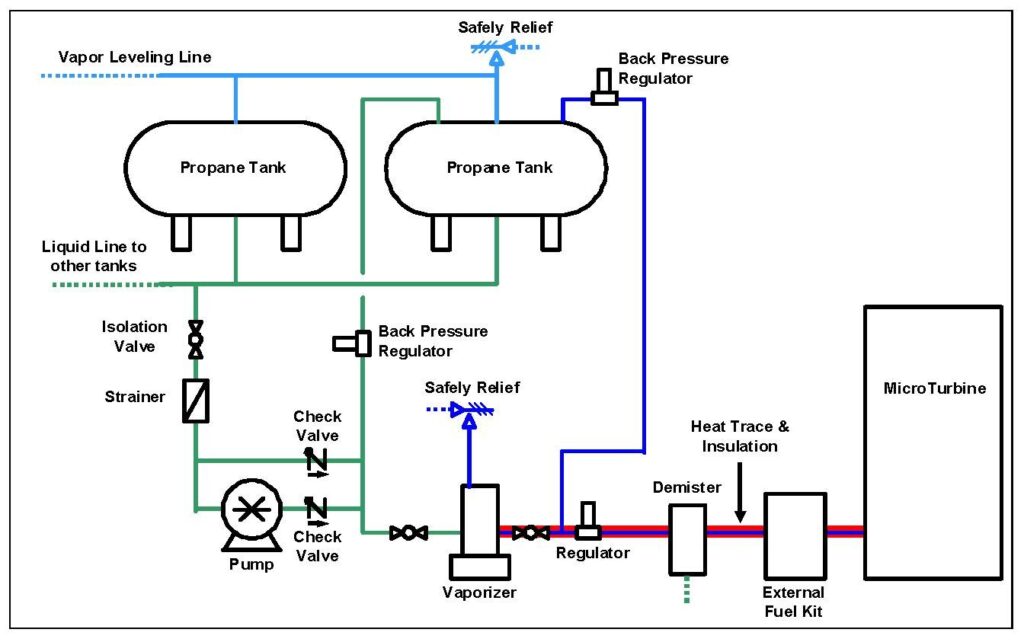
Generating Electricity using Heat from Liquefied Petroleum Gas (LPG) Production: Power plants utilizing steam turbines play a crucial role in global electricity generation. These plants operate on the principle of thermodynamic cycles, primarily the Rankine cycle, where water is heated to produce steam that drives a turbine connected to a generator. The process begins with a heat source, which can vary depending on the type of power plant. The most common sources include fossil fuels such as coal, natural gas, and oil, as well as nuclear energy, biomass, geothermal heat, and solar thermal energy. Regardless of the heat source, the goal is to convert thermal energy into mechanical energy and ultimately into electrical energy.
In a typical steam power plant, water is first fed into a boiler or steam generator, where it is heated under high pressure to produce superheated steam. This steam is then directed through a series of high-pressure turbines, where it expands and causes the turbine blades to rotate. The rotational energy is transferred to a generator via a shaft, converting mechanical energy into electrical power. After passing through the high-pressure turbine, the steam moves into intermediate- and low-pressure turbines, further expanding and transferring additional energy before being exhausted into a condenser.
The condenser plays a vital role in maintaining efficiency by cooling and condensing the steam back into liquid form. This condensed water, known as condensate, is then pumped back into the boiler through feedwater heaters that recover residual heat from extracted steam. This regenerative heating process improves overall efficiency by reducing the amount of fuel needed to reheat the water to its boiling point. The cycle continues, maintaining a continuous and efficient process of electricity generation.
Different types of steam power plants exist based on fuel source and operational characteristics. Fossil fuel power plants, including coal-fired and gas-fired plants, rely on burning hydrocarbons to generate heat. Coal-fired plants, though historically dominant, are facing increasing scrutiny due to environmental concerns related to carbon emissions and air pollution. Advanced technologies, such as supercritical and ultra-supercritical steam cycles, have been developed to enhance efficiency and reduce emissions. Natural gas power plants, particularly those using combined cycle technology, integrate steam turbines with gas turbines to maximize energy extraction, significantly increasing efficiency.
Nuclear power plants operate similarly but use nuclear fission to generate heat. In a pressurized water reactor (PWR), the reactor heats water in a primary loop, which then transfers heat to a secondary loop that generates steam to drive the turbine. Boiling water reactors (BWRs) produce steam directly within the reactor vessel. Nuclear plants provide a stable and continuous power supply, making them a key source of baseload electricity. However, they require strict safety measures and long-term solutions for radioactive waste disposal.
Renewable energy sources such as biomass, geothermal, and solar thermal also employ steam turbines. Biomass plants burn organic materials like wood, agricultural waste, or municipal solid waste to generate steam. Geothermal power plants utilize heat from beneath the Earth’s surface, extracting steam or hot water from underground reservoirs. Flash steam, dry steam, and binary cycle geothermal plants use different methods to harness this heat efficiently. Solar thermal power plants, such as concentrated solar power (CSP) systems, use mirrors to focus sunlight onto a receiver that heats a working fluid, producing steam to drive a turbine.
Efficiency improvements in steam power plants focus on advanced turbine designs, higher steam temperatures and pressures, and integrated heat recovery systems. Technologies such as cogeneration, also known as combined heat and power (CHP), increase efficiency by utilizing waste heat for industrial processes or district heating. Additionally, carbon capture and storage (CCS) is being explored to mitigate emissions from fossil fuel-based plants.
Steam turbine power plants continue to be a backbone of global electricity production due to their reliability and scalability. However, the transition towards cleaner and more sustainable energy sources is shaping the future of steam power technology. Research and development efforts are aimed at improving efficiency, reducing emissions, and integrating renewable energy with conventional steam turbine systems. The continued evolution of steam power plants will play a crucial role in balancing energy demand, environmental responsibility, and economic viability.
Small Scale Power Plants
Further advancements in steam power plant technology have been driven by the need for higher efficiency, reduced environmental impact, and increased reliability. One of the most significant developments has been the transition from subcritical steam cycles to supercritical and ultra-supercritical cycles. In subcritical plants, steam is generated at pressures below the critical point of water, meaning a distinct phase change from water to steam occurs in the boiler. However, supercritical plants operate at pressures above the critical point, eliminating the phase transition and allowing for more efficient thermal energy conversion. Ultra-supercritical plants push these limits further, achieving even higher steam temperatures and pressures, which significantly improve efficiency and reduce fuel consumption.
In addition to improvements in steam parameters, turbine technology itself has evolved. Modern turbines are designed with advanced aerodynamics, optimized blade profiles, and high-strength materials capable of withstanding extreme temperatures and pressures. Many large-scale power plants use multi-stage turbines, where high-pressure, intermediate-pressure, and low-pressure stages work together to extract the maximum amount of energy from the steam. Efficiency enhancements also come from reducing steam leakage, improving blade cooling techniques, and using coatings that prevent corrosion and wear.
Heat recovery is another crucial aspect of efficiency improvement in steam power plants. The use of regenerative feedwater heating allows for better utilization of extracted steam, reducing the amount of additional heat energy required in the boiler. Condenser efficiency is also vital, as the faster and more effectively steam is condensed back into water, the better the vacuum conditions in the turbine, which enhances power output. In combined cycle power plants, waste heat from a gas turbine is used to generate additional steam for a steam turbine, dramatically improving overall efficiency.
The environmental impact of steam power plants is a major concern, particularly for fossil fuel-based systems. Carbon dioxide (CO₂) emissions from coal and natural gas plants contribute to climate change, leading to increased focus on carbon capture and storage (CCS) technologies. These systems aim to capture CO₂ before it is released into the atmosphere and store it underground or utilize it in industrial applications. Other emission control technologies include flue gas desulfurization (FGD) for removing sulfur dioxide (SO₂), selective catalytic reduction (SCR) for nitrogen oxides (NOx), and particulate matter filters to reduce air pollution.
Water usage is another important factor in steam power plants. Traditional once-through cooling systems withdraw large amounts of water from natural sources, raising concerns about thermal pollution and water scarcity. As a result, many plants now use closed-loop cooling systems with cooling towers or air-cooled condensers to minimize water consumption. In regions with limited water resources, dry cooling technology is being adopted, though it can result in slightly lower plant efficiency due to higher condenser temperatures.
The role of digitalization and automation in modern steam power plants is growing, with advanced monitoring and control systems enhancing performance and reliability. Predictive maintenance using artificial intelligence (AI) and machine learning algorithms helps detect potential failures before they occur, reducing downtime and maintenance costs. Sensors and data analytics provide real-time insights into turbine performance, boiler efficiency, and emissions control, allowing for continuous optimization.
In addition to large centralized power plants, smaller-scale steam turbine systems are being used for industrial applications and distributed energy generation. Combined heat and power (CHP) plants, often found in industrial facilities, universities, and district heating systems, provide both electricity and useful heat, significantly increasing overall energy utilization. Some biomass and waste-to-energy plants use steam turbines to generate power while simultaneously addressing waste disposal and reducing landfill use.
The integration of renewable energy with steam turbine technology is another emerging trend. Hybrid systems that combine solar thermal energy with traditional steam power plants can improve efficiency and reduce fuel consumption. Some geothermal power plants utilize steam turbines to harness Earth’s natural heat, providing a renewable and stable source of electricity. In the long term, advancements in hydrogen combustion and zero-carbon fuels could allow steam power plants to operate sustainably while maintaining their reliability and large-scale energy production capabilities.
As global energy demand continues to grow, steam power plants will remain a key component of the energy mix. While renewable energy sources such as wind and solar are expanding rapidly, steam turbine plants provide a crucial source of baseload and dispatchable power. Future developments will likely focus on improving efficiency, reducing emissions, and integrating cleaner energy sources to ensure that steam power generation remains both viable and sustainable in a rapidly changing energy landscape.
Further improvements in steam turbine technology are focusing on efficiency gains through material advancements, operational optimizations, and alternative fuel sources. One of the key challenges in steam power plants is the thermal efficiency limitation imposed by the Carnot cycle. Since efficiency is directly related to the temperature difference between the heat source and the heat sink, researchers are exploring ways to increase maximum operating temperatures while maintaining the structural integrity of turbine components.
Advanced materials, such as nickel-based superalloys and ceramic matrix composites, are being developed to withstand extreme temperatures and pressures, allowing turbines to operate at higher efficiencies. These materials offer better resistance to thermal stress, oxidation, and corrosion, reducing maintenance needs and extending component lifespan. Additionally, advancements in coatings, such as thermal barrier coatings (TBCs), are helping to protect turbine blades from high-temperature degradation, further improving operational longevity.
Operational optimizations are also playing a crucial role in enhancing steam turbine performance. Digital twin technology, where a virtual model of a steam power plant is continuously updated with real-time data, is being used to optimize performance and predict potential failures. This approach allows operators to test different scenarios in a simulated environment, leading to better decision-making and efficiency improvements. Artificial intelligence (AI) and machine learning are being integrated into plant management systems to analyze vast amounts of operational data and identify opportunities for optimization.
One promising area of research is the development of closed-loop supercritical carbon dioxide (sCO₂) cycles as an alternative to traditional steam-based Rankine cycles. sCO₂ operates at higher efficiencies due to its superior thermodynamic properties, allowing for compact turbine designs and reduced heat losses. These systems have the potential to significantly improve efficiency while reducing water consumption, making them particularly attractive for arid regions where water availability is limited.
Another critical area of innovation is the transition toward alternative fuels in steam power plants. While coal remains a dominant fuel source in some regions, there is a growing push toward cleaner alternatives such as biomass, hydrogen, and ammonia. Biomass co-firing, where biomass is blended with coal, reduces net CO₂ emissions while utilizing existing infrastructure. Hydrogen, when produced using renewable energy sources, presents a zero-carbon alternative for steam generation, though challenges remain in terms of production costs and storage. Ammonia, which can be used as a hydrogen carrier, is also being explored as a potential fuel for steam power plants due to its high energy density and ease of transportation.
Nuclear steam power is another area of ongoing innovation. Small modular reactors (SMRs) are being developed as a more flexible and scalable alternative to traditional large nuclear power plants. These reactors use steam turbines for power generation but feature enhanced safety mechanisms, simplified designs, and reduced construction times. SMRs are expected to play a significant role in the future energy landscape, providing reliable, low-carbon electricity with improved adaptability to grid demands.
Hybrid power systems that integrate steam turbines with renewable energy sources are also being explored to enhance grid stability and reduce reliance on fossil fuels. Solar thermal plants with molten salt storage can generate steam for turbines even when sunlight is unavailable, providing a continuous power supply. Geothermal power plants utilizing steam turbines can serve as a baseload energy source, complementing intermittent renewables like wind and solar.
Waste heat recovery systems are further improving the efficiency of industrial processes and power generation. By capturing excess heat from industrial operations or power plants and using it to generate steam, these systems maximize energy utilization and reduce environmental impact. Combined cycle plants, which pair gas turbines with steam turbines, are an example of how waste heat can be effectively repurposed to increase overall efficiency.
As the global energy transition continues, steam turbine power plants will need to adapt to new regulatory requirements, carbon reduction targets, and evolving market demands. The future of steam power lies in greater efficiency, integration with cleaner energy sources, and the adoption of advanced digital technologies. With ongoing research and development, steam turbines will continue to be a vital part of the world’s energy infrastructure, balancing the need for reliability, sustainability, and economic feasibility.
The future of steam power plants is increasingly shaped by the need for sustainability, flexibility, and technological advancements. As global energy systems evolve, steam turbine technology must adapt to integrate with renewable energy, reduce emissions, and enhance efficiency through innovative engineering solutions. The role of steam turbines in power generation is being redefined, particularly as the world shifts toward decarbonization and grid modernization.
One of the most significant trends is the integration of steam turbines with renewable energy sources to create hybrid power systems. Solar thermal power plants, also known as concentrated solar power (CSP) plants, use mirrors to focus sunlight onto a receiver, which heats a working fluid to generate steam. Unlike photovoltaic solar panels that directly convert sunlight into electricity, CSP plants utilize steam turbines to produce power in a manner similar to conventional fossil-fuel plants. The advantage of CSP technology is its ability to incorporate thermal energy storage, such as molten salt systems, which allow for electricity generation even after sunset. This helps address the intermittency issues associated with solar energy and provides a stable, dispatchable power source.
Another promising area of development is geothermal power generation. Geothermal plants rely on heat from the Earth’s core to produce steam, which drives turbines to generate electricity. Unlike fossil fuel-based plants, geothermal energy is a renewable and consistent source of power with minimal carbon emissions. Enhanced geothermal systems (EGS) are being developed to expand the reach of geothermal energy by artificially creating reservoirs in deep rock formations, significantly increasing the potential for steam-based renewable electricity.
The role of steam turbines is also being explored in the hydrogen economy. Hydrogen combustion in steam power plants could provide a zero-emission alternative to natural gas and coal. Hydrogen-fueled boilers and combined-cycle plants are being tested as a means of decarbonizing traditional steam-based power generation. However, large-scale adoption will depend on advancements in hydrogen production, storage, and transportation infrastructure. Ammonia, as a hydrogen carrier, is being investigated as an alternative fuel for steam power plants, offering a potentially viable pathway for carbon-free electricity generation.
In parallel with fuel source diversification, advancements in turbine efficiency and design are pushing the limits of what steam power plants can achieve. Supercritical and ultra-supercritical steam cycles have already improved efficiency by increasing steam temperature and pressure, but new research is focusing on advanced cycles such as supercritical carbon dioxide (sCO₂) power cycles. Unlike traditional steam turbines, sCO₂ turbines operate at higher densities and require smaller components, allowing for more compact and efficient power plant designs. These systems have the potential to revolutionize steam-based power generation by reducing material costs, enhancing thermal efficiency, and minimizing water consumption.
The digital transformation of power plants is another key factor in the evolution of steam turbine technology. Modern plants are increasingly adopting advanced monitoring systems, artificial intelligence (AI), and automation to optimize performance, reduce downtime, and improve predictive maintenance. Digital twins—real-time virtual replicas of physical steam turbines—are being used to simulate operations, predict failures, and test optimization strategies before implementing them in actual power plants. This technology enhances reliability, reduces maintenance costs, and extends the lifespan of critical components.
The role of steam power plants in industrial applications is also expanding beyond traditional electricity generation. Industrial steam turbines are widely used in cogeneration (combined heat and power, or CHP) systems, which provide both electricity and process heat for manufacturing, chemical production, and district heating. These systems significantly increase overall energy efficiency by utilizing waste heat that would otherwise be lost in conventional power plants. Many industries, including pulp and paper, food processing, and petrochemicals, rely on steam turbines to meet their energy demands efficiently.
Despite these advancements, the transition toward a low-carbon future presents challenges for existing steam power plants, particularly those dependent on fossil fuels. Governments and regulatory bodies worldwide are implementing stricter emissions standards, prompting the need for carbon capture and storage (CCS) technologies. CCS systems capture CO₂ emissions from power plants and store them underground or use them for industrial applications, such as enhanced oil recovery or synthetic fuel production. While CCS offers a potential solution for reducing the carbon footprint of steam power plants, high costs and energy penalties associated with the process remain significant hurdles.
Additionally, the global shift toward decentralized and distributed energy generation is changing the way steam turbines fit into the energy landscape. Traditionally, large central power stations with steam turbines supplied electricity to vast regions, but modern energy grids are moving toward a mix of smaller, localized generation sources. This trend is driven by the expansion of renewable energy, microgrids, and energy storage technologies. To remain competitive, steam power plants must adapt to provide flexible and responsive generation, potentially operating in hybrid configurations with renewables and battery storage.
Ultimately, the future of steam power generation will be defined by a balance between innovation, environmental responsibility, and economic feasibility. While the share of fossil fuel-based steam power is expected to decline, steam turbine technology will continue to play a critical role in providing reliable baseload power, supporting industrial processes, and integrating with new energy systems. Whether through advanced steam cycles, alternative fuels, digital optimization, or hybrid renewable systems, steam turbines are set to remain a cornerstone of global energy infrastructure in the coming decades.
Small-scale power plants for landfill waste-to-energy (WTE) applications provide a sustainable solution for urban districts dealing with waste management and power reliability issues. These plants convert municipal solid waste (MSW) into electricity and heat through various thermal and biological processes, reducing landfill volumes while generating local, decentralized power. By integrating steam turbine technology with waste processing, small-scale landfill WTE plants offer a dual benefit: waste reduction and energy production, making them an attractive solution for cities looking to enhance sustainability and energy security.
Waste-to-Energy Conversion Methods in Small-Scale Plants
Several technologies can be used to convert landfill waste into energy, each with its advantages in terms of efficiency, environmental impact, and feasibility for small-scale applications:
- Incineration with Steam Turbines
- The most common method for landfill WTE plants is mass-burn incineration, where municipal solid waste is combusted in high-temperature furnaces.
- The heat generated from combustion produces steam, which drives a small steam turbine to generate electricity.
- Modern small-scale incineration plants incorporate advanced air pollution control systems (e.g., scrubbers, filters, and catalytic converters) to minimize emissions of particulates, dioxins, and NOx gases.
- These systems can be designed to support Combined Heat and Power (CHP), providing district heating or industrial process heat in addition to electricity.
- Gasification and Pyrolysis
- Gasification and pyrolysis break down waste at high temperatures in a low-oxygen or oxygen-free environment, converting it into syngas (a mixture of hydrogen, carbon monoxide, and methane).
- The syngas can then be combusted in a boiler to produce steam for a turbine or used in gas engines and fuel cells for electricity generation.
- These methods produce fewer emissions compared to direct incineration and allow for greater flexibility in fuel sources, including plastics and organic waste fractions.
- Gasification can also be integrated with carbon capture technologies, further reducing environmental impact.
- Landfill Gas (LFG) to Energy
- As organic waste decomposes in landfills, it releases methane, a potent greenhouse gas. Instead of allowing this gas to escape into the atmosphere, small-scale landfill WTE plants can capture it and use it as a fuel source.
- The methane-rich biogas is typically used in internal combustion engines or microturbines to generate electricity, but it can also be burned in a boiler to generate steam for turbines.
- This approach is particularly cost-effective for existing landfills with large amounts of organic waste, as it provides an ongoing energy source without requiring additional feedstock.
Advantages of Small-Scale Landfill WTE Plants
- Localized Energy Generation: By producing electricity and heat close to the point of consumption, small-scale WTE plants reduce transmission losses and enhance grid stability.
- Waste Reduction: Incineration and gasification can reduce landfill volumes by up to 90%, extending landfill lifespans and reducing the need for new waste disposal sites.
- Lower Greenhouse Gas Emissions: Converting landfill gas to energy prevents methane emissions, significantly reducing the carbon footprint of waste management.
- Revenue Generation: In addition to electricity sales, WTE plants can generate revenue from tipping fees, carbon credits, and by-products like ash (which can be used in construction materials).
- Energy Resilience: These plants can operate as microgrids, providing backup power during grid outages and supporting critical infrastructure.
Challenges and Future Innovations
Despite their advantages, small-scale landfill WTE plants face several challenges:
- Public Perception and Environmental Concerns: Incineration and gasification plants must comply with strict emissions regulations and address community concerns about air pollution.
- Feedstock Variability: MSW composition varies significantly, affecting combustion efficiency and energy output. Advanced sorting and preprocessing technologies can help optimize performance.
- Regulatory Barriers: Permitting for WTE plants can be complex due to stringent environmental regulations, requiring compliance with waste management, air quality, and energy policies.
Future innovations aim to enhance the efficiency and sustainability of small-scale landfill WTE plants:
- Plasma Arc Gasification: A next-generation technology that uses extremely high temperatures to break down waste into clean syngas with minimal emissions.
- Carbon Capture and Utilization (CCU): Emerging WTE plants are integrating CO₂ capture systems to reduce emissions and repurpose carbon for industrial applications or synthetic fuels.
- Hybrid Renewable Systems: Pairing WTE plants with solar, wind, or battery storage can improve overall energy reliability and efficiency.
Conclusion
Small-scale landfill waste-to-energy plants represent a practical solution for urban districts struggling with both waste management and power reliability. By converting landfill waste into electricity and heat using steam turbines, gasification, or landfill gas capture, these plants support sustainable energy production while reducing environmental impact. As technology advances and regulatory frameworks evolve, small-scale WTE facilities will play an increasingly important role in the circular economy, transforming urban waste into a valuable energy resource.
Small-scale landfill waste-to-energy (WTE) plants present a sustainable solution to urban waste management challenges while also addressing energy reliability concerns. By converting landfill waste into electricity and heat, these plants not only help reduce waste volumes but also provide a local, dependable power source for communities. With various technologies available, including incineration, gasification, and landfill gas capture, small-scale WTE plants can be tailored to fit the needs of specific urban districts, particularly in areas facing frequent power outages or grid instability.
Incineration is the most widely used method in small-scale WTE plants. The waste is burned in high-temperature furnaces, and the resulting heat is used to produce steam that drives a turbine for electricity generation. These plants can be configured as combined heat and power (CHP) systems, which provide both electricity and useful thermal energy for district heating or industrial applications. To ensure minimal environmental impact, modern WTE plants are equipped with advanced air pollution control systems, which limit harmful emissions such as particulates, nitrogen oxides, and dioxins. These measures make small-scale incineration plants viable even in densely populated urban areas.
