficient flow of water back into the boiler. The entire process is carefully monitored and controlled to maintain optimal operating conditions, ensuring the efficient conversion of heat energy into mechanical work.
One of the key challenges in steam turbine propulsion is the management of heat losses and the optimization of the energy conversion process. Engineers have developed various strategies to improve the efficiency of steam turbines, including the use of superheated steam, which increases the temperature of the steam above its saturation point, thereby increasing the efficiency of the energy transfer process. Additionally, the development of multi-stage turbines, where steam is expanded and energy is extracted in multiple stages, has significantly improved the efficiency of steam turbine systems.
Another important aspect of steam turbine propulsion is the lubrication and cooling of the turbine components. The high rotational speeds and temperatures involved require effective lubrication to reduce friction and wear on the turbine’s moving parts. Lubrication systems must be meticulously maintained to ensure the smooth operation of the turbine and to prevent mechanical failures.
Overall, the engineering principles and system components of steam turbine propulsion reflect a sophisticated integration of thermodynamics, fluid dynamics, and materials science. The precise control of steam generation, energy extraction, and heat dissipation is essential for the efficient operation of the propulsion system. The innovations in turbine design and materials have not only enhanced the performance and reliability of steam turbines but also paved the way for advancements in other fields of engineering. This complex interplay of components and principles underscores the ingenuity behind steam turbine propulsion and its lasting impact on maritime engineering.
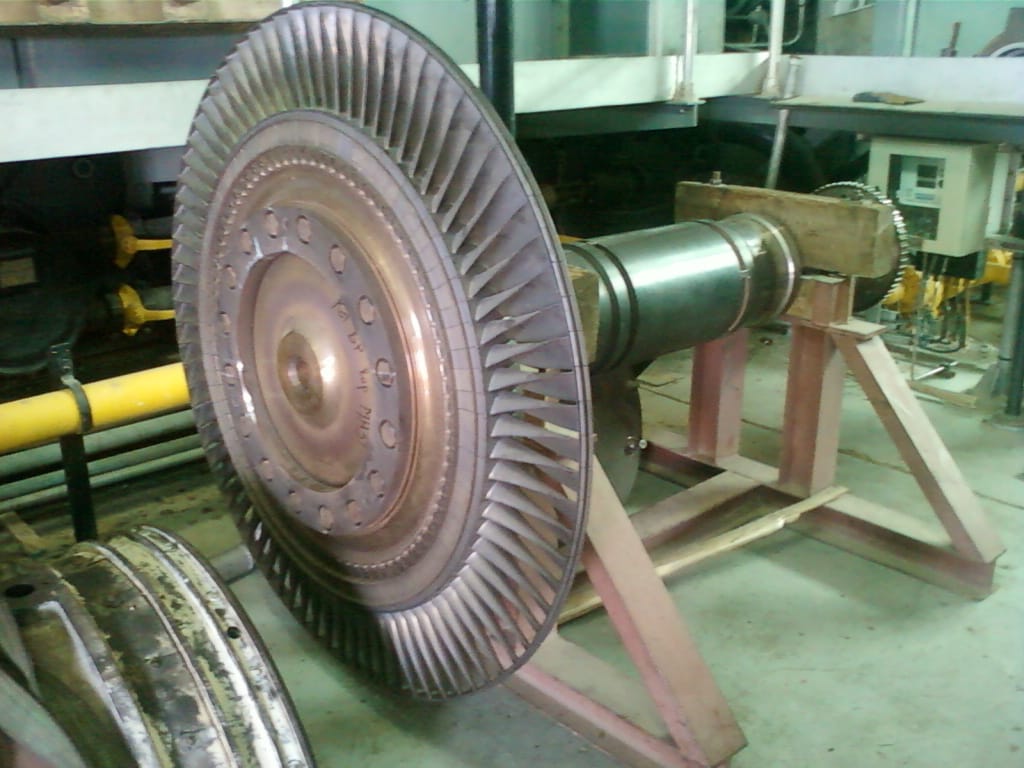
Building on the foundations of steam turbine propulsion, further advancements in engineering principles and system components have continued to shape the efficiency and adaptability of these systems. As steam turbines were implemented in a variety of vessels, engineers worked on refining the technology to optimize power output while minimizing fuel consumption and maintenance needs. One of the most significant advancements in this regard was the development of multi-stage turbines. These turbines utilize multiple stages of blades and nozzles, with steam passing through each stage sequentially. Each stage extracts a portion of the energy from the steam, which is then converted into mechanical energy, allowing for more efficient use of the steam’s potential. This multistage process ensures that the energy is not wasted and that the steam’s pressure and temperature are gradually reduced in a controlled manner, increasing the turbine’s overall efficiency.
A key consideration in steam turbine design is the thermodynamic efficiency of the system. The efficiency of converting heat into mechanical work is directly linked to the temperature difference between the steam and the surroundings. To maximize this efficiency, superheating the steam became a critical innovation. Superheating involves raising the temperature of the steam beyond its saturation point before it enters the turbine. This process prevents the steam from condensing within the turbine, ensuring that all of its thermal energy is available for work. Superheated steam also helps reduce moisture formation in the turbine, which can cause erosion and damage over time. These innovations have led to significantly improved performance, allowing turbines to operate at higher pressures and temperatures without compromising durability.
In conjunction with superheating, reheat systems were introduced to further boost the efficiency of steam turbines. In this setup, steam is passed through the turbine in stages, with the steam being partially expanded and then reheated before entering the next stage. This allows the steam to maintain its energy level and increase the turbine’s efficiency by avoiding significant temperature loss between stages. Reheating has been especially important for large marine vessels, where high efficiency and power output are essential for performance and fuel economy.
Another area of improvement has been the materials used in turbine construction. As turbines operate under extreme conditions of heat and pressure, the materials used to construct the turbine blades, rotors, and other components must be able to withstand significant mechanical stresses. Early turbines used simple metals, but as turbine efficiency increased and operating conditions became more demanding, advanced alloys and composite materials were developed. Nickel-based alloys, for example, have become common in turbine blades because of their ability to resist high temperatures and corrosion. These materials are also designed to maintain their strength and flexibility under the constant rotational forces that turbines generate. The choice of materials not only extends the operational life of the turbine but also helps reduce the frequency of maintenance and downtime.
Lubrication systems have also evolved to ensure the smooth operation of steam turbines, especially given the high rotational speeds involved. Proper lubrication is critical to minimizing friction and wear, which can significantly affect turbine efficiency and reliability. Advanced oils and synthetic lubricants have been developed to cope with the high temperatures and pressures within the turbine. These oils are specifically formulated to prevent oxidation and degradation, ensuring that the turbines remain well-lubricated throughout their lifespan.
One of the most innovative developments in steam turbine technology has been the advent of nuclear-powered steam turbines, which use the heat generated by nuclear reactors to produce steam. This marriage of nuclear energy and steam turbine propulsion has had a transformative effect on naval vessels, particularly submarines and aircraft carriers, where extended operational endurance without the need for refueling is crucial. The nuclear reactor heats the water to produce steam, which then drives the turbine in the same manner as conventional steam systems. This technology allows nuclear-powered vessels to remain at sea for months without needing to return to port for fuel, a game-changer for military applications, especially in strategic defense and long-range missions.
The integration of condensers in the steam cycle has further enhanced the efficiency of steam turbines. By recovering and condensing steam into water in a closed-loop system, condensers help minimize water wastage, reducing the need for large quantities of freshwater. In marine environments, this is particularly valuable as it conserves water resources and improves the overall sustainability of the propulsion system. The condenser works by transferring the heat from the steam to the surrounding environment—often using seawater as a cooling medium. The effectiveness of the condenser directly impacts the overall performance of the turbine, as the ability to rapidly cool and condense the steam allows the system to continuously operate at peak efficiency.
Feedwater heaters have also been introduced into the steam cycle to improve the overall thermal efficiency. These systems preheat the water before it enters the boiler, using waste heat from the exhaust steam. This minimizes the amount of energy required to bring the water to the necessary boiling point, reducing the fuel needed and improving the overall efficiency of the cycle. These components are typically installed as part of an integrated energy recovery system, which also helps to reduce the environmental impact of steam turbine propulsion.
As steam turbine technology advanced, its application spread beyond ships to other areas, such as land-based power generation. The same principles of energy conversion used in marine turbines were adapted to produce electricity in thermal power plants. The massive turbines in power plants often operate on the same basic principles as those used in marine vessels, except on a larger scale. These land-based applications also spurred further innovations in materials, heat transfer technologies, and turbine design, influencing the development of highly efficient power systems.
In summary, the engineering principles and system components of steam turbine propulsion are a study in continuous innovation and adaptation. From the basic components such as the boiler, turbine, condenser, and feedwater system to advancements like multi-stage turbines, superheating, and nuclear integration, the evolution of steam turbine technology has been shaped by a drive to maximize power output, efficiency, and reliability. The integration of advanced materials, lubricants, and energy recovery systems has made steam turbine propulsion a sophisticated and resilient technology, capable of meeting the demands of modern marine vessels and power plants alike. This legacy of innovation continues to influence modern engineering practices, ensuring that the principles of steam turbine propulsion remain a key part of the broader landscape of energy conversion technologies.
Challenges and Evolution
The challenges and evolution of steam turbine propulsion reflect the ongoing quest to improve efficiency, reduce operational costs, and address emerging needs in the maritime and energy sectors. While steam turbines offered significant advantages over reciprocating engines, particularly in terms of speed, reliability, and power output, they also presented a set of technical and operational challenges that engineers had to overcome. As the technology evolved, many of these challenges were addressed through innovations in design, materials, and operating practices. However, other new hurdles emerged, prompting further advancements in turbine technology and shifting the focus of propulsion systems in some areas of maritime and industrial engineering.
One of the primary challenges faced by steam turbine systems in the early stages of their development was the complexity and size of the machinery. Steam turbines required large, intricate components, and the associated systems, such as boilers and condensers, were similarly large and required significant amounts of space within a vessel. This space requirement limited the design flexibility of ships, particularly those with more compact hulls or specific operational needs. Moreover, the high operating pressures and temperatures of steam turbines demanded advanced engineering and materials capable of withstanding these extreme conditions. Early turbine systems were prone to failures caused by material fatigue, corrosion, and thermal stresses, which made maintenance and repairs more frequent and costly.
As a result, steam turbine technology had to undergo significant refinement in terms of both design and materials to ensure greater reliability. The development of high-strength alloys that could withstand higher temperatures and pressures led to more durable turbine blades and rotors. Additionally, the introduction of more advanced sealing systems and lubrication techniques helped reduce the incidence of mechanical failures. The evolution of multi-stage turbines was another response to the challenge of improving efficiency and reducing energy loss. In multi-stage systems, the steam is expanded and utilized in several stages, extracting energy progressively and improving the overall efficiency of the turbine. These improvements made steam turbines more reliable and operationally viable for larger vessels and power plants.
However, the inherent complexity and the need for frequent maintenance and repairs kept steam turbines from being a universally ideal solution. In the mid-20th century, the advent of diesel engines and gas turbines began to challenge the supremacy of steam turbines. Diesel engines, which operate using combustion rather than steam, offered several key advantages: they were more fuel-efficient, simpler to operate, and required less maintenance than steam turbines. Additionally, diesel engines could be more easily adapted to smaller vessels and commercial shipping, where the space and cost constraints of steam turbines were more pronounced. This shift to diesel-powered propulsion systems was further accelerated by advancements in fuel processing, engine design, and the growing need for more environmentally friendly options in terms of emissions and fuel consumption.
The rise of gas turbines, initially developed for military aircraft, also posed a challenge to steam turbines in naval and commercial ships. Gas turbines offered high power-to-weight ratios, quick startup times, and a simpler operational mechanism. Their efficiency at higher speeds and ability to produce thrust in a more compact form made them especially attractive for naval vessels, where maneuverability and speed were critical. Despite these advantages, gas turbines still faced limitations in terms of fuel efficiency and operational costs, which made steam turbines more appealing for certain applications, such as large merchant vessels and power generation plants.
One of the more unique evolutions of steam turbine technology came with the introduction of nuclear-powered ships. The coupling of nuclear reactors with steam turbines allowed for a new class of vessels, including nuclear submarines and aircraft carriers, to operate for extended periods without the need for refueling. Nuclear reactors provided a virtually unlimited source of heat to generate steam, which could then drive turbines, making these ships capable of remaining at sea for months or even years without refueling. The evolution of nuclear-powered steam turbines marked a significant milestone in the development of propulsion systems, as it demonstrated the adaptability of steam turbines to new sources of energy beyond traditional fossil fuels. Despite their complexity and high operational costs, nuclear-powered ships demonstrated the power and endurance that steam turbines could offer in specific military and strategic contexts.
However, as steam turbines were increasingly replaced by more efficient and simpler alternatives in the commercial and naval sectors, they found a new lease on life in power generation. Steam turbines became the backbone of many thermal power plants, where they were used to convert heat from burning fossil fuels or nuclear reactions into electrical power. This marked the beginning of a new chapter in the evolution of steam turbine technology, one that focused on large-scale energy production rather than transportation. The principles and components used in maritime steam turbines were adapted for land-based power stations, and innovations in turbine design, such as reheat cycles, condensate recovery, and feedwater heating, helped further improve the efficiency and environmental impact of steam turbine systems. Over time, these improvements allowed steam turbines to maintain a significant role in the energy sector, despite the growing dominance of other energy production methods like gas and wind power.
Throughout the latter half of the 20th century, steam turbine technology evolved in response to the global push for energy efficiency and environmental sustainability. Efforts were made to reduce the environmental impact of steam turbines, including the adoption of cleaner fuels and more advanced combustion techniques in fossil-fuel-powered turbines. Additionally, the development of combined-cycle systems, where gas turbines are paired with steam turbines to capture and reuse waste heat, significantly improved the overall efficiency of power plants, contributing to a more sustainable energy production process.
Despite facing competition from newer technologies, steam turbines have not disappeared from modern applications. They continue to be used in specialized fields where their benefits—such as high power output and long operational lifespans—are still valued. Their application in nuclear power plants, hydropower plants, and large industrial processes highlights the continued relevance of steam turbines in the global energy landscape. Furthermore, ongoing research into materials, cooling systems, and turbine blade designs continues to improve their performance, keeping steam turbines viable even in the face of alternative technologies.
In conclusion, the challenges and evolution of steam turbine propulsion have been marked by both technological advancements and shifts in operational requirements. Initially hindered by complexity and maintenance needs, steam turbines overcame many obstacles through innovations in materials, design, and efficiency. While they were largely displaced by diesel engines and gas turbines in certain maritime applications, steam turbines adapted to new roles in nuclear-powered ships and power generation. Their enduring legacy in the energy sector underscores their adaptability and efficiency, ensuring that steam turbine technology remains an important part of modern engineering, even as the search for more sustainable and efficient propulsion systems continues.
As the steam turbine continued to evolve, its role in modern engineering remained significant, especially in industries where reliability, large-scale power generation, and long operational cycles were paramount. One of the most notable evolutions in recent years has been the focus on enhancing operational efficiency while reducing the environmental footprint of steam turbines. In the wake of growing concerns about climate change and the demand for cleaner energy sources, many steam turbine systems have been adapted to work in tandem with renewable energy sources, such as biomass and geothermal energy, creating more sustainable power generation systems. These adaptations enable steam turbines to continue contributing to the global energy landscape while meeting the increasing emphasis on sustainability.
The integration of combined heat and power (CHP) systems has become another key development in the evolution of steam turbine technology. CHP systems, also known as cogeneration systems, utilize the waste heat from electricity generation to provide heating, either for industrial processes or residential use. By harnessing this otherwise wasted heat, CHP systems achieve much higher overall efficiency than traditional steam turbine-only power plants, reducing fuel consumption and greenhouse gas emissions. This technology is especially valuable in applications like district heating, where the heat produced can be distributed to large areas, providing both electricity and thermal energy from a single source.
Another emerging trend in the evolution of steam turbine technology is the push for hybrid propulsion systems in ships and industrial applications. These systems combine the benefits of steam turbines with other technologies, such as diesel engines or gas turbines, to optimize performance and fuel consumption under varying operational conditions. In marine vessels, for instance, hybrid systems may allow ships to operate at lower speeds using diesel engines for greater fuel efficiency, while employing steam turbines when higher speeds or greater power are needed. This flexibility can significantly reduce operating costs and emissions, providing a more adaptable solution to meet the diverse energy demands of modern fleets.
The continued development of advanced materials is also contributing to the ongoing evolution of steam turbines. Research into more heat-resistant and corrosion-resistant materials has led to the creation of advanced alloys and ceramic materials that can withstand higher operational temperatures and more aggressive chemical environments. These materials not only enhance the durability and efficiency of steam turbines but also help reduce the frequency and cost of maintenance. In particular, materials that can maintain structural integrity at high temperatures are critical for turbines in nuclear power plants, where consistent and reliable performance is essential. Furthermore, additive manufacturing (3D printing) technologies have begun to play a role in the design and production of steam turbine components, offering new opportunities for precision manufacturing and rapid prototyping.
In addition to materials innovation, digital technologies have increasingly been incorporated into steam turbine systems. Sensors, data analytics, and predictive maintenance tools are now integrated into modern steam turbines, enabling operators to monitor performance in real-time and identify potential issues before they lead to failures. These digital advancements provide significant cost savings by reducing unplanned downtime, extending the life of turbine components, and optimizing the operating conditions of the turbine to maximize efficiency. The trend toward Industry 4.0 and smart manufacturing is likely to further enhance the performance and lifespan of steam turbines, contributing to their continued viability as a key component in power generation and industrial applications.
Despite these advancements, the overall trend in the propulsion and power generation sectors has seen a shift toward alternative energy sources. The push for reducing reliance on fossil fuels has led to the development of renewable energy technologies like wind turbines, solar power, and hydroelectric plants, which, in some cases, offer more sustainable and cost-effective solutions than steam turbines. For instance, offshore wind farms and solar farms are rapidly growing in popularity due to their ability to harness clean energy without the environmental costs associated with fossil fuel combustion. This shift, while impacting the traditional roles of steam turbines, is also driving the evolution of hybrid energy systems where steam turbines may still play a role, but in conjunction with renewable energy sources.
The ongoing research into green hydrogen also presents a potential avenue for steam turbine technology. As a zero-emission fuel source, hydrogen could be used to generate steam without the harmful emissions typically associated with fossil fuel combustion. The development of hydrogen-powered steam turbine systems, coupled with the growing infrastructure for hydrogen production and distribution, could present a new and sustainable direction for steam turbines in the energy landscape. This transition would allow steam turbines to maintain relevance in the global effort to decarbonize energy systems while continuing to leverage their established strengths in large-scale, reliable power generation.
In conclusion, the evolution of steam turbine technology has been a dynamic journey, driven by continuous advancements in engineering, materials science, and energy efficiency. While steam turbines once faced significant challenges in terms of complexity, fuel consumption, and maintenance, their ongoing adaptation to modern energy demands has allowed them to remain a key player in power generation and maritime propulsion. As new technologies emerge, such as hybrid systems, renewable energy integration, and digital innovations, steam turbines continue to evolve, ensuring their role in a more sustainable and technologically advanced future. Even as other technologies vie for dominance, steam turbines’ capacity for large-scale power generation and their continued relevance in specialized applications underscore the enduring significance of this foundational technology in the modern world.
Legacy and Modern Relevance
The legacy and modern relevance of steam turbine technology are deeply intertwined with the evolution of industrial engineering, energy production, and transportation. Steam turbines, which first revolutionized power generation and propulsion in the late 19th and early 20th centuries, continue to influence a wide range of industries, even as newer technologies emerge. Their legacy can be seen in the lasting impact they’ve had on the design of power plants, the development of naval vessels, and the way energy systems are structured today. While they may no longer be the dominant technology in many fields, steam turbines have evolved and adapted to remain a critical part of modern infrastructure, especially in large-scale power generation, nuclear energy, and specialized applications in both maritime and industrial sectors.
At the heart of the steam turbine’s legacy is its profound influence on power generation. As the principal technology for converting thermal energy into mechanical work for much of the 20th century, steam turbines helped pave the way for large-scale electricity generation in power plants. The development of steam turbines allowed for a higher degree of efficiency in converting heat into electricity compared to earlier methods, such as reciprocating engines. This shift enabled power plants to become larger and more reliable, supporting the growth of modern cities and industries, and directly contributing to the rise of mass electrification. Today, steam turbines remain a key component in many of the world’s largest thermal power plants, including those that operate on fossil fuels (coal, natural gas) and nuclear power.
The advent of nuclear-powered steam turbines marked a pivotal moment in both the legacy of the technology and its continued relevance. Nuclear reactors, which generate heat through nuclear fission, pair with steam turbines to produce electricity, just as thermal power plants use fossil fuels. The ability to harness the immense energy of nuclear reactions and convert it into mechanical power for electricity generation represented a major breakthrough. Nuclear-powered steam turbines became the backbone of some of the most powerful and enduring energy systems, providing a low-carbon alternative to traditional fossil fuel-based plants. The role of steam turbines in nuclear power plants not only highlights their adaptability but also underscores their ongoing importance in meeting the world’s energy needs in the face of growing concerns about climate change and the need for cleaner energy sources.
In maritime propulsion, the legacy of steam turbines is just as enduring. The transition from slower, less reliable reciprocating engines to high-speed, efficient steam turbines revolutionized naval warfare and commercial shipping in the early 20th century. Steam turbines powered the great ocean liners, battleships, and aircraft carriers of the 20th century, enabling faster travel and more effective military capabilities. Despite the rise of diesel engines and gas turbines in the second half of the century, steam turbines are still used in specific maritime applications. Nuclear-powered submarines and aircraft carriers, for example, rely on steam turbines driven by nuclear reactors to provide sustained, high-performance propulsion without the need for frequent refueling. Even though other propulsion technologies may offer advantages in certain areas, steam turbines continue to offer unparalleled reliability and efficiency in specialized, long-range naval applications.
Steam turbines have also had a significant impact on the industrial sector, especially in large-scale manufacturing and processing industries. Many industries that rely on thermal energy, such as chemical plants, oil refineries, and paper mills, use steam turbines to generate the power needed to run various processes. In these industries, steam turbines often work in tandem with other systems to optimize energy efficiency, improve reliability, and reduce operational costs. The integration of steam turbines into these industries helped set the stage for the modern energy management practices we see today, where maximizing efficiency and reducing waste are primary considerations.
Despite their continuing importance, the legacy of steam turbines has not been without challenges. As diesel engines and gas turbines gained popularity due to their better fuel efficiency and flexibility, the role of steam turbines in commercial maritime propulsion decreased. Diesel engines, with their compact size, lower operational costs, and fuel flexibility, became the go-to propulsion system for most commercial shipping. Gas turbines, with their rapid acceleration capabilities and high power-to-weight ratios, found favor in military applications. However, steam turbines have not disappeared entirely. They remain in use where large, continuous power output is needed or where specific energy requirements exist, such as in power plants or certain naval vessels.
In modern applications, combined-cycle systems, which pair gas turbines with steam turbines, have enhanced the efficiency of power plants by allowing the waste heat from the gas turbine to be used to generate steam for the steam turbine. This combined approach significantly boosts the overall efficiency of power generation systems, and steam turbines continue to play a vital role in this process. The trend towards hybrid energy systems also keeps steam turbines relevant in modern times. For example, hybrid propulsion systems that combine steam turbines with other technologies—such as diesel engines or batteries—are increasingly used in maritime vessels and large industrial applications. These systems offer the best of both worlds by optimizing fuel consumption and reducing emissions, ensuring that steam turbines can still contribute to meeting modern energy demands.
Looking to the future, the renewable energy landscape presents both challenges and opportunities for steam turbine technology. As the world shifts away from fossil fuels and toward cleaner energy sources, there is a growing interest in integrating steam turbines with biomass and geothermal energy, which generate heat that can be used to produce steam. This shift allows steam turbines to continue to play a role in renewable energy systems, where they can help maximize energy efficiency and reduce waste. Additionally, green hydrogen—produced using renewable energy sources to split water into hydrogen and oxygen—has the potential to provide a clean fuel for generating steam. The combination of green hydrogen and steam turbines could offer a carbon-neutral solution for power generation, ensuring that steam turbines remain relevant as the world moves toward a more sustainable future.
Digital technologies are also helping to extend the life and improve the performance of steam turbines. The integration of sensors, smart monitoring systems, and predictive maintenance tools allows operators to optimize turbine performance and detect potential issues before they lead to significant failures. These advancements in data analytics and artificial intelligence can improve the efficiency of steam turbines by ensuring that they operate at optimal conditions and by minimizing unplanned downtime. In industries like power generation and naval propulsion, these technological enhancements are helping steam turbines remain a viable option in increasingly demanding operational environments.
In conclusion, the legacy of steam turbine technology is marked by its pivotal role in the development of modern power generation, naval propulsion, and industrial manufacturing. Though other technologies have emerged to meet the growing demands for energy efficiency, speed, and flexibility, steam turbines continue to evolve and maintain their relevance in specialized sectors. Their capacity to adapt to new energy sources, integrate with hybrid systems, and benefit from digital innovations ensures that steam turbines will remain a key component of the global energy and transportation infrastructure for years to come. As we move toward a future that emphasizes sustainability, reliability, and efficiency, the legacy of steam turbines and their continued evolution will remain integral to shaping the world’s energy landscape.