Another growing technology for landfill waste-to-energy is gasification. This process involves heating waste in a low-oxygen environment, which causes it to break down into syngas (a mixture of hydrogen, carbon monoxide, and methane) rather than directly combusting it. This syngas can then be used to generate electricity or further processed for other applications. Gasification offers advantages in terms of efficiency and emission reduction, as it produces fewer pollutants compared to traditional incineration. Additionally, gasification can handle a wider variety of waste types, including plastics and organic materials, making it a flexible and attractive option for small-scale plants.
Landfill gas-to-energy (LFG) systems capture methane emissions from landfills, converting this potent greenhouse gas into usable energy. By extracting methane from landfills and burning it in turbines or internal combustion engines, small-scale WTE plants can generate electricity while preventing harmful emissions from escaping into the atmosphere. This method is particularly useful for existing landfills with substantial organic waste decomposition, as it utilizes gas that would otherwise go untapped. The energy generated from landfill gas can be used locally, either for power generation or to supplement heating systems, and it can serve as a reliable source of energy for communities near landfills.
The benefits of small-scale landfill WTE plants are numerous. They offer a dual advantage of waste reduction and energy production, providing a sustainable alternative to traditional waste disposal methods. By reducing the volume of landfill waste by up to 90%, these plants help extend the lifespan of landfills and mitigate the environmental impact of waste accumulation. Furthermore, by harnessing landfill gas and converting waste into electricity and heat, these plants reduce reliance on fossil fuels, contributing to a cleaner, more sustainable energy mix.
From an energy security perspective, small-scale WTE plants provide localized power generation, which reduces dependence on centralized grids. This makes them particularly valuable in urban districts prone to power outages or facing grid instability. These plants can operate as part of microgrids, ensuring that critical infrastructure, such as hospitals, schools, and government buildings, remain operational even during power disruptions. In addition to improving power reliability, small-scale WTE plants contribute to the overall resilience of the energy system by diversifying the energy sources and increasing local self-sufficiency.
Despite their potential, small-scale landfill WTE plants face certain challenges. Public perception of waste-to-energy technologies can be negative due to concerns about air pollution, particularly from incineration. To mitigate these concerns, modern WTE plants use state-of-the-art emissions control technology to minimize their environmental footprint, but they still face regulatory hurdles and community opposition. Another challenge is the variability of waste feedstocks, which can affect plant performance and energy output. Variations in waste composition require advanced sorting and preprocessing systems to ensure that the plant operates efficiently and generates consistent energy.
Looking ahead, several innovations are set to improve the efficiency and sustainability of small-scale landfill WTE plants. Plasma arc gasification, a next-generation waste conversion technology, uses extremely high temperatures to break down waste into clean syngas with minimal emissions, offering a cleaner alternative to traditional methods. Additionally, carbon capture and utilization (CCU) technologies are being integrated into some WTE plants to capture CO2 emissions, reducing their environmental impact while also enabling the reuse of captured carbon for industrial purposes. Hybrid systems that combine waste-to-energy with renewable energy sources, such as solar or wind, are also becoming more common, helping to increase the overall energy reliability and reduce the plant’s carbon footprint.
Ultimately, small-scale landfill waste-to-energy plants offer a valuable solution for urban districts struggling with waste management and power reliability. By transforming landfill waste into a renewable energy resource, these plants support local energy needs while addressing environmental concerns. As technology advances, and as urban areas continue to grapple with the challenges of waste disposal and energy supply, the role of waste-to-energy systems will become increasingly important in creating resilient, sustainable cities.
As small-scale landfill waste-to-energy (WTE) plants evolve, their role in urban energy systems will continue to expand, driven by technological advancements, environmental goals, and the growing need for sustainable waste management solutions. As cities face increased pressure to reduce landfill waste and improve energy reliability, the integration of WTE systems offers a practical, scalable solution for both power generation and waste reduction. These plants not only help mitigate the environmental impact of landfills but also contribute to energy diversification and carbon footprint reduction.
In addition to the key technologies of incineration, gasification, and landfill gas recovery, further improvements in small-scale WTE plants are being driven by a focus on efficiency, fuel flexibility, and environmental impact. Advanced combustion and thermal treatment technologies are being developed to maximize the energy output from waste, increase operational efficiency, and reduce emissions. For instance, fluidized bed combustion systems, which use a bed of sand or other granular material to enhance the combustion process, can handle waste materials with high moisture content, such as food waste or certain industrial by-products, that traditional incinerators struggle with. These systems offer a more flexible, cost-effective solution for small-scale WTE plants in urban districts where waste composition varies widely.
Another innovation gaining traction in WTE technologies is the use of supercritical steam cycles in small-scale plants. In traditional subcritical steam turbines, steam is generated at lower pressure and temperature, limiting overall efficiency. However, supercritical steam cycles operate at higher pressures and temperatures, allowing the steam to have more energy, which is then converted into more electricity. This significantly increases the efficiency of small-scale WTE plants, making them more competitive in the energy market. This technology has historically been used in large, central power plants but is now being scaled down for smaller applications, helping to meet the increasing energy demand from urban districts while reducing operational costs.
Fuel flexibility remains a major focus for small-scale landfill WTE plants. Waste-to-energy systems are typically fueled by municipal solid waste, but there is growing interest in using alternative fuels, including biomass and plastic waste. As cities look to move away from single-use plastics, converting waste plastics into fuel through pyrolysis or gasification could provide a cleaner, more efficient energy source, while also addressing growing concerns over plastic pollution. Additionally, some WTE plants are exploring the use of co-firing with renewable fuels like wood chips or agricultural waste, which helps lower emissions and provide a reliable, continuous fuel supply. This co-firing strategy helps optimize fuel usage and reduce the environmental impact of traditional waste processing methods, especially in urban areas with abundant biomass resources.
The environmental impact of small-scale landfill WTE plants is increasingly being mitigated through the integration of carbon capture, utilization, and storage (CCUS) technologies. As carbon emissions are a key concern for waste-to-energy operations, capturing CO2 produced during the combustion or gasification process can help reduce the overall carbon footprint of these plants. The captured carbon can either be stored underground (carbon sequestration) or utilized in commercial applications, such as creating synthetic fuels, or in manufacturing materials like concrete. Some innovative systems are even working on using the captured CO2 to create value-added products like algae-based biofuels or chemicals, offering a potential revenue stream in addition to the energy produced.
Furthermore, the hybridization of small-scale landfill WTE plants with renewable energy technologies, such as solar, wind, and battery storage, is becoming a growing trend. By pairing waste-to-energy with renewable power generation, these plants can provide a more stable and resilient energy supply to urban districts. For instance, during periods when landfill waste generation is lower or the WTE plant is undergoing maintenance, solar or wind power can help meet electricity demand, while energy storage systems can smooth out fluctuations in supply. This hybrid approach enables the integration of multiple energy sources, creating a more reliable, sustainable, and flexible energy system that is resilient to grid disruptions and increases energy independence for urban districts.
Small-scale WTE plants are also becoming more digitally connected and data-driven, using sensors, artificial intelligence (AI), and predictive analytics to improve operational performance and reduce maintenance costs. These systems enable plant operators to monitor waste composition, optimize combustion or gasification processes, and predict when equipment might need maintenance or repair, improving plant efficiency and reducing downtime. Additionally, data from these systems can be used to inform waste management strategies, helping cities better manage waste streams and optimize resource recovery.
The role of microgrids is becoming increasingly important in urban energy strategies. Small-scale WTE plants integrated into microgrids can operate independently or in coordination with the larger grid, providing reliable electricity during power outages or periods of peak demand. This decentralized model increases energy resilience, as microgrids are less vulnerable to widespread grid failures. By combining WTE technologies with storage, renewable sources, and digital management systems, urban districts can create self-sustaining energy systems that reduce dependency on the central grid and improve energy security for communities.
Despite the many advancements in technology and the growing importance of small-scale WTE plants, regulatory and community acceptance remain critical challenges. Local governments often need to balance waste-to-energy projects with public concerns about air quality and waste management. Effective policy, clear regulatory frameworks, and ongoing community engagement are key to ensuring the success of these projects. Public education and transparent communication about the environmental benefits, safety measures, and technological improvements in WTE facilities can help build trust and support for these systems in urban districts.
In conclusion, small-scale landfill waste-to-energy plants will continue to play an essential role in urban energy systems as cities move toward greater sustainability, waste reduction, and energy resilience. With advances in fuel flexibility, efficiency, hybrid technologies, and carbon capture, these plants offer an attractive solution for turning waste into a valuable energy resource. As technological innovations evolve and regulatory frameworks support their growth, small-scale WTE plants will become an even more integral part of the global transition to cleaner, more resilient urban energy systems.
As the demand for sustainable energy and waste management solutions continues to grow, small-scale landfill waste-to-energy (WTE) plants are positioned to play an increasingly important role in the future energy landscape. These plants provide an effective means to manage municipal solid waste (MSW) while also addressing power reliability challenges faced by urban districts. With the combination of waste reduction, renewable energy generation, and reduced environmental impact, small-scale WTE facilities are emerging as a key component of a circular economy.
Further advancements in technology are likely to refine the efficiency and performance of small-scale landfill WTE plants. For example, advanced combustion technologies such as catalytic combustion or oxygen-enriched combustion are gaining traction in small-scale plants. These technologies help achieve more complete combustion of waste materials at lower temperatures, resulting in fewer pollutants and higher energy output. Oxygen-enriched combustion, in particular, enhances the combustion process by increasing the oxygen content in the furnace, allowing for more efficient energy recovery and reducing the formation of nitrogen oxides (NOx), which are a common pollutant in conventional incineration.
Waste sorting and pre-processing technologies are also evolving to improve feedstock quality and efficiency in small-scale WTE plants. By better sorting waste materials before they enter the processing phase, plants can ensure that only the most suitable materials, such as high-calorific-value waste, are burned or gasified. This can significantly improve energy efficiency while reducing the environmental impact. Advanced sensor systems and AI-powered sorting algorithms can automate this process, increasing the accuracy and speed of waste separation, as well as identifying potentially harmful or hazardous materials before they reach the processing stage.
Another emerging trend is the use of biological waste-to-energy (bio-WTE) technologies, which harness the natural decomposition process of organic waste to generate energy. Anaerobic digestion is one of the most well-known methods, in which organic waste is broken down by microorganisms in an oxygen-free environment to produce biogas (primarily composed of methane). This biogas can then be used for electricity generation in gas engines or turbines, providing a cleaner alternative to traditional incineration. The beauty of anaerobic digestion is that it not only produces energy but also produces valuable by-products such as digestate, which can be used as a fertilizer or soil conditioner. Small-scale bio-WTE systems are particularly suited for urban districts with significant organic waste fractions, such as food scraps or yard waste, and could help cities reach their sustainability goals while reducing methane emissions from landfills.
Distributed generation and modular WTE systems are becoming increasingly important as urban energy needs shift toward decentralized, localized power sources. Traditional large-scale WTE plants often face logistical challenges related to waste transportation, land availability, and high initial capital investment. Small-scale, modular WTE systems, however, are more adaptable and can be deployed in urban environments where land is limited and waste streams are more localized. These modular systems can be customized to fit the specific needs of an urban area, taking into account the volume and composition of waste, as well as local energy demand. The flexibility of these systems makes them well-suited for integration into urban microgrids, where multiple small-scale WTE plants work in conjunction with other distributed energy resources, such as solar, wind, and energy storage.
Energy storage technologies will also play a critical role in the future of small-scale landfill WTE plants. These technologies allow excess energy generated by WTE plants to be stored and used when demand is high or when the plant is not producing energy due to fluctuating waste supply. Batteries, pumped hydro storage, and thermal energy storage systems are just some examples of energy storage solutions that can be integrated with small-scale WTE plants to provide greater energy reliability and grid stability. For instance, thermal storage systems could store the excess heat generated during periods of low demand, which can then be used for electricity generation or district heating during peak demand times.
Hybrid systems that combine small-scale landfill WTE plants with other renewable energy technologies such as solar thermal and geothermal are increasingly being explored to provide a continuous, stable energy supply. In a hybrid WTE-solar thermal system, solar energy can supplement the waste-based energy generation by providing additional heat to the steam turbines, improving efficiency. Similarly, geothermal energy can be used to provide a base load supply of energy, supporting the WTE plant when waste generation or availability is low. This combination of renewable energy sources ensures that small-scale WTE systems are capable of delivering a more reliable and consistent power supply while contributing to carbon reduction efforts.
The future of small-scale landfill WTE plants also looks promising in terms of digitalization and smart grid integration. By leveraging Internet of Things (IoT) devices, big data analytics, and artificial intelligence (AI), WTE plants will be able to optimize operations and minimize waste in real-time. IoT sensors can monitor key parameters such as temperature, pressure, and gas composition, sending this data to centralized control systems where AI algorithms analyze it to make predictive adjustments to plant operations. This allows for better decision-making, improved energy efficiency, and reduced operational costs. Moreover, the integration of WTE plants with smart grids enables more dynamic and flexible energy distribution, where the energy generated from WTE systems can be distributed to the grid or used locally as needed, depending on real-time demand and supply conditions.
Policy and regulatory frameworks will continue to shape the development and deployment of small-scale WTE plants. Governments around the world are implementing stricter regulations on waste management, emissions, and carbon reduction. To remain competitive and meet these evolving standards, small-scale WTE plants will need to continually innovate and adopt cleaner, more efficient technologies. Incentive programs, carbon credits, and renewable energy standards may provide financial support to accelerate the deployment of small-scale WTE systems, particularly in regions where waste management is a significant challenge.
In conclusion, small-scale landfill waste-to-energy plants offer a versatile and sustainable solution for urban districts, addressing both waste management and energy reliability issues. With advancements in technology, regulatory support, and integration into the broader energy system, these plants are poised to play a critical role in the transition to cleaner, more resilient urban energy infrastructures. As cities continue to embrace circular economy principles and seek ways to minimize their environmental footprint, the role of small-scale landfill WTE systems will only become more essential, ensuring that urban waste becomes a valuable resource for sustainable energy production.
Small Scale Power Plant for dairy farms
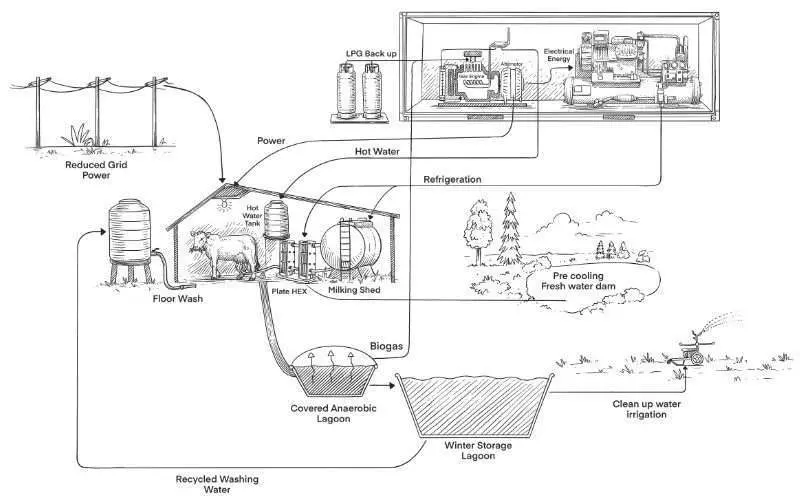
Small-scale power plants for dairy farms are emerging as an innovative solution to address both energy consumption and waste management challenges in the agricultural sector. Dairy farms, like many other agricultural operations, require a substantial amount of energy to run equipment such as milking machines, refrigeration systems, heating systems, and lighting. However, they also produce significant amounts of organic waste, including manure, which presents an opportunity to generate renewable energy locally and reduce operational costs.
One of the most effective ways small-scale power plants are being integrated into dairy farms is through biogas production from anaerobic digestion of manure and other organic farm waste. In this process, manure is collected and placed in an anaerobic digester, where bacteria break down the organic material in an oxygen-free environment, producing biogas—a mixture of methane and carbon dioxide. This biogas can be used to fuel combined heat and power (CHP) systems, providing both electricity and heat for the farm.
The biogas-powered CHP system typically includes a gas engine or turbine that converts the biogas into electricity, which can either be used on-site to power farm operations or sold back to the local grid. The heat generated during electricity production can be used for various farm needs, such as heating barns or water, thus reducing the need for external energy sources. This approach can help dairy farms become more self-sufficient in terms of energy, significantly lowering their dependence on the grid and providing a reliable, renewable energy source for daily operations.
In addition to biogas, small-scale power plants on dairy farms can also utilize solar energy or wind energy. By installing solar panels on barn roofs or in open areas, dairy farms can capture sunlight and convert it into electricity, which can supplement or even entirely offset their energy needs. Wind turbines, particularly in rural areas with consistent wind patterns, can also provide a viable renewable energy source to power the farm’s operations.
Small-scale biomass power plants can be another useful option for dairy farms, especially when combined with agricultural residues, such as leftover crop material or feedstock. Biomass can be burned or converted into gas to produce heat and electricity, helping dairy farms to manage waste while simultaneously generating power. Some farms also use digestate, the solid byproduct from anaerobic digestion, as a fertilizer or soil amendment, reducing the need for synthetic fertilizers and contributing to sustainable farming practices.
One significant benefit of small-scale power plants on dairy farms is that they can help to mitigate the environmental impact of manure and other waste products. Manure left untreated can release methane—a potent greenhouse gas—into the atmosphere, but by capturing this methane for energy production, dairy farms can reduce their carbon footprint while simultaneously improving waste management. Biogas production also minimizes the risk of water and soil pollution that can result from poorly managed manure disposal practices.
Small-scale power plants on dairy farms can also improve economic resilience. By generating their own renewable energy, dairy farms can lower their energy bills, which often represent a significant portion of operating costs. Additionally, excess energy can be sold back to the grid, providing a potential revenue stream and enhancing the farm’s profitability. In areas with feed-in tariffs or other incentive programs for renewable energy, small-scale power plants can provide dairy farms with a financial boost.
Another important aspect is the role these power plants can play in energy security for rural areas. Many dairy farms are located in regions that may be prone to power outages or unreliable grid access, making a local power generation solution even more critical. A small-scale power plant ensures that the farm can continue operations even during grid disruptions, providing uninterrupted service for milking, refrigeration, and other essential tasks that are time-sensitive and need a continuous power supply.
The integration of renewable energy technologies on dairy farms can also play an important part in enhancing sustainability. These systems reduce the reliance on fossil fuels and external energy sources, which contribute to air pollution and environmental degradation. Additionally, the holistic approach of using organic waste (manure) to produce energy can significantly contribute to a farm’s environmental footprint reduction, improving the overall sustainability of the dairy industry.
Despite the many advantages, there are some challenges and considerations when implementing small-scale power plants on dairy farms. The initial capital investment required for the installation of biogas digesters, solar panels, or wind turbines can be significant. However, various subsidies, grants, and financing options are available to assist farms in making these investments, especially in regions where renewable energy adoption is being actively promoted. Furthermore, the operation and maintenance of the equipment require ongoing effort and cost, including managing the anaerobic digestion process and ensuring that renewable energy systems are functioning efficiently.
The scalability of small-scale power plants is also an important factor to consider. Farms may need a power system that can adapt as their energy needs evolve. The installation of modular systems, where additional biogas or renewable energy capacity can be added as the farm grows, can provide the necessary flexibility to meet future demands.
Additionally, the regulatory environment for renewable energy generation may vary by region, and dairy farms need to ensure they comply with local regulations and permitting requirements when installing and operating their power plants. Regulations related to waste management, energy production, and emissions must all be carefully considered to ensure the farm’s operations remain in compliance.
Looking ahead, the integration of advanced energy storage systems could further enhance the efficiency and reliability of small-scale power plants on dairy farms. By using batteries or thermal energy storage systems, excess energy generated during periods of high production (e.g., sunny days for solar or windy days for wind energy) can be stored for use during periods of lower energy production or higher demand. This would make renewable energy systems even more reliable and reduce the farm’s reliance on the grid.
In conclusion, small-scale power plants on dairy farms present a promising solution to both energy and waste management challenges in the agricultural sector. By utilizing biogas, solar, wind, or biomass energy, dairy farms can improve their sustainability, reduce their environmental footprint, and achieve energy self-sufficiency. With the right support and technological innovations, these systems can provide dairy farms with the resilience and efficiency needed to thrive in a changing energy landscape. The ongoing development of renewable energy technologies, coupled with supportive policies and financial incentives, will continue to make small-scale power plants an attractive and economically viable option for dairy farms seeking to embrace sustainable energy practices.
Small-scale power plants for dairy farms not only offer energy independence but also enhance the economic viability and environmental sustainability of farm operations. These plants provide a practical solution for the growing demand for sustainable practices within agriculture, particularly in dairy farming, where energy costs can constitute a significant portion of the operational expenses. The ability to generate renewable energy on-site helps mitigate the volatility of energy prices, contributing to more stable financial planning and improving overall cost efficiency.
The adoption of biogas systems remains one of the most promising methods for generating energy on dairy farms, as it directly addresses the challenge of managing manure waste while also producing a valuable resource—energy. Anaerobic digestion systems can process manure from dairy cows, along with other organic waste materials such as crop residues or food waste, to produce biogas. This process not only helps manage the environmental issues associated with manure, such as odors and methane emissions but also recycles valuable nutrients back into the farm’s operations. The digestate that remains after the digestion process is rich in organic matter and can be used as a nutrient-rich fertilizer for crops, reducing the need for chemical fertilizers and promoting soil health.
Biogas-powered CHP systems on dairy farms can be configured to meet the farm’s specific energy needs. In addition to producing electricity, the heat generated during electricity production can be captured and used for heating barns, water systems, or milking parlors, making it a dual-purpose energy solution. This heat utilization is particularly valuable in colder climates where dairy operations require constant heating for both animal welfare and operational needs, reducing the demand for additional heating sources and helping to lower utility bills.
Beyond biogas, solar energy continues to be a growing option for dairy farms that have access to ample sunlight. Solar panels can be installed on barn roofs, open fields, or other available land areas to generate electricity during the day. Dairy farms can use this solar energy to meet their daily electricity demand, reducing reliance on the grid. Solar energy can be particularly effective when combined with battery storage systems, allowing the farm to store excess energy produced during sunny periods and use it during the night or cloudy days when solar generation is limited. This combination makes solar energy a flexible and reliable power source for small-scale dairy farms.
Similarly, wind power can play a complementary role, particularly for farms located in areas with consistent wind patterns. Small-scale wind turbines can harness the energy of the wind to generate electricity, contributing to the farm’s energy portfolio and providing a renewable energy source to offset grid reliance. While wind power may not always be as consistent as solar energy, in combination with biogas or solar power, it can contribute to a more diversified and reliable renewable energy solution.
Small-scale power plants for dairy farms also offer a potential avenue for carbon footprint reduction and sustainability. Dairy farms are often under pressure to reduce their greenhouse gas emissions, particularly methane, which is produced during the digestive process of cows and during manure decomposition. The conversion of methane into biogas for energy production reduces its release into the atmosphere and prevents it from contributing to global warming. Furthermore, by relying on renewable energy sources and reducing their dependency on fossil fuels, dairy farms can significantly lower their carbon emissions, meeting both regulatory requirements and environmental stewardship goals.
Moreover, small-scale power plants can enhance the resilience of dairy operations to external shocks such as grid outages, fuel price fluctuations, or extreme weather events. In rural areas, where power outages are more common, having an independent source of energy can ensure that critical operations—such as milking, refrigeration, and animal heating—continue uninterrupted. This resilience is increasingly important as climate change brings more frequent and severe weather events that could affect grid reliability, especially in agricultural areas.