As we continue to explore the future relevance of steam turbines, it’s clear that their adaptability will play a crucial role in modern energy systems. Although newer technologies such as solar, wind, and hydrogen energy are gaining traction, steam turbines are poised to continue contributing significantly to both the energy and industrial sectors. As nations and industries strive to meet more stringent environmental targets, steam turbines remain a vital technology due to their proven ability to handle large-scale energy production efficiently.
One of the major factors driving the continued relevance of steam turbines is their scalability. Steam turbines are capable of handling massive power generation needs, which makes them ideal for utility-scale power plants. While renewable sources like wind and solar are growing rapidly, they have limitations in terms of constant power generation, especially in regions where weather patterns are inconsistent. Steam turbines, particularly in combined-cycle plants, complement these renewable sources by providing consistent and stable baseload power. This integration ensures that power generation can remain reliable, even when renewable resources are not available, helping to balance the grid and ensure energy security.
Moreover, as the global demand for electrification and electrical storage solutions grows, steam turbines are finding renewed roles in thermal storage systems. In such systems, excess energy from renewable sources is used to generate heat, which is stored for later use. The stored heat is then used to generate steam, which drives the turbine to produce electricity when demand peaks. This integration of steam turbines with thermal storage technologies is helping to enhance the viability of renewable energy systems by addressing the challenges of intermittency and grid stability.
In industrial processes, steam turbines continue to play an essential role in cogeneration systems, where they provide both electricity and useful thermal energy. Industrial processes in sectors such as chemical manufacturing, oil refining, and pulp and paper production require substantial amounts of both electricity and steam. By using steam turbines in cogeneration, these industries can optimize their energy use, reduce overall fuel consumption, and lower emissions. These systems are particularly advantageous in industries that operate continuously and need reliable, 24/7 power, making steam turbines an invaluable component in industrial energy solutions.
Nuclear energy also continues to be a significant application for steam turbines, especially in nuclear power plants. As many countries transition toward low-carbon energy sources, nuclear power is being seen as a cleaner alternative to fossil fuels. Steam turbines, powered by nuclear reactors, remain central to electricity generation in nuclear plants due to their ability to handle high thermal energy efficiently. While the nuclear industry has faced challenges related to safety concerns and waste disposal, advances in reactor design, such as small modular reactors (SMRs), are reigniting interest in nuclear power. These smaller, more flexible reactors are expected to work in tandem with steam turbines, further ensuring the relevance of steam turbine technology in future energy systems.
The global energy transition is placing a stronger emphasis on decarbonization, and steam turbines are poised to adapt to these goals. As the push for renewable energy sources intensifies, technologies like green hydrogen and biomass offer new pathways to generate steam without the environmental impacts of fossil fuels. Steam turbines are being integrated into systems that produce energy from renewable sources, allowing them to maintain their role in power generation while helping to reduce the carbon footprint of industrial activities. The combination of clean fuels like hydrogen and advanced turbine technologies could play a major role in decarbonizing not only power plants but also hard-to-abate sectors such as heavy industry and shipping.
As the global shipping industry seeks to reduce its carbon emissions, there is also potential for steam turbines to play a part in the evolution of sustainable maritime propulsion. With international shipping being a significant contributor to global greenhouse gas emissions, the search for alternative propulsion technologies is critical. In the realm of hybrid propulsion systems for large ships, steam turbines could work alongside other green technologies, such as batteries and wind propulsion, to reduce reliance on traditional marine fuels. Moreover, nuclear-powered ships—such as aircraft carriers and submarines—will continue to rely on steam turbines for propulsion, as they provide a reliable and efficient solution for long-duration voyages without the need for frequent refueling.
The advancements in materials science are also contributing to the modernization of steam turbine technology. The development of superalloys and ceramic materials has allowed for higher operational temperatures and pressures, enhancing the efficiency and lifespan of turbines. Furthermore, additive manufacturing (3D printing) is playing an increasing role in the production of turbine components. This technology allows for the creation of more complex and lightweight turbine parts that would be difficult or impossible to manufacture using traditional methods. As these innovations continue to improve the performance and durability of steam turbines, they will remain a competitive technology in both energy generation and industrial applications.
In addition to these technical advancements, digital technologies are revolutionizing the way steam turbines are monitored and maintained. The incorporation of advanced sensors, Internet of Things (IoT) technology, and machine learning algorithms into steam turbine systems allows operators to monitor turbine performance in real-time. This enables predictive maintenance, where potential issues are identified before they lead to catastrophic failures, reducing downtime and maintenance costs. The ability to optimize turbine performance based on real-time data ensures that turbines can operate at peak efficiency, extending their operational lifespan and minimizing environmental impacts.
Despite the challenges posed by newer technologies and the transition to renewable energy sources, the legacy and modern relevance of steam turbines are undeniable. Their ability to generate reliable, large-scale power remains essential in many sectors, including power generation, industrial manufacturing, and maritime propulsion. Innovations in turbine design, materials, hybrid systems, and integration with renewable energy sources will ensure that steam turbines continue to evolve and remain a critical part of the global energy landscape. As the world seeks to meet its energy demands while addressing climate change, the steam turbine will continue to play a vital role in shaping the future of energy and industrial systems.
Turbo Generator on Ship
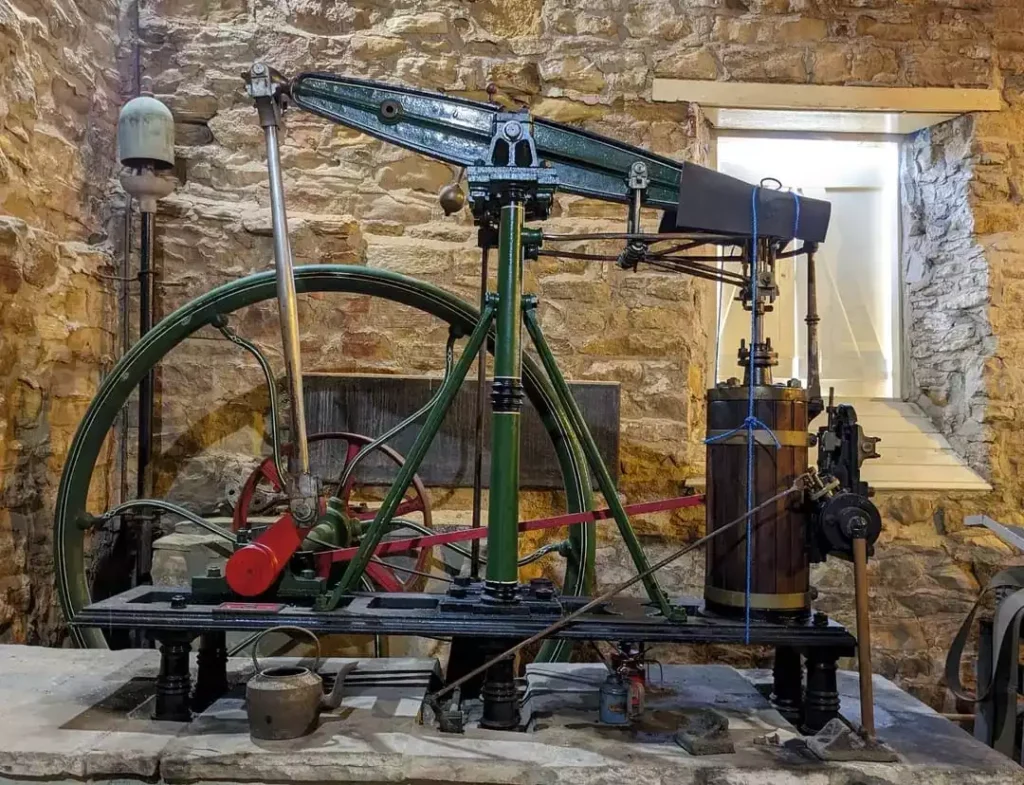
A turbo generator on a ship is an essential component of the vessel’s power generation system, playing a crucial role in ensuring the availability of electrical power for various onboard systems and operations. This complex piece of machinery combines a turbine with an electrical generator to convert mechanical energy into electrical energy, which is then distributed to power everything from navigation systems and communication equipment to lighting, HVAC systems, and propulsion in some cases.
At the core of a ship’s turbo generator is the steam turbine, which harnesses the energy of high-pressure steam generated by the ship’s boilers. This steam is directed through the turbine’s blades, causing them to spin and transfer mechanical energy to the generator. The steam turbine is designed to operate at high efficiency, converting as much thermal energy as possible into mechanical work. The output from the turbine is then used to drive the electrical generator, which converts the mechanical energy into electricity through electromagnetic induction. This electricity is distributed throughout the ship via an electrical distribution network, supplying power to various systems and equipment.
The use of turbo generators on ships, especially in larger vessels such as naval ships, cruise liners, and tankers, is primarily due to their ability to provide a reliable and steady supply of electrical power. Unlike smaller generators that might be used for auxiliary purposes, turbo generators are capable of producing large amounts of power to meet the demands of modern ships, which often require substantial electrical loads. The combination of steam turbines with electrical generators allows for efficient energy conversion and utilization, making turbo generators a preferred choice for ships that operate over long distances and require continuous power.
One of the key advantages of turbo generators is their efficiency in energy conversion. By using steam turbines, which operate at high temperatures and pressures, turbo generators can achieve a higher thermal efficiency compared to other types of generators. This is particularly important on ships, where fuel efficiency is a critical concern due to the high costs associated with marine fuel. Efficient turbo generators reduce fuel consumption, which not only lowers operating costs but also minimizes the environmental impact of the ship by reducing greenhouse gas emissions.
The integration of turbo generators into a ship’s power system also allows for flexibility in power management. On ships with combined propulsion systems, turbo generators can supplement or replace traditional propulsion methods, such as diesel engines, under certain conditions. For example, during cruising at steady speeds, the ship might rely on the turbo generator to provide the necessary electrical power for propulsion, thereby optimizing fuel usage and reducing engine wear. This hybrid approach can extend the life of the main engines and provide redundancy in case of engine failure, enhancing the overall reliability of the ship.
Turbo generators are also crucial in nuclear-powered ships, such as nuclear submarines and aircraft carriers, where the heat generated by the nuclear reactor is used to produce steam that drives the turbine. In these vessels, the turbo generator is part of a sophisticated power generation system that must operate under extreme conditions, including high pressures and temperatures. The reliability of the turbo generator in these settings is paramount, as it not only powers the ship’s systems but also ensures the safe operation of the nuclear reactor by supporting essential cooling and safety systems.
In modern shipbuilding, there is a growing emphasis on incorporating digital monitoring and control systems into turbo generators. These systems enhance the operational efficiency and maintenance of the turbo generator by providing real-time data on performance metrics such as temperature, pressure, vibration, and electrical output. With this data, operators can make informed decisions about load management, preventive maintenance, and troubleshooting. Advanced control systems can also optimize the operation of the turbo generator, adjusting parameters to ensure the most efficient use of steam and fuel, thereby further improving the overall efficiency of the ship’s power system.
The design and maintenance of turbo generators on ships require specialized knowledge and expertise. The engineering of the turbine blades, the generator’s electromagnetic components, and the control systems must all be carefully calibrated to ensure optimal performance. Regular maintenance is essential to prevent wear and tear, especially in the harsh marine environment where saltwater, humidity, and constant motion can accelerate the degradation of mechanical components. Maintenance routines typically include inspections, cleaning, lubrication, and the replacement of worn parts to ensure the turbo generator continues to operate reliably and efficiently.
Despite their advantages, turbo generators are not without challenges. The initial cost of installation can be high, and the complexity of the system requires skilled personnel for operation and maintenance. Additionally, the need for a continuous supply of high-quality steam places demands on the ship’s boiler system, which must be capable of producing the necessary steam at the required pressure and temperature. Any issues with the boiler can directly impact the performance of the turbo generator, making the maintenance of both systems critical to the ship’s overall operational readiness.
As the maritime industry continues to evolve, there is a growing focus on reducing emissions and improving energy efficiency, which has led to increased interest in alternative energy sources and hybrid power systems. While turbo generators are well-suited to integrate with these new technologies, such as LNG (liquefied natural gas) systems or battery storage, their fundamental role in providing reliable electrical power remains unchanged. The ability to integrate with these systems allows turbo generators to remain relevant in an industry that is increasingly looking towards sustainable solutions for power generation.
In conclusion, turbo generators are a vital component of a ship’s power generation system, providing reliable and efficient electrical power for a wide range of applications. Their ability to convert steam energy into electrical energy with high efficiency makes them a preferred choice for large vessels and those with specific power requirements, such as nuclear-powered ships. As the industry moves towards more sustainable practices, the flexibility and efficiency of turbo generators will continue to play a crucial role in meeting the evolving power needs of modern ships.
As the maritime industry advances, turbo generators are evolving to meet the increasing demands for efficiency, reliability, and environmental sustainability. The integration of turbo generators with renewable energy sources and energy storage solutions is becoming more prevalent, helping ships reduce their dependence on traditional fossil fuels and minimize their environmental impact. These advancements position turbo generators as a critical component in the transition towards greener shipping technologies.
One area of significant development is the use of waste heat recovery systems on ships. In such systems, waste heat from the ship’s engines or exhaust gases is captured and used to produce steam, which then drives the turbo generator. This process enhances the overall energy efficiency of the ship by utilizing energy that would otherwise be lost. By recovering and converting waste heat into useful electrical power, ships can reduce fuel consumption and emissions, contributing to both cost savings and environmental benefits.
Additionally, the integration of hybrid power systems that combine turbo generators with other forms of energy production, such as battery storage, is gaining traction. These hybrid systems allow for more flexible and efficient energy management. For example, during periods of low energy demand, the turbo generator can charge onboard batteries, which can then be used to power the ship during peak demand periods or when the generator is offline. This not only reduces fuel consumption but also enhances the redundancy and reliability of the ship’s power system.
The development of smart grid technologies on ships is another advancement that is enhancing the efficiency and management of turbo generators. Smart grids enable better distribution and use of electrical power generated by the turbo generator, optimizing energy flow based on real-time demand. This intelligent energy management system can automatically adjust the operation of the turbo generator to ensure that energy production matches consumption, reducing waste and improving overall efficiency.
In the context of future fuels, turbo generators are well-suited to work with alternative fuel sources such as hydrogen, ammonia, and biofuels. These fuels can be used to produce steam in the ship’s boilers, which then drives the turbo generator. The adaptability of turbo generators to different fuel types makes them a flexible solution for ships looking to transition to cleaner energy sources. As the maritime industry explores these alternative fuels to meet stringent emissions regulations, turbo generators will likely play a crucial role in enabling this transition.
Autonomous and remotely operated vessels are also expected to benefit from the robust and reliable nature of turbo generators. In such vessels, the need for dependable power systems that can operate with minimal human intervention is paramount. Turbo generators, with their established track record of reliability and efficiency, are an ideal choice for these advanced vessels. Furthermore, the integration of remote monitoring and diagnostics allows for continuous assessment of the turbo generator’s performance, enabling timely maintenance and ensuring optimal operation even in remote locations.
In summary, the role of turbo generators on ships is expanding and adapting to meet the challenges of modern maritime operations. With ongoing advancements in energy recovery, hybrid systems, smart grid technology, and alternative fuels, turbo generators are poised to remain a cornerstone of shipboard power generation. Their ability to provide reliable, efficient, and adaptable power solutions makes them indispensable as the maritime industry navigates towards a more sustainable and technologically advanced future.
The future trajectory of turbo generators on ships is marked by their capacity to adapt to rapidly changing technological and environmental demands. As shipping moves toward greater sustainability, turbo generators are likely to be central to various innovative propulsion and power generation strategies.
One of the promising developments is the adoption of supercritical and ultra-supercritical steam conditions in turbo generators. By operating at much higher pressures and temperatures than traditional systems, these advanced steam cycles significantly increase the thermal efficiency of power generation. This means more electricity can be produced from the same amount of fuel, thereby reducing fuel consumption and emissions. Ships equipped with these cutting-edge turbo generators can operate more efficiently, which is critical as environmental regulations tighten globally.
In conjunction with advanced steam cycles, carbon capture and storage (CCS) technologies are being explored to reduce the carbon footprint of ships using turbo generators. By capturing the CO2 emissions from the ship’s exhaust and storing or repurposing them, the overall environmental impact can be reduced, allowing ships to comply with stricter emission standards. The integration of CCS with turbo generator systems represents a forward-looking approach to balancing energy needs with environmental stewardship.
Another area of development is the use of digital twin technology for turbo generators. A digital twin is a virtual replica of the turbo generator that allows operators to simulate and analyze its performance under various conditions without affecting the physical equipment. This technology enables predictive maintenance, optimization of operations, and testing of new configurations or upgrades before they are implemented in the real world. By leveraging digital twins, ship operators can enhance the efficiency and reliability of turbo generators, reduce downtime, and extend the lifespan of the equipment.
The trend toward modular and scalable designs in turbo generators is also gaining momentum. Modular designs allow for easier installation, maintenance, and upgrading of turbo generator systems. This approach is particularly beneficial for retrofitting older ships with new technology, enabling them to meet modern efficiency and emission standards without the need for a complete overhaul. Scalable systems also offer flexibility in power generation, allowing ship operators to adjust the capacity of the turbo generator to match the specific needs of different voyages or operational scenarios.
In terms of operational efficiency, artificial intelligence (AI) and machine learning (ML) are becoming integral to the management of turbo generators on ships. These technologies can analyze vast amounts of data collected from the generator and other ship systems to optimize performance, predict failures, and suggest operational adjustments in real time. AI and ML can help in fine-tuning the operation of the turbo generator to achieve the best balance between fuel efficiency, power output, and emissions, making them invaluable tools for modern ship operations.
Energy diversification is another critical factor shaping the future of turbo generators. As ships increasingly adopt multi-fuel systems capable of using a variety of energy sources, turbo generators will need to be adaptable to these changes. Whether powered by conventional fuels, alternative fuels, or renewable energy sources, turbo generators must maintain high efficiency and reliability. This adaptability will ensure their continued relevance as part of the maritime energy ecosystem.
Finally, the focus on energy resilience and redundancy in ship design is driving the development of more robust and fail-safe turbo generator systems. With the increasing reliance on complex electrical systems aboard modern vessels, the importance of a reliable power supply cannot be overstated. Turbo generators that incorporate multiple redundancies, such as backup systems and fail-safe mechanisms, are essential to ensuring continuous power supply, even in the event of a failure in one part of the system. This resilience is critical for maintaining operational continuity and safety at sea.
In conclusion, turbo generators are set to remain a pivotal component of shipboard power systems well into the future. Their ability to evolve with advancements in technology, adapt to new fuels and energy systems, and integrate with digital and AI-driven management tools ensures that they will continue to play a key role in the maritime industry’s pursuit of efficiency, sustainability, and innovation. As ships become more advanced and environmentally conscious, turbo generators will be at the heart of these transformative changes, providing the power and flexibility needed to navigate the challenges of the modern maritime landscape.
1. Introduction to Turbo Generators on Ships
Turbo generators are a critical part of the power generation systems on ships, combining steam turbines with electrical generators to produce the electricity needed for various onboard systems. These systems are essential for powering navigation, communication, lighting, HVAC, and sometimes even propulsion. The integration of mechanical and electrical components allows turbo generators to efficiently convert thermal energy into electrical energy, making them indispensable on large vessels such as naval ships, cruise liners, and tankers.
2. Technical Operation and Components
At the core of a turbo generator is the steam turbine, which utilizes high-pressure steam to drive its blades, converting thermal energy into mechanical work. This mechanical energy is then transferred to the generator, which produces electricity through electromagnetic induction. Key components include the boiler (for steam generation), the turbine, the generator, and the control systems. Advanced materials and design innovations ensure the efficient and reliable operation of these components under the demanding conditions of marine environments.
3. Applications and Advantages
Turbo generators are widely used in ships for their ability to produce large amounts of power efficiently. They are particularly valuable in ships with high electrical demands, such as those with extensive onboard systems or hybrid propulsion. Their advantages include high thermal efficiency, fuel economy, and the ability to support large-scale power needs. In nuclear-powered ships, turbo generators are essential for converting the heat generated by nuclear reactors into electricity, ensuring the continuous operation of critical systems.
4. Challenges and Innovations
While turbo generators offer numerous benefits, they also face challenges such as high initial costs, complexity, and the need for skilled maintenance. Innovations in waste heat recovery, hybrid power systems, smart grid integration, and the use of alternative fuels like hydrogen and biofuels are addressing these challenges. Digital twin technology, AI, and machine learning are also being used to optimize performance, predict maintenance needs, and improve the overall efficiency and reliability of turbo generators on ships.
5. Future Prospects and Sustainability
The future of turbo generators in the maritime industry is promising, with ongoing advancements in supercritical steam technology, carbon capture, modular designs, and energy resilience. As the industry moves toward greater sustainability, turbo generators are expected to play a central role in reducing emissions and improving energy efficiency. Their ability to integrate with renewable energy sources and adapt to evolving energy systems ensures their continued relevance in the pursuit of greener, more efficient maritime operations.
Introduction to Turbo Generators on Ships
Turbo generators on ships are a fundamental component of the vessel’s power generation system, serving as a critical source of electrical energy for a wide array of onboard functions. These sophisticated machines blend the mechanical prowess of steam turbines with the electrical generation capabilities of alternators to convert thermal energy from steam into electrical power. This electricity is then distributed to various systems within the ship, including navigation, communication, lighting, and auxiliary equipment, ensuring the smooth and efficient operation of the vessel.
The use of turbo generators is particularly prevalent in larger ships, such as cruise liners, cargo ships, naval vessels, and specialized industrial ships. These ships require substantial amounts of electrical power, not just for basic operational needs but also for extensive auxiliary systems that enhance the functionality and comfort aboard the vessel. The robust design of turbo generators makes them well-suited for these high-demand environments, where reliability and efficiency are paramount.
At the heart of a turbo generator system is the steam turbine. Steam turbines harness the energy of high-pressure steam produced by the ship’s boilers. As the steam flows over the blades of the turbine, it causes the rotor to spin, converting thermal energy into mechanical energy. This mechanical energy is then used to drive an electrical generator, which produces electricity through the process of electromagnetic induction. The generated electricity is then routed through an onboard electrical distribution system, supplying power to the ship’s various systems.
One of the primary reasons for the widespread adoption of turbo generators on ships is their high thermal efficiency. The process of generating steam in boilers and using it to drive turbines is highly efficient, especially when compared to other methods of power generation. This efficiency is crucial for ships, as it directly impacts fuel consumption and operational costs. By maximizing the energy extracted from the fuel used to generate steam, turbo generators help reduce overall fuel consumption, leading to significant cost savings and a reduction in greenhouse gas emissions.
Another significant advantage of turbo generators is their ability to provide a steady and reliable source of power. Unlike internal combustion engines, which may experience fluctuations in power output, turbo generators offer consistent performance, which is essential for the stable operation of critical shipboard systems. This reliability is particularly important in situations where power loss could compromise the safety of the vessel, such as in naval operations or when navigating treacherous waters.
The integration of turbo generators into a ship’s power system also allows for greater flexibility in energy management. For instance, in some modern vessels, turbo generators are used in conjunction with diesel engines or other propulsion systems to create a hybrid power setup. This setup can optimize fuel use by allowing the ship to switch between different power sources depending on operational needs, further enhancing fuel efficiency and reducing wear on the primary engines.
In recent years, advancements in turbo generator technology have further improved their performance and efficiency. Innovations such as the use of advanced materials for turbine blades, improved aerodynamic designs, and the incorporation of sophisticated control systems have enhanced the capability of turbo generators to operate at higher temperatures and pressures, thus increasing their overall efficiency. Additionally, the development of compact and lightweight designs has made it easier to integrate turbo generators into a wider range of ship types, expanding their applicability in the maritime industry.
As the maritime industry continues to evolve, the role of turbo generators is becoming increasingly significant in the context of environmental sustainability. With stricter regulations on emissions and a growing emphasis on reducing the carbon footprint of shipping, turbo generators are seen as a key component in achieving greener shipping practices. Their ability to operate on a variety of fuels, including cleaner alternatives such as natural gas and biofuels, positions them as a versatile solution for reducing the environmental impact of marine operations.
In summary, turbo generators are a vital technology in the maritime sector, providing efficient and reliable electrical power for a wide range of shipboard applications. Their integration into ship power systems enhances operational efficiency, reduces fuel consumption, and supports the safe and effective operation of modern vessels. As technology continues to advance and the industry shifts towards more sustainable practices, the importance of turbo generators is likely to grow, cementing their role as a cornerstone of shipboard power generation.