The integration of smart technologies can further optimize the performance of small-scale power plants. IoT devices and smart meters can provide real-time monitoring of energy generation, consumption, and storage, allowing farm managers to adjust operations for maximum efficiency. AI-driven systems can predict energy needs, automatically adjusting energy production or usage to ensure that the farm runs smoothly and that energy is utilized optimally. For example, if the biogas plant produces more energy than needed during a certain period, the excess can be stored or sold back to the grid.
One of the challenges that dairy farms may face when adopting small-scale power plants is the initial investment required for infrastructure. However, with the availability of government incentives, subsidies, and financing options, many farms can offset the upfront costs. Incentive programs for renewable energy adoption, such as feed-in tariffs, tax credits, and low-interest loans, can help make the transition to renewable energy more affordable. Additionally, the operational savings and potential revenue from selling surplus energy back to the grid can offer a return on investment (ROI) that justifies the initial capital expenditure over time.
Another important consideration is the scalability of these systems. Small-scale power plants can be designed to match the energy needs of a farm, but as farms grow or energy demands change, the systems must be flexible enough to scale. The modular nature of many renewable energy technologies—such as biogas digesters, solar panels, and wind turbines—allows for gradual expansion, meaning that farms can increase their energy production capacity over time without needing to invest in entirely new systems. This scalability helps ensure that small-scale power plants remain a viable option as farms evolve.
Finally, community benefits should not be overlooked when considering small-scale power plants on dairy farms. These systems can create local jobs related to the construction, operation, and maintenance of renewable energy systems. Additionally, when farms generate surplus energy, they may have the opportunity to sell it back to the grid, benefiting the local power supply and contributing to the wider adoption of renewable energy in the region. By embracing renewable energy, dairy farms can be seen as leaders in sustainability, promoting a circular economy where waste is transformed into a valuable resource.
In conclusion, small-scale power plants on dairy farms present a comprehensive and sustainable solution to energy and waste management challenges in the agricultural sector. By utilizing renewable energy sources such as biogas, solar, and wind, dairy farms can reduce their energy costs, improve environmental sustainability, and increase resilience. With ongoing technological advancements, supportive policies, and innovative financing options, small-scale power plants will continue to offer dairy farmers a practical and economically viable path toward energy independence and sustainability. As the agricultural industry embraces renewable energy solutions, dairy farms will play a critical role in contributing to a more sustainable and resilient food production system.
As small-scale power plants on dairy farms evolve, the broader agricultural landscape is likely to witness a transformation in energy practices, with farms of all sizes increasingly adopting sustainable energy solutions. The integration of smart grid technologies and advanced renewable energy storage systems will play a critical role in this transformation, further enhancing the operational efficiency and energy security of dairy farms.
One of the next frontiers in optimizing small-scale power plants is the use of energy storage technologies. As mentioned earlier, battery storage systems offer the potential to store excess energy produced during periods of high renewable generation, whether from biogas, solar, or wind. Dairy farms can take advantage of this stored energy during peak demand periods or when energy production drops due to unfavorable weather conditions. In addition to battery storage, thermal energy storage can be utilized, where excess heat from the biogas-powered CHP systems or other renewable technologies can be stored and used for heating purposes, such as warming barns or water used for milking. This ensures that dairy farms can continue to operate efficiently even during periods of low energy generation.
Another development likely to emerge is the rise of hybrid power systems that combine multiple renewable energy sources to optimize energy production and supply. For example, biogas and solar hybrid systems could provide complementary energy. During sunny periods, solar energy can supplement the biogas generation, and during cloudy or nighttime hours, the biogas plant would take over. Similarly, wind and biogas hybrids could be implemented in regions with good wind resources, allowing the farm to diversify its energy mix and reduce dependency on a single renewable source. This hybrid approach improves the reliability and stability of the energy supply and can ensure that the farm has a consistent, renewable power source year-round.
The implementation of energy management systems (EMS) will also become increasingly important. These systems integrate all the farm’s energy resources, including renewable energy, storage, and traditional energy sources, into a centralized control system. The EMS monitors and manages energy flow, ensuring that energy production and consumption are balanced and optimized. For example, if the biogas system is producing excess electricity, the EMS can direct that energy to storage or the grid, or alternatively, use the excess energy to meet non-essential farm needs, such as charging electric vehicles or powering auxiliary equipment. This system helps to avoid wastage, lower energy costs, and ensure that energy is used as efficiently as possible.
In addition to energy systems, sustainable farming practices will continue to evolve in parallel. As the focus on renewable energy increases, there will likely be a greater integration of precision agriculture technologies, such as smart sensors and AI-driven analytics to monitor soil health, water usage, and crop growth. This will complement the energy strategies by ensuring that farms not only generate their own energy but also use resources more efficiently, minimizing water waste, reducing the need for fertilizers, and enhancing crop yields.
Small-scale power plants for dairy farms also align well with the increasing demand for sustainability certification. As consumers become more aware of the environmental impact of food production, many dairy farms are exploring certification programs that recognize sustainable practices. By adopting renewable energy technologies, dairy farms can improve their environmental credentials, enhancing their appeal to environmentally conscious consumers. Eco-labels and sustainability certifications can become a key marketing tool for dairy producers, differentiating their products in the marketplace and increasing consumer trust.
As the technology surrounding renewable energy systems continues to improve, cost reductions are expected across the board. Biogas digesters, solar panels, wind turbines, and energy storage systems are becoming increasingly affordable, allowing more dairy farms to access these technologies. In addition, the ongoing development of more efficient biogas production processes, lower-cost storage options, and innovative financing mechanisms (e.g., pay-as-you-go solar or green bonds) will make small-scale power plants even more economically accessible.
Microgrid systems—which allow a farm to operate independently or in conjunction with the grid—are also likely to become more common. A microgrid enables dairy farms to generate and manage their own electricity, ensuring that they can operate autonomously during power outages or supply disruptions. Microgrids can be equipped with energy storage systems and flexible controls to integrate renewable energy, providing the farm with not only energy security but also greater autonomy and cost savings.
Further advancements in biogas technology are expected to enhance the efficiency of biogas production on dairy farms. New developments in high-temperature anaerobic digestion and the use of co-digestion, where additional organic waste such as food scraps, agricultural residues, or other farm by-products are added to the digester, will improve gas yield and overall system efficiency. These innovations will help dairy farms maximize energy production while also providing enhanced waste management solutions. Furthermore, the growth of biogas-to-grid technologies, where biogas is converted into high-quality biomethane that can be injected into the natural gas grid, could offer dairy farms an additional revenue stream, allowing them to sell excess energy or gas.
On the regulatory front, policy incentives and subsidies for renewable energy adoption will continue to evolve. Governments around the world are increasingly focused on achieving carbon reduction targets, and many have implemented programs designed to support sustainable energy transitions in agriculture. Dairy farms adopting small-scale power plants can take advantage of these programs to help defray the upfront costs of installation. In regions where carbon trading or carbon credit markets are in place, dairy farms may also be eligible to participate, earning credits for reducing methane emissions through biogas systems or contributing to overall greenhouse gas reductions by using renewable energy.
Finally, collaboration between agricultural stakeholders, energy providers, and technology developers will be essential for the continued success of small-scale power plants on dairy farms. Partnerships between local utilities and dairy farmers could facilitate the development of community-based energy networks or energy cooperatives. This approach could help streamline the process of integrating small-scale renewable energy systems, making it easier for farms to access funding, technical expertise, and regulatory support.
In conclusion, the future of small-scale power plants on dairy farms looks increasingly bright as technologies advance, costs decrease, and regulations continue to support renewable energy adoption. The combination of biogas, solar, wind, and energy storage technologies, along with smart grid systems and hybrid solutions, will provide dairy farmers with a reliable, cost-effective, and sustainable way to meet their energy needs. With the right support from policy, financing, and technology, small-scale power plants will not only help dairy farms become more self-sufficient but will also make them leaders in the sustainable agricultural movement, contributing to a cleaner, more resilient energy future.
As small-scale power plants for dairy farms continue to evolve and gain traction, the potential for scalability and replicability will play a crucial role in expanding their adoption beyond individual farms. The concept of decentralized energy production, where energy is generated locally and consumed within the community, is gaining momentum as a model for sustainable farming. This model could transform rural areas, where many dairy farms are located, into energy hubs, supporting the broader adoption of renewable energy technologies and reducing reliance on centralized power grids.
Community-Based Energy Solutions
One of the key aspects of this transformation is the potential for community-based renewable energy projects, where multiple dairy farms and other local agricultural operations share the benefits of small-scale power plants. For instance, a cluster of dairy farms could install a cooperative biogas plant that processes manure and organic waste from several farms, producing biogas that is used to generate electricity and heat. The electricity produced could be distributed among the farms in the cooperative, helping each participant reduce their energy costs and improve their sustainability. The collective approach also spreads the upfront costs of renewable energy infrastructure, making it more affordable for individual farms.
Additionally, these community-based projects could integrate with larger local energy systems, such as microgrids or community-owned power plants. Microgrids are localized energy systems that can operate independently from the main grid or be connected to it, providing increased energy security and reliability. By pooling resources, dairy farms and neighboring farms could take advantage of shared renewable energy generation, storage solutions, and smart grid technologies that improve efficiency and lower costs.
In this scenario, the renewable energy produced could also be sold to local utilities or consumers, creating new economic opportunities for dairy farmers. If the power plant generates more energy than needed for farm operations, the surplus could be exported to the local grid, contributing to the broader renewable energy supply in the region. This not only generates additional income but also promotes the localization of energy markets, reducing transmission losses and enhancing energy resilience at the community level.
Technological Innovation and Integration
Technological innovation will be a driving force in making small-scale power plants even more efficient, cost-effective, and adaptable to the needs of dairy farms. As the demand for renewable energy grows, new breakthroughs in energy storage, energy conversion, and waste-to-energy technologies will further improve the feasibility of small-scale power plants.
For example, biogas production is likely to become more efficient with the development of advanced anaerobic digestion technologies. High-efficiency digesters can process manure and organic waste faster and produce more biogas, while modular biogas systems offer flexibility in scaling up capacity as the energy needs of a farm grow. The integration of AI and machine learning into biogas plants could optimize the anaerobic digestion process by continuously adjusting parameters such as temperature, feedstock composition, and microbial activity to maximize gas production. In addition, biogas purification technologies will enable the conversion of biogas into biomethane, a cleaner and more versatile form of natural gas that can be injected into the national grid or used for vehicle fuel.
Solar panel efficiency is also improving steadily. New technologies, such as bifacial solar panels (which can capture sunlight on both the front and back sides), perovskite solar cells, and thin-film solar modules, are expected to drive down the cost of solar energy production, making it even more accessible for small-scale dairy farms. As solar energy becomes cheaper and more efficient, the return on investment for solar panel installations will improve, allowing farmers to recoup their costs more quickly and invest in other renewable energy technologies.
Wind energy will also benefit from ongoing advancements. Small-scale wind turbines are becoming more efficient and cost-effective, with designs that are better suited to low-wind-speed environments—making wind a viable option for farms that might not have been able to utilize it before. As turbine technology improves, the energy generation capacity of small-scale wind systems will increase, helping to meet the farm’s energy needs and providing another source of renewable power.
Moreover, the integration of energy storage systems, particularly advanced battery technologies, will address one of the major challenges of renewable energy generation—intermittency. Lithium-ion batteries, solid-state batteries, and flow batteries are all advancing rapidly in terms of efficiency, cost, and lifespan. These energy storage systems will allow dairy farms to store excess power produced by solar, wind, or biogas systems, and use it during periods of low generation or high demand, ensuring a continuous and reliable energy supply.
Policy and Regulatory Landscape
The regulatory landscape will be key to accelerating the adoption of small-scale power plants on dairy farms. Government incentives and subsidy programs are already playing a significant role in reducing the financial burden of installing renewable energy systems. However, as renewable energy technologies continue to improve, policymakers must create more comprehensive long-term frameworks that ensure the ongoing viability of these systems.
This could include expanding the availability of financial incentives for renewable energy adoption, such as tax credits, grants, and low-interest loans. Programs that reward farms for reducing emissions—such as carbon credits or participation in carbon markets—could provide additional income streams for farms with biogas or other renewable energy systems. By expanding incentives for renewable energy adoption and waste-to-energy technologies, governments can help dairy farms achieve greater energy independence while also meeting their environmental goals.
Furthermore, regulations that promote energy grid integration will enable dairy farms to connect their small-scale power plants to local grids more easily. This could include simplifying the process for selling excess energy back to the grid through net metering or other compensation programs, and ensuring that the grid can accommodate the distributed generation of renewable energy.
Another important regulatory consideration is the creation of policies that support biogas production and waste management on farms. Regulations that make it easier to capture and utilize methane emissions from dairy farm operations could further incentivize the adoption of biogas systems. By supporting the development of biogas infrastructure and reducing barriers to entry, policymakers can encourage more dairy farms to participate in the renewable energy transition.
Consumer Demand and Market Trends
Increasing consumer demand for sustainably produced food is another powerful force driving the adoption of renewable energy technologies on dairy farms. As awareness of the environmental impact of food production grows, more consumers are seeking products from farms that prioritize sustainable practices. Dairy farms that invest in renewable energy can differentiate themselves in the market by promoting their environmental stewardship and commitment to sustainability. This is especially true for consumers who are willing to pay a premium for eco-friendly products.
In addition, the rise of plant-based alternatives to dairy products may prompt traditional dairy farms to reimagine their operations in order to stay competitive. By incorporating renewable energy systems and other sustainable farming practices, dairy farms can improve their sustainability profile and appeal to both traditional consumers and those seeking greener alternatives. The ability to market energy-efficient operations and environmentally friendly practices can strengthen a farm’s brand and improve its marketability.
The Future of Small-Scale Power Plants on Dairy Farms
In the coming years, small-scale power plants on dairy farms will likely become a common feature across the agricultural landscape. As renewable energy technologies become more affordable, efficient, and scalable, dairy farms will have a greater opportunity to decarbonize their operations, reduce costs, and enhance their resilience to energy disruptions. By embracing renewable energy, dairy farmers will not only benefit economically but will also contribute to a cleaner, more sustainable food production system.
The future of dairy farming is undeniably intertwined with the transition to renewable energy. Through biogas, solar, wind, and energy storage systems, dairy farms can achieve energy independence, economic resilience, and environmental sustainability. With continued advancements in technology, regulatory support, and market demand for sustainable practices, small-scale power plants will help dairy farms lead the way toward a greener, more sustainable future for agriculture.
Small Scale Power Plant for agricultural processing plants
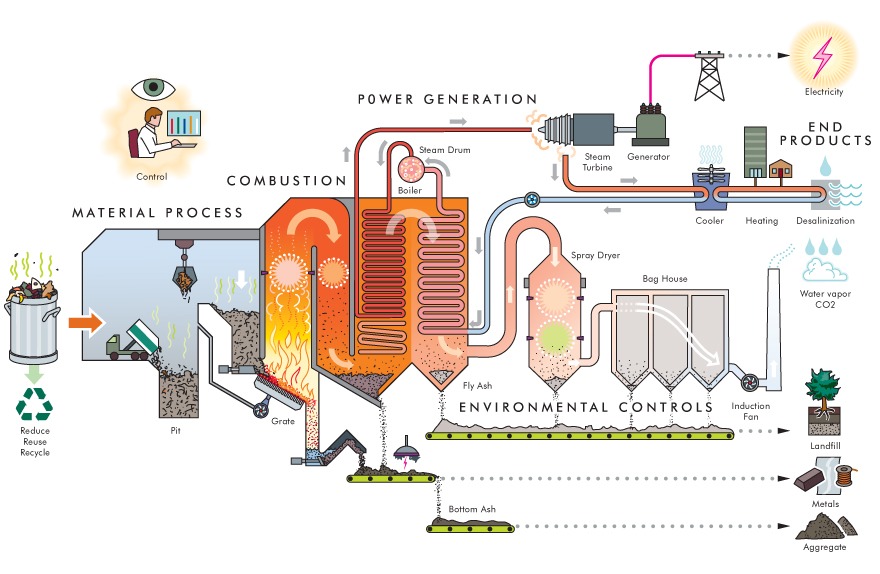
Small-scale power plants for agricultural processing plants represent a transformative opportunity for the agricultural and food processing industries. These plants are designed to harness renewable energy sources to meet the energy demands of processing facilities while simultaneously offering numerous environmental and economic benefits. As agricultural processing continues to grow globally, driven by the increasing demand for processed foods, biofuels, and other agricultural products, small-scale power plants are emerging as a strategic solution to address both energy and sustainability challenges in the industry.
Energy Demands of Agricultural Processing Plants
Agricultural processing plants typically require significant amounts of energy for various operations, including drying, grinding, milling, cooking, pasteurizing, and packaging. These energy-intensive processes often rely on traditional energy sources, such as grid electricity or fossil fuels, which can be costly, unreliable, and environmentally unsustainable. Small-scale power plants offer a way to meet the energy needs of these facilities by using renewable energy sources such as biomass, solar, wind, and biogas.
The main advantage of using small-scale power plants in agricultural processing is that they can provide localized, cost-effective, and reliable energy. These systems can help reduce energy costs, improve energy security, and contribute to greenhouse gas emissions reductions. By utilizing waste materials such as agricultural residues, organic waste, and even food processing by-products, these plants can turn what would otherwise be waste into a valuable source of energy. This process not only enhances the sustainability of the operation but also promotes the circular economy by reducing waste and utilizing renewable resources.
Biomass Energy in Agricultural Processing
One of the most promising renewable energy sources for agricultural processing plants is biomass. Biomass power plants can be fueled by agricultural residues such as crop waste, wood chips, straw, and husks, which are commonly available at agricultural processing sites. These residues are often underutilized or disposed of in ways that contribute to environmental pollution, such as open burning or landfill disposal. By converting these waste materials into energy through processes like combustion, gasification, or pyrolysis, small-scale biomass power plants can produce heat and electricity that can be used to power plant operations.
For example, rice mills generate large amounts of rice husks, and sugar mills produce bagasse (the fibrous remains of sugarcane stalks after juice extraction). These by-products can be converted into energy using biomass boilers or gasifiers to generate electricity and heat for the mill’s operations. Not only does this reduce the plant’s reliance on fossil fuels, but it also helps to manage waste products in a more environmentally friendly way. Biomass-based small-scale power plants are particularly beneficial for agricultural processing plants located in rural areas, where access to grid power can be limited or unreliable.
Biomass power plants are also an excellent solution for industries with a large amount of organic waste, such as food processing. Wastes from fruit and vegetable processing, meat processing, or dairy plants can be converted into biogas using anaerobic digestion. This technology breaks down organic waste in an oxygen-free environment, producing methane-rich biogas that can be burned to generate electricity or heat. The digestate produced can then be used as a nutrient-rich fertilizer, closing the loop on waste management and providing additional benefits for the agricultural community.
Solar and Wind Power for Agricultural Processing
In addition to biomass, solar energy is becoming an increasingly popular option for agricultural processing plants. Solar panels can be installed on the roofs of processing buildings, warehouses, and open land areas to generate electricity. Solar energy is especially effective for providing daytime power, helping to offset energy costs during peak production hours. While solar power is intermittent (i.e., it only generates electricity during daylight hours), it can be paired with battery storage systems to store excess energy for use during non-sunny periods, such as nighttime or cloudy days.
For agricultural processing plants that operate in areas with consistent sunlight, solar panels can be a key component of a small-scale power plant. The energy generated can be used for a wide range of operations, such as powering machinery, lighting, ventilation systems, and cooling equipment. Solar energy is especially effective in agricultural settings where there is often a large amount of unused roof space, which can be put to good use for energy generation without taking up valuable land.
Similarly, wind energy can complement solar and biomass energy systems. For agricultural processing plants located in windy areas, small-scale wind turbines can generate electricity, which can either be used on-site or stored for later use. Wind power is particularly useful when combined with other renewable sources to ensure a consistent energy supply. Wind energy works well alongside biomass or biogas systems, where the intermittent nature of one source can be balanced by the steady output of another.
Energy Storage and Efficiency Optimization
One of the key considerations for small-scale power plants in agricultural processing is ensuring the efficient integration of various energy sources, particularly in regions where renewable generation may be intermittent or unpredictable. Energy storage systems—such as batteries or thermal storage—play a critical role in enhancing the reliability and efficiency of renewable energy solutions.
In biomass or biogas systems, for example, the energy produced in the form of heat can be stored in thermal storage tanks for later use, especially during periods when the plant’s energy demand is lower. This stored heat can be used for drying agricultural products or steam generation for cooking or pasteurization. Similarly, battery storage systems can store excess electricity generated by solar or wind systems during peak production periods and release it during periods of high demand or low renewable generation.
The integration of smart grid and energy management systems (EMS) is also important for optimizing energy consumption. These systems can continuously monitor energy production and consumption, allowing the agricultural processing plant to adjust its operations accordingly. For instance, if there is a surplus of renewable energy, the EMS can automatically direct it to storage or export it to the grid, reducing the plant’s reliance on external power sources and maximizing its renewable energy utilization. If there is a shortfall in energy generation, the system can draw power from the grid or switch to backup fossil fuel generators. This real-time monitoring and adjustment help ensure a balance between energy production, storage, and consumption.
Economic Benefits and Sustainability
The implementation of small-scale power plants in agricultural processing offers significant economic benefits to both the plants and the surrounding communities. By investing in renewable energy systems, processing plants can reduce their reliance on external power sources and stabilize their energy costs. The long-term savings on energy bills can make a significant difference in the profitability of the plant, especially in energy-intensive industries such as food processing and textile manufacturing.
Additionally, the use of locally sourced renewable energy can foster greater energy security and reduce the impact of fluctuating energy prices. For agricultural processing plants located in rural areas, where grid power may be unreliable or costly, small-scale power plants offer a reliable and independent energy supply, reducing the risk of downtime due to power outages.
Another important economic benefit is the potential to sell excess energy back to the grid. Many countries have policies that encourage renewable energy producers to sell surplus electricity, either through feed-in tariffs or power purchase agreements (PPAs). By participating in these programs, agricultural processing plants can generate additional revenue streams, further improving their financial sustainability.
Beyond cost savings and revenue generation, small-scale power plants contribute to the environmental sustainability of agricultural processing. By utilizing renewable energy sources, these plants help reduce carbon emissions, decrease reliance on fossil fuels, and minimize the environmental impact of waste disposal. Moreover, the integration of circular economy principles—where waste is converted into a valuable resource—promotes more sustainable practices within the agricultural sector.
Conclusion
Small-scale power plants for agricultural processing plants are an increasingly viable and sustainable solution for meeting energy demands while contributing to environmental goals. By utilizing biomass, solar, wind, and biogas energy sources, these plants help reduce waste, lower energy costs, and enhance the resilience of agricultural processing facilities. The ability to generate energy locally and efficiently supports economic viability, energy security, and sustainability, making small-scale power plants an essential component of the future of agricultural processing.
As renewable energy technologies continue to evolve, these systems will become even more affordable, efficient, and adaptable, ensuring that agricultural processing plants can transition to a more sustainable and self-sufficient energy future. Through the continued adoption of small-scale power plants, the agricultural processing industry can play a critical role in promoting sustainability, reducing its carbon footprint, and contributing to a more resilient and circular economy.
The integration of small-scale power plants into agricultural processing facilities is a crucial step in making the industry more sustainable and resilient. As renewable energy technologies advance, these systems are increasingly capable of addressing the energy demands of diverse agricultural processing sectors. This shift towards localized, renewable energy production offers an array of benefits that go beyond cost savings and waste reduction, with broader implications for local communities, the environment, and the agricultural economy as a whole.
Decentralized Energy Systems for Agricultural Processing
One of the key advantages of small-scale power plants is their decentralized nature. Unlike large, centralized power stations, which require extensive infrastructure and long-distance transmission, small-scale power plants are located on-site, closer to the energy source and the area of consumption. This localization of power generation offers a number of important benefits for agricultural processing plants, particularly in remote or rural areas where grid access can be unreliable or expensive.
In rural settings, agricultural processing plants are often located far from the main energy grid, which can result in high transmission losses, limited grid access, and increased energy costs. Small-scale power plants mitigate these issues by providing a self-sufficient energy solution that eliminates the need for long-distance transmission. As such, energy costs are reduced, and the risk of power disruptions—such as grid failures or blackouts—is minimized, ensuring consistent power availability for critical processing operations. Furthermore, localized energy production promotes energy security, enabling agricultural processing plants to become more resilient in the face of external disruptions.
This energy independence is especially valuable during peak processing periods when energy demands are at their highest. For example, during harvest seasons, when agricultural products are being processed in large volumes, a steady and reliable energy supply is crucial. By using small-scale power plants, agricultural processors can ensure that they have the energy they need without relying on external grid sources, which may be overburdened or inefficient at times of high demand.
Technological Innovations in Renewable Energy Systems
As renewable energy technologies continue to evolve, small-scale power plants in agricultural processing facilities are becoming more efficient and adaptable. One of the most promising innovations is the integration of hybrid systems that combine multiple renewable energy sources—such as biomass, solar, wind, and energy storage—to create a reliable, 24/7 energy supply. These hybrid systems allow agricultural processing plants to optimize energy production, storage, and consumption based on the availability of each renewable source.
For example, during the daytime, solar panels can generate electricity, while excess energy can be stored in batteries or used to power biomass or biogas systems. When the sun sets or energy demand spikes, the stored energy or biomass-based generation can provide the necessary power to keep the plant running. Hybrid systems enhance grid resilience and improve the overall efficiency of small-scale power plants by ensuring that renewable energy is available even during periods when one source may not be generating power.
Technological advancements in energy storage are also playing a pivotal role in improving the effectiveness of small-scale power plants. The development of advanced battery storage technologies, such as solid-state batteries, flow batteries, and liquid air energy storage, are enabling more efficient energy storage and longer lifespans for storage systems. By integrating these advanced storage solutions into small-scale power plants, agricultural processing plants can store excess energy generated during periods of low demand and use it when energy generation is insufficient.
In addition, the ongoing research and development of more efficient biomass conversion technologies, such as advanced gasification and biomass-to-liquid fuels, are helping to further optimize the performance of biomass-based power plants. These innovations increase the energy output of biomass systems while reducing emissions and minimizing environmental impact.
Circular Economy and Waste Utilization
A key characteristic of small-scale power plants in agricultural processing is their ability to support the principles of the circular economy. The circular economy seeks to minimize waste, extend the lifecycle of products, and promote the reuse of materials. Agricultural processing plants often produce significant amounts of organic waste, such as fruit and vegetable peels, seeds, stems, and animal by-products, all of which can be utilized as inputs for renewable energy production.
By using biomass, biogas, or anaerobic digestion technologies, agricultural processing plants can convert these waste materials into valuable energy. For example, waste from fruit processing—including peels, pulp, and cores—can be used to produce biogas, which can then be used for electricity generation. Similarly, animal waste from meat processing plants can be treated using anaerobic digesters to produce biogas, which can be used to power the facility or even heat water for processing.
This waste-to-energy approach not only reduces the environmental impact of agricultural processing but also helps to close the loop on waste management. The use of organic waste as a feedstock for energy production eliminates the need for disposal methods such as landfilling or open burning, which can contribute to pollution and greenhouse gas emissions.
In addition to energy production, some small-scale power plants also produce valuable by-products that can be used for other purposes. For instance, the digestate from anaerobic digestion can be used as fertilizer for agricultural operations, returning nutrients to the soil and promoting sustainable farming practices. In this way, the by-products of the power generation process help to support the overall agricultural ecosystem.
Economic Viability and Job Creation
The economic viability of small-scale power plants in agricultural processing is largely driven by the cost savings from renewable energy generation, waste-to-energy technologies, and energy independence. By reducing reliance on expensive grid electricity or fossil fuels, agricultural processors can achieve long-term cost savings that improve their financial sustainability. Furthermore, the production of renewable energy can open up new revenue streams, such as selling excess energy back to the grid or participating in carbon offset programs.
Beyond financial benefits, small-scale power plants also create significant job opportunities in rural areas. The development, installation, and maintenance of renewable energy systems, such as biomass boilers, solar panels, and wind turbines, require skilled labor, which can create jobs in local communities. These jobs are especially valuable in rural areas where traditional employment opportunities may be limited. In addition, small-scale power plants contribute to local economic development by stimulating demand for local goods and services, such as feedstock for biomass systems or equipment for energy storage.
As small-scale power plants become more widespread, agricultural processing plants may also benefit from the growing global trend toward green certifications and sustainability labeling. Products from facilities that use renewable energy are increasingly viewed favorably by consumers, especially those who prioritize environmentally responsible sourcing. The ability to market products as being produced with renewable energy can enhance the competitiveness of agricultural processors and help them tap into premium markets for eco-friendly products.
Environmental Impact and Future Potential
In terms of environmental sustainability, small-scale power plants offer significant potential to reduce the carbon footprint of agricultural processing. By transitioning to renewable energy sources such as biomass, biogas, solar, and wind, agricultural processing plants can significantly reduce their greenhouse gas emissions, making a positive contribution to global climate goals. Additionally, the reduction of waste and the adoption of circular economy principles help to decrease environmental pollution and promote resource efficiency.
The potential for small-scale power plants in agricultural processing is vast. As the agricultural industry continues to embrace renewable energy, small-scale power plants can expand into new sectors and provide a wide range of solutions for food processors, textile manufacturers, and even biofuel producers. As technologies continue to improve and economies of scale are realized, small-scale power plants will become an increasingly essential part of the global transition toward a more sustainable and energy-efficient agricultural system.
In the future, agricultural processing plants may integrate advanced digital technologies, such as smart sensors, artificial intelligence, and data analytics, to further optimize energy consumption and improve efficiency. These technologies will help to predict energy demand, optimize the performance of renewable energy systems, and manage energy storage more effectively, creating a more intelligent and dynamic energy system.
Conclusion
The adoption of small-scale power plants in agricultural processing is a powerful tool for driving sustainability, energy independence, and economic growth in the agricultural sector. Through biomass, solar, wind, and waste-to-energy systems, agricultural processing plants can reduce waste, cut costs, and contribute to the overall environmental and economic resilience of local communities. As renewable energy technologies continue to evolve, small-scale power plants will become an increasingly viable solution for a wide range of agricultural industries, helping to foster a cleaner, more efficient, and sustainable future for food and agricultural processing.
The continued growth of small-scale power plants within agricultural processing plants offers immense potential for addressing the energy needs of the agricultural sector while contributing to global sustainability goals. As the industry moves toward a future with increased energy independence, decreased emissions, and better utilization of waste, several aspects of small-scale power plants stand to evolve and improve. These developments will lead to enhanced performance, economic benefits, and expanded integration into the agricultural value chain.
Advancements in Biomass and Biogas Technologies
One of the key areas where small-scale power plants are seeing innovation is in biomass and biogas technologies. These technologies are central to converting organic agricultural waste into energy, and their advancements will increase both their efficiency and affordability. Biomass systems, including advanced combustion, gasification, and pyrolysis technologies, are evolving to allow more diverse feedstocks to be processed and to produce greater amounts of usable energy. This enables agricultural processors to convert various types of waste—ranging from crop residues to wood by-products—into clean, renewable energy.
As gasification technologies improve, more complex and higher-moisture feedstocks, such as wet biomass (e.g., wet crop waste) and agricultural residues, can be processed into syngas (synthetic gas). This gas can then be burned to generate electricity or used for heating, improving the energy efficiency of the entire process. Advanced gasifiers are becoming more versatile, allowing for a greater variety of organic waste to be used as feedstock without compromising the efficiency of the system.
Similarly, biogas production through anaerobic digestion continues to improve, offering higher methane yields and more reliable system operation. New innovations in biogas upgrading also allow for the production of biomethane, a purified form of biogas that can be injected into natural gas grids or used as vehicle fuel. Agricultural processing plants that produce significant amounts of organic waste can use these systems to convert waste directly into usable energy. The digested residue (digestate) produced in biogas systems also provides a valuable by-product, which can be used as organic fertilizer for surrounding farms, closing the loop in the agricultural ecosystem.
Moreover, waste-to-energy technologies like these have significant environmental advantages. Not only do they reduce the carbon footprint of agricultural operations by avoiding the need for fossil fuels, but they also reduce the environmental impact of waste disposal practices that could otherwise result in air pollution (e.g., open burning) or water contamination (e.g., landfill runoff). In this way, small-scale power plants equipped with biomass and biogas technologies serve as an important component of sustainable agricultural systems.
Integration of Energy Storage and Smart Technologies
As renewable energy generation from sources like solar and wind tends to be intermittent, energy storage systems play a crucial role in ensuring a continuous and stable energy supply for agricultural processing plants. The integration of battery storage technologies, thermal energy storage, and even hydrogen storage into small-scale power plants will become increasingly important as the use of renewable energy grows.
For example, lithium-ion batteries and emerging technologies like flow batteries are becoming more efficient and cost-effective, providing reliable energy storage to balance supply and demand. These systems store excess electricity generated during periods of low demand or high renewable generation and then release it during peak hours or when renewable sources are not available. Similarly, thermal energy storage systems can store heat generated by biomass plants or solar thermal systems, providing consistent thermal energy for processes like drying, pasteurizing, or cooking in agricultural processing.
The integration of smart technologies, such as smart grids, energy management systems (EMS), and Internet of Things (IoT) solutions, is also transforming how small-scale power plants operate. These technologies enable real-time monitoring of energy production, storage, and consumption, optimizing energy use throughout the agricultural processing plant. For instance, a smart energy management system can automatically adjust the operation of renewable energy systems based on weather forecasts or energy demand, ensuring that the plant always has the necessary power to run efficiently. IoT-enabled sensors can track energy usage at different points in the plant, helping to pinpoint areas where energy efficiency can be improved.
By integrating these advanced technologies, small-scale power plants can maximize energy efficiency, improve cost-effectiveness, and reduce downtime. As the capabilities of smart grids and energy management systems continue to grow, agricultural processing plants will become increasingly adept at managing energy flows in a way that reduces waste and enhances overall productivity.
Policy Support and Incentives for Renewable Energy Adoption
Government policies, regulations, and incentives play a vital role in accelerating the adoption of renewable energy in agricultural processing plants. Many countries have introduced subsidies, tax incentives, and grant programs aimed at encouraging businesses to invest in clean energy technologies. This support is particularly critical for small-scale power plants, where the upfront capital cost of renewable energy systems, such as biomass boilers, solar panels, and wind turbines, can be a barrier to entry.
For instance, programs like feed-in tariffs (FiTs) and power purchase agreements (PPAs) allow agricultural processors to sell surplus renewable energy back to the grid at a guaranteed price. These programs create a financial incentive for agricultural processing plants to invest in small-scale renewable energy systems, as they offer a stable revenue stream from energy sales. Net metering policies, which allow plants to offset their energy costs by feeding excess energy back into the grid, further incentivize renewable energy adoption.
Additionally, countries and regions with aggressive climate action plans and emission reduction goals are increasingly introducing regulations that require businesses to adopt low-carbon technologies. By incentivizing or mandating the reduction of greenhouse gas emissions, governments are encouraging agricultural processing plants to adopt renewable energy systems. With ongoing policy support, renewable energy solutions for agricultural processing will become more accessible and economically attractive.
Resilience to Climate Change and Energy Supply Disruptions
As global energy markets continue to experience volatility and concerns about climate change increase, small-scale power plants offer agricultural processors a way to build resilience against energy supply disruptions. By utilizing locally sourced renewable energy, agricultural processing plants can diversify their energy supply and reduce reliance on fossil fuels and external energy sources. This diversification is particularly important for regions that are vulnerable to extreme weather events, such as droughts, floods, and storms, which can disrupt grid electricity and fossil fuel supplies.
For example, in areas prone to hurricanes or wildfires, small-scale power plants can continue to operate during grid outages, ensuring that food processing plants can maintain operations even when external energy supplies are compromised. By installing off-grid renewable energy systems that are tailored to the local environment, agricultural processing plants can safeguard their operations from disruptions that might otherwise affect their ability to meet demand.
Furthermore, small-scale power plants that utilize distributed energy generation—such as biomass, wind, or solar—contribute to more resilient energy systems that can adapt to local conditions. In regions where grid infrastructure is weak or overburdened, small-scale power generation reduces the strain on centralized systems, making local communities less vulnerable to energy shortages.
Circular Economy and Sustainable Practices
The incorporation of small-scale power plants into agricultural processing plants is a perfect example of how the agricultural industry can align itself with circular economy principles. A circular economy focuses on resource efficiency, waste minimization, and the creation of closed-loop systems where materials are continuously reused or recycled.
Small-scale power plants play a crucial role in this process by allowing agricultural processors to repurpose organic waste—such as crop residues, food processing by-products, and animal waste—into valuable energy. This not only helps reduce the amount of waste sent to landfills or incinerators but also ensures that the materials are put to productive use in a way that supports sustainable operations.
In addition to waste-to-energy technologies, agricultural processing plants can adopt other circular economy practices such as water reuse, composting, and organic fertilizer production. The integration of renewable energy systems into these practices further reinforces the sustainability of agricultural operations by decreasing reliance on external resources and minimizing negative environmental impacts.
Community Benefits and Rural Development
Small-scale power plants also have significant social and community benefits, especially in rural areas. By providing local, renewable energy, these power plants create new economic opportunities, including job creation in the fields of energy production, maintenance, and engineering. The establishment of renewable energy systems can provide stable, long-term employment opportunities for communities that may otherwise face limited job prospects.
In addition, small-scale power plants contribute to energy democratization, giving agricultural processing plants and local communities more control over their energy sources. This empowerment fosters economic independence and can help improve the quality of life in rural areas by making energy more affordable and reliable.
Furthermore, by reducing greenhouse gas emissions and minimizing the environmental impact of agricultural processing, small-scale power plants help improve air quality, soil health, and water quality in surrounding areas. This has direct positive effects on local ecosystems and the health of both humans and wildlife.
Conclusion
The future of small-scale power plants in agricultural processing plants is full of potential. These systems not only address the energy needs of agricultural processors but also contribute to global sustainability goals by promoting the use of renewable energy, reducing waste, and lowering emissions. With ongoing technological advancements, government incentives, and support for the circular economy, small-scale power plants will continue to evolve, becoming even more efficient, economically viable, and integrated into local communities.
As agricultural processing plants increasingly adopt renewable energy solutions, the industry will become more resilient, sustainable, and self-sufficient. Small-scale power plants represent a powerful tool for creating a more sustainable future for agriculture while simultaneously providing economic benefits, job opportunities, and a cleaner environment for local communities.
As small-scale power plants continue to grow in prominence within agricultural processing, several important trends and developments are emerging that will shape their future trajectory. These developments are particularly focused on improving scalability, efficiency, and integration of renewable energy systems, ensuring that these power plants are capable of meeting the evolving needs of the agricultural industry while contributing to broader global sustainability goals.
Innovations in Modular and Scalable Power Systems
A key trend in the development of small-scale power plants is the adoption of modular and scalable systems. These systems allow for more flexible deployment of renewable energy technologies, enabling agricultural processing plants to scale up or down based on their energy needs. Modular systems, such as biomass boilers, solar PV installations, and micro wind turbines, can be easily expanded or reconfigured as a plant’s energy demands change over time.
The scalability of these systems means that smaller operations can start with a limited energy capacity and gradually expand their renewable energy infrastructure as they grow, reducing the upfront investment needed. Similarly, modular systems allow plants to experiment with various renewable energy sources to find the optimal combination of technologies for their specific needs, taking into account factors such as feedstock availability, local climate, and energy demand patterns.
For example, a dairy farm or food processing facility may begin by installing a small biomass boiler or a solar array to generate a portion of its energy needs. As the operation grows or diversifies, additional modules—such as larger solar PV systems, biogas digesters, or wind turbines—can be added to meet increasing energy requirements. This approach not only ensures cost-effective and efficient energy generation but also future-proofs the facility against rising energy costs or changes in the energy market.
Integration of Digital Technologies and Smart Grids
The integration of digital technologies into small-scale power plants is transforming how energy is generated, managed, and consumed in agricultural processing facilities. The use of smart grids, Internet of Things (IoT) devices, and artificial intelligence (AI) enables real-time monitoring and optimization of energy systems, improving both efficiency and reliability. These technologies provide the ability to monitor energy production and consumption across a facility, detect inefficiencies, and automatically adjust energy use in response to changing conditions.
For instance, smart meters can track energy usage at various points throughout the plant, allowing operators to identify areas where energy is being wasted and take corrective actions. Similarly, AI algorithms can analyze historical energy demand patterns and optimize the performance of renewable energy systems based on forecasted weather conditions or production schedules. This helps ensure that energy is used as efficiently as possible, reducing costs and waste.
The implementation of smart grids in small-scale power plants further enhances energy resilience by allowing these systems to connect and communicate with the broader grid. Through the use of demand-response programs, power plants can adjust their energy consumption or feed excess energy back into the grid when demand is high, helping to balance grid load and improve overall system stability. This also opens the door for microgrids, which can operate autonomously during power outages, providing agricultural processing facilities with a reliable, self-sustaining energy source.
Expanded Utilization of Agricultural Waste
A growing trend is the expanded use of agricultural waste for energy generation, which supports both sustainability and waste management efforts. Many agricultural processing plants produce large amounts of organic by-products that are often discarded, such as crop residues, fruit skins, seeds, and animal manure. Rather than treating this waste as a liability, small-scale power plants are increasingly seen as an opportunity to convert it into valuable energy resources.
Technologies like anaerobic digestion and biomass gasification have made it possible to use these by-products for biogas or electricity production, reducing the need for waste disposal and significantly lowering the carbon footprint of agricultural operations. Anaerobic digesters, for instance, break down organic waste to produce biogas, a mixture of methane and carbon dioxide, which can be used for power generation. The by-product of anaerobic digestion, known as digestate, can also be used as organic fertilizer, further contributing to a circular economy and enhancing soil health.
Additionally, the use of advanced biomass gasification technology allows agricultural waste, including wood chips, straw, and other crop residues, to be converted into syngas (synthetic gas), which can be used to generate electricity or heat. This conversion of waste into energy helps address waste disposal challenges and supports sustainable agricultural practices.
The increased focus on utilizing agricultural waste as a feedstock for renewable energy generation aligns with the growing interest in zero-waste and circular economy models, where materials are kept in use for as long as possible and waste is minimized. Small-scale power plants, by converting agricultural waste into energy, not only reduce environmental impact but also create new revenue streams for farmers and agricultural processors.
Energy Resilience and Climate Change Adaptation
As climate change continues to impact global weather patterns and agricultural operations, small-scale power plants offer a way to increase energy resilience in the face of extreme weather events. Events such as droughts, floods, storms, and heatwaves can disrupt conventional energy supplies, particularly in rural and remote areas. By relying on locally generated renewable energy, agricultural processing plants can better prepare for these challenges, ensuring that they have a consistent energy supply, even when the central grid is affected.
For example, during prolonged droughts or heatwaves, traditional power grids may struggle to meet energy demand, particularly if hydropower or coal-fired power plants are impacted by water shortages or heat-related efficiency declines. Small-scale power plants, on the other hand, which rely on locally sourced biomass, wind, solar, or waste-to-energy technologies, can continue to operate without the vulnerabilities associated with external grid disruptions. Additionally, these renewable energy sources are more adaptable to varying local conditions, making them better suited for regions with high variability in weather patterns.
Microgrids and distributed energy systems offer another layer of protection, allowing agricultural processing plants to operate independently of the central grid during grid outages. These systems enhance the energy autonomy of agricultural operations, enabling them to remain functional in the face of disruptions while minimizing the impact of natural disasters or supply chain interruptions.
Collaborative and Community-Based Energy Solutions
Another significant shift in the future of small-scale power plants is the rise of community-based energy solutions. In rural areas, where many agricultural processing plants are located, the deployment of cooperative energy systems can help reduce costs, improve energy access, and foster a sense of community ownership and involvement.
Agricultural processing plants can work together with local farmers, cooperatives, and communities to share renewable energy resources, such as biogas plants, solar arrays, or wind turbines. These community-based energy solutions allow smaller farms or processing plants to pool resources, share the costs of renewable energy infrastructure, and benefit from economies of scale.
For instance, a community-owned biomass power plant could source feedstock from multiple local farms, generating renewable energy for the entire community while also providing a local job market and economic development opportunities. Such collaborative models not only reduce energy costs but also strengthen local economies by keeping energy production and revenue within the community. Furthermore, they can be a model for energy democracy, where communities have greater control over their energy future and are empowered to adopt sustainable practices.
The Role of Education and Workforce Development
As the demand for renewable energy in agricultural processing grows, there will be an increasing need for skilled workers in the renewable energy and energy efficiency sectors. The adoption of small-scale power plants will create a need for specialized training and workforce development programs that focus on the installation, maintenance, and operation of renewable energy systems.
These programs can range from vocational training in specific technologies, such as solar PV or biogas systems, to university-level degrees focused on renewable energy engineering and sustainable agricultural practices. Additionally, offering training opportunities for local workers ensures that the transition to renewable energy benefits local communities and creates long-term employment in the region.
Training workers to manage the new technologies integrated into small-scale power plants also provides an opportunity to upskill the workforce in rural areas, enhancing their future job prospects. Many agricultural processing plants will require workers who can operate and troubleshoot advanced smart grids, AI-based energy management systems, and energy storage solutions. By fostering a skilled workforce, agricultural processing plants can ensure that their renewable energy systems are properly maintained and optimized for maximum efficiency.
Conclusion
Small-scale power plants are transforming the way agricultural processing facilities operate, providing a more sustainable, resilient, and economically viable energy solution for the industry. With innovations in modular systems, digital technologies, agricultural waste utilization, and community-based energy solutions, these plants are poised to become even more integrated into the agricultural sector, offering a range of benefits that go beyond energy generation. They help improve energy independence, reduce environmental impact, and create new economic opportunities for rural communities.
As the agricultural sector continues to evolve, small-scale power plants will be an essential part of the solution to ensure that food production is both sustainable and resilient to the challenges of climate change. With the right combination of technology, policy support, and community involvement, small-scale power plants can significantly contribute to a greener, more sustainable agricultural future.
Small Scale Power Plant for Agricultural Processing Plants
Small-scale power plants are becoming increasingly important for agricultural processing plants, providing cost-effective, reliable, and sustainable energy solutions. These plants play a vital role in meeting the energy demands of the agricultural sector, which includes processing, packaging, and value-added operations. Agricultural processing plants, such as those involved in food, beverage, and textile production, often face fluctuating energy demands and high operational costs, which can be mitigated by adopting small-scale power generation systems. These systems use renewable resources like biomass, solar, wind, and biogas to generate energy locally, improving energy security, reducing emissions, and enhancing economic sustainability.