As the maritime industry embraces technological advancements and sustainability goals, the evolution of turbo generators continues to be a focal point. One of the emerging trends is the integration of smart systems that enhance the monitoring and control of turbo generators. These systems leverage real-time data analytics, remote diagnostics, and predictive maintenance to optimize performance, reduce downtime, and extend the operational lifespan of the equipment. By utilizing sensors and advanced software, operators can continuously monitor key performance indicators such as temperature, pressure, and vibration, enabling them to detect anomalies early and take corrective action before a failure occurs.
Another significant area of development is the incorporation of hybrid energy systems on ships, where turbo generators are combined with renewable energy sources such as solar panels or wind turbines. This hybrid approach allows ships to harness renewable energy when conditions are favorable, reducing reliance on fossil fuels and lowering emissions. Turbo generators play a crucial role in these systems by providing a reliable backup power source, ensuring that the ship’s energy needs are met even when renewable sources are insufficient. This integration not only enhances energy efficiency but also aligns with global efforts to reduce the carbon footprint of the shipping industry.
In addition to hybrid systems, the use of alternative fuels is becoming increasingly important for turbo generators. As the industry shifts towards low-sulfur fuels, LNG (liquefied natural gas), and emerging alternatives like hydrogen and ammonia, turbo generators must be adapted to handle these new fuel types. This transition involves modifications to the fuel handling systems, combustion processes, and emissions controls to ensure that the turbo generators can operate efficiently and safely with these fuels. The ability to use a variety of fuels enhances the versatility of turbo generators and supports the industry’s move towards cleaner, more sustainable energy sources.
The focus on modular and scalable designs is also reshaping the landscape of turbo generators on ships. Modular designs allow for easier installation, maintenance, and upgrades, providing ship operators with the flexibility to adapt their power systems to changing needs. This approach is particularly beneficial for retrofitting existing ships with modern, more efficient turbo generators, helping older vessels meet current efficiency and emissions standards without the need for a complete overhaul. Scalable systems enable operators to adjust the capacity of the turbo generator to match the specific power requirements of different operational scenarios, optimizing energy use and minimizing waste.
Training and skill development are essential to fully leverage the capabilities of modern turbo generators. As these systems become more sophisticated, the need for skilled personnel who can operate and maintain them is growing. Training programs that focus on the latest technologies, maintenance practices, and operational strategies are crucial to ensure that the crew can effectively manage these complex systems. Investing in the education and training of marine engineers and technicians is key to maximizing the benefits of turbo generators and ensuring their reliable operation.
Looking ahead, the continued advancement of digital twin technology and artificial intelligence in the maritime sector promises to further enhance the functionality and efficiency of turbo generators. Digital twins provide a virtual replica of the turbo generator, allowing operators to simulate different operating conditions, test new configurations, and predict the impact of potential changes without affecting the actual equipment. AI-driven analytics can offer insights into optimal operating parameters, predictive maintenance schedules, and energy-saving opportunities, helping to improve overall system performance and reduce operational costs.
In conclusion, turbo generators are an indispensable part of modern shipboard power systems, offering a combination of efficiency, reliability, and adaptability that meets the evolving demands of the maritime industry. As the sector continues to innovate and push towards more sustainable practices, turbo generators will remain at the forefront of these advancements, supporting the industry’s goals of reducing emissions, enhancing energy efficiency, and ensuring the safe and effective operation of ships. Their ongoing evolution reflects the dynamic nature of maritime technology and underscores their critical role in the future of shipping.
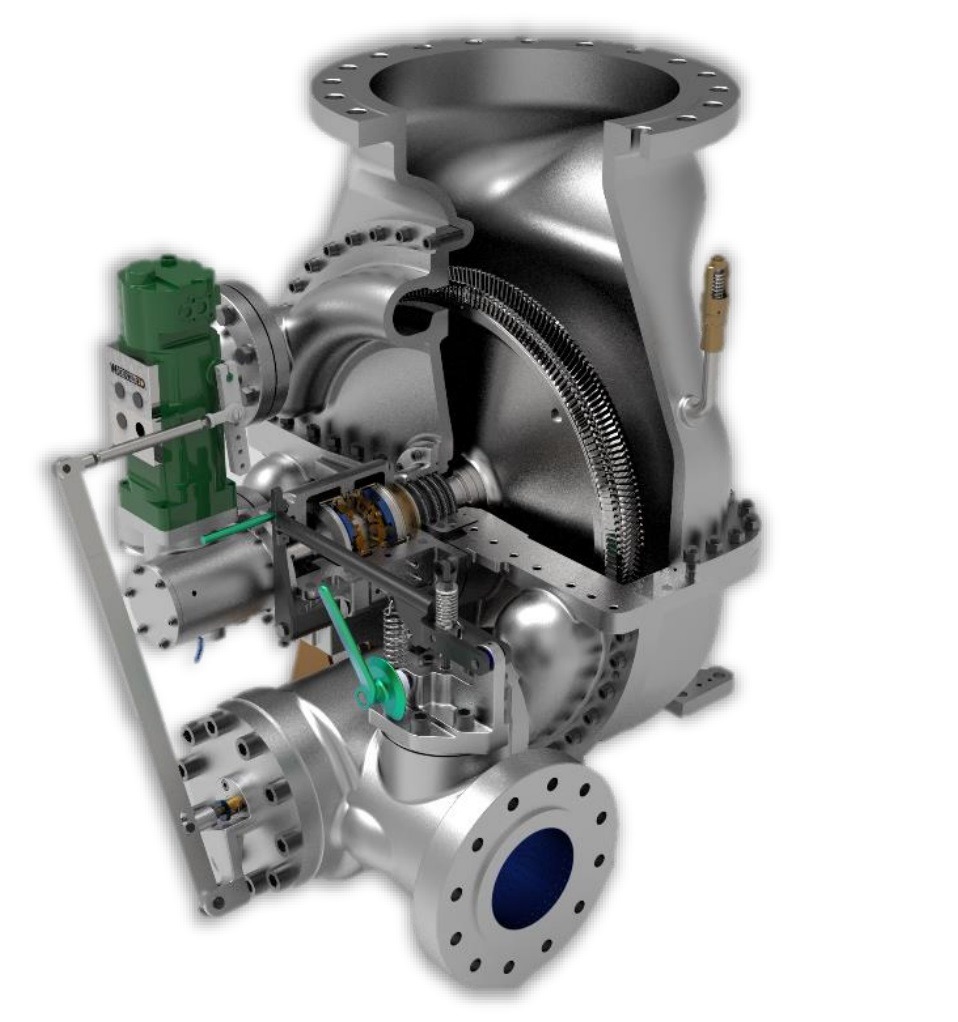
As turbo generators continue to evolve, their integration into the broader framework of smart shipping and automation represents one of the most exciting frontiers in maritime technology. Smart shipping involves the use of advanced digital technologies to optimize the performance, safety, and efficiency of ship operations. In this context, turbo generators are not just power generators but become integral components of a connected ecosystem where data-driven insights guide decision-making.
Automation and autonomy are key elements of this transformation. In autonomous ships, where human intervention is minimized or eliminated, the reliability and self-sufficiency of onboard systems become paramount. Turbo generators, equipped with advanced sensors and autonomous control systems, can monitor and adjust their performance in real-time to adapt to varying operational conditions. This capability ensures that even in the absence of a human crew, the ship’s power generation system can maintain optimal performance and handle unexpected scenarios, such as changes in power demand or system faults.
Cybersecurity is another critical aspect as turbo generators become more connected and reliant on digital control systems. Protecting these systems from cyber threats is essential to prevent disruptions in power supply, which could compromise the safety and operation of the ship. Implementing robust cybersecurity measures, such as encrypted communication channels, secure software updates, and real-time threat detection, is essential to safeguarding the integrity of turbo generators and the broader shipboard systems they support.
The integration of blockchain technology offers potential solutions for enhancing the transparency and security of supply chains and maintenance records for turbo generators. By maintaining a secure, immutable ledger of parts, maintenance activities, and performance data, blockchain can help ensure the authenticity and traceability of components and services. This transparency can improve the reliability of maintenance operations, reduce the risk of counterfeit parts, and streamline regulatory compliance.
Environmental regulations are continuously shaping the design and operation of turbo generators. International standards, such as those set by the International Maritime Organization (IMO), require ships to meet stringent emission targets. Turbo generators must comply with these regulations by incorporating technologies such as selective catalytic reduction (SCR) systems, exhaust gas recirculation (EGR), and advanced emissions monitoring and control systems. These measures help reduce nitrogen oxides (NOx), sulfur oxides (SOx), and particulate matter emissions, ensuring that ships contribute to cleaner air and water.
Energy storage systems, such as batteries and supercapacitors, are increasingly being integrated with turbo generators to enhance their efficiency and flexibility. These systems can store excess energy generated during low demand periods and release it during peak demand, reducing the need for additional fuel consumption and enhancing the overall efficiency of the power system. Energy storage also provides a buffer that can help smooth out fluctuations in power supply, ensuring a stable and reliable energy output.
As the maritime industry progresses towards decarbonization, turbo generators are expected to play a pivotal role in the adoption of zero-emission technologies. Concepts such as fuel cells, which convert chemical energy directly into electrical energy with minimal emissions, are being explored as complementary or alternative power sources. Turbo generators, with their proven efficiency and reliability, can serve as a bridge technology, supporting the transition to these new energy systems while ensuring that ships remain operationally efficient and compliant with evolving environmental standards.
In summary, turbo generators are poised to remain a cornerstone of shipboard power systems, driving the maritime industry’s efforts towards greater efficiency, sustainability, and technological innovation. Their ongoing development, characterized by the integration of smart technologies, alternative fuels, and advanced energy management systems, reflects their critical role in shaping the future of maritime operations. As ships become more autonomous, connected, and environmentally friendly, turbo generators will continue to provide the reliable and efficient power needed to navigate the challenges and opportunities of modern shipping.
Technical Operation and Components
The technical operation of turbo generators on ships is a sophisticated process that revolves around the efficient conversion of thermal energy into electrical energy. This system combines a steam turbine and an electrical generator, forming a compact and powerful unit that meets the energy demands of modern maritime vessels. Understanding the intricate components and operational principles of turbo generators is crucial for appreciating their role in maritime power systems.
At the heart of a turbo generator is the steam turbine, a device that converts the thermal energy of steam into mechanical work. Steam is generated in a boiler, where water is heated to produce high-pressure steam. This steam is then directed through a series of nozzles and blades within the turbine. As the steam expands and loses pressure, it flows over the turbine blades, causing the rotor to spin. The rotational energy produced by the spinning rotor is the mechanical power that drives the next critical component—the electrical generator.
The electrical generator is a device that converts mechanical energy into electrical energy through the principle of electromagnetic induction. The spinning rotor of the steam turbine is connected to the generator’s rotor, which is encased within a stator. The rotor contains a magnetic field, and as it spins within the stator, it induces an electrical current in the stator windings. This current is then harnessed as electrical power, which can be distributed throughout the ship to power various systems and equipment.
The operation of turbo generators is governed by several key parameters, including steam pressure, temperature, and flow rate. These parameters must be precisely controlled to ensure optimal performance and efficiency. The boiler’s operation is critical in this regard, as it must maintain a consistent supply of high-quality steam at the right pressure and temperature. Any variations in steam quality can affect the turbine’s performance, leading to inefficiencies or potential damage.
To manage these parameters, turbo generators are equipped with sophisticated control systems. These systems monitor the operation of the turbine and generator, adjusting the steam flow, temperature, and pressure as needed to maintain stable and efficient operation. Control systems also include safety mechanisms to protect the equipment from conditions that could cause damage, such as over-speed, over-temperature, or excessive vibration.
One of the essential components of the steam turbine is the rotor assembly, which includes the rotor shaft, blades, and seals. The blades are designed to withstand high temperatures and pressures, as well as the forces generated by the steam flow. They are typically made from high-strength alloys that provide the necessary durability and performance. The seals prevent steam from leaking out of the turbine, ensuring that all the energy is used to drive the rotor.
The bearings support the rotor shaft, allowing it to spin freely while minimizing friction and wear. Bearings are critical to the turbine’s operation, as any failure in the bearings can lead to rotor imbalance and potentially catastrophic damage. They are lubricated with oil, which reduces friction and dissipates heat generated during operation. The lubrication system is monitored and maintained to ensure that the bearings remain in good condition.
The condenser is another vital component of the turbo generator system. After the steam has passed through the turbine, it enters the condenser, where it is cooled and condensed back into water. This water is then returned to the boiler to be heated again, completing the cycle. The condenser helps improve the efficiency of the system by recovering and reusing the water, reducing the need for additional fresh water.
In addition to these primary components, turbo generators include auxiliary systems such as the feedwater system, cooling system, and exhaust system. The feedwater system supplies water to the boiler, while the cooling system ensures that the turbine and generator do not overheat during operation. The exhaust system manages the release of steam and gases from the turbine, ensuring that they are safely vented away from the machinery.
The operation of a turbo generator is a continuous process that requires careful monitoring and maintenance. Regular inspections, lubrication, and replacement of worn parts are essential to ensure the longevity and reliability of the system. Advanced diagnostic tools and sensors are often used to monitor the condition of the turbine and generator, detecting issues early before they lead to significant problems.
In summary, the technical operation of turbo generators on ships involves a complex interplay of mechanical and electrical systems designed to convert thermal energy into electrical power efficiently. Each component, from the steam turbine and generator to the control and auxiliary systems, plays a crucial role in ensuring the reliable and efficient performance of the turbo generator. Through precise control, regular maintenance, and the use of advanced technologies, turbo generators continue to be a cornerstone of shipboard power systems, providing the necessary energy to keep modern maritime vessels operational.
The intricacies of turbo generator operation are further enhanced by the integration of monitoring and diagnostic technologies, which play a crucial role in ensuring optimal performance and longevity. These systems use a network of sensors to continuously track the operational parameters of the turbo generator, such as temperature, pressure, vibration, and rotational speed. The data collected by these sensors are processed in real-time by the control system, which can make immediate adjustments to optimize performance and prevent potential failures.
Vibration monitoring is particularly important in the operation of turbo generators. Excessive vibration can be a sign of rotor imbalance, misalignment, or bearing wear. By monitoring vibration levels, operators can identify these issues early and take corrective actions, such as rebalancing the rotor or replacing worn components. This proactive approach helps to prevent more severe damage and reduces downtime, ensuring that the ship’s power supply remains uninterrupted.
Temperature and pressure sensors provide critical information about the conditions within the turbine and the generator. Maintaining the correct temperature and pressure is essential for efficient operation and to prevent overheating or overpressurization, which could lead to equipment failure or safety hazards. The control system uses this data to adjust the steam flow and other operational parameters, ensuring that the turbo generator operates within safe and optimal ranges.
The use of advanced control algorithms and automated control systems has revolutionized the management of turbo generators. These systems can process vast amounts of data and make real-time decisions to optimize the operation of the turbo generator. For example, they can adjust the steam flow to match the electrical load demand, ensuring that the generator operates at its most efficient point. This load-following capability is essential for ships that have varying power demands, such as cruise ships or naval vessels, which may require different amounts of power depending on their activities.
Predictive maintenance is another significant advancement made possible by modern diagnostic technologies. By analyzing the data collected from the turbo generator, predictive maintenance systems can forecast when components are likely to fail or require servicing. This allows maintenance to be scheduled at convenient times, minimizing disruption to ship operations and reducing maintenance costs. Predictive maintenance helps extend the lifespan of the turbo generator and ensures that it remains in peak condition.
Redundancy and reliability are critical considerations in the design of turbo generator systems for ships. Many ships are equipped with multiple turbo generators to provide redundancy, ensuring that power is always available even if one generator fails. This is especially important for vessels that operate in remote areas or undertake long voyages, where access to repair facilities may be limited. Redundant systems are designed to automatically switch over to a backup generator in the event of a failure, providing a seamless transition and maintaining power supply to the ship’s systems.
Environmental considerations are also integral to the operation of turbo generators. With increasing regulations on emissions and fuel efficiency, turbo generators are designed to minimize their environmental impact. This includes the use of advanced combustion technologies to reduce emissions, as well as the implementation of waste heat recovery systems that capture and reuse heat energy from the exhaust, improving overall efficiency.
The integration of turbo generators with shipboard energy management systems is another critical aspect of their operation. These systems provide a holistic view of the ship’s energy use, allowing operators to optimize power distribution and reduce energy consumption. For instance, during periods of low energy demand, the energy management system can reduce the output of the turbo generator or switch to alternative power sources, such as batteries, to conserve fuel. This dynamic energy management helps to lower operating costs and reduces the environmental footprint of the ship.
In conclusion, the technical operation of turbo generators on ships is a complex and finely-tuned process that involves the seamless coordination of numerous components and systems. The integration of advanced monitoring, control, and diagnostic technologies enhances the efficiency, reliability, and sustainability of these systems, making them indispensable to modern maritime operations. As technology continues to advance, turbo generators will remain at the forefront of shipboard power generation, adapting to meet the evolving needs of the maritime industry.
As turbo generators continue to evolve, their role in shipboard power systems becomes increasingly integral to achieving operational efficiency, sustainability, and resilience. The ongoing advancements in materials science and manufacturing techniques have led to the development of more durable and efficient components. For example, the use of advanced alloys and composite materials in turbine blades enhances their ability to withstand high temperatures and pressures, which improves overall efficiency and extends the lifespan of the equipment.
Additive manufacturing, or 3D printing, is another emerging technology that has the potential to revolutionize the production and maintenance of turbo generators. By enabling the rapid and cost-effective production of complex components, additive manufacturing can reduce lead times and inventory costs. It also allows for the production of custom parts that can be tailored to specific operational needs, further enhancing the performance and efficiency of the turbo generator.
The adoption of artificial intelligence (AI) and machine learning (ML) in the control and monitoring of turbo generators is a growing trend. These technologies can analyze vast datasets to identify patterns and insights that might be missed by traditional monitoring systems. AI-driven predictive analytics can enhance the accuracy of maintenance predictions, optimize operational parameters in real-time, and improve the overall reliability of the system. Machine learning algorithms can continuously learn and adapt to changing operating conditions, ensuring that the turbo generator operates at peak efficiency under a wide range of scenarios.
Integration with renewable energy sources is another area of development for turbo generators. As the maritime industry seeks to reduce its reliance on fossil fuels and lower greenhouse gas emissions, the ability to integrate with renewable energy systems becomes increasingly important. Turbo generators can be paired with energy storage systems and renewable energy sources, such as solar panels or wind turbines, to create a hybrid power system. This setup allows ships to maximize the use of clean energy while ensuring a reliable backup power source through the turbo generator.
Energy recovery systems are also being incorporated into modern turbo generator designs to enhance energy efficiency. For instance, exhaust gas heat recovery systems capture waste heat from the turbine exhaust and use it to preheat the feedwater entering the boiler. This reduces the amount of fuel needed to generate steam, improving overall fuel efficiency and reducing emissions. Other energy recovery technologies, such as combined heat and power (CHP) systems, can further enhance the energy efficiency of the ship by using waste heat for additional onboard energy needs.
Noise reduction technologies are becoming increasingly important for turbo generators, particularly on passenger ships and vessels operating in noise-sensitive environments. Advanced acoustic enclosures, vibration damping systems, and optimized blade designs help minimize the noise produced by the turbo generator, improving comfort for passengers and crew and reducing the environmental impact of noise pollution in marine habitats.
In terms of future developments, the concept of fully autonomous turbo generators is being explored. These systems would be capable of self-regulating and self-maintaining, requiring minimal human intervention. Autonomous turbo generators would use a combination of AI, robotics, and advanced sensors to monitor their condition, perform routine maintenance tasks, and adapt to changing operational conditions. This level of autonomy could significantly reduce operational costs and improve the reliability of shipboard power systems.
Research and development in the field of turbo generators are also focused on improving the adaptability of these systems to alternative fuels, such as hydrogen and ammonia, which are seen as promising options for decarbonizing the maritime industry. The development of turbines and generators that can efficiently operate with these fuels is crucial for the transition to a low-carbon shipping industry.
In conclusion, turbo generators are a vital component of modern shipboard power systems, combining advanced engineering, cutting-edge technology, and sustainable practices to meet the growing demands of the maritime industry. Their evolution reflects a broader shift towards greater efficiency, environmental responsibility, and technological integration in maritime operations. As the industry continues to innovate, turbo generators will remain at the core of these advancements, driving progress and shaping the future of maritime power systems.
Applications and Advantages
Turbo generators play a crucial role in the maritime industry, providing reliable power generation on ships. Their applications span a wide range of vessels, from commercial and passenger ships to military and research vessels, where they serve as the primary source of electrical energy. The versatility and efficiency of turbo generators make them an essential component of modern shipboard power systems. By converting thermal energy from steam into mechanical energy, and subsequently into electrical power, turbo generators ensure that ships can operate smoothly and meet their energy demands while at sea.
One of the most significant advantages of turbo generators is their high efficiency. Steam turbines, when combined with modern generators, can operate at a high level of thermal efficiency. This means that they can extract more energy from the fuel used, making them highly efficient for power generation. On large vessels, the ability to generate substantial amounts of electricity with relatively low fuel consumption is a key factor in reducing operating costs and enhancing the economic viability of maritime operations. Furthermore, turbo generators are capable of maintaining stable and reliable output, even in harsh operational environments, which is essential for ships that operate in remote areas or under challenging conditions.
Another advantage of turbo generators is their reliability. As ships are often required to operate in isolated areas where access to maintenance and repair services is limited, having a reliable power generation system is crucial. Turbo generators are designed to withstand the rigors of maritime operations, including vibrations, saltwater exposure, and fluctuating environmental conditions. Their robust construction ensures long-lasting performance, even under continuous use. This reliability is critical not only for maintaining power supply to essential shipboard systems, but also for ensuring the safety and comfort of passengers and crew.
Fuel flexibility is another notable advantage of turbo generators. While traditional turbo generators are designed to run on heavy fuel oil (HFO), advances in technology have made it possible for these systems to operate efficiently with a variety of fuels, including marine diesel oil (MDO), liquefied natural gas (LNG), and even alternative fuels such as hydrogen or ammonia. This flexibility allows ship operators to choose fuels based on availability, cost, and environmental considerations. As the shipping industry moves towards cleaner fuels to meet stringent emissions regulations, turbo generators that can operate with alternative fuels will become increasingly important in reducing the carbon footprint of maritime operations.
Turbo generators are also known for their compactness and ability to integrate into a ship’s power generation system. Unlike older mechanical systems, which might require large amounts of space and complex infrastructure, modern turbo generators are designed to be more space-efficient. This compactness is particularly beneficial for vessels that have limited space for power generation equipment. Additionally, the integration of turbo generators with other shipboard systems, such as energy storage solutions, energy management systems, and renewable energy sources, enhances their versatility and allows for more efficient power distribution throughout the ship.
In terms of environmental benefits, turbo generators help ships comply with increasingly strict emissions regulations imposed by organizations like the International Maritime Organization (IMO). By operating at high efficiency, turbo generators contribute to fuel savings, which in turn reduces the overall carbon emissions of the vessel. Furthermore, with the growing use of scrubbers, exhaust gas recirculation (EGR), and selective catalytic reduction (SCR) systems, turbo generators can be optimized to meet the demanding emissions standards. This helps mitigate the impact of shipping on the environment, particularly in terms of sulfur oxide (SOx), nitrogen oxide (NOx), and particulate matter emissions.
Another key advantage of turbo generators is their long operational life. With proper maintenance and periodic overhauls, turbo generators can operate efficiently for many years. Their robust design and ability to handle the high stresses and temperatures encountered during operation ensure that they are a durable and cost-effective solution for power generation on ships. Additionally, because turbo generators are often used in critical systems, such as propulsion and auxiliary power, their longevity and reliability are essential for ensuring the safe and continuous operation of the vessel.
The ability to handle varying loads is another feature that makes turbo generators well-suited to maritime applications. On many ships, power demand fluctuates depending on the ship’s activities, such as cruising, docking, or cargo handling. Turbo generators are capable of adjusting their output to match the load, providing the necessary energy when it is needed and reducing fuel consumption when demand is low. This dynamic load-following ability makes turbo generators a versatile and adaptable power solution for a wide range of maritime operations.
Noise and vibration reduction technologies have also been incorporated into turbo generators, particularly on passenger vessels, where comfort is a key concern. The combination of advanced turbine blade design, vibration damping materials, and noise isolation measures helps minimize the acoustic impact of turbo generators on the ship’s interior, ensuring a more pleasant experience for passengers and crew. These innovations are especially important on luxury cruise liners and other vessels where a quiet, comfortable environment is a priority.
Finally, turbo generators contribute to enhanced energy security for ships. By providing a reliable and independent power source, turbo generators ensure that essential systems—such as navigation, communications, and safety systems—remain operational even in the event of a failure in other parts of the ship’s power grid. This is particularly important for vessels operating in remote locations, such as deep-sea research ships or offshore platforms, where access to external power sources is limited.
In summary, turbo generators offer a range of applications and advantages that make them a cornerstone of modern maritime power generation. Their efficiency, reliability, and fuel flexibility, combined with their compact design and environmental benefits, make them an essential component for ships of all sizes. As the maritime industry continues to evolve, turbo generators will remain a critical element in driving operational efficiency, sustainability, and safety.