Benefits of Small-Scale Power Plants in Agricultural Processing
The main appeal of small-scale power plants for agricultural processing plants lies in their ability to provide on-site, reliable, and affordable energy. By utilizing renewable energy sources such as biomass, solar, and wind, these power plants reduce reliance on external energy suppliers and the grid, which can often be unstable or expensive, especially in rural areas. Biomass systems, for example, can be fueled by organic waste from agricultural operations, such as crop residues, animal manure, and food processing by-products. In this way, small-scale power plants address two significant challenges in the agricultural sector: waste disposal and energy generation.
These power plants offer cost savings by offsetting high electricity bills, reducing fuel costs, and increasing overall energy efficiency. Since energy costs are often one of the largest operational expenses for agricultural processors, the use of small-scale renewable energy systems offers significant financial savings in the long term. These savings are particularly valuable for small and medium-sized agricultural processing businesses that may struggle with the high costs of energy in traditional systems.
Additionally, small-scale power plants contribute to the reduction of greenhouse gas emissions. By using renewable sources instead of fossil fuels, these power plants help agricultural processors reduce their carbon footprints and comply with increasingly stringent environmental regulations. Moreover, small-scale power plants can be integrated with energy storage systems, enabling better load management and increased reliability in energy supply, especially during peak demand periods or when renewable energy sources are intermittent.
Biomass as a Primary Energy Source
One of the most widely used renewable energy sources in small-scale agricultural power plants is biomass. Agricultural processing facilities generate significant quantities of organic waste, including corn stalks, rice husks, wood chips, fruit and vegetable scraps, and even animal waste. Rather than discarding this waste, small-scale power plants can use it as fuel for energy generation. The process of converting biomass into usable energy is typically done through combustion, gasification, or biogas production.
In a biomass combustion system, organic waste is burned to produce heat, which is then used to generate steam for power turbines. Biomass gasification, on the other hand, converts solid biomass into a synthetic gas (syngas), which can be used to produce electricity. Anaerobic digestion, which breaks down organic waste in the absence of oxygen, produces biogas, a mixture of methane and carbon dioxide. The methane in the biogas can then be used to generate electricity and heat.
By adopting these systems, agricultural processing plants not only reduce waste but also create a reliable source of energy that can meet the plant’s ongoing needs. This approach is particularly effective in rural areas, where biomass feedstocks are abundant and energy infrastructure may be limited or underdeveloped.
Solar and Wind Energy Integration
While biomass is a popular energy source for small-scale agricultural power plants, solar and wind energy are also becoming increasingly common, particularly for operations in regions with high solar irradiance or wind speeds. Solar photovoltaic (PV) systems can be installed on-site to harness the power of the sun and convert it into electricity, which can be used to power various operations within the agricultural processing plant, such as refrigeration, lighting, and machinery.
In addition, small wind turbines can generate electricity from wind, offering another renewable energy option for agricultural processing plants located in wind-rich areas. Wind energy, like solar energy, can be intermittent, but when integrated with energy storage solutions like batteries, it becomes a reliable power source. Small-scale wind and solar systems can be used independently or in conjunction with biomass systems to create a hybrid energy solution. These hybrid systems improve energy security by providing power during different times of day or in varying weather conditions, ensuring that the agricultural plant has a consistent and reliable energy supply.
Energy Storage and Smart Grid Integration
As with any renewable energy source, intermittency is a challenge for both wind and solar energy. To address this, small-scale power plants are increasingly incorporating energy storage systems, such as lithium-ion batteries, flow batteries, and thermal storage solutions. These storage systems store excess energy generated during periods of high renewable output and discharge it when energy demand is high or when renewable energy generation is low.
For example, solar PV systems produce energy during the day, and excess energy generated can be stored in batteries or thermal storage for later use. Similarly, wind turbines generate power when the wind is blowing, and any surplus energy can be stored for use during times of calm wind. The incorporation of energy storage enables agricultural processing plants to avoid relying on the grid during peak hours, reducing their energy costs while ensuring that they have access to power when they need it most.
Moreover, the integration of smart grid technologies and energy management systems (EMS) is improving the efficiency of small-scale power plants in agricultural processing operations. These systems enable real-time monitoring of energy production, consumption, and storage, providing actionable data that can be used to optimize energy use and reduce waste. For example, smart meters can track the energy consumption of specific equipment, while EMS can automatically adjust energy use based on the availability of renewable power and the operational demands of the facility.
Waste-to-Energy Systems
One of the most promising aspects of small-scale power plants for agricultural processing is the use of waste-to-energy technologies. Agricultural processing plants often generate large quantities of organic waste, such as food scraps, crop residues, and animal manure. Instead of disposing of this waste through traditional methods (e.g., landfilling or incineration), small-scale power plants can convert it into energy, benefiting both the environment and the bottom line.
For example, anaerobic digestion can be used to process organic waste, converting it into biogas. This biogas can be burned to generate electricity or heat, which can be used on-site. Similarly, agricultural waste can be fed into pyrolysis or gasification systems to convert it into syngas or bio-oil, which can be used for electricity production or as a feedstock for further processing. By adopting waste-to-energy systems, agricultural processing plants can reduce their waste disposal costs and minimize the environmental impact of waste management.
In addition to providing a renewable energy source, waste-to-energy systems also produce valuable by-products, such as compost and digestate, which can be used as organic fertilizers for local farming operations. This closes the loop in the agricultural ecosystem, where waste is not only used to generate energy but also contributes to soil health and agricultural productivity.
Economic and Environmental Impacts
The economic and environmental benefits of small-scale power plants in agricultural processing are significant. First, by generating renewable energy locally, agricultural processing plants can reduce their reliance on imported electricity and lower their energy costs, improving their profitability. This is especially important in areas with high energy prices or unreliable grid infrastructure. In addition, small-scale power plants contribute to the local economy by creating jobs in the renewable energy sector, including jobs in installation, maintenance, and operation of energy systems.
From an environmental perspective, small-scale power plants help agricultural processing plants reduce their carbon emissions by displacing fossil fuel-based energy sources. This is increasingly important as governments worldwide implement stricter environmental regulations and companies face growing pressure to reduce their carbon footprints. By adopting renewable energy technologies, agricultural processors can meet sustainability goals, attract environmentally conscious consumers, and improve their corporate social responsibility (CSR) standing.
Policy Support and Government Incentives
Government policies and incentives play a critical role in the adoption of renewable energy technologies by agricultural processing plants. Many governments offer tax incentives, grants, and subsidies to encourage the adoption of small-scale renewable energy systems. Programs such as feed-in tariffs (FiTs), power purchase agreements (PPAs), and renewable energy certificates (RECs) provide financial incentives for agricultural processors to invest in renewable energy technologies.
For example, feed-in tariffs guarantee a fixed price for the electricity produced by renewable energy systems, providing a stable income stream for agricultural processors that generate surplus energy. Renewable energy certificates allow processors to earn credits for the renewable energy they generate, which can be sold or used to meet sustainability requirements. These financial incentives help offset the initial capital costs of renewable energy systems, making them more accessible to agricultural processing businesses.
In addition, government policies that encourage energy efficiency and sustainability in the agricultural sector are likely to drive further adoption of small-scale power plants. By incorporating renewable energy technologies, agricultural processors can reduce their energy consumption and minimize their environmental impact, positioning themselves as leaders in the transition to a low-carbon economy.
Conclusion
Small-scale power plants for agricultural processing plants represent a significant opportunity to improve the energy efficiency, sustainability, and profitability of the agricultural sector. Through the use of renewable energy sources like biomass, solar, and wind, these power plants can provide cost-effective, reliable energy while reducing waste and lowering carbon emissions. With the growing adoption of energy storage and smart grid technologies, small-scale power plants will continue to evolve, offering even greater efficiency and integration into the agricultural value chain.
The economic and environmental benefits of small-scale power plants are compelling, offering agricultural processors a path to greater energy independence, sustainability, and resilience. With the right combination of technology, policy support, and community involvement, small-scale power plants will play a crucial role in the future of the agricultural sector, ensuring that it meets the energy demands of today while contributing to a sustainable and prosperous future.
As the role of small-scale power plants continues to evolve within agricultural processing, several emerging trends and technologies will further shape their future. These trends focus on advancing energy efficiency, flexibility, and sustainability. The integration of new technologies and optimized energy management strategies will enhance the resilience of agricultural processing facilities, making them more adaptable to changing market conditions, environmental challenges, and regulatory pressures.
Integration with Modern Agricultural Practices
The integration of small-scale power plants with modern agricultural practices is increasingly becoming a priority. The concept of precision agriculture—which uses technology such as drones, satellites, sensors, and data analytics—is gaining traction in agricultural processing. These technologies enable farmers and agricultural processors to gather real-time data on factors like soil conditions, crop health, weather patterns, and energy consumption, optimizing resource usage and improving productivity.
Small-scale power plants can play a critical role in this context by supporting data-driven energy management systems. For example, advanced energy management platforms can integrate data from both the agricultural operation and the power plant, enabling real-time adjustments to energy production and consumption. This can be done by adjusting power output from renewable sources like solar or wind, depending on current weather conditions and energy needs. Additionally, smart grids can further optimize energy use, connecting agricultural processing plants to other energy assets in a local area, which can be shared between nearby facilities or farms.
This integration improves operational efficiency and helps agricultural processors reduce both energy waste and costs, aligning with broader goals of resource conservation and sustainability. By aligning power generation and consumption with crop cycles, weather patterns, and market demands, agricultural operations can ensure they are making the most of their resources, while still achieving high levels of energy reliability.
Microgrids for Energy Resilience
The concept of microgrids is also emerging as a critical solution for ensuring the energy resilience of small-scale agricultural processing plants. Microgrids are localized energy networks that can operate independently from the larger grid, providing a reliable and self-sustaining energy supply. They can integrate renewable energy sources like solar PV, wind, and biomass, along with energy storage systems, to ensure power availability in remote or rural areas, where energy supply from the central grid may be unreliable or costly.
By adopting a microgrid, agricultural processing plants can ensure that they are not vulnerable to power outages, which are increasingly common in many parts of the world due to extreme weather events and other disruptions. For example, floods, hurricanes, and wildfires can damage power lines and disrupt grid electricity supply, which could halt critical operations in agricultural processing facilities, leading to significant financial losses.
Microgrids provide autonomy to agricultural plants, allowing them to continue operating in isolated conditions, such as during a grid outage or in areas without access to a central electricity network. This energy independence increases business continuity and reduces the potential for production downtime, especially during high-demand periods or peak harvest seasons when operations are at their most critical.
Additionally, microgrids offer flexibility, as they can easily scale up or down based on the energy needs of the processing facility. A growing agricultural operation can add new renewable energy modules, expand storage capacity, or integrate new technologies as needed, creating a system that is adaptable to changing demands.
Hybrid Systems for Increased Efficiency
One of the most promising developments in small-scale power plants for agricultural processing is the adoption of hybrid energy systems. These systems combine multiple renewable energy sources—such as solar, wind, biomass, and geothermal—to create a balanced and reliable energy generation model. The hybrid approach allows agricultural processing plants to maximize energy production by relying on different energy sources that complement each other throughout the year.
For instance, solar energy is typically most effective during the day, while wind turbines generate electricity when the wind is strong, often at night or during the colder months. Biomass can provide a continuous energy supply, regardless of weather conditions, as it uses locally available organic waste as fuel. By combining these sources, small-scale power plants can maintain a constant and reliable energy supply, even during periods of low sunlight or wind.
In some cases, hybrid systems can also incorporate energy storage technologies, such as batteries or thermal storage, to store surplus energy for later use. This makes hybrid systems highly adaptable to fluctuations in energy demand, especially in agricultural operations where energy needs can vary seasonally or based on the timing of harvests.
Furthermore, hybrid systems allow agricultural processing plants to diversify their energy generation, reducing reliance on any single energy source and decreasing their exposure to the risks associated with supply chain disruptions, price fluctuations, or resource depletion. This diversification provides a more sustainable, resilient, and economically viable energy solution for agricultural processing.
Sustainability and Circular Economy Models
As agricultural processing facilities continue to seek ways to improve sustainability, small-scale power plants are increasingly being incorporated into circular economy models. The concept of a circular economy aims to minimize waste, make the most of available resources, and close the loop between production and consumption. Small-scale power plants, particularly those utilizing biomass and waste-to-energy technologies, fit seamlessly into this model by repurposing agricultural waste to generate energy, rather than sending it to landfills.
Anaerobic digesters that process food scraps, manure, and other organic waste not only generate biogas for energy production but also create nutrient-rich digestate that can be used as organic fertilizer for farming. This by-product can be returned to the land, improving soil fertility and closing the loop between agricultural production, processing, and energy generation.
Similarly, biomass gasification and pyrolysis systems use crop residues, such as corn stover or rice husks, to generate heat or electricity. This process not only provides a sustainable source of energy but also reduces the environmental burden of waste disposal, which would otherwise contribute to landfill accumulation or air pollution through burning.
Small-scale power plants that follow circular economy principles also have the potential to reduce carbon emissions by minimizing waste and using energy more efficiently. By utilizing renewable energy, agricultural processing facilities can reduce their reliance on fossil fuels, leading to a significant decrease in greenhouse gas emissions, and contributing to global efforts to tackle climate change.
Collaboration with Local Communities
As the need for renewable energy solutions grows, small-scale power plants in agricultural processing will increasingly foster collaboration with local communities. These partnerships can be mutually beneficial, as small-scale power plants can contribute to the local economy, improve energy access, and create jobs in rural areas.
By working with local farmers and agricultural cooperatives, processing plants can ensure a steady supply of biomass feedstock for energy generation, while also improving the profitability of nearby farms. This creates a sustainable and closed-loop model in which agricultural producers benefit from both energy generation and waste management. In some cases, small-scale power plants can even support community-scale energy projects, such as shared biogas systems or solar cooperatives, where local residents and businesses collectively own and operate renewable energy infrastructure.
Moreover, such partnerships can help address energy access issues in remote or underserved rural areas. Small-scale power plants can provide a much-needed alternative to the central grid, bringing affordable and reliable energy to regions that may otherwise have limited access to electricity. This enhanced energy access can stimulate local economic development, improve education and healthcare infrastructure, and empower rural communities to achieve energy independence.
The Role of Policy and Financial Incentives
The widespread adoption of small-scale power plants in agricultural processing will depend heavily on government policies and financial incentives. Many governments have recognized the need to support renewable energy adoption in the agricultural sector, and several policies exist to incentivize the transition to cleaner energy solutions. Programs such as tax credits, grants, subsidies, and feed-in tariffs can significantly reduce the initial capital investment required to install renewable energy systems.
In particular, governments may introduce incentives to support energy efficiency measures and the adoption of low-carbon technologies in agricultural processing. This could include funding for energy audits, assistance with system installation, or low-interest loans for small-scale power plant projects. Additionally, policy frameworks that promote the circular economy, waste-to-energy systems, and cooperative energy models will help align the interests of agricultural processors, farmers, and local communities, creating more opportunities for sustainable development.
Moreover, international trade agreements and partnerships between governments, non-governmental organizations, and industry associations can support the expansion of renewable energy infrastructure in the agricultural sector. Financial models such as public-private partnerships (PPPs) and green bonds could also be explored to attract investment in small-scale renewable energy systems for agricultural processing.
Conclusion
Small-scale power plants are emerging as a key solution for improving the sustainability, energy efficiency, and resilience of agricultural processing plants. By integrating renewable energy technologies, waste-to-energy systems, and energy storage solutions, these plants provide affordable and reliable energy that supports agricultural operations while reducing their environmental impact. The continued advancement of energy management systems, microgrids, and hybrid technologies will further optimize these energy systems, creating more flexible and resilient energy solutions for the agricultural sector.
Through collaboration with local communities, governments, and agricultural producers, small-scale power plants can contribute to the growth of a sustainable agricultural economy that benefits both people and the planet. With the right support, policy frameworks, and financial incentives, small-scale power plants have the potential to play a transformative role in the agricultural processing industry, ensuring that it remains both economically viable and environmentally responsible in the face of evolving energy challenges.
As small-scale power plants continue to shape the future of agricultural processing, their role in sustainable development and energy independence will only become more pronounced. These energy solutions are essential not just for operational efficiency but for creating a more resilient, eco-friendly agricultural sector that can weather the challenges of climate change, fluctuating energy prices, and regulatory changes. The integration of advanced technologies and sustainable practices is enabling agricultural processing plants to operate in a way that supports not only their profitability but also the broader global sustainability goals.
Technological Advancements in Small-Scale Power Plants
The rapid pace of technological advancement is expanding the capabilities of small-scale power plants in agricultural processing. Innovations in energy generation and management technologies are enhancing the efficiency, scalability, and flexibility of renewable energy solutions. As more advanced energy systems become accessible to agricultural processors, they can integrate cutting-edge solutions that improve operational performance while ensuring long-term sustainability.
- Enhanced Biomass Conversion Technologies: Biomass energy systems are continually evolving. New technologies, such as advanced combustion systems, integrated pyrolysis, and improved gasification units, are increasing the efficiency of biomass-to-energy conversion. By improving the conversion efficiency, biomass systems can generate more energy from smaller quantities of raw biomass material, making them more cost-effective and reducing the need for large amounts of feedstock. Additionally, next-generation anaerobic digesters are enhancing the biogas production process, enabling agricultural processors to get more energy from organic waste and minimizing waste disposal issues.
- Solar Efficiency and Storage Innovations: Solar energy technologies are also becoming more efficient. The development of bifacial solar panels, which capture sunlight on both the front and rear sides, is increasing the energy output of solar PV systems. Furthermore, solar concentrators are being utilized to concentrate sunlight onto a small, highly efficient solar cell, providing greater energy yields even in areas with lower solar irradiance. Coupled with innovations in battery storage, such as solid-state batteries, the storage and discharge capacity of solar-generated energy is improving, making solar a more reliable and consistent energy source, even during periods of cloud cover or at night.
- Advanced Wind Turbines: Wind energy, too, is benefiting from innovations in turbine design. Modern wind turbines are more aerodynamically efficient, quieter, and better suited for smaller land areas, making them ideal for integration into small-scale agricultural operations. These turbines are capable of harnessing energy from even modest wind speeds, providing agricultural processors with another viable renewable energy option.
- Smart Energy Management and AI Integration: One of the most exciting areas of development is the integration of artificial intelligence (AI) and machine learning (ML) into the management of small-scale power plants. These technologies allow for real-time monitoring and predictive maintenance, ensuring that renewable energy systems operate at optimal efficiency. AI algorithms can also predict energy demand patterns, allowing for more accurate energy storage and distribution. Furthermore, these intelligent systems can automatically adjust the output of various energy sources in response to demand fluctuations, improving the overall performance of hybrid energy systems.
- Microgrid Optimization: As microgrids become an essential feature of small-scale power plants, technologies that optimize their operation are also evolving. Microgrid control systems are becoming smarter, allowing agricultural processing plants to better manage the balance between distributed energy generation, energy storage, and load demands. These systems enable plants to operate in an isolated mode when necessary, ensuring that they can continue functioning even during grid failures or outages. Advanced demand-response systems within microgrids allow for the automatic adjustment of energy usage in response to peak pricing periods or grid instability, helping to lower costs and improve efficiency.
The Role of Digitalization and Automation
Digitalization and automation technologies are becoming integral parts of small-scale power plants in agricultural processing. These technologies not only optimize energy production and consumption but also contribute to a more sustainable and efficient operation. As more agricultural processing plants embrace Industry 4.0 principles, the ability to digitally monitor and control energy systems becomes a game-changer.
- Real-Time Energy Monitoring: Digital platforms that offer real-time monitoring of energy consumption, production, and storage are allowing agricultural processing plants to track performance metrics with unprecedented accuracy. Smart meters and Internet of Things (IoT) devices embedded throughout the plant can collect data on equipment performance, energy usage, and environmental conditions, feeding this data into centralized management systems. This data allows for predictive analytics, which can identify potential issues before they become major problems, enabling better maintenance scheduling and system optimization.
- Automation of Energy Distribution: Automation technologies are increasingly being used to control the distribution of energy within small-scale power plants. Through automated control systems, power plants can adjust the operation of renewable energy systems and storage units to match real-time energy demand. For example, energy usage in certain sections of a processing plant can be automatically reduced during peak hours to avoid costly grid energy consumption, while energy from stored batteries or renewable sources is used to power the operations. This automation reduces the risk of human error, improves energy efficiency, and lowers operational costs.
- Blockchain for Energy Transactions: One potential future development in the energy sector is the integration of blockchain technology for managing energy transactions. Blockchain could allow agricultural processing plants to engage in peer-to-peer energy trading with nearby businesses or farms. This decentralized approach to energy distribution could allow agricultural plants to buy and sell energy within their local communities, facilitating decentralized energy economies and providing greater financial incentives for renewable energy producers.
Challenges and Solutions for Widespread Adoption
Despite the significant advantages of small-scale power plants for agricultural processing, several challenges must be addressed to increase their widespread adoption. These challenges primarily involve upfront capital costs, technological integration, and regulatory compliance. However, there are several promising solutions that can help overcome these barriers.
- Initial Capital Investment: One of the major obstacles to the adoption of small-scale power plants is the initial capital required for purchasing and installing renewable energy systems, energy storage units, and smart grid technologies. However, government incentives and private sector financing are playing a crucial role in reducing these upfront costs. Green bonds, tax credits, and grant programs can significantly offset the initial investment burden. In addition, power purchase agreements (PPAs) allow agricultural processors to engage in long-term contracts with energy providers, ensuring they can access renewable energy at competitive rates without incurring the upfront capital costs.
- Technological Integration: Integrating new energy technologies into existing agricultural processing facilities can be challenging, particularly in older plants with outdated infrastructure. To address this, modular energy solutions are being developed that allow for easy upgrades and expansions to existing power systems. Modular renewable energy systems—such as solar kits, small-scale wind turbines, and biomass boilers—can be added incrementally, reducing the need for large-scale infrastructure overhauls. These modular systems also allow for future scalability, enabling agricultural plants to gradually increase their energy generation as their operational needs grow.
- Regulatory Compliance and Incentive Utilization: Navigating government regulations and accessing financial incentives can be a complicated process, particularly for smaller agricultural processors. Many governments are simplifying the regulatory framework for renewable energy adoption and creating more streamlined permitting processes for small-scale power plant installations. Additionally, industry associations and trade groups are offering technical support and advisory services to help agricultural processors access available incentives and subsidies.
- Workforce Training: As agricultural processing plants transition to renewable energy solutions, there will be an increasing need for skilled labor to operate and maintain these systems. The development of training programs for employees, focusing on renewable energy technologies and smart grid management, will be essential to ensuring the long-term success of small-scale power plants in the agricultural sector. Collaboration with vocational schools and technical colleges can help develop a skilled workforce capable of supporting the growing renewable energy sector in agriculture.
Conclusion: The Future of Small-Scale Power Plants in Agricultural Processing
The future of small-scale power plants for agricultural processing is bright, with increasing opportunities for energy independence, cost savings, and sustainability. As renewable energy technologies advance and become more cost-effective, agricultural processing plants are well-positioned to benefit from a shift toward cleaner, more efficient energy solutions. The integration of biomass, solar, wind, and energy storage technologies will continue to evolve, enabling plants to become more energy-efficient, reliable, and self-sustaining.
Small-scale power plants are also contributing to broader climate change mitigation efforts by reducing carbon emissions, supporting circular economies, and improving local energy access. Through innovation, collaboration, and ongoing technological advancements, agricultural processing plants will play a critical role in building a sustainable agricultural future, promoting economic resilience, and fostering a cleaner, greener planet.
In the coming years, small-scale power plants will continue to empower the agricultural sector to meet both its operational and environmental goals, supporting the global transition to a more sustainable and low-carbon economy. As adoption grows, these systems will be instrumental in ensuring that the agricultural industry remains energy resilient, cost-effective, and environmentally responsible in the face of a rapidly changing world.
As we continue to explore the evolving role of small-scale power plants in agricultural processing, it’s clear that their impact will be significant, not only in terms of energy efficiency but also in fostering economic growth, rural development, and environmental sustainability. In the next phase of this transition, the integration of digital technologies, smart grids, and advanced data analytics will become central to maximizing the potential of small-scale energy solutions. Furthermore, the development of new policy frameworks and public-private partnerships will ensure that these systems can be scaled up and adopted by a wide range of agricultural processing plants across various regions.
Smart Agriculture and Energy Systems
The role of smart agriculture will increasingly intersect with small-scale power plants. As agricultural processing becomes more reliant on data-driven technologies, energy systems will need to be increasingly adaptive, efficient, and flexible. The growing trend toward precision agriculture means that data gathered from fields, weather patterns, and agricultural operations can be fed directly into energy management systems to optimize power generation and consumption.
- Precision Energy Management: Smart sensors and data analytics are enabling agricultural processing plants to better predict energy needs based on real-time conditions. For instance, weather forecasts can influence when to store solar or wind energy for later use, ensuring that the plant always has sufficient power when demand peaks, such as during harvest seasons or processing surges. By combining real-time energy data with agricultural data, plants can tailor their power generation strategies more effectively. This ensures that energy production aligns with the dynamic needs of the agricultural operation, reducing waste and maximizing the utility of renewable energy resources.
- Agriculture-as-a-Service (AaaS): In the future, agricultural processing plants might adopt a more service-oriented approach to energy provision. Similar to other industries, Agriculture-as-a-Service (AaaS) could offer energy management solutions that allow farmers and processors to lease or share renewable energy equipment, such as solar panels or biogas systems, with neighboring facilities. This shared economy model would allow smaller operations to access the benefits of small-scale power generation without having to invest in expensive infrastructure upfront. It could also help stabilize energy prices for those in remote or underserved regions.
- IoT-Enabled Monitoring: The Internet of Things (IoT) is already being used in agricultural processing plants to remotely monitor operations, track machinery performance, and improve supply chain logistics. This technology will also extend to power plants, where smart meters and sensors will provide real-time insights into energy consumption, efficiency, and system health. By allowing operators to remotely adjust energy settings and perform maintenance tasks as needed, IoT-enabled power plants will significantly enhance the efficiency and reliability of renewable energy generation.
- Edge Computing for Real-Time Decision Making: As small-scale power plants rely more heavily on digital technologies, the integration of edge computing—which processes data locally, rather than in a centralized cloud—will enable faster decision-making. This technology will help power plants respond more quickly to fluctuating energy demands, manage distributed energy resources (DERs), and optimize grid integration in real time. With edge computing, the combination of renewable energy production and smart grid integration can be optimized on-site, resulting in enhanced overall system performance.
Policy and Regulatory Landscape
For small-scale power plants to realize their full potential, supportive policy frameworks and regulatory incentives will be essential. Government policies are crucial in providing a conducive environment for the development and implementation of renewable energy solutions in agricultural processing. The role of local governments, regional authorities, and international organizations in promoting sustainable energy solutions will continue to grow.
- Incentives for Renewable Energy Integration: Governments can provide a range of incentives to encourage small-scale power plants. These might include tax credits, grants, subsidies, and feed-in tariffs to reduce the upfront costs of renewable energy installations. Additionally, carbon pricing mechanisms can make fossil-fuel-based energy sources more expensive, creating a financial incentive for agricultural processing plants to switch to renewable energy solutions.
- Decentralized Energy Policies: In many regions, the focus is shifting toward decentralized energy systems, where power generation is localized rather than relying solely on centralized grids. Policies that promote microgrids and local energy markets will be key in enabling small-scale power plants to thrive. For example, governments could create frameworks that allow agricultural processing plants to sell surplus energy to neighboring communities or other businesses, fostering an energy-sharing economy that benefits local communities.
- Support for Research and Development: Governments can support research and development in the energy sector to accelerate the adoption of new technologies and innovations that benefit small-scale power plants. R&D funding for the development of advanced storage technologies, smart grids, energy efficiency solutions, and emission-reducing technologies will help lower costs and increase the performance of small-scale renewable energy systems in agricultural processing.
- Standardization and Certification: To ensure that small-scale power plants are operating efficiently and safely, clear standards for installation, operation, and maintenance must be developed. Standardization will help establish minimum performance thresholds, ensuring that small-scale power systems deliver on their promises of efficiency and reliability. Certifications for renewable energy products, such as biogas digesters, wind turbines, and solar panels, will promote industry-wide best practices, reducing the risk of system failures and helping to increase consumer confidence in renewable technologies.
- Long-Term Policy Vision: Governments must craft long-term strategies that not only encourage the growth of small-scale power plants but also support the sustainability and scalability of these systems. A national energy transition plan that includes clear sustainability targets, net-zero goals, and energy resilience objectives will provide clarity and stability for agricultural processors looking to adopt renewable energy technologies. This will be particularly critical for farmers and processors in regions where energy supply is unreliable or where access to affordable energy is limited.
Financial Models and Investment Opportunities
As the demand for small-scale power plants in agricultural processing grows, so too will the need for innovative financing models. Securing capital for renewable energy investments remains one of the biggest hurdles for agricultural operations. However, with the right financial tools in place, these plants will become increasingly viable.
- Green Bonds: One promising financial vehicle for funding small-scale power plants is the green bond. These bonds are specifically designed to fund environmentally sustainable projects. Agricultural processing plants could issue green bonds to attract investment for the installation of renewable energy systems. By linking bond issuance with specific energy-saving goals and environmental impact measures, agricultural processing plants can raise capital while promoting their sustainability credentials.
- Power Purchase Agreements (PPAs): PPAs provide an alternative funding model, allowing agricultural processors to enter into long-term contracts with energy providers. These agreements can help ensure that renewable energy is provided at a predictable cost, reducing the financial risks associated with volatile energy prices. By guaranteeing a steady stream of revenue over time, PPAs can make small-scale power plants more attractive to investors and lenders.
- Crowdfunding for Local Energy Projects: For smaller agricultural operations, crowdfunding platforms could provide a way to finance the installation of renewable energy systems. By raising capital from the local community, crowdfunding can engage stakeholders and create a sense of shared responsibility for the project. In this model, participants could receive discounts on energy costs or share in the financial returns from selling excess energy.
- Energy Service Companies (ESCOs): ESCOs can provide an effective financing solution for agricultural processing plants looking to invest in renewable energy systems. Under the ESCO model, an energy service company finances the installation and operation of energy-saving technologies, while the agricultural processor pays for the energy savings over time. This arrangement eliminates the need for large upfront investments, making renewable energy adoption more accessible to smaller operations.
The Path Forward for Small-Scale Power Plants in Agricultural Processing
Looking ahead, small-scale power plants will increasingly be a cornerstone of the agricultural processing sector’s energy future. Their integration with cutting-edge technologies, such as smart grids, IoT-based monitoring, and artificial intelligence, will continue to improve efficiency and optimize energy production. As agricultural processors increasingly demand sustainable and reliable energy, small-scale renewable energy systems will be poised to meet these needs, providing both cost savings and environmental benefits.
Government policies will play a crucial role in supporting this transition, ensuring that the appropriate regulatory and financial frameworks are in place to enable the growth of small-scale power plants. With continued research, development, and the adoption of innovative financial models, small-scale power plants will continue to be a key driver of sustainable agricultural practices, energy resilience, and local economic development.
As these systems gain broader acceptance and scale, the future of small-scale power plants in agricultural processing will contribute to a greener, more efficient world where agricultural operations are not only economically competitive but also environmentally responsible. Through continued collaboration between governments, industry, and local communities, small-scale power plants can play a pivotal role in achieving a low-carbon future for the agricultural sector.
Small Scale Power Plant with steam turbine for Offshore Oil and Gas Rigs
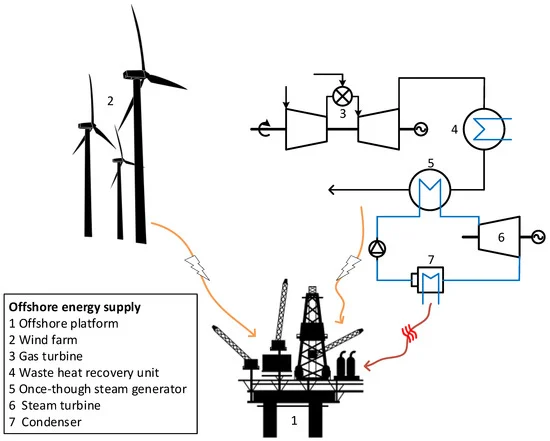
Small-scale power plants with steam turbines can offer significant benefits for offshore oil and gas rigs, where power needs are high, and energy reliability is critical. These platforms are often located in remote areas, far from the mainland grid, and need robust energy solutions that can function in harsh, variable environmental conditions. Traditional power systems on offshore rigs rely heavily on diesel generators, which have high operational costs and a larger environmental footprint. Small-scale power plants that integrate steam turbines can offer a more sustainable and cost-effective alternative for energy generation on these platforms.
The Role of Small-Scale Steam Turbine Power Plants in Offshore Oil and Gas Rigs
- Energy Efficiency and Sustainability: Offshore rigs have large energy demands for drilling operations, production processes, lighting, air conditioning, and other vital functions. Integrating a small-scale steam turbine with renewable or waste heat recovery systems can significantly improve energy efficiency. These turbines can be coupled with heat recovery steam generators (HRSGs) to capture excess heat from the rig’s operations—such as from exhaust gases or flue gases—and convert it into usable energy. By utilizing waste heat, offshore oil and gas rigs can reduce fuel consumption, minimize greenhouse gas emissions, and improve overall sustainability.
- Hybrid Energy Systems: Small-scale steam turbines can be used as part of a hybrid energy system, combining conventional energy sources (such as diesel generators) with renewable energy systems like solar power, wind power, or biogas. This hybrid approach helps balance the need for reliable and continuous energy with the benefits of renewable energy sources, which can reduce operating costs and improve the sustainability of offshore rigs. For example, solar thermal systems can provide heat that drives the steam turbine or contributes to the HRSG process, further enhancing energy efficiency.
- Reliability and Redundancy: Offshore oil and gas operations are particularly sensitive to power interruptions, as any downtime can result in significant financial losses and potential safety hazards. Small-scale steam turbines can provide reliable backup power in the event of failure in the primary power generation system, offering an additional layer of security. With multiple steam turbine units or hybrid configurations, rigs can ensure that they maintain constant power supply, even in the event of unexpected mechanical failures or fuel shortages.
- Modular Design and Scalability: Small-scale steam turbine systems are designed to be modular, meaning they can be scaled up or down depending on the energy needs of the offshore rig. As production demands fluctuate or as the rig undergoes upgrades, the energy system can be adjusted to ensure the power supply matches operational requirements. These turbines can also be integrated into microgrid systems that allow the rig to operate autonomously, managing power generation and consumption efficiently without relying on external sources.
Fuel Flexibility and Cost Savings
One of the key advantages of small-scale steam turbine systems for offshore oil and gas rigs is their fuel flexibility. While conventional diesel generators are commonly used, steam turbines can operate on a variety of fuels, including natural gas, which is often readily available on offshore rigs. They can also be designed to run on alternative fuels, such as biogas produced from organic waste on the rig or liquefied petroleum gas (LPG), which could be a more environmentally friendly option compared to traditional diesel. This fuel flexibility allows operators to optimize energy costs and reduce dependence on fuel imports.
- Natural Gas Utilization: In offshore oil and gas fields, natural gas is often a byproduct of extraction. Instead of flaring this gas, it can be used as a fuel source to generate electricity via a small-scale steam turbine. This gas-to-power approach reduces waste, increases energy efficiency, and lowers emissions, while also helping to meet the rig’s energy needs.
- Operational Cost Reduction: Steam turbines provide higher efficiency when running at constant load for extended periods, making them more suitable for the continuous power demands of offshore rigs. Compared to diesel generators, which have higher operational and fuel costs, steam turbines can offer significant cost savings in the long term. By combining renewable energy sources, waste heat recovery, and optimized fuel consumption, the overall operating costs of offshore oil and gas rigs can be lowered.
- Lower Emissions and Environmental Impact: Small-scale steam turbines can play a key role in reducing the environmental impact of offshore oil and gas operations. By using more sustainable fuel options, such as natural gas and biogas, and integrating renewable energy sources, steam turbine-based power plants can significantly reduce carbon emissions and the rig’s overall environmental footprint. Moreover, the use of heat recovery systems helps to minimize fuel waste and cut down on flaring, which is a major source of greenhouse gases in offshore oil operations.
Operational Benefits and Technological Advancements
The operational benefits of steam turbine-based power plants for offshore oil and gas rigs are numerous, driven by technological advancements that are continuously improving turbine performance, reliability, and efficiency.
- High Power Density: Steam turbines have a high power density relative to their size, making them ideal for offshore applications where space is limited. A small-scale steam turbine can generate a significant amount of power while taking up less physical space compared to other types of power generation units, such as diesel engines or gas turbines. This makes them particularly suitable for offshore rigs where compact, efficient systems are needed to meet high energy demands.
- Improved Fuel Efficiency: Recent innovations in steam turbine design, such as advanced materials, heat exchangers, and control systems, have improved the efficiency of small-scale steam turbines, making them even more effective for offshore applications. These improvements reduce fuel consumption, improve energy output, and increase turbine lifespan, further lowering operational costs over time.
- Autonomous and Remote Monitoring: Small-scale steam turbines on offshore rigs are increasingly integrated with remote monitoring systems and condition-based maintenance (CBM) technologies. These systems use sensors and IoT devices to collect real-time data on turbine performance, including vibration, temperature, pressure, and fuel efficiency. This data is sent to onshore control centers where it is analyzed, allowing for predictive maintenance and reducing the risk of unplanned downtimes. The use of artificial intelligence (AI) and machine learning algorithms can help optimize steam turbine operations by forecasting energy demand and adjusting the turbine’s load accordingly.
- Reduced Maintenance Needs: Steam turbines are typically more reliable than other types of power generation systems due to their fewer moving parts and ability to operate continuously at stable loads. They also have lower maintenance requirements compared to conventional engines or gas turbines, which require frequent overhauls and repairs. Robust design and the use of high-quality materials ensure that steam turbines can withstand the demanding offshore environment, including high humidity, corrosive saltwater, and extreme temperatures.
Challenges and Considerations
While small-scale steam turbine power plants provide numerous advantages for offshore oil and gas rigs, there are some challenges that need to be addressed for their widespread adoption:
- Installation and Integration: Installing a small-scale steam turbine system on an offshore rig requires careful planning and engineering, particularly to ensure that the system is compatible with existing infrastructure. The integration of heat recovery systems, fuel storage, and grid connection also requires substantial expertise and specialized equipment. This can result in higher initial installation costs, though these can be offset by long-term savings in fuel and maintenance costs.
- Availability of Fuel: While natural gas is often available on offshore rigs, the availability of alternative fuels such as biogas may be limited depending on the waste processing systems installed on the rig. In regions where natural gas is not available or in situations where renewable energy sources are more viable, steam turbine systems might need to be integrated with other fuel solutions to ensure consistent operation.
- Environmental Conditions: Offshore rigs operate in challenging environments that can affect the performance of energy systems. Factors such as saltwater corrosion, vibration, and extreme weather conditions can impact the longevity of equipment and require frequent maintenance. Steam turbines must be designed with these environmental challenges in mind to ensure their reliability and efficiency.
- Financial and Regulatory Factors: The adoption of small-scale steam turbine systems may require substantial initial capital investment. However, the long-term operational cost savings, combined with potential government incentives and carbon credit programs, can make steam turbine technology an attractive option. Regulatory frameworks and compliance standards for offshore energy systems may also require specific certifications and testing to ensure safety and environmental standards are met.
Conclusion
Small-scale steam turbine-based power plants have the potential to significantly improve the energy systems on offshore oil and gas rigs, offering reliable, efficient, and sustainable power generation. Through their ability to integrate waste heat recovery, renewable energy, and hybrid systems, steam turbines can lower operational costs, reduce environmental impacts, and enhance the overall sustainability of offshore oil and gas operations.
As technological advancements continue to enhance the performance of steam turbines, and as the demand for greener and more efficient offshore energy solutions grows, these systems are well-positioned to become a key part of the offshore industry’s energy mix. By improving fuel efficiency, increasing reliability, and providing flexibility in fuel choice, small-scale steam turbine power plants will help to meet the evolving energy needs of offshore oil and gas rigs while supporting the industry’s shift toward greater sustainability and environmental responsibility.
Expanding further on the role of small-scale steam turbine power plants for offshore oil and gas rigs, it’s important to address some of the long-term strategic advantages these systems can offer. As the offshore energy sector continues to evolve, focusing on sustainability, energy security, and cost-effectiveness, small-scale steam turbines integrated into hybrid energy systems will become an essential part of this transformation. Their ability to enhance energy reliability, improve operational efficiency, and reduce carbon emissions will make them increasingly attractive as offshore platforms aim to meet both regulatory compliance and corporate sustainability goals.
Optimization of Energy Use Through Hybrid and Integrated Systems
- Hybrid Renewable Energy Integration: One of the most promising developments for small-scale steam turbines on offshore rigs is their ability to integrate seamlessly with renewable energy sources, especially as offshore wind and solar power technology continues to mature. These renewable sources can help power the steam turbine system through electrical heating elements or supplement the power generation capacity during periods of high wind or sunlight. By combining solar thermal energy or wind-driven electrical generation with steam turbines, offshore rigs can further reduce their reliance on fossil fuels, lowering operational costs and improving their sustainability profile.
- Example of Wind-Steam Hybrid: Offshore wind farms are increasingly common in proximity to oil and gas platforms. Integrating steam turbines with the power generated by offshore wind systems can significantly reduce the reliance on diesel-powered generators, cutting fuel costs and ensuring more stable power production, especially during high-energy demand periods like drilling operations. In such a setup, wind power could be used to directly power electrical heaters that pre-heat fluids in the heat recovery systems, while steam turbines would convert waste heat into electricity for use by the platform.
- Advanced Heat Recovery Systems (HRSG): The optimization of heat recovery systems on offshore rigs can directly improve the performance of small-scale steam turbines. By capturing waste heat from rig operations (such as from exhaust gases or hot engine coolant), the overall energy efficiency of the platform is boosted. Advanced heat recovery steam generators (HRSGs) can convert lower-temperature waste heat into high-quality steam, which drives the turbines more efficiently. This can also contribute to the overall energy efficiency of the rig by reducing the need for additional fuel input, therefore lowering fuel costs and greenhouse gas emissions.
- Heat Recovery in Offshore Operations: For example, excess heat generated from drilling machinery or gas turbines used for mechanical work can be captured through HRSGs. This captured heat could then drive a small-scale steam turbine, generating electricity that offsets the power drawn from the grid or the main power generators. The ongoing optimization of HRSG systems ensures that more energy is derived from otherwise wasted heat, contributing to overall fuel savings.
- Combined Heat and Power (CHP) Systems: Small-scale steam turbines can also be integrated into Combined Heat and Power (CHP) systems, which provide both electricity and useful thermal energy (steam or hot water) for offshore platforms. This integrated approach ensures that power production is optimized by reducing waste heat and improving energy conversion rates. By capturing and utilizing the heat generated during power production, CHP systems offer improved fuel efficiency and lower emissions than traditional, separate power generation and heating systems.
- Example of CHP Use on Rigs: Offshore rigs require substantial amounts of thermal energy for processes like water desalination, heating fluids, and processing equipment. By pairing a steam turbine with CHP technology, the thermal energy produced can be used for these processes, while the turbine simultaneously generates electricity. The combination of heat and power reduces overall fuel consumption, as energy needs for both power and heating are met more efficiently.
- Microgrids and Energy Storage: Offshore rigs are increasingly adopting microgrid technology, which allows for better management of distributed energy resources and ensures that energy production and consumption are aligned in real time. Steam turbine-based power plants can be integrated into these microgrids, enabling the rig to operate as an independent, self-sustaining energy system. In addition, integrating energy storage systems such as batteries or thermal storage can help balance power supply and demand, storing excess renewable energy when available for use during periods of high energy demand.
- Microgrid Management: A small-scale steam turbine power system connected to a microgrid can automatically adjust its power output based on demand signals from the system. In addition, energy storage systems such as lithium-ion batteries or flow batteries can store excess energy generated during times of high wind or sunlight, providing backup power when the renewable generation drops. The integration of a microgrid ensures that the offshore rig remains powered in a reliable and efficient manner, even when renewable energy sources fluctuate.
- Autonomous Operation and AI-Based Optimization: The use of artificial intelligence (AI) and machine learning to optimize steam turbine operations will revolutionize their role on offshore oil and gas rigs. AI algorithms can monitor real-time operational data, anticipate energy demand fluctuations, and automatically adjust power generation from steam turbines, renewable sources, and backup systems. This AI-driven approach enhances efficiency by ensuring that power is always available in the most cost-effective manner while maintaining system stability.
- Predictive Maintenance and Remote Monitoring: With AI-driven diagnostics and predictive maintenance systems, steam turbines can continuously monitor their performance and anticipate potential failures before they occur. Sensors can track performance metrics, such as temperature, pressure, and vibrations, to alert maintenance crews to any issues. This remote monitoring system not only prevents unexpected downtimes but also reduces operational costs by ensuring that the turbine is always running at peak performance, extending the turbine’s lifespan and minimizing unplanned maintenance.
Long-Term Benefits and Strategic Considerations
While small-scale steam turbines offer a range of operational and environmental advantages, there are additional strategic factors to consider in their long-term implementation on offshore oil and gas rigs.
- Enhanced Energy Security and Independence: Small-scale steam turbines, when combined with renewable energy sources, provide offshore rigs with greater energy security by reducing dependence on external fuel supplies. This is especially important for rigs operating in regions with unstable supply chains or political instability that could impact fuel deliveries. By generating a portion of the energy required for operations locally, the rig can avoid supply chain disruptions and safeguard its energy needs.
- Regulatory Compliance and Carbon Footprint Reduction: Regulatory standards regarding emissions and environmental impact are becoming increasingly stringent, and offshore rigs are under increasing pressure to reduce their carbon footprint. Small-scale steam turbines powered by renewable energy or waste heat recovery systems can help meet these environmental targets by significantly reducing reliance on traditional fossil fuels, thereby lowering overall emissions. The use of clean energy sources and the reduction of flaring practices also align with international regulations designed to mitigate climate change and reduce offshore operations’ environmental impact.
- Long-Term Cost Competitiveness: Despite the initial investment required for installing small-scale steam turbine systems, their long-term cost-effectiveness makes them a compelling choice for offshore operations. The combined savings from reduced fuel consumption, lower maintenance costs, and the potential for integration with renewable energy sources help offset the upfront investment. Additionally, as technology continues to evolve and economies of scale are realized, steam turbines are likely to become even more affordable and efficient, further improving their financial viability for offshore rigs.