In addition to the advantages already outlined, the ongoing technological advancements in turbo generators are further enhancing their performance and making them even more beneficial for maritime applications. These innovations aim to improve power output, reduce operating costs, and ensure that turbo generators are equipped to handle the demands of future shipping.
One such innovation is the development of advanced control systems. These systems use sophisticated algorithms and real-time data analysis to optimize the operation of turbo generators. By adjusting operational parameters like steam flow, temperature, and pressure, modern control systems ensure that turbo generators operate at peak efficiency across a variety of operational conditions. The integration of artificial intelligence (AI) and machine learning (ML) into these control systems further enhances their capability to predict power demand, adjust generator output dynamically, and maintain optimal operational efficiency while minimizing fuel consumption and emissions. This predictive control not only reduces the risk of system failures but also extends the service life of the components by ensuring they are not overworked.
Another area of development is the integration of turbo generators with hybrid power systems. These systems combine turbo generators with other power generation sources, such as batteries, fuel cells, or renewable energy sources like solar or wind. By utilizing hybrid systems, ships can optimize their energy use, reducing dependence on fossil fuels and lowering operating costs. For example, during low-demand periods, the ship might rely on stored energy from batteries or solar panels, while turbo generators can be used when higher power outputs are needed. This integration allows for a smoother transition between different power sources, reducing wear and tear on the turbo generator and contributing to a more sustainable energy mix.
The maritime industry is also moving toward decarbonization and sustainable shipping as part of broader efforts to reduce the environmental impact of transportation. Turbo generators are central to this transition. Their ability to run efficiently on alternative fuels, such as LNG or hydrogen, positions them as key players in reducing carbon emissions from ships. The development of turbo generators capable of operating on zero-emission fuels is expected to gain momentum in the coming years. LNG, for instance, is a cleaner alternative to traditional marine fuels, emitting fewer particulate matter and NOx emissions, while hydrogen holds the promise of even lower emissions, producing only water vapor as a byproduct when burned.
As the demand for eco-friendly shipping increases, turbo generators that can use biofuels or synthetic fuels will become increasingly relevant. These fuels are made from renewable resources or synthetic processes, and their use can significantly reduce the carbon footprint of maritime operations. Turbo generators that are compatible with these fuels can contribute to the decarbonization of the maritime sector, ensuring compliance with evolving regulations set by the International Maritime Organization (IMO) and other governing bodies.
Modular and scalable designs are another emerging trend in turbo generator technology. By designing turbo generators with modular components, manufacturers can provide more adaptable systems that can be scaled up or down based on the specific energy needs of the vessel. This allows for greater flexibility in ship design and operation, making it easier to retrofit existing vessels with more efficient and environmentally friendly power generation systems.
Moreover, remote monitoring and diagnostic capabilities are transforming how turbo generators are operated and maintained. With the advancement of IoT (Internet of Things) technologies, turbo generators are increasingly connected to centralized monitoring systems that track performance data and operational status in real time. These systems allow operators to remotely assess the condition of the turbo generator, receive alerts for potential issues, and even make adjustments to optimize performance. Remote diagnostics also enable more efficient and timely maintenance, as technicians can identify and address issues before they lead to system failures or costly repairs.
These innovations also lead to a shift in how maintenance is approached. The use of predictive maintenance driven by real-time data allows operators to avoid unnecessary downtime and repair costs. By monitoring wear and tear on components such as turbine blades, bearings, and seals, maintenance can be performed at the optimal time rather than based on a fixed schedule. This not only improves the availability of the turbo generators but also reduces the overall maintenance costs for ship operators.
The combination of efficiency improvements, fuel flexibility, and advanced technologies positions turbo generators as indispensable for the future of maritime power generation. As global shipping continues to face pressure to reduce emissions and increase sustainability, turbo generators will remain central to the shift toward greener, more efficient vessels. Their versatility, reliability, and ability to adapt to emerging energy sources make them a foundational technology for the ongoing evolution of the maritime industry.
In conclusion, turbo generators offer a multitude of applications and advantages that make them a critical component in modern shipboard power systems. Their ability to provide reliable and efficient power, combined with advancements in technology that improve performance, reduce environmental impact, and enhance operational flexibility, ensures that turbo generators will continue to play a vital role in the maritime industry for years to come. The ongoing innovations and adaptations in turbo generator design, control systems, and fuel compatibility further solidify their position as one of the most important technologies for shaping the future of sustainable shipping.
As the maritime industry looks to the future, the adoption of digital technologies is expected to further enhance the performance and integration of turbo generators. Digitalization enables operators to not only monitor the performance of turbo generators in real-time but also collect vast amounts of data that can be analyzed to improve operational efficiency and extend equipment life. Advanced data analytics tools, powered by artificial intelligence (AI) and machine learning (ML), will be able to identify trends, predict failures, and suggest optimizations based on historical performance data.
This capability will be particularly important in helping ship operators optimize fuel consumption, as fuel is one of the most significant operational costs for vessels. By leveraging data from turbo generators and other ship systems, operators can fine-tune the entire power generation system to ensure that the right amount of energy is produced based on real-time demand. This can lead to significant fuel savings over the long term, reduce the environmental impact of fuel consumption, and help vessels meet stricter emissions standards.
Cybersecurity is also becoming an increasingly important aspect of turbo generator operation as digital systems become more interconnected. The rise of remote monitoring systems and automated control systems exposes turbo generators to potential cybersecurity threats, such as data breaches, hacking, and system manipulation. Therefore, ensuring the security of digital control systems is essential for safeguarding the performance and reliability of the turbo generator. Robust cybersecurity measures, including encryption, firewalls, and access controls, will be critical to maintaining the integrity and safety of the ship’s power generation systems.
The integration of turbo generators with autonomous systems is another exciting development on the horizon. With the growing interest in autonomous shipping, the ability to integrate turbo generators into self-regulating, automated ship systems is gaining momentum. These autonomous systems can optimize power generation, predict maintenance needs, and adjust operational parameters based on real-time data without human intervention. Turbo generators on autonomous ships could be part of a fully integrated system that allows the ship to operate with minimal oversight, improving efficiency and reducing the need for manual operation.
In terms of future fuel technologies, the development of methanol as an alternative maritime fuel is gaining attention. Methanol is a cleaner, more sustainable fuel option compared to conventional marine fuels, and its use in turbo generators could become more widespread in the coming years. Methanol can be produced from renewable sources such as biomass or even from carbon captured from the atmosphere, making it a potentially carbon-neutral fuel. Turbo generators designed to run on methanol would provide a cleaner alternative to traditional fuels while maintaining the performance and efficiency expected of modern power generation systems.
In addition to methanol, ammonia is being explored as a potential zero-carbon fuel for the maritime industry. Ammonia can be used in turbo generators as an alternative to conventional fuels, with the added benefit of producing no CO2 emissions when burned. However, ammonia’s use is still being studied in terms of safe storage, handling, and combustion, and further research will be required to fully optimize turbo generators to run on this fuel. If successfully integrated, ammonia-powered turbo generators could play a major role in achieving the maritime industry’s decarbonization goals.
Another significant advancement is the development of hybrid energy storage solutions. Batteries and other energy storage systems are increasingly being used alongside turbo generators to improve the flexibility of power generation systems. Batteries can store excess energy generated by the turbo generator during periods of low demand, which can then be discharged when higher power is needed, such as during peak demand or while navigating in specific operational conditions. This hybrid approach helps reduce fuel consumption and improve overall efficiency by utilizing stored energy rather than running the turbo generator at full capacity during periods of low energy demand.
The potential for integration with shore power systems is also an emerging area for turbo generators, particularly for vessels operating in port areas. In many ports around the world, there are initiatives to provide shore power (also known as cold ironing) to ships while they are docked, allowing them to switch off their turbo generators and plug into the grid. This reduces emissions in port areas and eliminates the need to burn fuel while the vessel is at rest. Ships that can seamlessly transition between turbo generator power and shore power are well-positioned to comply with environmental regulations and contribute to cleaner air in port cities.
With the continued pressure to reduce emissions and improve energy efficiency, carbon capture and storage (CCS) technologies could be an integral part of turbo generator systems in the future. CCS involves capturing CO2 emissions produced during power generation and storing them safely underground or converting them into useful products. This technology could help mitigate the environmental impact of turbo generators, particularly for those using fossil fuels. As CCS technologies advance, they may become a viable option for reducing the carbon footprint of maritime power generation.
The demand for sustainable shipping is also driving the development of turbo generators that are capable of running on sustainable biofuels. These biofuels, derived from renewable biological sources such as algae or plant-based oils, offer a more environmentally friendly alternative to traditional marine fuels. As the maritime industry increasingly shifts toward renewable energy sources, the compatibility of turbo generators with biofuels will play a critical role in achieving the industry’s sustainability targets.
Collaboration among industry stakeholders will be vital in addressing the challenges and opportunities associated with turbo generators and their future applications. Ship owners, manufacturers, and regulatory bodies must work together to ensure that turbo generators meet the evolving demands of the maritime industry. Through innovation and collaboration, turbo generators will continue to evolve as a reliable, efficient, and sustainable power source for vessels worldwide.
In summary, the ongoing development of turbo generators, including advancements in fuel flexibility, integration with renewable energy systems, hybrid power solutions, and digital technologies, ensures that these systems will remain at the core of shipboard power generation. As the maritime industry embraces digitalization, autonomous shipping, and decarbonization, turbo generators will continue to play an essential role in providing clean, reliable, and efficient energy solutions for the future of maritime operations. The future of turbo generators looks bright, with a growing emphasis on sustainability, innovation, and the ability to adapt to new energy sources and technologies.
Looking to the future, turbo generators will increasingly be influenced by global regulatory frameworks aimed at reducing the environmental impact of maritime operations. As nations and international organizations set more stringent regulations around emissions and fuel usage, turbo generators will need to meet increasingly demanding standards. The International Maritime Organization (IMO) has already laid out ambitious targets for reducing greenhouse gas (GHG) emissions from shipping, and turbo generators will play a crucial role in helping vessels achieve these goals. This will include not only adapting to cleaner fuels like LNG, hydrogen, and ammonia, but also incorporating more efficient energy generation systems that minimize waste and optimize fuel consumption.
Energy efficiency measures are likely to be a major focus of turbo generator technology development. Beyond fuel efficiency, advancements in heat recovery and waste energy capture systems will allow ships to make better use of the energy they generate. Combined heat and power (CHP) systems or cogeneration systems could become more common in marine applications, where the waste heat produced by turbo generators is used to produce steam for other systems on the ship, such as heating or even propulsion. By maximizing the use of generated heat, these systems can improve overall energy efficiency, reduce fuel consumption, and minimize environmental impact.
Additionally, the integration of smart grid technology into shipboard power systems may enhance the capabilities of turbo generators. Smart grids can allow for the efficient distribution of power within the ship, ensuring that turbo generators are utilized optimally and that excess energy is stored or distributed to where it is most needed. By incorporating AI-driven algorithms, smart grids can monitor real-time energy usage across the ship, making adjustments to minimize waste and ensure the power system is running efficiently. This could have significant economic benefits by reducing energy costs and improving operational uptime.
Another avenue for turbo generator development lies in alternative marine propulsion systems. While turbo generators are already essential for generating electrical power for a variety of shipboard functions, the integration of hybrid and alternative propulsion systems, such as wind-assisted propulsion or fuel cell technology, may reduce reliance on traditional turbo generators for propulsion power. In such systems, turbo generators could be used for auxiliary power or as backup systems, ensuring that the vessel remains operational even when the primary propulsion system is not in use.
Turbo generators could also see innovations in automation and robotics for maintenance and operational oversight. The future of shipboard operations is moving towards automation and autonomy, where turbo generators could be monitored, adjusted, and maintained by autonomous systems. These systems could be equipped with predictive analytics and diagnostic capabilities that would allow for early detection of faults, minimizing the need for human intervention. In cases of component failure, robotic maintenance systems could perform basic repairs, ensuring that the turbo generator remains operational without requiring extensive downtime.
As shipping continues to embrace decarbonization and a transition to greener and more sustainable practices, turbo generators will be key in ensuring that vessels meet the environmental standards set by regulatory bodies. Their role will not only focus on efficiency and reliability but also on reducing carbon emissions and enabling vessels to run on cleaner fuels. The technological advancements in turbo generators are aligned with the industry’s larger goals of achieving a sustainable and eco-friendly future for shipping.
The global supply chain will also benefit from advancements in turbo generator technology. Efficient power generation leads to improved operational efficiency and fuel savings for shipping lines, which can pass on these savings to customers. By reducing the overall operating costs of vessels, turbo generators help companies stay competitive in a challenging global market, while also supporting sustainable practices that are increasingly demanded by stakeholders and consumers.
Additionally, the growth of electrification in the maritime industry could play a key role in the evolution of turbo generators. As more ships begin to rely on electric propulsion systems, turbo generators will need to adapt to supply electricity to these systems. Future turbo generators might be designed specifically to integrate seamlessly with fully electric or hybrid propulsion systems, ensuring that the vessels’ power needs are met regardless of the operational mode.
International collaboration will be essential for accelerating the development and deployment of next-generation turbo generators. Researchers, engineers, and manufacturers must work closely with policymakers, fuel suppliers, and shipping companies to develop systems that meet regulatory standards while also reducing the environmental impact of maritime transport. Additionally, shared knowledge across industries could lead to faster implementation of sustainable solutions that benefit the entire shipping ecosystem.
In conclusion, turbo generators are poised to continue playing a critical role in the future of maritime power generation. Their ability to evolve alongside emerging technologies, cleaner fuels, and greener practices will be key to their continued relevance in the shipping industry. As innovation accelerates, turbo generators will become even more efficient, adaptable, and environmentally friendly, ensuring that they remain an essential part of the future maritime landscape.
As the maritime industry advances, sustainability will become an even more integral part of the design, operation, and function of turbo generators. Shipping companies, facing increasing pressure from both regulators and the public to reduce their environmental impact, will increasingly invest in technology that supports both their economic viability and environmental responsibility. One significant trend will be the growing interest in carbon-neutral technologies, with turbo generators evolving to run on fuels that produce zero or near-zero emissions.
With the emphasis on green shipping, turbo generators capable of operating with renewable energy sources will likely be more in demand. Solar panels, wind turbines, and even ocean-based energy solutions such as wave or tidal energy can be integrated into ship power systems, with turbo generators supplying auxiliary power or adjusting to fluctuating energy inputs. These technologies could complement one another in hybrid systems, allowing vessels to reduce their reliance on traditional marine fuels while still maintaining stable power generation.
Moreover, the use of fuel cells as a complementary power source for turbo generators could be a key area of future development. Fuel cells, which convert chemical energy directly into electrical energy, offer high efficiency and low emissions compared to conventional combustion engines. Integrating fuel cells with turbo generators could allow ships to generate cleaner power while reducing emissions of greenhouse gases and pollutants. Combined with battery storage systems, fuel cells could offer an energy-efficient, low-emission alternative to conventional turbo generators for certain types of vessels, particularly those operating in emission-controlled areas (ECAs).
The development of hybrid energy storage systems will also have significant implications for turbo generators. As ships move towards more advanced electrification and reliance on electric propulsion, turbo generators will become part of increasingly complex systems that manage a mix of fuel-based and electric power. Batteries, supercapacitors, and other energy storage devices could work alongside turbo generators, helping to balance supply and demand while reducing fuel consumption. In this context, turbo generators may serve as the primary energy source, with batteries providing peak shaving (covering short-term surges in power demand) and acting as backups during periods of low energy production.
The growing trend toward smart shipping will also require turbo generators to be integrated into more intelligent, automated systems. Ships will become more connected through IoT devices and other smart technologies, allowing turbo generators to communicate with other shipboard systems to optimize energy distribution and consumption. For instance, if the vessel’s speed is reduced, the power demand will likely drop, allowing the turbo generator to operate more efficiently. Automated systems could automatically adjust the turbo generator’s output to match the ship’s current power requirements, thereby optimizing fuel usage and minimizing emissions. This type of automation could reduce the need for manual intervention and improve the overall performance of the ship’s power systems.
As shipyards and naval architects continue to embrace green technologies, turbo generators will likely see further refinement in terms of their compactness and efficiency. The space available on ships is often limited, and turbo generators are already highly optimized to fit within these constraints. However, as ships continue to grow in size and complexity, manufacturers will focus on making turbo generators more space-efficient, with enhanced modularity and scalability to fit the unique needs of various vessels. These modular turbo generators could be configured to meet specific power demands, offering greater flexibility for both shipbuilders and operators.
Furthermore, energy management systems (EMS) will increasingly become part of turbo generator technologies. EMS can monitor energy production, consumption, and storage in real-time, optimizing the distribution of power throughout the ship. This system can ensure that power from the turbo generator is used as efficiently as possible, adjusting for load fluctuations, and ensuring that auxiliary systems (such as lighting, air conditioning, and water desalination) receive the power they need without compromising the main propulsion system’s operation. EMS could also integrate with smart grid systems on board, enabling better management of power from renewable sources and reducing reliance on traditional marine fuels.
The emphasis on zero-emissions technologies will likely bring about new partnerships between fuel suppliers, technology developers, and shipowners. For instance, manufacturers of green fuels such as bio-methanol or bio-LNG may collaborate with turbo generator producers to develop optimized systems capable of efficiently running on these alternative fuels. The fuel industry is also expected to expand the availability of sustainable fuels that are compatible with turbo generators, ensuring that maritime operators have a reliable source of low-carbon energy to meet increasingly stringent emissions regulations.
In addition to fuel diversification, turbo generators will benefit from advancements in heat recovery systems that can help further reduce a ship’s environmental impact. For example, capturing waste heat from the exhaust gases of turbo generators or other engines on the ship and converting it into usable energy can improve the vessel’s overall energy efficiency. This energy recovery could be directed towards heating, desalination, or even auxiliary propulsion, reducing the vessel’s dependence on fuel and lowering its carbon emissions.
The role of turbo generators will also extend to electric propulsion technologies. While turbo generators are primarily used for producing electrical power, their integration with electric propulsion systems will be key to the ongoing transition to greener ships. Electric propulsion is becoming more common on both large commercial vessels and smaller passenger ships, as it offers a cleaner, quieter alternative to traditional mechanical propulsion systems. Turbo generators will be responsible for supplying the electrical energy needed to power electric motors, helping these vessels to operate more efficiently and with fewer emissions.
The global push for sustainable maritime transport is supported by the growing popularity of environmental certification programs and green ship initiatives. Certification programs such as the Energy Efficiency Existing Ship Index (EEXI) and Carbon Intensity Indicator (CII) are pushing shipowners to adopt cleaner technologies, including more efficient turbo generators. These certifications encourage the adoption of energy-saving devices, including turbo generators designed for lower emissions and better fuel efficiency. The pressure to meet these standards is driving innovation, ensuring that turbo generators continue to evolve in line with environmental expectations.
Ultimately, turbo generators will continue to be an essential component of the energy ecosystem on ships, ensuring that vessels are both economically efficient and environmentally responsible. Their role in improving fuel efficiency, reducing emissions, and supporting the use of alternative fuels and renewable energy sources will be crucial in the evolution of the global maritime industry.
In conclusion, the future of turbo generators is tightly interwoven with broader trends in the maritime industry, including decarbonization, digitalization, and energy diversification. With a focus on sustainability and technological innovation, turbo generators will evolve to meet the changing needs of the shipping industry, providing reliable, efficient, and eco-friendly power generation for vessels of all sizes. The continued collaboration between stakeholders in the shipping, fuel, and technology sectors will ensure that turbo generators remain a central component of the maritime energy landscape for years to come.
Challenges and Innovations
The development and implementation of turbo generators on ships face a range of challenges, many of which are driven by the need to meet increasingly stringent regulatory requirements, the evolution of fuel technologies, and the growing emphasis on sustainability. While turbo generators have proven to be reliable and efficient components of marine power systems, the challenges that accompany their use require constant innovation and adaptation to new circumstances.
One of the primary challenges is fuel efficiency. Turbo generators must be able to operate efficiently over a wide range of conditions, from full-load operation during high-speed cruising to partial-load conditions during slow steaming. The ability to maintain high levels of efficiency in these varying conditions is crucial for reducing operational costs and minimizing environmental impact. Furthermore, fuel consumption remains one of the largest contributors to a vessel’s operational expenses. As the maritime industry faces pressure to cut down on emissions and reduce fuel consumption, turbo generators need to evolve to be more fuel-efficient, potentially by incorporating new materials, advanced control systems, or optimized turbine designs that reduce fuel consumption and improve performance.
The increasing demand for environmentally friendly technologies presents another challenge. The maritime industry is under growing pressure to reduce its carbon footprint, driven by international regulations such as the IMO’s greenhouse gas reduction targets and regional initiatives aimed at controlling air pollution in port cities. This requires the development of turbo generators that can operate on alternative fuels such as liquefied natural gas (LNG), hydrogen, ammonia, and biofuels, all of which present distinct operational challenges compared to traditional fuels like marine diesel. These alternative fuels often have different energy densities, combustion characteristics, and storage requirements, meaning that turbo generators must be adapted or entirely redesigned to operate efficiently and safely with them.
In particular, the use of hydrogen and ammonia as fuels for turbo generators presents significant hurdles. Hydrogen, for instance, has a very low energy density by volume, requiring advanced storage and distribution systems to be integrated into the ship’s design. Similarly, ammonia is toxic and poses a risk to crew safety, necessitating the development of systems that ensure safe handling, storage, and combustion. In addition, the combustion of ammonia can produce nitrogen oxide (NOx) emissions, which would require advanced scrubbing systems to minimize their environmental impact.
Another challenge is technological obsolescence. As ship operators strive to meet evolving environmental regulations, there is a risk that existing turbo generators may become obsolete or too expensive to retrofit with the required new technologies. The integration of new control systems, advanced monitoring tools, and fuel systems into existing turbo generators could be costly and complex, especially when considering the varying designs and specifications of vessels. Consequently, manufacturers must find ways to make new turbo generator models more modular and adaptable, allowing shipowners to upgrade their systems incrementally rather than investing in entirely new power generation solutions. This is particularly important for vessels with long operational lifespans, as retrofitting technology must remain flexible enough to accommodate changes in regulations and operational requirements over time.
Space constraints onboard vessels also present an ongoing challenge. Turbo generators must be designed to fit within the limited space available on ships, which often requires compromises in terms of size, weight, and efficiency. Moreover, space limitations extend to the ancillary systems that turbo generators require, such as exhaust systems, fuel delivery systems, and waste heat recovery units. As ships are increasingly outfitted with new systems for sustainability and efficiency, ensuring that there is enough space for these systems, without negatively impacting turbo generator performance or vessel operation, will require continued innovation in both design and technology.
The evolving nature of the digital and automation landscape also poses challenges. While modern turbo generators can be equipped with digital control systems, sensors, and predictive analytics tools to improve efficiency and anticipate maintenance needs, these systems come with their own set of challenges. One of the key issues is cybersecurity. As ships become more reliant on connected systems, the risk of cyber-attacks on shipboard equipment—including turbo generators—becomes a significant concern. Hackers could potentially interfere with the operation of turbo generators, either by manipulating their settings or causing disruptions in power generation, leading to operational failures or even safety hazards. Protecting the cyber infrastructure of turbo generators will be crucial as the industry becomes increasingly digitized.
Moreover, the increasing complexity of turbo generator systems, as well as the integration with smart grids, autonomous systems, and renewable energy sources, presents the need for highly skilled operators and maintenance personnel. The workforce must be trained to manage and maintain these advanced systems, and the required skill sets may differ significantly from those needed to operate traditional, mechanical power systems. Ensuring that personnel have the necessary training to operate and troubleshoot these new systems is vital for maintaining the reliability and efficiency of turbo generators.
Another significant challenge is global supply chain issues. The availability of high-quality materials and components for turbo generators is essential for maintaining high standards of performance and reliability. However, supply chain disruptions, which have become more frequent in recent years due to geopolitical tensions, economic instability, or natural disasters, could delay the production of critical components or make them more expensive. This could result in longer downtimes for vessels undergoing maintenance or retrofitting, increasing operational costs for ship operators and affecting the overall efficiency of the shipping industry.
Despite these challenges, innovation within the field of turbo generators has been advancing steadily. Engineers and researchers are continuously developing new technologies to overcome these obstacles and improve the performance, efficiency, and sustainability of turbo generators. Some of the key innovations that are shaping the future of turbo generators include additive manufacturing, which allows for the creation of more complex, lightweight components; the use of advanced coatings and materials to improve turbine durability and reduce wear and tear; and intelligent control systems that optimize turbo generator performance based on real-time data analysis.
Moreover, hybrid systems that combine turbo generators with energy storage technologies or fuel cells are being developed to improve overall efficiency and reduce emissions. By storing excess energy in batteries or supercapacitors, these hybrid systems can help to ensure that turbo generators operate at peak efficiency and reduce the need to rely on conventional fuels. These systems are particularly valuable in maritime applications where energy demand can fluctuate rapidly, as they provide a more flexible, responsive solution for power generation.