- Scalability and Flexibility: Small-scale steam turbines offer flexibility and scalability to adapt to changing energy demands. Offshore rigs, depending on their stage of development or production, may require varying energy capacities over time. The modular design of steam turbines makes them easy to scale up or down, enabling the rig to adapt to different energy needs as production activities evolve.
- Technological Advancements and Future Trends: The ongoing development of more efficient turbines, energy storage systems, and hybrid energy technologies will continue to drive down costs and increase the efficiency of small-scale power plants. Innovations in materials, design, and integration with digital systems (such as IoT and AI) will further improve the reliability and performance of steam turbine systems on offshore rigs. Moreover, the growing emphasis on hydrogen-based power and zero-carbon technologies may eventually complement or even replace conventional fuel sources, integrating with small-scale steam turbine systems for future-ready energy solutions.
Conclusion
Small-scale steam turbine power plants present a promising solution for offshore oil and gas rigs that need to meet growing energy demands while reducing costs, improving reliability, and enhancing sustainability. By integrating advanced heat recovery systems, hybrid energy solutions, renewable energy sources, and AI-driven optimization, these systems can significantly enhance the efficiency of offshore energy production.
As the offshore energy sector moves towards greater energy independence, regulatory compliance, and sustainability, small-scale steam turbines will play a pivotal role in this transformation. With continued advancements in technology, regulatory support, and financial incentives, the future of offshore oil and gas operations can be increasingly powered by cleaner, more efficient, and more cost-effective energy solutions, ensuring that these critical operations can continue to thrive in an environmentally responsible manner.
As small-scale steam turbine power plants continue to evolve in offshore oil and gas rigs, additional opportunities and benefits become apparent, especially when considering long-term efficiency, technological advancements, and integration with the broader energy ecosystem. These developments highlight the role of steam turbines as a crucial element in energy resilience and sustainability for offshore operations.
Innovative Design Features and Efficiency Gains
- Enhanced Heat Recovery and Multi-Stage Turbines: One of the key drivers for improving the efficiency of small-scale steam turbine power plants is the ongoing development of multi-stage turbines and enhanced heat recovery systems. Multi-stage turbines are designed to extract energy from steam at different pressure levels, optimizing the process and recovering more heat from the system. This means that the same amount of fuel input generates more power, which is especially beneficial in environments like offshore rigs where energy efficiency is paramount.
- Advanced Steam Conditions: Recent innovations allow for superheated steam conditions in small-scale turbines, increasing the thermal efficiency of the system. Superheating steam—by raising its temperature before it enters the turbine—improves the overall conversion of thermal energy into mechanical work, making the system more efficient. This becomes especially advantageous when paired with high-efficiency heat recovery systems, ensuring that the most energy is extracted from available fuel and waste heat.
- High-Temperature Materials and Durability: Offshore conditions expose energy generation systems to extreme challenges, including corrosion, vibration, and salinity, which can reduce equipment lifespan. The use of advanced materials in steam turbine construction is helping mitigate these challenges. New materials, including ceramics, composite alloys, and heat-resistant coatings, enable turbines to operate at higher temperatures and pressures without degrading. These innovations improve the longevity of the turbines and reduce the frequency of maintenance interventions, which are crucial for offshore operations where access to maintenance facilities may be limited.
- Digital Twin Technology: The integration of digital twin technology into small-scale steam turbine systems is a game changer. A digital twin is a virtual replica of the turbine system, which can simulate the turbine’s behavior in real time using data collected from sensors and performance metrics. This digital model allows engineers and operators to remotely monitor the turbine’s performance and conduct predictive maintenance without the need for physical inspections, reducing downtime and increasing operational efficiency.
- Simulation and Optimization: By using digital twins, operators can simulate various operating conditions and assess the turbine’s performance under different scenarios. This enables them to fine-tune the system for maximum efficiency, predict potential system failures, and plan maintenance schedules proactively. The use of this technology also reduces the reliance on offshore staff for on-site troubleshooting, as remote monitoring and adjustments can be made more effectively.
- Modular and Flexible Energy Storage: As part of a comprehensive energy solution, modular energy storage systems can enhance the effectiveness of small-scale steam turbine power plants on offshore rigs. Energy storage systems like lithium-ion batteries or flow batteries can store surplus electricity generated during low-demand periods, which can then be used during high-demand periods, reducing the need for additional fuel consumption.
- Buffer for Renewable Energy: When renewable sources such as wind or solar are used in tandem with steam turbines, fluctuations in energy production can occur. Energy storage systems serve as a buffer, ensuring that the power supply remains stable and continuous. By storing energy generated from renewable sources or from excess steam power, these systems prevent over-reliance on backup diesel generators, which still remain costly and less environmentally friendly.
The Role of Artificial Intelligence (AI) and Machine Learning (ML)
- Dynamic Load Management: One of the most powerful applications of AI in small-scale steam turbine operations on offshore rigs is its ability to manage dynamic loads and optimize energy distribution. AI-driven load forecasting systems can predict the energy demand of the platform, adjusting the output of the turbine and hybrid energy systems accordingly. This ability to anticipate demand changes enables offshore operations to avoid energy shortages or overproduction, resulting in more efficient fuel consumption and less wear and tear on the equipment.
- Real-Time Data Analytics: Machine learning algorithms can analyze large volumes of real-time data generated by the turbines and other energy generation assets, such as solar panels or wind turbines. By analyzing parameters like turbine speed, steam pressure, temperature, and fuel consumption, the system can identify inefficiencies or unusual patterns. The data collected can then be used to make real-time adjustments, such as optimizing the load distribution or altering turbine settings to meet changing demands.
- Predictive Maintenance: AI and ML models can also predict maintenance needs based on historical performance data, usage patterns, and environmental conditions. This helps prevent costly breakdowns by scheduling maintenance when necessary, avoiding unplanned outages that could otherwise disrupt the rig’s operations.
- Energy Demand Response: AI can further enhance the ability of the rig to respond to external energy market signals in real-time. For instance, if the energy market signals high prices for electricity or low availability, AI can trigger the steam turbines to produce additional power, while also optimizing fuel usage to meet local demands. This feature enhances the economic competitiveness of offshore rigs, allowing them to react intelligently to external energy fluctuations.
Cost-Effectiveness and Long-Term Financial Benefits
- Reduced Operational Costs: Over time, the integration of small-scale steam turbines into offshore oil and gas platforms can lead to significant reductions in operational costs. By utilizing waste heat recovery, renewable energy sources, and hybrid systems, the dependency on expensive fuels such as diesel is reduced, resulting in lower fuel costs. Furthermore, the operational efficiency gains from AI-driven optimization, advanced heat recovery systems, and reduced maintenance needs can contribute to considerable cost savings, making small-scale steam turbine power plants an increasingly cost-effective solution for offshore platforms.
- Capital Investment and Financial Support: While the initial capital investment for installing steam turbine systems can be high, the long-term benefits of reduced operational and maintenance costs help offset this investment. Moreover, government incentives for renewable energy integration, carbon reduction programs, and energy efficiency improvements may also reduce upfront costs. In some regions, offshore oil and gas operators can receive subsidies or tax credits for installing energy-efficient systems, which makes the transition to small-scale steam turbines financially attractive.
- Scalability and Adaptability: Steam turbine power plants are inherently scalable, meaning they can be designed to grow with the rig’s energy needs. As production increases or energy demands fluctuate, additional steam turbine units can be integrated, and existing systems can be upgraded without significant disruption to the platform’s operations. This scalability ensures that the offshore rig can adapt to evolving energy needs while maintaining the same level of operational efficiency.
Environmental and Sustainability Considerations
- Reduction in Greenhouse Gas Emissions: One of the most compelling reasons to adopt small-scale steam turbine power systems on offshore rigs is the reduction in greenhouse gas emissions. By replacing diesel generators with more efficient systems powered by renewable energy and waste heat, the overall carbon footprint of offshore operations can be significantly reduced. The use of natural gas as a fuel for the steam turbine—compared to more carbon-intensive fuels—also helps minimize carbon emissions, aligning with both corporate sustainability goals and government regulations.
- Flaring Reduction: Flaring, a common practice in the oil and gas industry, is responsible for large volumes of methane and CO2 emissions. Small-scale steam turbines can help reduce flaring by utilizing associated natural gas or biogas as a fuel source, converting this otherwise wasted gas into useful energy. This reduction in flaring not only lowers emissions but also complies with increasingly stringent environmental regulations.
- Circular Economy Approach: Small-scale steam turbines can contribute to a circular economy approach by enabling offshore rigs to recycle energy in the form of waste heat. This approach maximizes the utility of every unit of fuel, minimizes waste, and reduces reliance on external fuel supplies, making offshore operations more sustainable and efficient in the long term.
Looking Ahead: Future Opportunities
As the offshore energy industry evolves and adapts to the needs of a more sustainable future, small-scale steam turbine systems will likely play an increasingly pivotal role. The industry is transitioning toward more integrated, renewable energy-powered platforms, and steam turbines—especially when combined with innovative technologies like heat recovery, AI, and hybrid systems—will be a central component of this shift. Furthermore, the development of hydrogen-powered turbines and zero-emission technologies may redefine the role of steam turbines in offshore power systems, opening up new avenues for environmentally friendly, cost-effective, and reliable energy generation.
In conclusion, small-scale steam turbine power plants offer significant advantages for offshore oil and gas rigs. Through improved energy efficiency, renewable energy integration, waste heat recovery, and AI-driven optimization, these systems help offshore operations achieve greater sustainability, cost-effectiveness, and energy security. As technology continues to advance, the future of offshore energy will be driven by cleaner, more efficient, and resilient energy solutions, with small-scale steam turbines at the forefront of this transformation.
Building on the advantages and innovations of small-scale steam turbine power systems for offshore oil and gas rigs, there are several additional strategic considerations and long-term benefits that continue to shape the evolution of these systems. These systems not only enable offshore operations to meet immediate energy needs but also position rigs to benefit from future technological advancements and environmental shifts in the energy sector. Below, we explore additional operational efficiencies, potential challenges, and opportunities for the integration of small-scale steam turbines in offshore environments.
Operational Efficiencies and Reliability
- Load Flexibility and Optimization: Small-scale steam turbines are inherently flexible in their ability to adjust their power output based on load demand, which is crucial in offshore oil and gas operations. Since energy demand on a rig can fluctuate due to varying operations, such as drilling, pumping, or water desalination, steam turbines can quickly adjust their output to match these changing demands, avoiding energy shortages or excess generation.
- Load-following Capability: Unlike large, centralized power generation systems, which often operate at a fixed output level, small-scale steam turbines have the ability to follow load demands. This is particularly important for offshore platforms where energy needs fluctuate throughout the day and during different operational phases. With this capability, the steam turbine systems can balance energy consumption more effectively and efficiently, contributing to overall cost savings.
- High Reliability and Durability: Offshore oil and gas rigs require highly reliable power systems, as any downtime or failure in power generation can have serious operational and safety implications. Steam turbines, especially when paired with advanced sensors, AI-driven monitoring systems, and predictive maintenance, are able to provide high reliability, ensuring that critical functions like lighting, drilling equipment, and safety systems are always powered.
- Redundancy Systems: Small-scale steam turbines can be equipped with redundancy systems to ensure that, even in the event of a failure or malfunction, power generation continues without significant disruptions. These systems can include backup turbines, parallel systems, and fail-safe mechanisms, all of which help maintain the uninterrupted operation of the rig, reducing the risk of downtime.
- Reduced Environmental Impact: Small-scale steam turbines have a much lower environmental impact compared to traditional diesel generators or large, conventional power plants. By utilizing renewable energy sources, waste heat recovery, or cleaner-burning fuels like natural gas, these turbines can significantly reduce the emission of harmful pollutants such as carbon dioxide (CO2), nitrogen oxides (NOx), and particulate matter.
- Minimized Flaring: One of the greatest environmental challenges in offshore oil and gas operations is gas flaring, which is the burning of excess natural gas that cannot be processed or transported. By using small-scale steam turbines powered by this excess gas, rigs can eliminate or significantly reduce flaring, minimizing environmental pollution and reducing the overall carbon footprint of operations.
- Carbon Capture and Utilization (CCU): In the future, steam turbines could be paired with carbon capture technologies to further reduce emissions. These technologies capture CO2 emissions from the power generation process and either store them underground or use them for other purposes, such as enhancing oil recovery or creating synthetic fuels. This additional step would make offshore operations even more environmentally friendly and aligned with global efforts to combat climate change.
Challenges and Considerations for Small-Scale Steam Turbines
While the advantages of small-scale steam turbine power plants are clear, there are also challenges that need to be addressed to ensure their successful implementation in offshore environments. These challenges include technical, logistical, and economic considerations that impact their adoption and long-term viability.
- High Initial Capital Investment: One of the primary barriers to the widespread adoption of small-scale steam turbines is the initial capital cost. The infrastructure required to install and maintain these systems can be expensive, particularly in offshore settings where space, access, and materials are limited. However, as technology continues to advance, and economies of scale are realized, the upfront investment costs are expected to decline.
- Financing Options: Offshore oil and gas companies may be able to take advantage of financing mechanisms such as government incentives, grants, and subsidies for renewable energy adoption and energy efficiency improvements. Furthermore, some rigs may benefit from cost-sharing partnerships with turbine manufacturers or renewable energy providers to help reduce initial investment barriers.
- Technical Integration with Existing Systems: Many offshore rigs are already equipped with older power generation systems, including diesel engines or larger gas turbines, which may present challenges when integrating new steam turbine power plants. The compatibility of these systems with steam turbines needs to be carefully assessed to ensure that the integration does not cause disruptions or inefficiencies.
- Retrofit and Integration Solutions: Fortunately, advances in modular systems and plug-and-play technology allow steam turbines to be more easily integrated into existing infrastructure. Offshore operators can consider a retrofit approach, gradually replacing or augmenting older power systems with smaller steam turbines over time, ensuring that the energy transition process is seamless and cost-effective.
- Maintenance Challenges in Harsh Offshore Environments: Offshore rigs present unique challenges for maintenance due to the harsh environmental conditions, including saltwater corrosion, high humidity, and extreme weather. Steam turbines, like any complex machinery, require regular maintenance and monitoring to ensure optimal performance. In the offshore context, this becomes more challenging due to the remote location and limited access to skilled technicians.
- Remote Monitoring and Predictive Maintenance: To mitigate this challenge, small-scale steam turbines on offshore rigs can be equipped with remote monitoring systems and predictive maintenance tools. By leveraging sensors, AI, and machine learning algorithms, operators can remotely monitor the health of turbines and identify potential issues before they result in costly breakdowns. This proactive approach to maintenance reduces the need for on-site inspections and can extend the life of the turbine systems.
- Fuel Supply and Availability: While natural gas is an attractive fuel for small-scale steam turbines, its availability and price fluctuations can pose challenges in certain offshore regions. In some locations, the infrastructure required to supply consistent, high-quality fuel may be lacking. Additionally, the transportation and storage of fuel on offshore rigs need to be managed carefully to avoid supply chain disruptions.
- Local Fuel Sources: In these cases, offshore rigs may need to rely on locally sourced fuels, such as associated gas from nearby oil production activities or biogas from waste processing. Additionally, hybrid systems that combine steam turbines with other renewable energy sources, such as wind or solar, can further mitigate the risk of fuel supply disruptions.
Opportunities for Growth and Innovation
As the offshore energy sector evolves, small-scale steam turbines will continue to offer substantial opportunities for growth, innovation, and technological advancements. Some key areas of future development include:
- Hydrogen-Powered Steam Turbines: The potential for hydrogen to serve as a clean fuel source for steam turbines is becoming more viable as the hydrogen economy grows. Hydrogen, when burned in a steam turbine, produces only water vapor as a byproduct, making it a zero-emission solution. Offshore rigs could eventually adopt hydrogen-powered turbines, either as a stand-alone solution or in combination with other energy sources.
- Green Hydrogen Production: Offshore rigs with access to renewable energy sources, such as offshore wind or solar power, could produce green hydrogen through electrolysis. This hydrogen could then be used to power steam turbines, offering a sustainable energy solution with minimal environmental impact.
- Energy-as-a-Service (EaaS): Offshore oil and gas companies may explore Energy-as-a-Service (EaaS) models, where power generation systems are outsourced to third-party service providers. This model would allow rigs to access advanced steam turbine systems and other energy technologies without the burden of ownership, operation, and maintenance. In turn, service providers would be incentivized to optimize energy efficiency and reduce costs for offshore operations.
- Advanced Grid Integration: As offshore rigs become more integrated into the broader offshore energy grid or microgrids, the role of steam turbines will expand beyond providing power to individual platforms. Small-scale steam turbines could form part of a distributed energy system, where multiple offshore rigs or platforms work together to stabilize the grid, manage energy resources efficiently, and share power.
- Grid Stabilization and Load Balancing: By integrating with offshore grids, steam turbines can provide grid stabilization services, smoothing out energy fluctuations caused by varying demand or renewable energy intermittency. Additionally, steam turbines could be used to store excess energy during times of low demand and release it when the grid requires additional support, improving overall energy security and reliability.
Conclusion
The future of small-scale steam turbine power plants in offshore oil and gas rigs is marked by technological innovation, enhanced energy efficiency, and environmental sustainability. As operators seek to balance energy security with cost-effectiveness and regulatory compliance, steam turbines provide a reliable, flexible, and scalable solution. By integrating renewable energy, waste heat recovery, and AI-driven optimization, small-scale turbines are poised to play a central role in the offshore sector’s transition toward cleaner, more efficient energy solutions.
Despite challenges such as high capital costs, technical integration, and maintenance complexities, the continued development of energy storage systems, hybrid technologies, and hydrogen-powered turbines will overcome these hurdles. As offshore platforms evolve to meet the demands of a low-carbon economy, small-scale steam turbines will be an essential component of this transformation, ensuring that offshore operations remain efficient, resilient, and sustainable in the years to come.
Small Scale Power Plant with a Steam Turbine for Private Islands
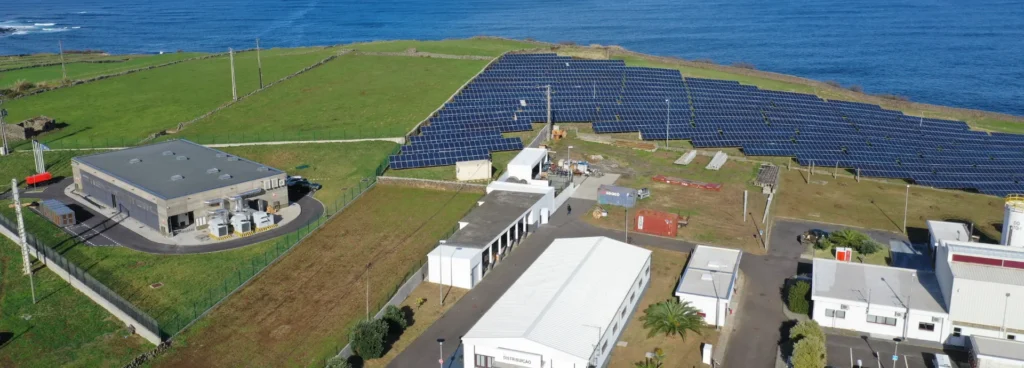
Small-scale power plants using steam turbines are becoming an increasingly popular solution for private islands, particularly those that are remote or off the grid. These islands often face challenges such as energy reliability, limited access to fuel supplies, high energy costs, and a need for sustainability. A steam turbine-based power system can address these challenges by providing a reliable, efficient, and flexible energy source. Here’s an overview of the potential benefits, design considerations, and operational factors for small-scale power plants with steam turbines on private islands.
Energy Requirements on Private Islands
Private islands typically have varying energy demands depending on the size of the island, the number of residents or visitors, and the nature of the activities conducted there. The energy needs can include residential power, lighting, air conditioning, desalination of water, and energy-intensive activities like pools, spas, and large kitchens. Given the limited infrastructure and resources available, it is important to design a power plant system that provides consistent, sufficient energy while minimizing environmental impact and operational costs.
Why Steam Turbines for Private Islands?
- Energy Efficiency: Steam turbines are known for their high efficiency, particularly when they are used in conjunction with waste heat recovery systems. By utilizing both thermal energy and waste heat from various sources, steam turbines can generate more power with less fuel consumption. This efficiency is especially valuable on private islands, where fuel might need to be transported by boat or plane, which increases costs and logistical challenges.
- Fuel Flexibility: One of the advantages of steam turbine systems is their fuel flexibility. Steam turbines can be powered by a variety of fuels, including biomass, natural gas, waste oils, or diesel. For private islands, this is a key benefit as it provides options for sustainable fuel sources. Biomass, for instance, can be produced locally from agricultural waste, wood, or organic materials. Additionally, waste heat from industrial processes, such as generators or desalination plants, can also be harnessed to fuel steam turbines, further improving energy efficiency and sustainability.
- Sustainability: With growing interest in green energy solutions, many private island owners are looking for sustainable power generation options that minimize their environmental footprint. Steam turbines, especially when paired with renewable energy sources (e.g., solar power, wind, or biogas), provide an environmentally friendly alternative to conventional fossil fuel-based power systems. On islands that produce their own biomass or are equipped with renewable energy resources, steam turbines can operate in a hybrid mode, using renewable sources when available and supplementing with conventional fuels when necessary.
- Grid Independence: For private islands that are not connected to a larger power grid, having an independent power generation system is crucial. A small-scale steam turbine-based power plant can act as a self-sustaining off-grid power system, ensuring that energy needs are met without relying on external suppliers. This is particularly important in remote locations where access to the mainland may be limited and the cost of importing energy is prohibitive.
Design and Technological Considerations
- Power Capacity and Scale: The size and capacity of the steam turbine system must be tailored to the island’s specific needs. For a small private island, a modular steam turbine system with a capacity of 100 kW to 500 kW may be sufficient to provide power to the island’s main facilities. However, larger islands or those with a high demand for power during peak seasons may require turbines capable of producing more power.
- Modular Turbines: Modular turbines offer the flexibility to scale up or down depending on the island’s energy demand. These systems can be designed with multiple smaller turbines that can be added or removed based on seasonal requirements or power consumption.
- Hybrid Power Systems: Combining a steam turbine with renewable energy sources (such as solar panels, wind turbines, or hydropower, if applicable) can create a hybrid system that reduces reliance on fossil fuels. During the day, when solar energy is abundant, the turbines can generate power using solar power while the steam turbine can be used during the night or when renewable energy production is insufficient.
- Energy Storage: To complement the hybrid system, battery storage can store excess energy generated by renewable sources, ensuring a continuous power supply even during periods of low solar or wind activity. This can reduce the need for backup diesel generators and lower operational costs.
- Desalination Integration: Many private islands rely on desalination plants to produce fresh water from seawater. Steam turbines can be particularly effective when integrated with desalination systems because the waste heat from the turbine can be used to power the reverse osmosis or distillation process of desalination, improving overall efficiency. This integration not only provides clean water but also reduces the operational costs associated with the energy-intensive desalination process.
- Waste Heat Recovery: In addition to generating electricity, steam turbines can be used to recover waste heat from other island operations, such as cooking, heating systems, or industrial processes. This waste heat can be used to pre-heat water or air, reducing the overall energy demand for these activities and enhancing the sustainability of the island’s energy system.
- Fuel Storage and Supply: For private islands relying on fuel-based systems, the design must include fuel storage tanks that are adequately sized to meet the island’s energy needs. The logistics of fuel supply and storage can be challenging on islands, and careful consideration must be given to the available space, safety, and access to fuel delivery. Biomass or waste fuels, if locally sourced, could also minimize reliance on imported fossil fuels.