Finally, collaboration between various stakeholders—shipowners, manufacturers, regulatory bodies, and research institutions—will play a crucial role in overcoming these challenges. By working together, these groups can share knowledge, pool resources, and develop innovative solutions that address the complex issues facing turbo generator technology. This collaboration will be essential in driving forward the development of turbo generators that meet the needs of the evolving maritime industry, ensuring that vessels can operate more efficiently, sustainably, and safely in the years to come.
In summary, while turbo generators continue to be a critical component of modern marine power systems, the challenges they face in terms of fuel efficiency, environmental impact, technological integration, and operational complexity are significant. However, ongoing research and development, alongside innovations in materials, control systems, and hybrid energy solutions, are helping to overcome these challenges. As the maritime industry moves toward greener, more efficient technologies, turbo generators will continue to evolve to meet the demands of a more sustainable future.
Future Prospects and Sustainability
The future of turbo generators on ships is deeply intertwined with the broader trends in the maritime industry, particularly those focused on sustainability, energy efficiency, and regulatory compliance. As global pressures to reduce emissions and improve fuel efficiency intensify, turbo generators will evolve to meet these demands. Their role in the energy systems of ships is expanding, and they are likely to become even more critical in the transition to greener, more sustainable maritime transport. The development of turbo generators that can operate with cleaner fuels, reduce emissions, and integrate with renewable energy sources is expected to be a key factor in the future of shipping.
One of the most significant shifts that will shape the future of turbo generators is the global push toward decarbonization. As international bodies such as the International Maritime Organization (IMO) set increasingly stringent targets for reducing greenhouse gas emissions, the shipping industry must find ways to meet these goals while maintaining operational efficiency. Turbo generators will play a pivotal role in this transition by improving energy efficiency and facilitating the use of low-carbon fuels, such as liquefied natural gas (LNG), hydrogen, ammonia, and biofuels. These fuels produce fewer carbon emissions than traditional marine fuels, and turbo generators will need to be adapted or redesigned to accommodate their unique properties.
Hydrogen, in particular, holds great promise as a clean fuel for turbo generators. With no carbon emissions when burned, hydrogen could help vessels achieve near-zero emissions, making it an attractive option for meeting decarbonization targets. However, hydrogen presents several technical challenges, including its low energy density and the need for specialized storage and handling systems. For turbo generators to operate efficiently with hydrogen, they will need to be redesigned to handle its unique combustion characteristics and integrate with fuel cells and other energy systems. This may require the development of advanced materials, more efficient combustion systems, and safety protocols for handling this highly flammable fuel.
Ammonia, another potential fuel for turbo generators, also presents opportunities for reducing carbon emissions. While ammonia is carbon-free, it is toxic and poses significant safety risks to crew members and the environment. As such, turbo generators that run on ammonia will need to incorporate advanced safety measures, including leak detection systems and robust fuel handling protocols. Additionally, the combustion of ammonia can produce nitrogen oxides (NOx), which contribute to air pollution. Turbo generators that operate on ammonia will need to be equipped with advanced emissions control systems, such as selective catalytic reduction (SCR) technology, to reduce NOx emissions and meet environmental standards.
The growing reliance on renewable energy sources in the maritime sector will also influence the future design and operation of turbo generators. The integration of renewable energy systems such as wind, solar, and wave energy into the power grid of ships could reduce the need for traditional fuel consumption. Turbo generators could be used to supply backup power when renewable sources are insufficient or to support the overall power needs of the vessel. The development of hybrid power systems, which combine turbo generators with renewable energy sources and energy storage systems, could help optimize fuel consumption and reduce the environmental impact of vessels. For instance, excess power generated by solar panels could be stored in batteries or used to supplement the turbo generator’s output during periods of high demand.
In addition to fuel diversification, energy efficiency will continue to be a central focus of turbo generator innovation. The pressure to reduce fuel consumption and operational costs means that turbo generators will need to become more efficient at converting fuel into usable energy. Advances in materials science, such as the development of high-temperature alloys and ceramic coatings, will enable turbo generators to withstand higher operating temperatures and improve efficiency. Moreover, heat recovery technologies will play a crucial role in improving overall shipboard efficiency. By capturing waste heat from the exhaust gases of turbo generators and converting it into useful energy, these technologies can reduce the need for additional fuel consumption, lowering both operational costs and emissions.
The integration of smart technologies into turbo generators will also enhance their performance in the future. Digitalization and predictive analytics are transforming the way shipboard systems are managed, and turbo generators will be no exception. By incorporating advanced sensors and control systems, turbo generators can be monitored and adjusted in real-time to optimize their performance based on current conditions. For example, digital controls could adjust the power output of the turbo generator depending on the vessel’s speed, load, and energy needs. Predictive maintenance technologies, powered by machine learning algorithms, could forecast potential failures before they occur, reducing downtime and maintenance costs. These technologies will help ship operators maximize the efficiency of their turbo generators, improving both their economic viability and their environmental performance.
Hybrid power systems that combine turbo generators with batteries or fuel cells will become more common as shipping companies seek to reduce their reliance on conventional fuels. By storing excess energy generated by turbo generators in batteries or supercapacitors, vessels can reduce fuel consumption during periods of low energy demand. Alternatively, fuel cells, which generate electricity from hydrogen or other fuels through an electrochemical process, could be integrated with turbo generators to provide cleaner power. These hybrid systems can operate more efficiently than traditional power generation systems, reducing emissions and improving fuel efficiency. In some cases, fuel cells may even replace turbo generators entirely, particularly in vessels that operate on short routes or in emission-controlled areas where zero-emissions technologies are required.
The continued development of autonomous ships will also have an impact on the future role of turbo generators. As autonomous vessels become more common, turbo generators will need to be integrated into automated systems that manage the ship’s power needs. Autonomous ships are likely to be powered by highly efficient, low-emission systems, and turbo generators will need to be optimized for remote monitoring and control. For example, predictive analytics could be used to adjust the performance of the turbo generator based on factors such as fuel efficiency, environmental conditions, and operational goals. Autonomous vessels will also require highly efficient power systems that can ensure reliable operation without the need for constant human intervention.
The focus on sustainability in the maritime industry is driving the adoption of green shipping technologies, and turbo generators are at the heart of this transformation. To meet the growing demand for cleaner, more efficient vessels, turbo generators must be designed to be more adaptable, fuel-efficient, and environmentally friendly. As the shipping industry works toward achieving net-zero emissions by mid-century, turbo generators will play a key role in reducing the carbon footprint of maritime transport.
The future of turbo generators is also shaped by the increasing importance of international collaboration. Governments, regulatory bodies, shipping companies, fuel suppliers, and technology developers must work together to develop and implement the technologies that will help meet the maritime industry’s sustainability goals. This collaboration will foster innovation and lead to the development of turbo generators that are not only more efficient but also capable of operating in an increasingly complex and dynamic global shipping environment.
In conclusion, the future of turbo generators is closely tied to the maritime industry’s ongoing efforts to achieve greater sustainability and efficiency. With continued innovations in fuel technology, energy management, digitalization, and hybrid systems, turbo generators will evolve to meet the challenges of a rapidly changing world. As the shipping industry moves toward greener, more sustainable practices, turbo generators will play an essential role in ensuring that vessels can operate efficiently, reduce their environmental impact, and comply with stringent regulatory standards. The development of turbo generators capable of integrating with renewable energy sources, cleaner fuels, and smart technologies will be crucial in helping the maritime sector achieve its sustainability goals in the years to come.
Steam Boat
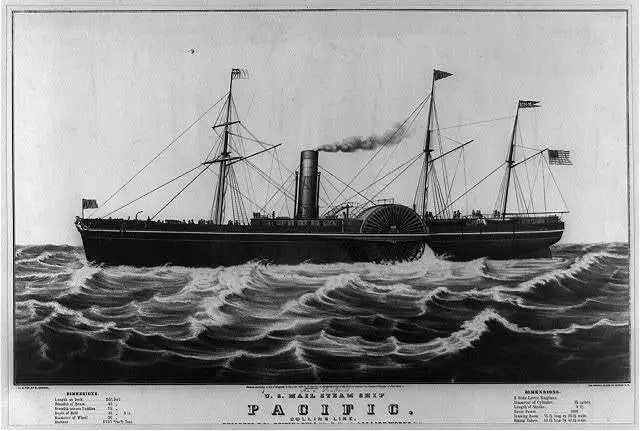
The steam boat, a revolutionary invention in maritime history, played a pivotal role in transforming global trade, transportation, and travel during the 19th and early 20th centuries. The steam boat, powered by steam engines, provided a significant leap forward from earlier, sail-powered vessels by offering greater reliability, control, and efficiency in propulsion. The development and widespread use of steam boats fundamentally changed how goods and people were transported across rivers, seas, and oceans, making them an indispensable part of the global maritime network.
The concept of steam-powered boats can be traced back to the early 18th century, with various inventors attempting to harness the power of steam for propulsion. However, it wasn’t until the early 19th century that the technology matured and became practical for widespread use. The breakthrough moment came in 1807 when Robert Fulton, an American engineer and inventor, successfully launched the Clermont, a steam-powered vessel that traveled the Hudson River in New York. Fulton’s steam boat demonstrated the viability of steam power for commercial transportation, and it marked the beginning of the steam boat era. Fulton’s success paved the way for the construction of steam boats in other parts of the world, leading to the rapid expansion of steam-powered vessels in the years that followed.
The steam engine, which was the heart of the steam boat, had been developed over several decades. James Watt, a Scottish inventor, made key improvements to the steam engine in the late 18th century, making it more efficient and practical for a variety of applications, including steam boat propulsion. By using steam to drive a piston, the steam engine was able to convert heat energy into mechanical energy, which could be used to turn a wheel or drive a propeller. These engines provided a steady and reliable source of power, allowing steam boats to navigate upstream on rivers, a feat that was previously impossible with sail-powered vessels. The ability to move against the current gave steam boats a significant advantage over traditional sailing ships, which were subject to the whims of the wind.
Steam boats quickly became a preferred mode of transportation for a variety of purposes. In the early days, steam boats were primarily used for river and canal transport, but their reach soon expanded to the high seas. They revolutionized the shipping industry by reducing travel time and increasing the frequency of voyages. Prior to steam boats, ocean voyages could take months, depending on the wind, and were often uncertain. The introduction of steam boats made it possible to travel more consistently, with ships running on predictable schedules and significantly faster speeds.
One of the most significant contributions of steam boats was in the field of trade. The use of steam-powered vessels drastically reduced the cost of shipping goods across bodies of water. Steam boats could move cargo more quickly and reliably than their sail-powered counterparts, which were often delayed by adverse winds. This made global trade more efficient, allowing goods to be transported over long distances at a lower cost and with greater speed. Steam boats played a critical role in the expansion of colonial empires, enabling them to transport raw materials and goods between distant territories and Europe, often with far more efficiency than previously possible.
Steam boats also revolutionized passenger travel. The ability to travel across oceans and rivers in steam-powered vessels allowed for the growth of tourism and passenger services. By the mid-19th century, steam boats were offering regular services between cities, with passengers able to travel in comfort and on a timetable. These boats became an important part of the growing network of transportation that connected people and places, facilitating both domestic and international travel. The increase in the frequency of passenger steam boat services led to the development of new trade routes, with steam boats becoming the most popular means of transport for passengers traveling across large bodies of water.
As the steam boat gained popularity, there were innovations in its design and construction. Initially, steam boats were large, cumbersome vessels powered by external combustion engines. Over time, these engines became more efficient, and the boats themselves became sleeker and faster. The development of iron-hull steam boats, as opposed to wooden-hull boats, provided greater durability and allowed for larger vessels. Steam boats were equipped with side paddles, stern paddles, and later propellers, each providing different advantages depending on the intended use of the vessel. Side-wheel steam boats were commonly used for river and coastal travel, while stern-wheel steam boats were preferred for navigating shallow or narrow rivers. Propeller-driven steam boats eventually became the most common design for ocean-going vessels, offering greater speed and maneuverability.
The rise of the steam boat also had significant economic and social impacts. In the United States, the development of steam-powered vessels led to the growth of river-based industries, such as cotton, tobacco, and lumber. The steam boat made it possible to transport these products to market much more quickly than before. In turn, the increased availability of these goods helped to fuel the growth of new industries and the expansion of cities along the riverbanks. Additionally, steam boats helped to expand the railroad network, as goods and passengers could be transported by steam-powered boats to railheads, where they would continue their journey by train. This interconnected transportation network played a crucial role in the industrialization of many countries, particularly in North America and Europe.
However, the widespread adoption of steam boats was not without its challenges. The technology was still in its infancy, and accidents involving steam boats were not uncommon. Early steam engines were prone to explosions, which could be catastrophic due to the high pressure at which the boilers operated. These incidents led to strict regulations and improvements in steam engine safety, including the development of better pressure-relief valves and more robust boiler construction. As steam engines became more reliable, these accidents became less frequent, but they remained a concern throughout the early history of steam boating.
The decline of the steam boat industry began in the early 20th century as new technologies emerged. The advent of the diesel engine and internal combustion engines offered greater fuel efficiency, speed, and reliability. Diesel-powered ships, which did not require the bulky and labor-intensive boilers of steam engines, quickly supplanted steam boats in most commercial and military applications. Steam boats continued to be used for certain purposes, particularly in regions with limited access to diesel fuel or for tourist cruises, but their dominance in the shipping industry diminished over time.
Despite their decline, steam boats have left a lasting legacy in maritime history. Many aspects of modern ship design, including hull construction, navigation systems, and engine efficiency, owe much to the innovations brought about by steam boat technology. Moreover, steam boats remain an important part of the cultural heritage of many countries, especially in places where they played a central role in transportation and trade. Today, preserved steam boats serve as museum pieces, and some are still used for tourism, offering passengers a nostalgic glimpse into the past and a reminder of the transformative impact of steam power on the world’s waterways.
In conclusion, steam boats were a groundbreaking innovation that reshaped the maritime world. They provided a more reliable, efficient, and cost-effective means of transportation that helped to facilitate the growth of global trade and passenger travel. The steam boat era, while short-lived in terms of technological development, laid the foundation for modern shipping and transportation systems. The legacy of steam boats continues to be felt today, as their influence on ship design, propulsion technology, and maritime history endures.
As steam boats became a hallmark of the 19th century, their influence extended beyond transportation and commerce to shape various aspects of society, culture, and even the environment. The rise of steam-powered vessels marked the beginning of a new age of mobility, connecting previously isolated communities and fostering the exchange of ideas, goods, and people. Steam boats contributed significantly to the globalization of trade, helping to establish maritime routes that linked continents and facilitated the movement of raw materials, manufactured goods, and people. This shift in transportation helped stimulate economic growth, particularly in regions that were previously inaccessible or dependent on slower, less efficient methods of transport.
In Europe, steam boats played a key role in the development of river and canal systems, linking inland cities to major ports and opening up new areas for agricultural and industrial development. The British Empire, with its vast network of colonies, utilized steam boats extensively for both military and civilian purposes, ensuring the rapid transportation of troops, supplies, and mail across its territories. The strategic importance of steam-powered vessels during this era cannot be overstated, as steam boats played an essential role in naval warfare, particularly during the Crimean War and the American Civil War, where they were used for patrolling rivers and coastal regions.
Steam boats also revolutionized tourism during the 19th century. Before the advent of steam-powered travel, long-distance travel was a slow, expensive, and often perilous undertaking. The introduction of steam boats made it possible for individuals to travel longer distances with greater comfort and safety. Popular tourist destinations, particularly along the banks of major rivers, experienced a surge in visitors due to the improved accessibility provided by steam boat travel. The Grand Tour, a cultural journey undertaken by wealthy Europeans, often included steam boat journeys along scenic rivers such as the Danube, the Rhine, and the Mississippi. Steam boats became iconic symbols of leisure and luxury, offering travelers a chance to experience both the romance of the open water and the convenience of modern technology.
At the same time, steam boats began to serve an important role in human migration. They facilitated the movement of people from one region to another, particularly during periods of mass emigration, such as the Irish Famine in the mid-19th century or the California Gold Rush. Steam-powered vessels carried immigrants across the Atlantic to the Americas, where they sought better opportunities and a new life. The development of faster, more efficient steam boats helped reduce the time it took to travel across the ocean, which in turn made the idea of emigration more feasible for many. These journeys, while often long and difficult, played a central role in shaping the demographic and social fabric of countries like the United States, Canada, and Australia.
The environmental impact of steam boats also warrants consideration, as their widespread use contributed to the increased demand for coal during the Industrial Revolution. Steam boats required vast quantities of coal to fuel their engines, and this led to the expansion of coal mining industries in places like Wales, the Midlands, and Appalachia. While steam boats were more efficient than previous forms of transportation, they were not free from environmental concerns. The reliance on coal as a fuel source contributed to air pollution, particularly in port cities and industrial regions, where large concentrations of steam-powered ships were stationed. This early industrialization of transportation, while transformative, laid the groundwork for the environmental challenges that would arise in the 20th century as fossil fuels became the dominant energy source.
As technology progressed, steam boats gradually gave way to more modern forms of maritime transport. The advent of the diesel engine in the early 20th century, with its superior fuel efficiency and lower maintenance costs, eventually displaced steam engines as the preferred propulsion system for ships. The efficiency of diesel engines, coupled with their ability to operate on a variety of fuels, made them the ideal choice for most commercial and military vessels. However, despite the decline of steam boats in commercial shipping, they continued to be used in certain specialized areas, such as river transport and tourist cruises. Some of the most iconic steam-powered riverboats, such as the famous Mississippi River steamers, are still preserved today and operate as floating museums, offering passengers a nostalgic glimpse of the past.
The steam boat era also had a lasting impact on the engineering and shipbuilding industries. The development of steam-powered vessels required significant advancements in the design and construction of ships. Early steam boats were often based on existing sailboat designs, but over time, the increasing size and power of steam engines led to the development of purpose-built steam ships with more robust hulls and more advanced propulsion systems. Innovations such as the development of steel-hull ships and the use of compound steam engines helped improve the efficiency, speed, and durability of steam boats. These engineering advances laid the foundation for the modern ships that followed, including the development of large, ocean-going liners and cargo vessels.
In the realm of naval warfare, steam boats also played a transformative role. The introduction of steam power to warships fundamentally changed naval tactics and strategies. Before the advent of steam-powered ships, naval battles relied on the use of wind-powered vessels and oars, which made them slow and dependent on favorable wind conditions. Steam-powered warships, on the other hand, could maneuver independently of the wind, providing them with greater flexibility and control in battle. The steam-powered ironclads that emerged during the American Civil War and the Crimean War were among the first examples of steam boats used in combat, and they marked the beginning of a new era in naval warfare. The ability to steam into battle, regardless of wind conditions, and the increased firepower offered by steam-powered warships, marked a dramatic shift in the strategic importance of naval forces.
Despite the advancements in technology that led to the decline of steam boats, their legacy remains an integral part of maritime history. The development of steam-powered vessels not only revolutionized transportation but also set the stage for the technological innovations that would define the 20th century. From the early steam engines to the massive ships of the modern era, the evolution of the steam boat is a testament to human ingenuity and the relentless pursuit of progress in the face of new challenges.
Today, while steam boats are no longer the dominant form of maritime transportation, their influence can still be seen in modern shipping and transportation technologies. The principles behind steam-powered propulsion systems, as well as the innovations in ship design and engineering that arose during the steam boat era, continue to shape the development of contemporary vessels. Moreover, steam boats remain a symbol of adventure, exploration, and progress, evoking a sense of nostalgia for a time when the world seemed vast and full of untapped potential.
In conclusion, the steam boat era was a defining moment in the history of maritime transportation, shaping the course of global trade, travel, and technological innovation. The rise of steam boats revolutionized the way people and goods were transported, providing greater speed, reliability, and accessibility than ever before. While steam boats eventually gave way to more efficient and powerful technologies, their legacy endures, influencing the development of modern ships and continuing to capture the imagination of those who look back on the golden age of steam-powered travel.
As the steam boat era gradually gave way to the innovations of the 20th century, the lessons learned from steam power continued to influence not only the development of new propulsion technologies but also the broader understanding of energy use, engineering practices, and global connectivity. While steam boats may no longer dominate the seas, they left an indelible mark on the way humans think about transportation, commerce, and technological progress.
One of the lasting effects of steam boats is the way they influenced the development of large-scale infrastructure. The construction of docks, canals, and port facilities to accommodate steam-powered vessels was a monumental task that required significant investment and innovation. These developments became critical in expanding international trade and commerce by providing the physical and logistical foundations for the global shipping network. Cities that were once isolated from the broader world now became hubs for the import and export of goods, and steam boats made it possible for goods to travel more efficiently across vast distances.
The introduction of steam boats also helped pave the way for later innovations in engine technology. The steam engine, which had powered everything from early locomotives to industrial machinery, provided the foundation for later advances in internal combustion engines and gas turbines that would come to dominate not only maritime transportation but also the automotive and aviation industries. Steam boats were one of the earliest and most visible applications of steam engines in practical, everyday life, helping to demonstrate the potential of this form of power. The rise of steam boats was a precursor to the industrial revolution’s broader reliance on steam-powered technologies that spanned multiple sectors of the economy.
Another significant aspect of the steam boat era was its impact on human migration. Steam boats were a critical part of the global movement of people, facilitating emigration from Europe, Africa, and Asia to the Americas and other regions during times of economic hardship, war, or colonial expansion. Steam-powered ships carried millions of passengers across the oceans, contributing to the creation of new societies and cultures. This movement of people not only reshaped demographics but also helped forge new national identities in places like the United States, Canada, and Australia. The ability to travel across vast distances in a matter of weeks, rather than months, changed the way people viewed their world and their opportunities, making the dream of a better life attainable for many.
While steam boats were instrumental in economic growth, they also had a profound impact on the cultural and social landscape of the time. They became symbols of progress, representing the cutting-edge technology of their day. Writers, artists, and thinkers often used steam boats as metaphors for industrial progress, speed, and human achievement. In literature, steam boats featured prominently as symbols of adventure and discovery. Mark Twain’s famous novel, The Adventures of Huckleberry Finn, for instance, is set along the Mississippi River, where steam boats were a central part of life. The image of the steam boat became synonymous with the spirit of exploration and the possibility of overcoming the barriers of nature.
Furthermore, steam boats played a significant role in shaping modern naval strategy. While steam-powered warships were already used in combat during the Crimean War and American Civil War, the later evolution of steam boats into more specialized military vessels furthered the development of naval power. The ironclad warships and battleships of the late 19th and early 20th centuries were direct descendants of the steam boats used in earlier conflicts. These vessels were far more powerful, faster, and capable than their wind-powered predecessors, and they helped shape naval warfare strategies throughout the world. The shift from sail to steam power also influenced the tactics used during wars, allowing for more dynamic and coordinated naval operations.
Even as steam boats faded from the commercial scene, their influence persisted in the maritime and engineering sectors. The marine engineering principles that were developed to design and build steam boats continued to evolve and influence shipbuilding techniques in the subsequent decades. Steam-powered vessels, for all their drawbacks, had been remarkably adaptable. Their ability to navigate in any direction regardless of wind, their ability to carry large amounts of cargo and passengers, and their reliability on inland waterways all paved the way for further advancements in ship propulsion and navigation systems.
The tourist industry also owes much to the legacy of steam boats. The concept of leisure travel by water became popular during the steam boat era, and today, cruise ships, which are descendants of steam-powered vessels, have become one of the most popular forms of travel. The luxury, comfort, and convenience of modern cruise ships hark back to the era of steam-powered liners, and many contemporary cruise ships still use steam turbines as part of their propulsion systems. Steam boats opened the door to the possibility of combining transportation with recreation, a concept that remains integral to the travel industry.
The environmental impacts of the steam boat era, though largely positive in terms of expanding the global economy, were also significant. The heavy reliance on coal as a fuel source led to significant air pollution and ecological changes in the regions where steam boats operated. Port cities, especially those in industrial areas, often suffered from poor air quality, as the emissions from steam engines contributed to smog and other forms of pollution. The widespread use of coal for steam generation had long-lasting environmental consequences, particularly during the industrial revolution when steam engines became ubiquitous across transportation networks.
In terms of legacy, the steam boat era had an undeniable impact on shaping the future of global trade, cultural exchange, technology, and transportation systems. It laid the foundation for the interconnected world we live in today, where the movement of goods and people across continents is a matter of routine. While the steam boat itself is no longer a primary mode of transportation, the innovations it introduced in terms of engine design, propulsion, and efficiency continue to inform modern engineering practices. Steam boats were a testament to the transformative power of technology, and their impact continues to resonate in fields ranging from shipbuilding to energy production.
In conclusion, the steam boat was more than just a technological advancement; it was a catalyst for broader societal and industrial changes that reshaped the world in profound ways. From its early days of innovation to its eventual decline in favor of more modern technologies, the steam boat was a symbol of progress, an embodiment of human ingenuity, and a driving force behind the global interconnectedness that we now take for granted. Even as steam boats are now relegated to history, their legacy endures, serving as a reminder of the transformative power of technology in shaping human civilization.
Here’s a breakdown of the long text into five main sections:
- Introduction to Steam Boats and Their Impact
This section discusses the inception of the steam boat, beginning with early experiments in steam power and culminating in the launch of Robert Fulton’s Clermont in 1807. It emphasizes how steam boats revolutionized maritime transport by offering faster, more reliable travel and how they reshaped global trade, commerce, and human migration. - Technological Evolution and Advancements
This section delves into the development and refinement of steam-powered vessels. It covers the key technological advances in steam engine design, including the contributions of James Watt and other engineers, the progression from external combustion engines to more efficient internal systems, and the innovations in ship construction, such as iron-hulled steam boats and various propulsion methods like paddles and propellers. - Societal and Economic Impacts
The widespread adoption of steam boats significantly affected both local and global economies. This section highlights how steam boats facilitated the growth of global trade, making the movement of goods faster and more reliable, and how they opened new markets. It also touches on the role of steam boats in fostering tourism, passenger travel, and migration, as well as their contribution to the industrial and economic expansion of key regions. - Environmental and Safety Considerations
This section addresses the environmental impact of steam boat technology, focusing on the reliance on coal, the pollution it caused, and its effects on the industrial landscape. It also touches on the safety challenges associated with early steam engines, such as boiler explosions, and how these challenges were addressed over time with improved safety protocols and engineering innovations. - Decline of Steam Boats and Lasting Legacy
This section discusses the eventual decline of steam boats as diesel engines and other more efficient technologies emerged. It explores how steam boats, while no longer dominant in commercial shipping, left a lasting impact on maritime engineering, naval warfare, and global trade systems. Additionally, it reflects on the cultural legacy of steam boats, particularly in literature, tourism, and modern cruise ships, which carry forward the principles of steam-powered travel.
Introduction to Steam Boats and Their Impact
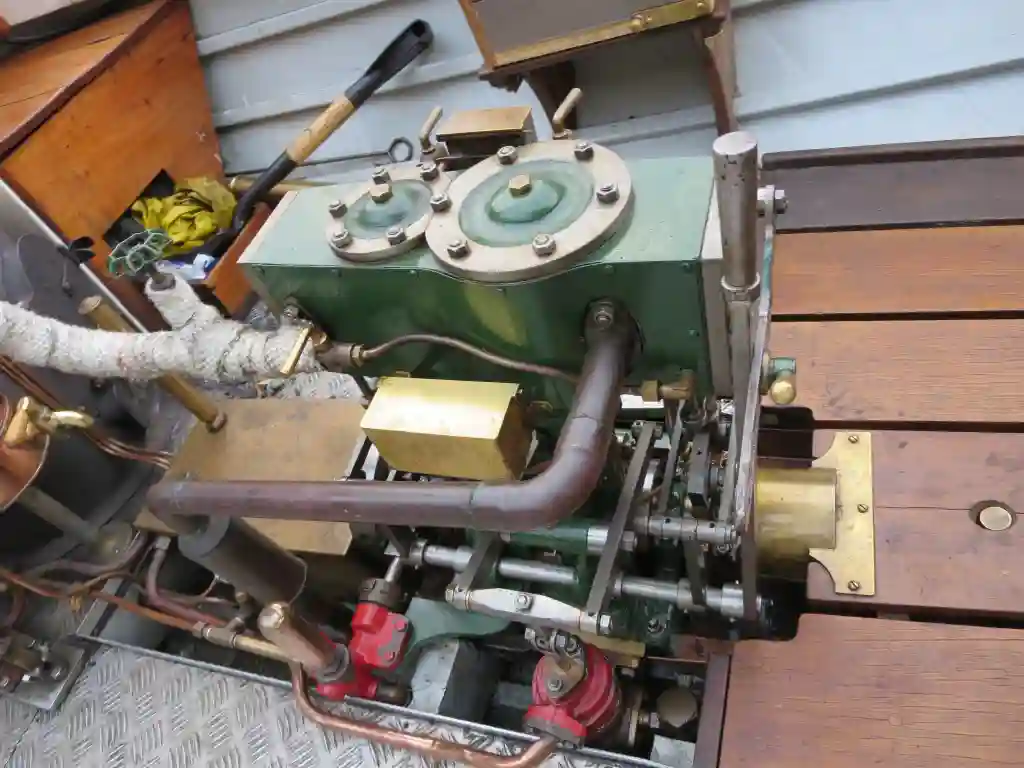
The steam boat, a revolutionary form of maritime transportation, emerged as one of the most significant inventions of the early industrial era, dramatically transforming the way people and goods moved across water. Before the advent of steam power, travel by water was heavily dependent on wind or manpower, which made it slow, unreliable, and limited in scope. Early seafaring vessels relied on sails or oars, and navigating vast rivers, oceans, or coasts was often a matter of luck, waiting for favorable weather or tides. The steam engine, powered by burning coal, offered an alternative that would eventually reshape global commerce, passenger travel, and even military operations.
The development of the steam boat can be traced back to the work of several inventors, but it was the efforts of figures like Robert Fulton and James Watt that truly paved the way for the steam boat’s widespread use. In 1807, Fulton’s successful voyage of the Clermont, a steam-powered vessel on the Hudson River, marked a defining moment in maritime history. While earlier steam-powered boats had been attempted, Fulton’s boat was the first to demonstrate that steam engines could be used effectively for consistent, reliable transportation. Unlike its predecessors, which often struggled with performance and reliability, the Clermont was capable of traveling against the current, providing a more efficient and predictable mode of transport compared to wind-powered vessels.
Fulton’s success quickly spurred further interest in steam-powered vessels, and soon steam boats began appearing on major waterways around the world. These vessels became a backbone of industrial and commercial expansion, enabling the rapid transportation of goods and people. In the United States, steam boats became essential for navigating the vast river systems like the Mississippi and Ohio Rivers, vastly improving the efficiency of trade and opening new markets in previously remote areas. Similarly, in Europe, steam boats played a critical role in enhancing the connectivity between inland cities and coastal ports, facilitating trade and commerce across major rivers like the Rhine, Danube, and Thames.
The impact of steam boats went beyond just transportation; they fundamentally altered the way society interacted with its environment and resources. For the first time, the ability to navigate waterways was independent of the unpredictable forces of wind, tides, and weather. This independence allowed steam boats to establish regular schedules, something that was never before possible with sailing ships. The predictable schedules of steam boats made travel more accessible, creating the foundation for what would eventually become the global tourism industry. Long-distance travel by water, which once took months and was fraught with danger, became faster, safer, and more affordable, opening up previously unreachable destinations for the average traveler.
The widespread adoption of steam boats also had a profound impact on global trade. Before steam power, maritime trade was limited by the slow speeds of sailing ships, which often required months to cross oceans or travel between distant ports. Steam boats, on the other hand, dramatically reduced travel time, allowing for faster shipments of goods and facilitating the growth of international commerce. This had a particularly transformative effect on the economies of nations that relied on maritime trade, such as Britain, the United States, and various colonial powers. Steam boats helped establish efficient, reliable trade routes and supported the flow of goods like cotton, grain, coal, and manufactured products across the globe. In addition, they opened up new avenues for transporting passengers, especially immigrants, who sought new lives in distant lands.
The steam boat’s influence on human migration was significant. As steam-powered ships made transatlantic crossings faster and more affordable, large waves of immigration to the United States, Canada, Australia, and other parts of the world took place. Many people from Europe, particularly during times of economic hardship or famine, took advantage of the faster and more reliable steam boats to make the long journey to the Americas. The ability to travel by steam boat also increased the volume of trade in people, as it opened up new opportunities for settlement and labor in newly developing industrialized nations. The steam boat thus played a role in shaping the demographic and social landscapes of many countries, leaving a lasting imprint on the cultural fabric of regions that received immigrants.
In terms of military applications, steam boats revolutionized naval warfare. Before steam, naval fleets were at the mercy of wind patterns, and battles were dictated by the ability to position ships in the most advantageous way given the weather. The introduction of steam-powered warships changed this dynamic entirely. With the ability to move regardless of wind conditions, steam boats made it easier to control the positioning of ships, leading to more strategic engagements. Steam-powered vessels also had the advantage of speed, which allowed them to outmaneuver traditional sail-powered ships, making them essential during times of war. The significance of steam boats in naval warfare became apparent during the American Civil War, where steam-powered ironclads played pivotal roles in river battles, signaling a new era in military naval technology.
The introduction of steam boats also prompted a shift in engineering and technological thinking. Engineers and inventors had to develop increasingly sophisticated steam engines to meet the demands of commercial and military use. The steam engine, initially a crude and inefficient machine, evolved into a more powerful and reliable mechanism capable of powering ships of ever-larger sizes. These advancements had far-reaching implications beyond the maritime world, influencing the development of railways, factories, and other industries that relied on steam power. The principles of steam power that emerged from the steam boat era continued to be integral in driving the progress of the Industrial Revolution.
In conclusion, the impact of steam boats on society, commerce, and technology was monumental. They transformed global transportation, enabling faster and more reliable movement of goods and people. The steam boat revolutionized naval warfare, industry, and trade, while also spurring economic and social changes that reshaped the modern world. Their widespread adoption helped propel the Industrial Revolution forward, paving the way for later technological innovations in energy generation, transportation, and global commerce. Today, while steam boats may no longer be the primary mode of maritime transport, their legacy endures as a symbol of technological progress and the transformative power of human ingenuity.
The steam boat era not only changed the physical landscape of global transport and trade but also had profound implications on the cultural and social aspects of the time. It became an emblem of progress, showcasing the power of human innovation and engineering. As steam boats became more widespread, they symbolized the transition from the limitations of nature to human control over energy sources, offering a new sense of possibility and freedom.
In literature, steam boats were often depicted as vehicles of adventure, exploration, and freedom. Mark Twain’s famous works, particularly The Adventures of Huckleberry Finn, are set along the Mississippi River, a vital route for steam boats. The river, powered by steam, was seen as both a source of opportunity and danger, echoing the broader themes of personal and societal transformation. The image of the steam boat became inseparable from the concept of new frontiers, not just in terms of geography, but also in terms of industrial and social progress.
Similarly, in art and visual culture, steam boats were celebrated as symbols of human achievement. Artists and engravers of the period often depicted steam boats in their works, emphasizing their sleek lines and the smoke that billowed from their funnels—powerful symbols of industry and transformation. These images helped reinforce the idea that technological advancements could be seen as beautiful and worthy of admiration, contributing to the growing public interest in technology and its role in shaping the modern world.
Steam boats also had a significant impact on the tourism industry. With the ability to navigate rivers and coasts more efficiently, they opened up new opportunities for leisure travel. Passenger steam boats became popular for river cruises, offering a novel way for people to experience nature and distant regions. By making travel faster, more comfortable, and more predictable, steam boats also made it more affordable for a wider range of people to travel. What was once an arduous and expensive journey became accessible to the middle class, which sparked the growth of vacation travel and the tourism industry.
At the same time, steam boats had a major influence on human migration. Before their introduction, long-distance travel by sea or river was an ordeal that could take months. Steam-powered vessels dramatically reduced travel times, making it easier for people to move from one continent to another. The steam boat era coincided with waves of immigration, especially to the Americas, where large numbers of Europeans sought better opportunities. This migration helped shape the modern demographics of many countries, and the ease of travel made it possible for families to reunite across vast distances.
Moreover, the development of steam-powered transportation also had significant economic implications for industry. By enabling the efficient movement of goods, steam boats facilitated the expansion of global markets. Manufactured goods from Europe could now be shipped faster and more reliably to the Americas, Africa, and Asia. Likewise, raw materials like cotton, tobacco, and minerals were able to flow more smoothly from colonies to industrial centers. This exchange of goods helped fuel the Industrial Revolution, ensuring that countries with steam-powered vessels had an economic advantage over others without such technology.
The industrial impact of steam boats was not just confined to transport. The need for reliable steam engines led to improvements in engineering, which spread to other sectors. Factories began adopting steam engines to power machinery, allowing mass production of goods at unprecedented rates. The development of steam engines for boats and ships laid the groundwork for further innovations in railways and land-based transportation, both of which relied heavily on steam power to move people and freight.
The widespread adoption of steam boats also meant that new forms of infrastructure were needed. Ports, docks, and shipping lanes had to be built or expanded to accommodate these larger, faster vessels. This led to the development of coastal cities and inland shipping routes, many of which became major economic centers. The construction of these infrastructure projects further spurred economic growth, with industrial cities benefiting from the creation of jobs and the influx of goods and people brought about by steam-powered shipping.
As the steam boat era continued to expand, it also began to influence military applications. The ability to move ships rapidly and independently of wind conditions was a significant advantage in warfare. Steam-powered warships, especially ironclads, revolutionized naval combat. They were faster, more maneuverable, and less vulnerable than traditional sailing ships, making them invaluable during conflicts like the American Civil War and the Crimean War. The success of steam-powered warships in these conflicts helped to further solidify the steam engine’s role in military technology, leading to the development of modern naval fleets.
As steam boats became more common, they also became integral to the cultural identity of many nations. For example, in the United States, the steam-powered riverboats that plied the Mississippi River became iconic, associated with the notion of the American frontier and the country’s ongoing expansion into new territories. These boats became cultural symbols of America’s progress and industrial prowess, woven into the national mythos.
In summary, the steam boat era had far-reaching implications, not only transforming global transportation and trade but also influencing cultural, social, and economic landscapes across the world. The steam boat was not just a technological marvel—it was a driving force that reshaped how people moved, communicated, and interacted with the world around them. While steam boats eventually gave way to more advanced technologies, their legacy remains deeply ingrained in the fabric of modern society, serving as a reminder of how a single innovation can alter the course of history. The steam boat era, though a chapter in the past, continues to resonate through the technologies and infrastructures that arose from it.
As the steam boat era faded into history, it left behind not just the remnants of its physical infrastructure but also the foundations for modern transportation systems. The advancements in steam engine technology and shipbuilding that were spurred by the demand for steam boats directly influenced the development of railroads and automobiles. The knowledge gained from building and maintaining steam engines, along with the experience in mass-producing steam-powered vessels, contributed to the innovation of similar technologies in land-based vehicles. Steam engines evolved into more powerful and efficient forms, eventually leading to the internal combustion engine that revolutionized automotive transportation.
Moreover, the environmental implications of steam boats—particularly their reliance on coal as a fuel source—became a critical aspect of the ongoing conversation around energy consumption and pollution. The coal-powered steam engines emitted large amounts of smoke and particulate matter, contributing to air pollution in port cities and industrial areas. While the steam boat was a marvel of human ingenuity, its environmental cost was considerable. As the world moved into the 20th century, alternative fuels such as oil began to replace coal, and the development of diesel engines offered a more efficient and cleaner alternative to steam power. The environmental issues associated with steam boats thus became an early precursor to the global conversation on sustainable energy and cleaner technologies.
The transition from steam-powered vessels to more advanced engines also paved the way for the global shipping industry we know today. Modern container ships, powered by diesel engines or gas turbines, owe much to the early innovations in steam boat design. The basic principles of shipbuilding and navigation established in the steam boat era, such as hull design and propulsion mechanics, continue to inform the design and construction of today’s vessels. The globalization of trade and the movement of goods across oceans that is now a hallmark of the world economy can trace its origins back to the steam boat’s ability to open new maritime trade routes and establish consistent, regular transportation of goods over long distances.
The decline of steam boats did not erase their cultural significance, either. They continued to captivate the imagination, with many people today romanticizing the era of the great steam-powered liners that crossed the Atlantic, such as the RMS Titanic or the Cunard Line’s Queen Mary. These vessels became symbols of luxury, opulence, and the promise of new opportunities across the ocean. Even as steam boats were phased out in favor of more modern vessels, their place in popular culture remained strong, often evoked in literature, film, and art as representations of adventure, discovery, and the grandiosity of early 20th-century travel.
In the realm of tourism, the legacy of the steam boat era continues to thrive. Today, river cruises and historical steamboat tours attract travelers seeking a nostalgic glimpse into the past. These modern steam-powered excursions are often built around vintage steam boats or replicas that allow passengers to experience the same sense of wonder and exploration that steam boat travel once provided. Cruise lines often use the aesthetic and romanticized image of the steam boat to promote their products, capitalizing on the enduring appeal of these iconic vessels.
In the naval and military sectors, steam boats also contributed to the evolution of modern naval warfare. The steam-powered warships that emerged during the 19th century laid the groundwork for the development of more advanced vessels, eventually leading to the construction of aircraft carriers and nuclear submarines. The technological advancements in propulsion, weaponry, and hull design that were first tested in steam-powered ships were continually refined and adapted to meet the demands of modern defense strategies. The steam boat, while long outdated as a combat vessel, remains an important chapter in the ongoing evolution of naval technology.
The influence of steam boats extended beyond the maritime world into the industrial and engineering fields. The principles of steam engine design and mechanics informed the development of early power plants and industrial machinery. The innovations in mechanical engineering, particularly the advancements in steam turbines and internal combustion engines, helped shape the industrial landscape of the 20th century. The evolution of steam technology also set the stage for breakthroughs in aerospace engineering, as the experience in producing efficient, high-power engines and systems was applied to the development of aircraft and rockets.
Finally, the steam boat era was instrumental in shaping the idea of interconnectedness in the modern world. The expansion of steam-powered transportation networks—whether through steam boats, railroads, or industrial systems—created an intricate web of global connectivity that brought people, goods, and ideas closer together. This interconnected world continues to be driven by technological advancements in transportation, communications, and trade. The steam boat was one of the early forces that helped pave the way for the highly interconnected global system we live in today, where distances between countries seem smaller, and the movement of people and goods is constant and efficient.
In retrospect, the legacy of the steam boat era is more than just the development of a new mode of transport; it was the catalyst for a technological and cultural revolution that fundamentally changed the course of history. It was a symbol of progress and human ingenuity, ushering in a new age of industry, exploration, and international cooperation. Although the steam boat itself has largely been replaced by more advanced technologies, its imprint on modern society remains indelible. Whether in the form of advanced maritime technology, the global shipping industry, or the cultural legacy of steam-powered adventure, the steam boat’s influence is still felt today, a testament to the lasting power of innovation and progress.
Technological Evolution and Advancements
The technological evolution of steam boats is a story of constant innovation and refinement, shaped by the quest for greater efficiency, power, and reliability. The steam boat’s development can be traced through various stages, from early rudimentary designs to the advanced vessels that revolutionized global transportation. Over time, these vessels evolved from inefficient, primitive machines into highly sophisticated and powerful ships that could navigate vast waterways and seas, dramatically altering global trade, commerce, and the course of history.
The earliest attempts at steam-powered boats were experimental and crude, primarily designed by engineers and inventors experimenting with the power of steam engines. The very first recorded use of steam power in a boat can be attributed to Denis Papin, a French inventor, in the late 17th century. In 1704, Papin demonstrated a steam-powered boat that could propel itself across water, but the technology was not advanced enough to be practical for widespread use. These early experiments were crucial in proving the concept of using steam as a means of propulsion, although it would take nearly a century for the idea to truly take hold.
The most significant breakthrough came in the early 19th century, with the work of James Watt, a Scottish inventor and mechanical engineer, who improved the efficiency of steam engines. Watt’s innovation was the separate condenser, which prevented the engine from losing power with each cycle, making the steam engine more practical for industrial use. While Watt is more often associated with the development of steam engines for factories and trains, his work laid the foundation for the steam-powered boat. His invention made it possible for engines to run more efficiently, enabling steam boats to travel at faster speeds and carry heavier loads.
The next major development in steam boat technology came with Robert Fulton, whose successful demonstration of the Clermont in 1807 revolutionized the transportation of goods and passengers. Fulton’s innovation was in adapting steam engines, primarily designed for stationary industrial purposes, to the propulsion of a moving vessel. By employing a more efficient steam engine and integrating it with a boat hull designed for sustained travel, Fulton was able to create a steam boat capable of traveling reliably against the current of the Hudson River. His success demonstrated the potential of steam power as a practical means of navigation, making steam boats commercially viable.
In the early years following Fulton’s success, steam boats began to proliferate across major rivers and waterways, with engineers and shipbuilders continuing to experiment with new designs to enhance performance. One of the major areas of innovation was the development of steam engines. Initially, steam engines used a single-cylinder configuration, which limited their power and efficiency. As demand for faster and more powerful boats grew, engineers began experimenting with multi-cylinder steam engines, which allowed for more consistent and powerful operation. By the mid-19th century, steam engines had evolved to incorporate more advanced mechanisms, such as the high-pressure steam engine, which increased the overall power output of steam boats, allowing for faster speeds and longer voyages.
Simultaneously, the design of the steam boat hull itself was refined. Early steam boats used paddle wheels to generate propulsion, with the wheels mounted on either side or at the rear of the vessel. These paddle steamers were an improvement over earlier designs, but they were still inefficient in some cases, particularly when traveling at higher speeds or in rougher waters. The limitations of paddle wheels prompted the development of alternative methods of propulsion, such as the propeller. The screw propeller, first successfully tested by Isambard Kingdom Brunel in the 1840s, marked a significant step forward in steam boat design. The propeller offered better efficiency than paddle wheels, allowing steam boats to travel faster and with more control, especially in choppy waters or open seas. This new method of propulsion quickly became the standard for steam-powered vessels, and its efficiency continued to improve with the advancement of materials, manufacturing techniques, and engine design.
As steam boats became more widely used, they were adapted for various types of water transportation. River steamers served as crucial means of transport for goods and passengers along the inland river systems of the United States and Europe. These vessels were often smaller and built to navigate the shallow waters and winding courses of rivers. On the other hand, ocean-going steamships required more robust designs to cope with the challenges of long-distance sea voyages. The hulls of these steamships had to be strengthened, and the engines had to be more powerful to maintain speed and efficiency over longer distances. The increasing demand for transatlantic travel and trade in the mid-19th century prompted the development of larger, more advanced steamships capable of carrying thousands of tons of cargo and large numbers of passengers. These ships also required advanced navigation systems and more precise control over engine power to ensure smooth and efficient long-distance travel.
The evolution of steam boat technology reached new heights in the late 19th and early 20th centuries with the advent of steam turbines. Steam turbines, first developed by Charles Parsons in the 1880s, represented a major leap forward in steam engine technology. Unlike the reciprocating engines used in earlier steam boats, steam turbines could generate much more power from a smaller, more compact unit. This allowed for faster speeds, greater fuel efficiency, and the ability to handle larger, more complex ships. Steam turbines quickly found their place in both military vessels and luxury liners, where speed and efficiency were paramount.
In parallel with the development of more powerful engines, steam boat design also saw innovations in materials. The shift from wooden hulls to iron and steel was one of the most significant advancements in steam boat technology. Iron and steel provided greater strength and durability, which was essential for large, powerful steam boats. The transition to metal hulls also allowed for larger, more spacious ships, leading to the rise of luxury steamships that could accommodate hundreds or even thousands of passengers. Furthermore, the increased use of metal in ship construction allowed for better waterproofing and the ability to build ships that could withstand the pressures of high-speed travel over long distances.
As steam boats continued to evolve, the integration of newer technologies such as electricity, telegraphy, and radio communications began to play a larger role in their operation. Electric lighting and communication systems were installed aboard ships, improving the safety and comfort of passengers and crew. The advent of the marine radio allowed steam boats to communicate over long distances, providing crucial information about weather conditions, ship locations, and other vital data. These technological advancements further enhanced the steam boat’s role as a key tool for both commercial and military purposes.
Despite the innovations that pushed steam boat technology to greater heights, the rise of new propulsion systems such as diesel engines and nuclear power in the 20th century eventually led to the decline of the steam boat. However, the technological breakthroughs achieved during the steam boat era laid the groundwork for many of the modern advancements in transportation, including air travel, automobile engines, and electrical generation. Even today, the legacy of steam boat technology can be seen in the design of modern ships, the power generation sector, and the fundamental principles of engine efficiency and mechanical engineering.
In conclusion, the technological evolution of steam boats was a continual process of refinement and innovation, driven by the need for greater power, efficiency, and reliability. From the early experiments with steam engines to the development of steam turbines and metal hulls, steam boat technology played a crucial role in shaping the modern world. It enabled the rapid growth of global trade and travel, contributed to the advancement of engineering and mechanical principles, and set the stage for the development of newer, more advanced propulsion systems that continue to influence maritime technology today.
As the steam boat era evolved, the advancements in technology spurred new developments in various sectors, many of which continue to influence modern industries. The transition from steam-powered vessels to more advanced systems, such as diesel engines, gas turbines, and nuclear propulsion, marked a shift in the engineering principles governing waterborne transport, but the legacy of steam technology remains foundational in these newer systems.
One of the most notable contributions from the steam boat era was the development of thermodynamics as a scientific discipline. Steam engines, which operated based on the principles of converting heat into mechanical energy, were some of the first widespread applications of thermodynamic concepts. The study of heat engines and their efficiency became a central focus of engineering and physics in the 19th and 20th centuries. The steam boat and its engines provided real-world experiments and applications that drove the development of Carnot’s Theorem, the first law of thermodynamics, and other essential thermodynamic principles that govern energy conversion in modern machines. These fundamental concepts continue to underpin the design of engines, turbines, and power plants used across various industries, from aviation to energy production.
The era of steam boats also saw the early integration of automation and control systems. As steam engines became larger and more powerful, it became essential to implement systems for safety and regulation to ensure that the engines operated within safe parameters. Pressure gauges, automatic feed systems for coal and water, and governors to control the speed of the engines were developed to maintain smooth operation and prevent accidents. These early automation techniques laid the foundation for the modern systems used in both industrial and transportation sectors, such as the closed-loop control systems seen in today’s advanced manufacturing and energy systems.
As the technology of steam-powered boats continued to improve, the design of steam propulsion systems was adapted for use in military applications, particularly in the context of naval warfare. The steam-powered ironclad warships that emerged during the 19th century represented a monumental shift in naval power. These warships were faster and more maneuverable than their sail-powered counterparts, and they were equipped with powerful weapons that could strike at long range. The development of ironclads during the American Civil War and the Crimean War marked the beginning of the decline of traditional sailing navies and set the stage for the rise of modern naval fleets. The integration of steam power into military vessels also spurred the development of naval strategies that emphasized mobility, speed, and firepower—concepts that remain at the core of modern naval operations today.
The commercial maritime industry also saw lasting impacts from steam boat technology. As steam-powered vessels replaced sailing ships on transoceanic routes, the capacity to carry larger amounts of cargo increased dramatically, enabling the expansion of global trade. The development of cargo liners and passenger ships that could reliably traverse the world’s oceans allowed for the establishment of regular shipping lines, connecting distant countries and regions in ways that were previously unthinkable. The rise of these regular, dependable shipping services laid the groundwork for the global supply chain that is essential to modern economies. Today, container ships, though powered by more advanced propulsion systems, continue to follow many of the same principles that steam ships pioneered in terms of large-scale cargo transport.
The push for efficiency and sustainability in modern maritime transport can also be traced back to the steam boat era. While steam engines were a significant improvement over earlier methods of propulsion, they were still relatively inefficient, especially in terms of fuel consumption. The advent of the steam turbine was an attempt to improve efficiency by harnessing steam’s energy more effectively. Modern marine engines, although powered by a variety of fuels, continue to incorporate principles that were first developed in the steam boat era, such as optimizing fuel use and improving the energy conversion process.
In terms of environmental impact, the legacy of the steam boat era has shaped much of the modern maritime industry’s focus on reducing carbon emissions and fuel consumption. Steam boats were notorious for their reliance on coal, and while they provided significant advancements in transportation and industrialization, they also contributed to air pollution. As the world moved into the 20th and 21st centuries, the need for cleaner and more sustainable energy sources became clear. The development of diesel engines and later liquefied natural gas (LNG) engines in the maritime industry is a direct response to the environmental challenges posed by early steam-powered vessels. Today, there is also growing interest in electrification and alternative fuels, such as hydrogen, to further reduce the maritime industry’s carbon footprint, a direct reflection of lessons learned from the steam boat era.
The technological advancements in steam boats also laid the groundwork for aerospace technologies. Many of the mechanical innovations in steam propulsion—such as the development of efficient, high-pressure engines and turbines—were applied in the development of aviation. Early pioneers of flight, such as Wright brothers, and later the designers of commercial jets, drew from the principles of steam turbine efficiency and engine cooling systems, adapting them for use in aircraft engines. The idea of propulsive power—whether in steam, jet, or rocket form—was shaped by the principles developed for steam-powered ships, with the engines in these vessels serving as testbeds for future innovations in air and space travel.
While the steam boat’s role as the dominant form of transportation waned in the 20th century, its impact on modern technologies and industries cannot be overstated. From its foundational contributions to thermodynamics to its lasting influence on the global shipping industry, the steam boat era represents a critical chapter in the development of modern technology. The engineering principles and innovations born out of the need for more efficient, reliable, and powerful steam-powered vessels provided the catalysts for the development of subsequent technologies in both maritime and industrial fields. Today, the legacy of steam boats lives on in the continuous push toward energy efficiency, sustainability, and technological advancement, which remain at the forefront of engineering innovation across various sectors.
In conclusion, the steam boat era was not just a turning point in the history of transportation; it was the crucible in which many foundational technologies and principles were forged. From steam engines to turbines, from propulsion systems to safety mechanisms, the technological advancements that originated with steam boats have had a lasting impact across industries, shaping the modern world in profound ways. The evolution of steam-powered vessels laid the groundwork for a range of innovations that continue to influence and inspire new technologies, ensuring that the steam boat era will remain a key chapter in the history of human progress and innovation.
Steam Driven Boat
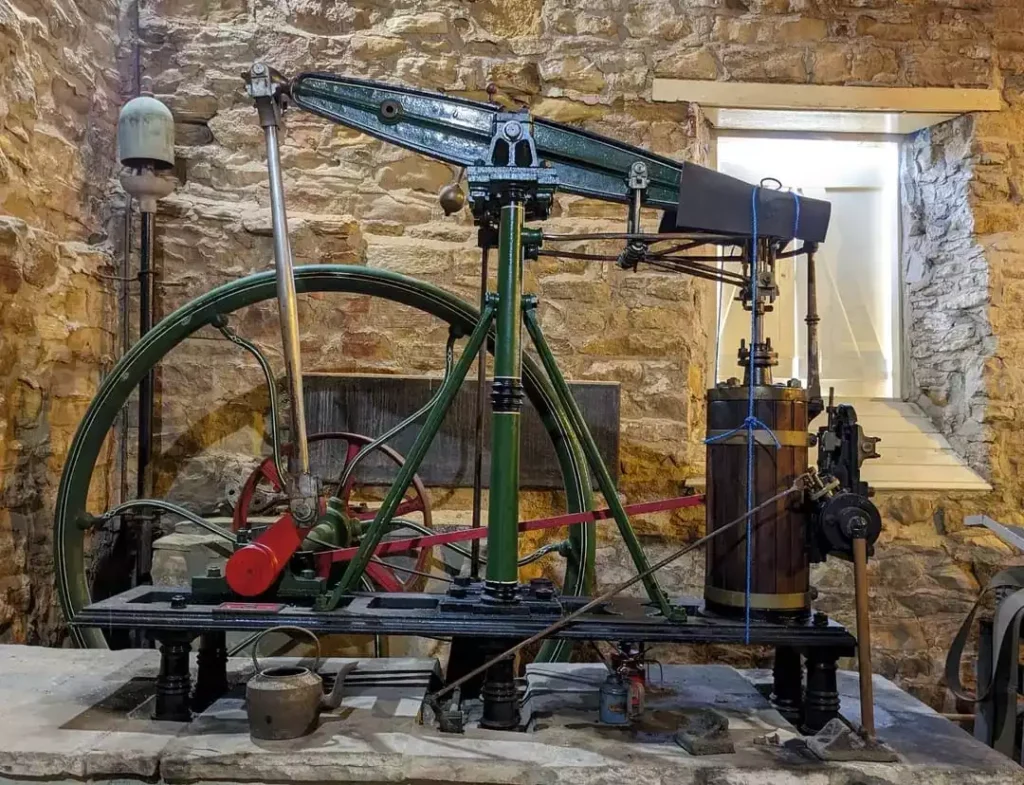
The steam-driven boat, often considered one of the most significant technological innovations in maritime history, marked a turning point in the way people understood and utilized waterborne transportation. It revolutionized not only the maritime industry but also had a profound impact on global trade, the economy, and the movement of people. Before the advent of steam-driven boats, ships relied primarily on wind power and human labor, such as rowing, for propulsion. While sailing vessels had dominated the seas for centuries, their speed and efficiency were limited by wind conditions, and they could not effectively navigate against the current. The introduction of steam power provided a solution to these limitations, bringing about a new era in maritime transport.
The first practical steam-driven boat was developed in the early 19th century, building on the work of earlier inventors and engineers who experimented with steam engines. The breakthrough came with the development of more efficient steam engines capable of powering boats and other vessels. Before this, steam engines were primarily used for stationary applications in industries such as mining and textiles. The challenge was to adapt these engines for use in a moving vessel, where power, durability, and efficiency were crucial.
One of the earliest pioneers in the field was Robert Fulton, an American engineer and inventor. In 1807, Fulton’s successful launch of the Clermont, a steam-powered boat that traveled along the Hudson River, marked a defining moment in the history of maritime transportation. The Clermont demonstrated the practicality of steam power on water and opened up new possibilities for long-distance, reliable transportation. Fulton’s innovation used a steam engine that had been developed by James Watt and others, which allowed the boat to travel upstream at a steady speed—something that was previously impossible with sailboats, which were at the mercy of wind currents. This achievement was significant not just in terms of engineering but also in its potential to transform industries that relied on river and coastal shipping. The success of the Clermont proved that steam power was viable for larger, more substantial vessels, providing the foundation for future steam-powered boats.
Fulton’s achievement was quickly followed by other inventors and engineers who sought to refine the design and increase the efficiency of steam-powered boats. One of the most notable developments during the early 19th century was the paddle wheel—a mechanism that powered many of the first steam boats. Paddle wheels, typically located on either side or at the rear of the boat, used rotating blades to propel the vessel through the water. Although efficient in some conditions, paddle wheels were limited by factors such as speed, the type of water they were traveling through, and their vulnerability to damage in rough seas. These issues prompted further experimentation and improvements in propulsion systems, leading to the eventual development of the screw propeller.
In the mid-19th century, the screw propeller, invented by Isambard Kingdom Brunel, revolutionized steam boat design. The screw propeller was more efficient than the paddle wheel, as it allowed for smoother travel through the water, especially in choppier seas. The propeller provided a much greater thrust relative to the size of the engine, and it could operate at higher speeds with greater control. The development of the screw propeller, along with improvements in steam engine technology, allowed steam-driven boats to operate with greater reliability and for longer distances. This advancement significantly improved the ability of steam boats to cross oceans, and it made them ideal for long-distance trade routes, particularly on the Atlantic and Pacific Oceans.
Steam-driven boats also played a key role in the development of global trade networks. Their ability to navigate both upstream and down, regardless of wind conditions, opened up new avenues for the transport of goods and passengers. Before steam power, rivers and oceans were navigated based on seasonal winds and tides, which made scheduling and planning of shipping routes more uncertain. Steam boats allowed for scheduled, regular trips between ports, providing a level of consistency and reliability that was previously unattainable. This change spurred the growth of port cities and the expansion of trade between nations, especially during the 19th century, when global commerce began to accelerate.
Beyond the economic implications, steam-driven boats also had a profound effect on the social and cultural landscape of the time. As steam-powered vessels allowed for more reliable and faster travel, they contributed to the growth of tourism and the ease of immigration. People could now travel greater distances more quickly, opening up new frontiers in terms of settlement, exploration, and cultural exchange. Steam boats became symbols of progress and industrial might, and they were featured prominently in both literature and art as representations of modernity and the excitement of technological achievement.
The introduction of steam-powered vessels was not without challenges. Early steam boats, while groundbreaking, were still subject to numerous technical difficulties. One of the most persistent challenges was the reliability of steam engines, which were prone to breaking down or exploding under high pressure. These accidents led to the development of more stringent safety protocols and engineering improvements in the design of both the engines and the boats themselves. In addition, steam-driven boats required a steady supply of fuel, typically coal, which had its own logistical challenges. Coal had to be transported to docks and loaded onto vessels, adding extra costs to their operation.
As steam boats became more widespread, they played a crucial role in the military as well. The steam-powered warship quickly emerged as a force to be reckoned with in naval warfare. Steam power provided a level of maneuverability and firepower that had not been possible with sailing ships. The ironclad warship, for instance, was a new type of vessel that combined steam propulsion with iron armor to create a formidable tool of war. Steam-driven boats also became important for patrolling rivers and lakes, particularly in the United States, where river navigation was crucial for internal trade and security.
By the late 19th and early 20th centuries, the development of steam-driven boats had reached a peak in terms of speed and power. These vessels were now capable of crossing vast distances in shorter periods of time, changing the way both people and goods moved across the globe. The arrival of ocean liners, many of which were steam-powered, marked the beginning of the modern cruise industry and passenger travel. These ships were designed for luxury, comfort, and speed, offering travelers an unprecedented experience at sea.
As steam-powered boats became more advanced, they began to be replaced by diesel engines and other internal combustion technologies. Diesel engines were more fuel-efficient, required less maintenance, and allowed ships to operate for longer periods without refueling. However, the legacy of the steam-driven boat remains a cornerstone of maritime history. Many modern ships still owe their design and operational principles to the innovations that first emerged with steam power. Moreover, the steam turbine—a direct descendant of early steam engine technology—continues to be used in a variety of applications, from electric power generation to marine propulsion in large vessels.
In conclusion, the steam-driven boat was a groundbreaking technological advancement that shaped the course of history. It not only transformed the way people traveled and traded but also had far-reaching effects on global economic systems, military power, and societal structures. Its development was driven by innovation and experimentation, which laid the foundation for the more advanced propulsion systems used in today’s vessels. While steam boats have largely been replaced by more efficient technologies, their legacy endures in both the physical and cultural landscapes of the modern world.
The legacy of the steam-driven boat extends beyond its direct contributions to maritime technology. It helped shape the industrial landscape, influencing engineering practices, economic systems, and even cultural perceptions of progress. As the steam boat gained popularity, the demand for railroads, canals, and infrastructure to support these vessels led to a surge in the construction of transport networks. These networks facilitated the rapid movement of goods and people, propelling industrialization in many parts of the world, particularly in the United States, Europe, and parts of Asia. The steamboat industry not only contributed to the transportation sector but also played a vital role in the development of ironworks, coal mining, and other industries that supported steam propulsion.
Additionally, the rise of steam-powered vessels had important cultural implications. The steam boat symbolized modernity, marking the transition from older, slower, and less reliable methods of transportation to faster and more predictable systems. This shift coincided with the broader trend of the Industrial Revolution, which saw profound changes in the way society viewed technology, productivity, and innovation. Steam boats were a visible manifestation of progress and human ingenuity, inspiring a sense of optimism about the potential for technological advancement to solve problems and improve quality of life. This spirit of innovation laid the groundwork for later technological revolutions, as industries began to adapt steam power for other applications, such as factories, railroads, and power plants.
Furthermore, the steam boat’s influence extended beyond the maritime world, with significant contributions to navigation and cartography. The ability of steam-driven boats to navigate rivers and lakes more efficiently led to the mapping of previously unreachable areas, fostering the expansion of exploration in regions like Africa, Asia, and the Americas. Steam-powered vessels were used in scientific expeditions and military missions, often assisting in the opening up of new territories and the establishment of trade routes. The geographical reach of steam boats helped expand the boundaries of the known world and facilitated global exploration during the 19th century.
In the field of military strategy, the steam boat played a crucial role in naval warfare. The introduction of steam power in naval vessels led to the development of faster, more maneuverable ships that could be used in combat. This shift was pivotal during the American Civil War, where the Union and Confederate navies used steam-powered vessels for both offensive and defensive purposes. The introduction of steam-powered warships, such as ironclads, marked the end of the era of sail in naval warfare. Steam-driven boats were able to engage in more precise operations, including blockades, reconnaissance, and offensive strikes, which changed the dynamics of naval engagements for years to come. As naval technology continued to evolve, steam-driven ships laid the groundwork for the advanced military vessels that dominate modern navies today.
The environmental impact of steam-driven boats, particularly in the early days, was significant. The reliance on coal to fuel steam engines produced emissions that contributed to air and water pollution. However, steam boats also played a role in developing the broader understanding of environmental sustainability. Over time, as technology progressed, new methods for reducing emissions and improving fuel efficiency were implemented. Steam engines began to evolve into more sophisticated, cleaner, and more efficient systems, such as the steam turbine, which is still used today in power plants and modern marine vessels. The shift toward more efficient technologies that arose from the era of steam boats helped lay the foundation for modern efforts to reduce the carbon footprint of industrial transportation.
In terms of economic and global trade, steam-powered boats were pivotal in opening new markets and fostering international commerce. The steam boat’s ability to travel faster and with more reliability than previous vessels enabled the expansion of global supply chains and interconnected economies. Major ports around the world began to grow in importance as steam-powered vessels could carry larger amounts of cargo more regularly, creating a more reliable and predictable shipping network. This had a lasting effect on industries, particularly in the movement of raw materials such as coal, cotton, and agricultural products. The ability to ship goods more efficiently and reliably led to lower shipping costs and increased global trade, facilitating the growth of many economies that relied on the sea as a primary means of transportation.
The continued advancements in steam technology, such as the steam turbine, contributed to the eventual evolution of modern power generation systems. The principles that were first applied to steam-driven boats became foundational in the development of large-scale power plants, where steam is used to drive turbines that generate electricity. Today, thermal power stations around the world rely on steam turbines, which are direct descendants of those used in steam ships. These turbines are used to produce electricity, which powers homes, businesses, and industries. This connection between the early steam engines and modern power generation highlights the lasting impact of steam technology on global energy systems.
While steam-driven boats have largely been replaced by diesel and nuclear-powered vessels in commercial and military contexts, the principles they introduced remain relevant. Many modern ships, including cargo ships, luxury liners, and naval vessels, still incorporate steam turbines, particularly in large, high-power applications. The engineering principles behind steam propulsion systems continue to inform the development of more efficient, sustainable, and reliable transportation systems in various industries.
In modern times, eco-friendly and sustainable technologies are a central focus in the maritime industry. While steam boats were once seen as the epitome of technological progress, they have now given way to more environmentally conscious innovations. The current emphasis on reducing emissions, minimizing fuel consumption, and transitioning to cleaner energy sources is a natural evolution of the lessons learned during the steam boat era. Technologies such as LNG-powered ships, battery-electric vessels, and wind-assisted propulsion are increasingly being explored as alternatives to traditional fuel sources. These advancements draw inspiration from the pioneering work of steam engineers and their determination to push the boundaries of what was possible with the technology of their time.
Looking ahead, the maritime sector is exploring ways to integrate hydrogen fuel cells, solar power, and other renewable energy sources into ship propulsion systems. While these innovations are still in the early stages, the foundational work established during the age of steam-driven boats will undoubtedly continue to shape the future of maritime transportation. In this way, the steam boat’s influence on global trade, engineering, and technology will endure for generations to come.
In conclusion, the steam-driven boat stands as a symbol of technological progress, industrial growth, and global interconnectedness. It marked the beginning of an era in which human ingenuity and technological innovation transformed not only maritime transportation but also the broader landscape of global commerce, military strategy, and industrial development. Though its role in direct transportation has diminished, the legacy of the steam-driven boat continues to resonate, providing a foundation for the modern world’s continued evolution toward more efficient, sustainable, and technologically advanced transportation systems.
As we continue to look back on the era of steam-driven boats, it is clear that their impact on modern engineering and design extends far beyond their original scope. The technical innovations that arose during the development of steam-powered boats provided the framework for much of the world’s industrial evolution. The principles of thermodynamics, heat exchange, and mechanical power transmission that were refined in the context of steam propulsion were directly applied to the development of many other machines and systems, from railroads to aircraft and industrial machinery. This process of technological spillover shows the far-reaching influence that steam boats had on the development of modern mechanical engineering and manufacturing practices.
Additionally, steam-driven boats played a crucial role in the social and political dynamics of the 19th and early 20th centuries. Their ability to connect distant parts of the world transformed not only economic networks but also political relationships. Nations began to view each other with greater interconnectedness, and the ability to move troops, resources, and goods quickly changed the nature of both imperialism and international diplomacy. Steam-powered vessels were vital during the expansion of empires, as they facilitated the rapid transportation of goods and military personnel across oceans. Countries with advanced steam fleets gained a strategic advantage in global politics, influencing international relations and territorial disputes.
On a more local scale, the development of steam boats also influenced communities along major rivers, lakes, and coastal areas. Ports that once relied on slower sailing ships experienced economic booms as steam vessels brought in goods faster and more reliably. Cities grew and prospered as steam-driven boats connected them to broader trade networks. For instance, cities like New Orleans, San Francisco, and Liverpool saw tremendous growth due to their role as key points on the steam-powered shipping routes, becoming bustling hubs for international trade. The rise of steam technology also spurred the development of local industries to support this new maritime infrastructure, creating jobs in shipbuilding, fuel production, and port management.
Steam boats also had a profound influence on labor in the maritime sector. The introduction of steam propulsion meant a transition from traditional manual labor, such as sail handling and manual rowing, to more mechanized systems. This shift reduced the dependence on human muscle power and increased the focus on mechanical skills for maintaining and operating steam engines. Steam engineers, firemen, and deckhands became essential to the smooth operation of steam-driven vessels, and new training programs and institutions were created to educate workers in these skills. This transformation in the workforce led to broader societal shifts, with steam-driven boats contributing to the industrialization of labor in the broader context of society.
At the same time, steam-driven boats had an undeniable environmental cost that was evident even during their heyday. The massive demand for coal to fuel steam engines resulted in increased mining activities, deforestation, and pollution. Coal-burning engines emitted large amounts of smoke and particulate matter, contributing to environmental degradation in many areas. While the steam boat played a critical role in transforming global trade and economies, it also introduced challenges in terms of sustainability and environmental impact. This tension between technological progress and environmental responsibility would continue to be a central theme in the evolution of energy sources and transportation technologies.
In the realm of education, steam-driven boats became an important subject for study in engineering schools and universities around the world. As a new and groundbreaking technology, the design and operation of steam-powered vessels prompted widespread interest in steam technology and mechanical engineering. Many of the engineering principles applied in steam boat design were fundamental to the development of later technologies, and the study of steam engines helped shape the education of engineers and designers throughout the 19th and 20th centuries. Steam-driven boats thus played a key role in the evolution of technical education, influencing the training of engineers who would go on to design the machines and technologies that powered the modern world.
In terms of military applications, steam-driven boats dramatically altered the way naval forces were organized and deployed. The ability of steam-powered warships to travel faster and more predictably than sail-powered ships gave rise to a new type of naval strategy that relied on steam’s power for maneuverability and speed. This transformation was most evident during the American Civil War, where steam-powered vessels played a critical role in naval engagements and blockades. The Ironclad warship—a steam-powered vessel protected by iron armor—ushered in a new era of naval warfare, with significant implications for military strategy and technology in the following decades.
The decline of the steam boat era came with the widespread adoption of diesel engines and more advanced internal combustion technologies. Diesel engines, which offered superior fuel efficiency, lower maintenance requirements, and the ability to run on a variety of fuels, quickly overtook steam power in both commercial and military vessels. However, steam-powered ships did not disappear entirely. They remained in operation for many years, particularly in ocean liners and military vessels, and they were still considered reliable and powerful in certain situations. Even today, the legacy of the steam-driven boat lives on in the form of steam turbines used in large vessels, power plants, and other industrial applications. The transition to more modern propulsion systems has not erased the significance of steam power, but rather integrated it into new forms of energy generation and transportation.
In conclusion, the steam-driven boat represents a milestone in human ingenuity, industrial advancement, and societal transformation. Its contributions to the realms of global trade, military power, transportation infrastructure, and technological progress are immeasurable. The steam boat laid the foundation for many of the engineering marvels that followed, while also fostering important social, political, and economic changes that continue to resonate today. While modern propulsion systems have replaced steam-powered vessels in most commercial and military applications, the steam-driven boat’s legacy remains a crucial chapter in the history of human progress and innovation.
Here are five main topics that encapsulate the key aspects of the steam-driven boat:
- Technological Innovation and Engineering
This topic covers the advancements in steam engine technology, including the development of steam engines, the shift from sail to steam power, and key innovations like the paddle wheel and screw propeller. It also explores the evolution of steam engines, from early designs to the steam turbines used in modern applications. - Impact on Global Trade and Economic Development
This area discusses how steam-driven boats revolutionized global trade, creating regular, reliable shipping routes, reducing transportation costs, and facilitating the movement of goods and people across oceans. The steam boat contributed to the expansion of international commerce, economic growth, and the development of key port cities. - Social, Cultural, and Military Effects
This topic delves into the broader cultural implications of steam boats, from their influence on the Industrial Revolution to the way they changed how people perceived technology and progress. It also highlights their role in military strategy, particularly during the American Civil War and the introduction of ironclad warships. - Environmental Impact and Sustainability
The environmental consequences of steam boats, particularly their reliance on coal, are explored here. This section looks at the challenges posed by pollution and the eventual transition to more sustainable energy sources in maritime transportation. - Legacy and Modern Applications
This topic examines the enduring legacy of steam-driven boats, focusing on how steam technology influenced modern engineering and power generation systems, including the use of steam turbines in electric power plants and naval vessels. It also touches on the continued relevance of steam power in certain sectors, despite the rise of more efficient technologies.
Technological Innovation and Engineering
The steam-driven boat was one of the most significant innovations in the history of transportation, revolutionizing both maritime and land-based engineering practices. Its technological advancements were pivotal in shaping the trajectory of industrialization and engineering. At the heart of the steam boat was the steam engine, a device that converted heat energy from burning fuel into mechanical energy. The first practical steam engines were developed in the late 17th and early 18th centuries, but it was the integration of steam engines into boats that truly marked a turning point in engineering.
In its earliest forms, the steam engine was rudimentary, and it struggled with issues like inefficiency and limited power. However, as inventors and engineers like James Watt refined the design, steam engines became more powerful, reliable, and efficient. Watt’s improvements in the double-acting engine, which allowed for continuous power, were crucial for the success of steam-powered boats. His advancements made it possible to produce the consistent and reliable power needed to propel vessels at sea. This innovation was instrumental in overcoming one of the major challenges faced by earlier steam-powered watercraft, which was the ability to generate enough power to move a boat against currents and winds.
The early steam-powered boats relied on paddle wheels as a means of propulsion. These large, rotating wheels located at the sides or rear of the vessel were powered by steam engines that drove pistons connected to the wheels. The paddle wheel was an important early solution for creating forward motion, but it had several drawbacks. The paddles were not as efficient in rough seas, and they required significant maintenance and fuel to operate. Nonetheless, the paddle wheel allowed for the initial success of steam boats, enabling vessels to travel faster and more reliably than traditional sailing ships or oar-powered boats.
As steam technology advanced, so too did the design of steam boats. One of the key innovations in this field was the development of the screw propeller. Invented by Isambard Kingdom Brunel in the 1830s, the screw propeller provided a more efficient means of propulsion compared to the paddle wheel. Unlike paddle wheels, which generated significant drag in the water, the screw propeller worked by rotating a helical screw through the water, creating thrust while minimizing drag. This innovation drastically improved the performance of steam-driven boats, particularly in rough waters and at higher speeds. The screw propeller’s efficiency made it a preferred choice for many steam-powered vessels, particularly as ships became larger and required more powerful engines.
Another critical advancement in the technology of steam-driven boats was the steam turbine. By the late 19th century, the steam turbine became an important part of marine propulsion systems, particularly for larger, high-speed vessels. Unlike the reciprocating steam engines, which used pistons to drive mechanical movement, the steam turbine used steam pressure to spin a set of blades connected to a shaft, generating continuous rotational power. The introduction of the steam turbine allowed for greater speeds and more efficient fuel consumption, especially in naval and commercial vessels. This technology was particularly useful in large ships, such as ocean liners and naval warships, where the demand for speed and power was high.
The use of steam turbines also led to the development of more sophisticated systems for power distribution and control. Modern steam turbines, for example, are equipped with governors that regulate the speed and power output by controlling the amount of steam fed into the turbine. These systems ensure that the vessel operates efficiently, without wasting fuel or exceeding operational limits. The ability to precisely control the steam engine’s output revolutionized not just maritime propulsion but also power generation in other sectors, including electricity generation and industrial machinery.
One of the most notable innovations in the engineering of steam-driven boats was the transition from coal-powered engines to oil and gas. While early steam boats relied heavily on coal, which was bulky, difficult to store, and produced significant pollution, the later adoption of oil as a fuel source allowed for more efficient and cleaner operations. Oil provided higher energy content per unit of fuel and was easier to store and transport. The shift to oil-powered steam engines allowed steam boats to operate for longer distances with fewer refueling stops, making them more reliable for long voyages. This shift also reduced some of the environmental challenges posed by coal, although the advent of cleaner technologies in the 20th century would eventually replace oil as the dominant fuel source in marine propulsion.
The mechanical components of steam engines, including pistons, cylinders, boilers, and valve mechanisms, evolved in tandem with improvements in steam power. Early steam engines had limitations in their design that hindered efficiency and power output. For example, single-cylinder engines often lacked the necessary pressure and power to move large ships at high speeds. Over time, multi-cylinder designs, which included both high-pressure and low-pressure cylinders, became the norm, allowing steam engines to operate more efficiently at higher power outputs. Innovations like compound engines, where steam is used in multiple stages, allowed engineers to extract more energy from the same amount of steam, increasing the overall efficiency of the system.
Moreover, the integration of steam engines with other marine systems, such as rudders, steering mechanisms, and navigation tools, resulted in the development of more complete and sophisticated vessels. Steam boats became not just powerful engines, but full-fledged maritime machines capable of navigating and operating in a wide variety of conditions. These vessels were equipped with advanced navigation technologies, such as compasses, sextants, and chronometers, which enabled them to travel vast distances across oceans with greater accuracy and reliability. The combination of steam propulsion with advanced navigational tools transformed the way ships were operated and played a pivotal role in the growth of global trade networks.
In summary, the technological innovations that led to the development of the steam-driven boat had far-reaching consequences not just for maritime engineering but for the broader industrial landscape. The evolution from simple steam engines to more advanced systems like steam turbines, oil-powered engines, and multi-cylinder designs contributed significantly to the efficiency and reliability of steam boats. These innovations not only made steam boats more powerful and versatile but also laid the groundwork for the continued advancement of industrial technologies in the 19th and 20th centuries. The legacy of steam boat engineering can be seen in modern maritime propulsion systems, power generation, and industrial machinery, all of which trace their origins to the innovations of the steam boat era.
Impact on Global Trade and Economic Development
The advent of steam-powered boats had a profound and lasting impact on global trade and economic development. Before the steam boat, maritime transport was heavily reliant on sailing ships, which were at the mercy of the wind and weather conditions. This made trade and transportation slow, unreliable, and often inefficient. The introduction of steam-powered vessels revolutionized maritime logistics, creating faster, more predictable, and more cost-effective means of transporting goods and people across vast distances. This transformation had far-reaching effects on global trade patterns, economic relationships, and the growth of key industries.
One of the most immediate effects of steam boats was the dramatic reduction in the time it took to travel between continents and major ports. Steam-powered vessels could travel at consistent speeds, regardless of wind conditions, and could maintain more regular schedules. This made trade routes more reliable, which in turn fostered increased commercial activity. Cities that were once distant or isolated from global trade networks suddenly found themselves linked to major economic hubs. The Atlantic Ocean became a bustling trade highway, as steam boats dramatically reduced travel times between Europe, the Americas, and the Caribbean. Similarly, steam boats on the Indian Ocean and Pacific Ocean enabled trade between Asia, Africa, and the Americas, opening up new markets and fostering global commerce on a scale that was previously unimaginable.
For countries with access to major bodies of water, the rise of steam boats facilitated the growth of port cities and trade centers. Cities such as New York, Liverpool, Shanghai, and Sydney became increasingly important as hubs of global commerce. The establishment of reliable steamship routes meant that goods could be transported in bulk more efficiently, allowing businesses to scale up their operations and expand their reach. For example, cotton from the southern United States could be transported more quickly to the mills of England, while tea from China and spices from India could reach European markets at a faster pace. This facilitated the growth of industries like textiles, agriculture, and mining, which depended on efficient transportation for raw materials.
The increased efficiency of steam-powered shipping also led to a reduction in shipping costs. With steam boats able to carry larger loads and maintain more reliable schedules, economies of scale came into play. The cost of transporting goods per ton decreased, allowing for the transportation of larger quantities of goods at a lower cost. This had an enormous effect on global markets, as it made goods more affordable and accessible to a wider population. As transportation costs fell, global supply chains grew, and nations could more easily access products that were previously expensive or hard to obtain. For example, the rise of steam-powered boats contributed to the mass production of goods, which could now be shipped to markets around the world with greater ease.
Steam boats also played a crucial role in the development of the transcontinental railroad systems, particularly in the United States. The completion of the First Transcontinental Railroad in 1869, coupled with the expansion of steam-powered boats along the Mississippi River and other major rivers, provided a more integrated transportation network that linked the east and west coasts of the United States. This integration facilitated the movement of goods and people across the country, creating a truly national economy. The railroad and steam boat systems worked in tandem to transport raw materials like coal, timber, and grain from the interior of the country to ports, where they could be shipped to international markets.
The economic impact of steam boats was not confined to Europe and North America. In regions such as Asia, Africa, and South America, steam-powered vessels played a crucial role in the colonial economies that were emerging at the time. European powers used steam boats to expand their influence and control over distant territories. Steamships enabled the rapid movement of troops, supplies, and goods to and from colonies, facilitating the expansion of imperial powers. In Africa, steam boats were used to navigate the Nile River and other major waterways, allowing colonial powers to assert greater control over the interior regions. Similarly, in South America, steam boats played a key role in connecting interior regions with coastal ports, enabling the flow of raw materials to European markets.
The rise of steam boats also contributed to the growth of the tourism industry. As steam-powered vessels made it easier for people to travel long distances by sea, they also opened up new avenues for leisure travel. The luxury ocean liners that emerged in the late 19th century became symbols of wealth and status, and they attracted tourists from around the world. Steam-powered boats enabled the rise of the transatlantic passenger trade, where wealthy individuals could travel in comfort and luxury between Europe and the United States. The development of leisure cruises and ocean travel played a significant role in the growth of the travel and hospitality industries, which became an important sector in many countries’ economies.
In addition to the economic opportunities created by steam boats, their widespread use also led to the growth of related industries. The demand for coal to fuel steam engines led to the expansion of coal mining operations in regions like Wales, Scotland, and the United States. The construction of steam-powered ships spurred the development of the shipbuilding industry, with major shipyards emerging in cities like Glasgow, Belfast, and New York. The engineering expertise required to design and maintain steam engines contributed to the growth of the broader mechanical engineering and manufacturing industries. The rise of steam boats thus played a crucial role in the broader industrialization of many nations.
At the same time, the increased volume of goods being transported by steam-powered vessels also contributed to the growth of ports and maritime infrastructure. Ports were expanded and modernized to accommodate larger steam-powered vessels, leading to the creation of new dockyards, warehouses, and logistics centers. This spurred investment in infrastructure and created a new set of economic opportunities in the cities that hosted major ports.
In conclusion, the steam-driven boat was a catalyst for global trade and economic development. Its ability to move goods faster, more reliably, and at a lower cost transformed the way nations interacted with each other in the marketplace. It facilitated the rise of international trade networks, helped shape the growth of industrial economies, and played a crucial role in the expansion of imperialism. The steam boat’s impact on global commerce continues to be felt today, as it laid the groundwork for the modern shipping and transportation systems that power the global economy.
Social, Cultural, and Military Effects
The steam-driven boat had profound social, cultural, and military effects that extended far beyond the confines of maritime transportation. As one of the first truly transformative technologies in the age of industrialization, the steam boat altered how people lived, worked, and interacted with the world. Its effects reached deep into the social fabric of both industrialized and colonial societies, influencing everything from labor and social mobility to military strategies and national identities.
In the social realm, the rise of steam-powered boats heralded a new era of mobility and connectivity. Previously, travel across oceans or rivers was time-consuming and unpredictable, relying heavily on wind patterns and weather conditions. The steam boat, with its mechanical propulsion, provided a reliable, consistent, and faster means of transportation. This increased mobility allowed for the easier movement of people across continents, fostering greater cultural exchanges and interaction. It opened up opportunities for individuals to travel for work, leisure, or even migration, reshaping the global movement of populations. For example, with the advent of steam-powered vessels, migration to the United States from Europe became far more feasible. Immigrants could cross the Atlantic in weeks instead of months, fundamentally altering the demographic makeup of many regions, especially the Americas.
The steam boat also played a significant role in reshaping urbanization and economic development. Major port cities, such as Liverpool, New York, San Francisco, and Shanghai, experienced rapid growth as a result of increased trade and more efficient transportation networks. These cities became hubs of commerce, attracting migrants, workers, and entrepreneurs seeking new opportunities. The rise of steam-powered ships facilitated the expansion of urban areas, especially those with access to key waterways and ports. It also contributed to the growth of industrial economies, with industries such as coal mining, shipbuilding, and engineering receiving significant boosts from the demand for steam vessels. The economic dynamism generated by steam-powered shipping and trade spurred the development of infrastructure, including ports, railways, warehouses, and communication networks.
On the cultural front, the steam boat became a symbol of technological progress and human ingenuity. It was hailed as a marvel of modern engineering, embodying the aspirations of the industrial age. The invention of the steam boat not only represented a leap forward in mechanical design but also captured the imagination of the public, becoming a focal point of cultural fascination. In literature, art, and music, steam boats were often portrayed as symbols of adventure, exploration, and innovation. In the United States, the rise of the steamboat culture was tied to the mythology of westward expansion and the American Dream. Steam-powered boats were celebrated as tools of progress and freedom, opening up the Mississippi River and other inland waterways to settlers and entrepreneurs looking to capitalize on new economic opportunities.
The social changes brought about by steam boats were not limited to those in urban and industrial centers. The accessibility of steam-powered vessels allowed for greater interaction between people in different parts of the world. Tourism, for example, grew significantly during the steam boat era, as travel became more affordable and accessible. Luxury ocean liners, such as those operated by Cunard and White Star Line, offered transatlantic voyages that catered to the wealthy elite, while more affordable routes brought mass numbers of immigrants and travelers across oceans. The steam boat became a tool of leisure and discovery, reshaping how people experienced the world beyond their immediate surroundings.
However, the impact of steam boats was not universally positive. The rise of steam-powered vessels exacerbated social inequalities in many societies. While they provided new opportunities for travel and trade, steam boats were also instrumental in the expansion of colonialism. European imperial powers used steam-powered ships to assert control over distant colonies, transporting troops, supplies, and resources with unprecedented speed and efficiency. Steam boats were crucial in establishing and maintaining European dominance over regions in Africa, Asia, and the Americas. In Africa, for example, steam boats were used to navigate the Nile and other inland rivers, enabling European powers to penetrate deep into the continent and establish colonial control. The steam boat, therefore, became a symbol of imperialism, carrying both economic exploitation and cultural dominance to far-flung regions of the world.
In terms of military effects, the steam boat had a transformative impact on naval warfare. The introduction of steam-powered ships marked the end of the era of sailing vessels and reshaped the tactics and strategies of naval combat. Steam-powered warships could now maneuver more effectively, travel faster, and be more predictable in their movements. This newfound mobility gave rise to modern naval warfare, as steam ships could quickly deploy forces and launch attacks without being reliant on wind patterns. The first major demonstration of the steam boat’s military significance came during the American Civil War, with the use of ironclad warships—steam-powered ships with iron plating that offered greater protection against enemy fire. The Battle of Hampton Roads in 1862, where the Union’s USS Monitor and the Confederate CSS Virginia fought, marked a pivotal moment in naval history and demonstrated the superiority of steam-powered ships in warfare.
The use of steam-powered boats in military operations also altered the strategic value of waterways and naval bases. As steam ships could cover long distances with relative ease, nations began to focus on securing key maritime routes and establishing naval superiority across the seas. The ability to project military power via steam-powered ships contributed to the establishment of powerful navies that could control vast oceanic territories. The British Empire, in particular, used its fleet of steam-powered warships to maintain dominance over its colonies and trade routes, effectively using the steam boat as a tool of imperial control.
Beyond naval combat, the steam boat played a key role in military logistics. The ability to quickly transport troops and supplies across seas and rivers had a significant impact on military campaigns. In both World War I and World War II, steam-powered ships were used to transport soldiers, ammunition, and equipment, and played a vital role in troop deployments, naval blockades, and amphibious assaults. While steam boats eventually gave way to more advanced technologies like diesel-powered ships and aircraft, their military role in the 19th and early 20th centuries cannot be overstated.
In conclusion, the social, cultural, and military effects of steam-driven boats were transformative and far-reaching. These vessels revolutionized how people traveled, traded, and interacted with one another, while also playing a critical role in military and imperial strategies. Steam boats contributed to the globalization of the economy, the spread of Western culture, and the expansion of empires, but they also amplified social inequalities and environmental degradation. They were symbols of progress and power, offering new opportunities for some while reinforcing the dominance of others. The legacy of steam boats, in both their positive and negative aspects, continues to influence the world today, shaping our understanding of technology, warfare, and the global economy.
Environmental Impact and Sustainability
The environmental impact of steam-driven boats has been a subject of considerable historical significance, particularly as the industrial era progressed and steam propulsion became the dominant mode of transportation for both goods and people. The steam boat, while a groundbreaking advancement in maritime engineering, also introduced significant environmental challenges that would have long-lasting effects. The primary environmental concern tied to steam-powered boats stems from their reliance on fossil fuels, particularly coal, as the primary energy source for their steam engines.
Coal, as the fuel of choice for early steam boats, brought with it a range of negative environmental consequences. The combustion of coal released large amounts of carbon dioxide (CO2) into the atmosphere, contributing to the buildup of greenhouse gases that would later become recognized as a driver of climate change. This was particularly concerning given the sheer volume of coal consumed by steam ships during their peak in the 19th and early 20th centuries. Steam boats required vast quantities of coal to operate, and the coal had to be mined, transported, and burned, each step contributing to environmental degradation.
The process of coal mining itself was highly destructive to the environment. As mining operations expanded to meet the growing demand for coal, landscapes were altered through deforestation, habitat destruction, and soil erosion. Surface mining techniques, such as mountaintop removal, became prevalent in certain areas, further disturbing ecosystems and waterways. Additionally, the transportation of coal to ports for steam boats relied heavily on railroads and horse-drawn carts, which added to the environmental toll by contributing to air pollution and the degradation of local infrastructure.
Once coal reached the steam boat, the environmental impacts persisted. The burning of coal in the boilers of steam-powered vessels produced not only CO2 but also other pollutants, including sulfur dioxide (SO2), nitrogen oxides (NOx), and particulate matter, which were released into the air and the surrounding waters. These pollutants contributed to the acidification of rainwater and the degradation of air quality, which could have negative health effects on both the crew of the vessel and the populations living near major shipping routes or ports. In addition, the excessive soot and ash produced by burning coal often settled on nearby vegetation, buildings, and landscapes, causing long-term damage to local environments and agricultural productivity.
Another environmental concern associated with steam boats was their impact on water quality. Steam boats operated in rivers, lakes, and oceans, often discharging waste materials directly into these bodies of water. The engines themselves would generate heat, which was often expelled into the water, raising the temperature and affecting local aquatic life. In addition to thermal pollution, steam boats were frequently responsible for the release of oil, grease, and other by-products from engine maintenance. As these pollutants entered the water, they could harm marine life and disrupt the delicate ecosystems that depended on clean water. The rise of steam boats also contributed to the erosion of riverbanks, particularly in areas where steam boats traveled frequently, as their large hulls and propellers disturbed the water and destabilized the surrounding landscape.
The proliferation of steam boats also led to the increased use of shipyards and other industrial facilities for the construction and maintenance of vessels. These industrial activities often involved the use of toxic materials, such as paints, solvents, and heavy metals, which could leach into the environment. Over time, shipbuilding became concentrated in urban areas near major bodies of water, where waste from industrial activities would inevitably flow into nearby rivers, lakes, or coastal areas, further exacerbating the environmental toll.
In terms of biodiversity, steam boats had an indirect impact through the alteration of natural habitats. As the demand for coal, iron, and other materials used in steam boat construction grew, vast swathes of land were cleared for mining, logging, and other forms of resource extraction. The expansion of port cities and shipping routes also contributed to the encroachment of urban development on natural habitats, leading to the displacement of plant and animal species. River systems, in particular, were often dredged or modified to accommodate the larger, heavier vessels, changing the natural flow of water and disrupting ecosystems. The introduction of steam boats into previously remote or ecologically sensitive areas could have lasting effects on the environment, as they opened up new regions to exploitation and human activity.
As awareness of environmental issues grew in the late 19th and early 20th centuries, there was increasing concern about the sustainability of steam-driven boats. The coal-powered ships were inherently inefficient and unsustainable due to the finite nature of fossil fuel resources and the environmental damage associated with their use. While steam boats were undeniably revolutionary for their time, their environmental costs became more apparent as the world moved toward a greater understanding of resource conservation and environmental stewardship.
In response to these challenges, the late 19th century saw the development of cleaner alternatives to the steam engine, such as the internal combustion engine and electric motors. The rise of diesel-powered ships in the 20th century offered a more efficient and less polluting alternative to the traditional coal-powered steam boat. Diesel engines produced less particulate matter and sulfur dioxide, and they were capable of running for longer periods without the need for constant refueling, reducing the environmental impact of marine transportation. The advent of nuclear power in the mid-20th century also offered the possibility of virtually emission-free power for ships, though its adoption in civilian maritime applications remained limited.
Despite these technological advances, the legacy of steam boats continues to impact discussions around sustainability and environmental protection in maritime industries. The environmental footprint of steam boats, particularly their contribution to air and water pollution, served as an early example of the hidden costs of industrial progress. Today, the lessons learned from the steam boat era are informing efforts to reduce the ecological impact of modern ships. Innovations in green shipping technologies, such as liquefied natural gas (LNG), wind-powered vessels, and solar energy, are helping to reduce the carbon footprint of global shipping. The move toward more sustainable and eco-friendly ships represents a modern continuation of the drive to balance technological advancement with environmental responsibility.
The environmental impact of steam boats also raises important questions about the balance between technological innovation and sustainability. While steam boats were a significant leap forward in transportation, their reliance on coal and other pollutants highlighted the long-term environmental costs of unchecked industrial progress. Today, as the world continues to grapple with the effects of climate change, the historical context of steam boats serves as a reminder of the need to prioritize sustainable practices in the development of future technologies. The shift away from fossil fuels, the exploration of renewable energy sources, and the adoption of cleaner technologies will be crucial in ensuring that the legacy of steam boats serves not only as a testament to human ingenuity but also as a cautionary tale for future generations.
EMS Power Machines
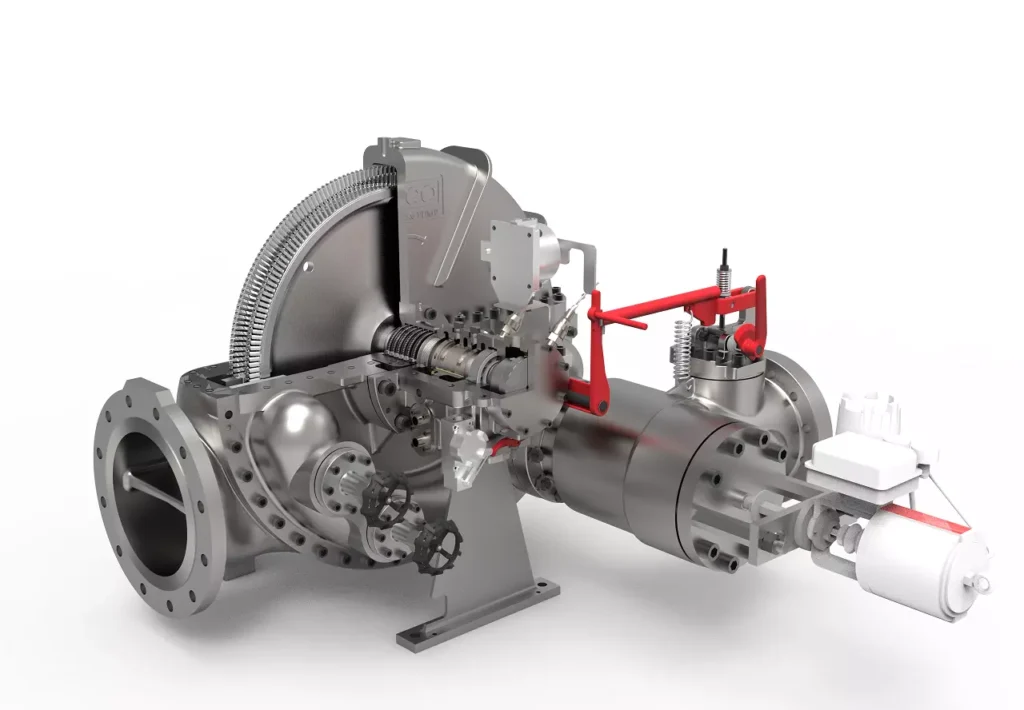
We design, manufacture and assembly Power Machines such as – diesel generators, electric motors, vibration motors, pumps, steam engines and steam turbines
EMS Power Machines is a global power engineering company, one of the five world leaders in the industry in terms of installed equipment. The companies included in the company have been operating in the energy market for more than 60 years.
EMS Power Machines manufactures steam turbines, gas turbines, hydroelectric turbines, generators, and other power equipment for thermal, nuclear, and hydroelectric power plants, as well as for various industries, transport, and marine energy.
EMS Power Machines is a major player in the global power industry, and its equipment is used in power plants all over the world. The company has a strong track record of innovation, and it is constantly developing new and improved technologies.
Here are some examples of Power Machines’ products and services:
- Steam turbines for thermal and nuclear power plants
- Gas turbines for combined cycle power plants and industrial applications
- Hydroelectric turbines for hydroelectric power plants
- Generators for all types of power plants
- Boilers for thermal power plants
- Condensers for thermal power plants
- Reheaters for thermal power plants
- Air preheaters for thermal power plants
- Feedwater pumps for thermal power plants
- Control systems for power plants
- Maintenance and repair services for power plants
EMS Power Machines is committed to providing its customers with high-quality products and services. The company has a strong reputation for reliability and innovation. Power Machines is a leading provider of power equipment and services, and it plays a vital role in the global power industry.
EMS Power Machines, which began in 1961 as a small factory of electric motors, has become a leading global supplier of electronic products for different segments. The search for excellence has resulted in the diversification of the business, adding to the electric motors products which provide from power generation to more efficient means of use.