Challenges of Steam Turbine Power Plants on Private Islands
- Logistical Challenges: Transporting and storing fuel on remote islands can be complicated and costly, especially if the island is located far from the mainland. Fuel supply chains need to be carefully planned to avoid interruptions, particularly during bad weather conditions when transportation can be delayed.
- High Initial Investment: Although steam turbine systems can offer long-term energy savings, the initial capital cost for installation can be high. The infrastructure required for a steam turbine, fuel supply, waste heat recovery, and integration with other systems (e.g., renewable energy) requires significant upfront investment. However, this cost is often offset over time through operational savings and the ability to avoid reliance on expensive external energy suppliers.
- Maintenance and Operation: Steam turbines, like any mechanical equipment, require regular maintenance to ensure optimal performance. Maintenance can be more challenging on private islands due to limited access to qualified technicians or spare parts. Remote monitoring systems and predictive maintenance tools can help mitigate this issue by allowing island operators to monitor the health of the turbine and schedule maintenance proactively.
- Environmental Impact: While steam turbines offer a cleaner alternative to traditional diesel generators, they still rely on fuel sources that may contribute to emissions and environmental degradation. Ensuring the sustainability of the power plant requires careful management of fuel sources, waste heat, and emissions. Hybridizing the system with renewable energy can help offset the environmental impact, but proper environmental management practices should still be in place.
Long-Term Financial and Sustainability Benefits
- Lower Operating Costs: Once the steam turbine power plant is in place, the ongoing operating costs are typically lower than other traditional energy sources, particularly if the system is hybridized with renewable energy. Fuel costs can be reduced by utilizing locally sourced biomass or waste fuels, and energy efficiency improvements through waste heat recovery and integration with desalination plants can further lower costs.
- Energy Independence: A small-scale steam turbine power plant provides significant energy independence, allowing private island owners to avoid the volatility of energy prices and supply disruptions from external suppliers. This can be especially beneficial in remote locations where external power is expensive or unreliable.
- Resilience and Reliability: In addition to cost savings, steam turbine systems provide greater reliability for private islands, ensuring that critical services like water desalination, lighting, and refrigeration continue to operate during power outages or fuel delivery delays. With the integration of backup energy storage and renewable energy, the power system can be designed to be both resilient and reliable.
- Sustainability and Reputation: As sustainability becomes a higher priority for many individuals and businesses, private islands that invest in green energy solutions such as steam turbines will be able to market themselves as environmentally responsible destinations or living spaces. This could be a valuable selling point, particularly as demand for eco-friendly and off-grid luxury properties grows.
Conclusion
A small-scale power plant with a steam turbine offers a practical, efficient, and sustainable energy solution for private islands. These systems provide energy independence, cost savings, and environmental benefits, making them ideal for remote or off-grid locations. By using flexible fuels, incorporating renewable energy sources, and integrating with desalination systems, steam turbines can help meet the diverse energy needs of private islands. Despite initial investment costs and logistical challenges, the long-term financial and sustainability benefits make steam turbines a strong contender for private island energy solutions in the future.
Building on the advantages of small-scale steam turbine power plants for private islands, several further considerations and advancements enhance their appeal and viability for remote island operations. Let’s explore some of the technical, operational, and long-term perspectives that can maximize the effectiveness of these systems.
Integration with Renewable Energy Sources
- Solar and Wind Power Hybridization: One of the most effective ways to increase the sustainability of a steam turbine system on a private island is by integrating renewable energy sources, particularly solar and wind power. These sources can work in tandem with the steam turbine, reducing reliance on fossil fuels or biomass and providing a cleaner energy mix.
- Solar Power: Solar photovoltaic (PV) panels are an excellent match for private island environments, where sunlight is abundant. Solar energy can be harvested during the day when energy demand is high, and the steam turbine can supplement energy needs during the night or when solar power is insufficient.
- Wind Power: Wind turbines can be particularly useful in areas where wind is a consistent resource. By combining wind and solar power with the steam turbine, islands can build a resilient and reliable power system that can function independently of external supply lines.
- Energy Storage Solutions: To ensure continuous and stable power supply on a private island, energy storage is crucial. Solar and wind power can be intermittent, so batteries or thermal storage systems, such as molten salt storage (used for concentrating solar power plants), can store excess energy generated during peak hours for later use.
- Battery Storage: Lithium-ion or advanced flow batteries can store energy from solar or wind power during surplus generation periods. This energy can then be discharged when the demand for electricity exceeds renewable generation, ensuring a stable supply.
- Thermal Energy Storage: Another option for integrating renewable energy is thermal energy storage. When solar or wind power is available, excess energy can be used to heat a thermal storage medium (e.g., molten salt). This stored thermal energy can then be converted into electricity using the steam turbine during periods of low renewable generation.
- Optimization of Energy Generation: Combining multiple renewable sources with steam turbines and storage systems allows for load balancing. When one system (like solar) is underperforming, the other (wind) can compensate, while steam turbines provide base-load power and manage peak demands. The result is a resilient, flexible, and efficient energy network that ensures a constant supply of electricity for all island needs.
Waste Management and Biomass Utilization
- Biomass as Fuel Source: For private islands with access to organic waste or agricultural byproducts, biomass can serve as an effective and sustainable fuel source for steam turbines. Biomass can be derived from various local resources such as wood, crop residue, animal waste, or even algae, which can be processed into biomass pellets or used directly in combustion chambers to generate steam.
- Waste-to-Energy Solutions: Waste-to-energy (WTE) technologies, which utilize organic waste to generate electricity, align well with steam turbine systems. Islands often produce substantial amounts of waste, especially in tourist-heavy environments. Instead of landfilling organic waste, these materials can be converted into renewable energy via combustion or anaerobic digestion processes.
- Local Circular Economy: Biomass utilization aligns with the circular economy, promoting sustainability on the island. Organic waste and local resources that would otherwise be discarded can instead be used to generate electricity, reducing dependence on external fuel sources and lowering waste disposal costs.
- Integration with Wastewater Treatment: Another innovative approach is to use biogas from wastewater treatment or from organic waste processing to fuel the steam turbine. Biogas, produced by the anaerobic digestion of organic waste, can be purified and converted into methane. This methane can then be used to fuel the steam turbine, providing both renewable energy and addressing waste disposal challenges.
- Anaerobic Digesters: These digesters can be implemented on the island to break down organic waste and produce biogas, reducing landfill use while providing a consistent, renewable source of energy for the steam turbine.
- Sustainability and Self-Sufficiency: Islands that are committed to sustainable living can establish closed-loop systems where the energy and waste management systems are tightly integrated. Biomass and waste-to-energy solutions can be used to generate power while addressing waste disposal and land management challenges, ensuring the island operates sustainably with minimal outside input.
Reliability and Maintenance Considerations
- Remote Monitoring and Predictive Maintenance: One of the key challenges in operating a power plant on a private island is the remoteness of the location. Access to qualified technicians and spare parts can be limited, leading to potential delays in maintenance. To mitigate this, advanced remote monitoring systems and predictive maintenance technologies can be used to keep the steam turbine system running optimally.
- Condition-Based Monitoring: With the use of IoT sensors, turbines can be monitored in real-time for factors such as temperature, vibration, and pressure. This data can be analyzed to predict when maintenance is needed, allowing operators to take action before an issue escalates into a failure.
- Remote Diagnostics: Remote diagnostics allow off-site technicians or service providers to assist in troubleshooting turbine issues, perform software updates, and monitor performance metrics, which can extend the operational life of the equipment.
- Self-Sufficiency in Maintenance: For private islands that prefer to remain entirely self-sufficient, operators can receive specialized training in turbine maintenance and operation. This can include everything from daily checks to more advanced troubleshooting, ensuring that island staff are well-equipped to handle basic maintenance tasks without the need for external help.
- Modular Systems for Ease of Repair: Another benefit of small-scale steam turbines is their modular nature. Components of the system can be easily replaced or repaired, minimizing downtime. Instead of waiting for an entire turbine overhaul, island operators can quickly swap out damaged parts, ensuring the continued operation of the power plant.
- Redundancy Systems: To further enhance reliability, a redundant power system can be set up. This involves having a second smaller steam turbine or backup power generation system in place that can take over in the event of a turbine malfunction or planned maintenance. This ensures there is no interruption in power supply, particularly for critical systems such as lighting, desalination, and refrigeration.
Cost and Economic Viability
- Initial Capital Costs: One of the primary challenges for private islands considering small-scale steam turbines is the capital cost associated with installation. The costs of turbine equipment, fuel systems, renewable energy infrastructure, and energy storage solutions can be significant. However, the long-term operational savings and energy independence typically offset the initial investment.
- Financing Options: Islands may explore financing options, such as energy-as-a-service (EaaS) models or power purchase agreements (PPAs), where third-party providers install and maintain the power system in exchange for a fixed fee over time. These models lower upfront capital costs and allow islands to manage their energy costs more predictably.
- Operational Savings: Over time, small-scale steam turbine power plants can significantly reduce fuel costs. By utilizing local resources, waste heat, and renewable energy, private islands can lower their reliance on expensive, imported fossil fuels. The hybrid nature of the systems ensures they run efficiently, providing cost savings for energy generation and reducing the need for backup diesel generators, which are costly and polluting.
- Return on Investment (ROI): Over the lifespan of the steam turbine system, owners can expect a favorable return on investment due to reduced energy costs and enhanced energy reliability. Additionally, utilizing renewable sources and waste-to-energy technologies can provide positive public relations opportunities for private islands committed to sustainability and eco-tourism.
- Tourism and Branding Opportunities: For private islands used as luxury vacation destinations, promoting the use of green energy and sustainable practices can provide a significant marketing advantage. Eco-conscious travelers may be attracted to islands that prioritize environmental sustainability, helping operators position their islands as leaders in responsible tourism.
Conclusion
Small-scale steam turbine power plants are a promising solution for private islands looking to achieve energy independence, sustainability, and operational efficiency. By integrating renewable energy sources like solar and wind, utilizing biomass and waste-to-energy solutions, and implementing energy storage systems, private islands can create a reliable, flexible, and environmentally friendly power system. While the upfront costs and logistical challenges of installing and maintaining steam turbines can be significant, the long-term economic and sustainability benefits make them a valuable investment for island owners seeking to reduce their carbon footprint, ensure energy security, and enhance their island’s attractiveness as a self-sustaining, eco-conscious destination. With the proper integration of technologies and effective maintenance strategies, steam turbine systems can provide an optimal energy solution for private islands, enhancing their autonomy and long-term viability.
Continuing from the previous discussion, small-scale steam turbine power plants offer additional benefits and advancements that can enhance the efficiency, resilience, and sustainability of power generation on private islands. Let’s explore the operational strategies, technological improvements, and considerations for further optimizing these systems.
Operational Strategies for Small-Scale Steam Turbine Power Plants
- Energy Management Systems (EMS): To optimize the operation of a steam turbine-based power plant, private islands can benefit from implementing a sophisticated Energy Management System (EMS). EMS software integrates all energy-producing and energy-consuming systems on the island, ensuring optimal performance and energy distribution.
- Dynamic Load Distribution: The EMS ensures that energy generation from the steam turbine, solar, wind, or any backup system is used in the most efficient manner possible. The system can also prioritize energy sources based on availability and cost—giving preference to renewable energy when it’s available, and switching to steam turbine power during periods of low renewable output.
- Demand Response: The EMS can adjust power consumption based on demand fluctuations throughout the day, ensuring that the system remains stable even during peak load times. By managing peak loads through demand response, the plant can avoid overloading the system or excessive fuel consumption.
- Microgrid Implementation: A small-scale steam turbine power plant can be part of a microgrid. A microgrid is a localized energy network that can operate independently from the larger grid, providing a self-sufficient solution for islands. Microgrids are particularly advantageous because they can seamlessly switch between operating as part of a larger grid or in island mode, depending on the availability of external resources.
- Grid-Tied or Off-Grid Operation: For islands that are connected to a mainland grid, the microgrid system can be designed to work in tandem with the grid, drawing power when needed and feeding excess electricity back to the grid during periods of high generation. For completely isolated islands, the microgrid operates autonomously, with the steam turbine acting as a central power source alongside renewable energy systems.
- Resilience to External Grid Failures: If the island is connected to a larger grid and experiences outages, the microgrid ensures uninterrupted power by switching to local generation, ensuring the island remains powered without reliance on mainland infrastructure.
- Flexible Operation Modes: The operational flexibility of small-scale steam turbine plants makes them ideal for islands with fluctuating energy demand. Islands that experience seasonal surges in power needs (such as during peak tourist seasons) can design their steam turbine systems to ramp up or down based on demand.
- Seasonal Adaptability: The ability to adjust turbine output based on seasonal fluctuations in power consumption is crucial. During off-peak seasons, when fewer visitors are present, the steam turbine system can run at lower capacity, conserving fuel and resources. In contrast, during peak seasons, the system can be configured to generate higher outputs, meeting the increased demand efficiently.
- Load Shedding: In scenarios where power demand exceeds generation capacity, load shedding can be implemented to prioritize essential services (e.g., water desalination, lighting, and refrigeration) while temporarily cutting power to non-essential areas.
Technological Advancements for Steam Turbines on Private Islands
- Advanced Steam Turbine Designs: Modern steam turbines have undergone significant advancements in efficiency and size, making them more suitable for small-scale applications like those on private islands.
- Compact and Modular Turbines: Advances in turbine design have led to more compact, modular units that are easier to transport and install on private islands with limited space. These units can be scaled up or down based on the island’s power requirements, and some models are specifically optimized for low-pressure and low-temperature applications, which are more common in small-scale operations.
- High-Efficiency Turbines: Efficiency improvements in steam turbines, such as multi-stage turbines and regenerative cycles, allow these systems to extract more energy from the same amount of fuel, making them more cost-effective over time. These improvements are especially beneficial on islands where fuel supply is limited or costly.
- Waste Heat Recovery Innovations: Leveraging waste heat from the steam turbine operation is one of the most effective ways to boost the overall system efficiency on private islands.
- Combined Heat and Power (CHP): A CHP system, also known as cogeneration, allows the steam turbine to generate both electricity and useful heat. This excess heat can be used for hot water generation, space heating, or industrial processes (e.g., food production, laundry, or desalination). Islands with residential facilities or high-demand services like spas and restaurants can benefit from CHP, as it helps meet both electrical and thermal energy needs simultaneously.
- Organic Rankine Cycle (ORC): The ORC system is a technology designed to recover waste heat from lower-temperature sources (below 400°C), making it suitable for use with small-scale steam turbines. In this system, waste heat is converted into additional electricity, improving the system’s overall efficiency. Islands with limited fuel sources or reliance on waste heat recovery systems can benefit from ORC, enabling them to extract more energy from existing infrastructure.
- Integration with Battery Storage Systems: To complement the steam turbine’s base-load power generation, advanced battery storage systems can be integrated into the power plant setup. These systems can store excess power generated during periods of low demand or when renewable energy is available and discharge it during high-demand periods.
- Reducing Diesel Dependence: Battery storage not only provides backup energy during peak loads but also helps reduce the reliance on diesel generators. By using batteries to balance the demand-supply gap, private islands can significantly reduce their carbon footprint and avoid the operational costs associated with diesel backup systems.
- Smart Energy Management: The combination of battery storage and energy management systems allows for smart grid functionalities on the island, improving the flexibility and reliability of power generation and ensuring that stored energy is used when most needed.
- Desalination Plant Optimization: The integration of steam turbines with desalination plants on private islands is a powerful combination. Desalination often requires significant amounts of energy, and using steam turbine waste heat or cogeneration can significantly improve the efficiency of these operations.
- Thermal Desalination: In thermal desalination methods such as multi-effect distillation (MED) or multi-stage flash distillation (MSF), the waste heat from the steam turbine can be directly used to produce freshwater. This integration makes the power plant more than just an electricity generator—it becomes a sustainable water production facility as well.
- Reverse Osmosis Integration: Even in reverse osmosis (RO) desalination systems, the energy demands for high-pressure pumps can be reduced with heat recovery or low-energy desalination technologies, enhancing the overall sustainability of the island’s water supply system.
Long-Term Benefits and Future Prospects
- Sustainable Development Goals (SDGs): Many private islands are increasingly committed to contributing to global sustainability goals. By adopting steam turbine-based power plants, islands can demonstrate their commitment to energy efficiency, climate action, and responsible consumption (SDG 7, 13, and 12). These plants not only reduce the island’s carbon footprint but also provide a model of self-sufficiency and sustainability that can be adopted by other island communities or businesses.
- Technological Leadership and Innovation: As private island owners continue to prioritize sustainability, the use of small-scale steam turbines combined with renewable energy will position them as leaders in technological innovation. Their ability to integrate cutting-edge energy solutions sets a precedent for other remote or off-grid communities to follow, influencing future developments in sustainable energy practices.
- Reputation and Eco-Tourism: For private island owners focusing on eco-tourism, the use of renewable energy and green technologies like steam turbines will serve as a powerful marketing tool. Eco-conscious travelers will be more likely to choose destinations that prioritize sustainability, clean energy, and responsible environmental management. As demand for eco-tourism continues to grow, private islands investing in renewable energy systems will enjoy a competitive edge in the hospitality and leisure market.
- Scalability and Expansion: Small-scale steam turbine power plants on private islands are not just limited to current energy needs but can also be scaled to accommodate future growth. As tourism increases or infrastructure expands, the steam turbine system can be upgraded with additional turbines, energy storage, and renewable energy sources. This scalability ensures that islands can future-proof their power generation systems, avoiding costly upgrades or disruptions.
Conclusion
Small-scale steam turbine power plants offer a highly adaptable and sustainable solution for energy generation on private islands. The combination of steam turbines with renewable energy sources, energy storage, and waste heat recovery creates a flexible, resilient, and eco-friendly power system that can meet the unique demands of island environments. As technology continues to improve, these systems will become even more efficient and cost-effective, further enhancing the appeal of small-scale steam turbine power plants for private islands. With a focus on long-term sustainability, cost savings, and operational efficiency, private island owners can ensure that their energy needs are met while contributing to global sustainability goals, making their islands not just isolated getaways, but models of self-sufficient, green living.
Building on the previous points, further considerations can help refine the implementation, operation, and future-proofing of small-scale steam turbine power plants for private islands. These additional strategies focus on enhancing resilience, reducing operational complexity, and expanding energy solutions to ensure long-term sustainability.
Energy Security and Resilience
- Microgrid Resilience and Disaster Recovery: Islands are often vulnerable to natural disasters, including hurricanes, floods, and seismic events. Therefore, ensuring the resilience of the energy system is a key consideration when implementing a small-scale steam turbine power plant.
- Robust Microgrid Design: A well-designed microgrid can provide the flexibility to continue functioning during island-wide power outages or natural disasters. With an integrated islanding capability, the microgrid can disconnect from the mainland grid during emergency situations and operate independently, ensuring critical systems like healthcare, water supply, and emergency services remain powered.
- Redundancy and Backup Systems: In addition to a steam turbine’s primary function, adding redundant systems, such as a backup generator or auxiliary turbine, can ensure that there is no disruption to power during unexpected circumstances. Redundancy can be crucial for keeping power flowing to essential services, especially when external systems are unavailable.
- Climate Resilience with Green Energy: As climate change continues to affect island ecosystems, integrating green energy technologies into the power plant design improves resilience to climate impacts. By reducing dependence on fossil fuels and external grid infrastructure, private islands are less susceptible to disruptions caused by price fluctuations, political instability, or natural resource depletion.
- Solar Panels and Wind Turbines: Leveraging solar and wind power on islands offers more predictable and renewable energy sources, especially in areas where sunlight and wind are abundant year-round. A hybrid power generation system with steam turbines can reduce the carbon footprint and contribute positively to the island’s climate resilience efforts.
- Battery Storage and Load Balancing: By incorporating battery storage systems, islands can ensure that renewable energy generated during favorable weather conditions (such as sunny or windy periods) can be stored for use during adverse weather or low-generation periods. This helps in stabilizing the grid and reducing reliance on fossil fuel-based backup power.
Operational Efficiency Improvements
- Advanced Control Systems and Automation: Incorporating advanced control systems for managing steam turbine operations can enhance both operational efficiency and the ability to respond quickly to changes in energy demand. These systems can automatically adjust turbine output based on real-time data about energy consumption, weather conditions, and renewable generation levels.
- Automated Load Distribution: By integrating real-time data from renewable energy sources (such as solar and wind) and storage systems, advanced control systems can dynamically adjust the output from the steam turbine to meet demand without overshooting or wasting resources. This reduces fuel consumption, maximizes efficiency, and helps keep operational costs low.
- Predictive Analytics: By using predictive analytics and machine learning, the system can analyze historical data to forecast energy demand and adjust turbine operations accordingly. This predictive capability allows for smarter scheduling of turbine runs, ensuring that power is produced efficiently when needed and preventing unnecessary wear on machinery.
- Energy Efficiency Upgrades: Over time, there may be opportunities to further increase the efficiency of the steam turbine plant by retrofitting it with new technologies.
- Supercritical and Ultra-Supercritical Steam Cycles: Traditional steam turbines operate on subcritical steam cycles, but transitioning to supercritical or ultra-supercritical steam cycles can offer significant improvements in thermal efficiency. By increasing steam pressure and temperature, these systems extract more energy from the same fuel, lowering fuel consumption and reducing operating costs.
- Heat Exchanger Technologies: Advanced heat exchangers can be incorporated to optimize the transfer of waste heat from the turbine to other parts of the system (such as water heaters or desalination plants). This would further enhance the overall energy efficiency of the plant and reduce the need for external energy inputs.
Sustainability and Eco-Friendly Power Generation
- Circular Economy through Waste Management: One of the main advantages of a small-scale steam turbine power plant on a private island is the ability to implement circular economy principles by utilizing waste for power generation. This can significantly reduce the environmental impact while contributing to sustainability goals.
- Organic Waste for Biomass: Private islands, especially those with agricultural operations, can produce significant amounts of organic waste, such as crop residues, food waste, and animal manure. By converting this waste into biomass or biogas, islands can fuel their steam turbines with locally sourced, renewable materials, avoiding the need for imported fossil fuels.
- Waste-to-Energy Systems: A waste-to-energy (WTE) facility can be designed to convert the island’s waste into energy. Instead of sending waste to landfills, the organic portion can be used to produce energy via combustion or anaerobic digestion. The use of such technology turns waste into a valuable resource, generating both electricity and heat for the island.
- Low-Carbon Footprint: By investing in steam turbines powered by renewable resources or biomass, private islands can achieve a low-carbon footprint and avoid dependence on high-emission energy sources, such as diesel or coal. A focus on reducing greenhouse gas emissions aligns with both local and global sustainability goals, helping to mitigate climate change and contribute to cleaner energy solutions.
- Carbon Credit Opportunities: Islands adopting green energy technologies such as steam turbines powered by renewable energy may also be eligible for carbon credits or incentives under various international carbon trading schemes. These credits could provide additional revenue streams for island owners while further supporting the move toward sustainability.
Long-Term Growth and Future-Proofing
- Scalable Energy Solutions: As the needs of private islands evolve over time, steam turbine power plants can be scaled to meet growing energy demands, whether from increased residential needs, tourism, or new facilities (e.g., hotels, restaurants, or water sports complexes).
- Modular Expansion: The modular nature of small-scale steam turbines allows for future upgrades. As the island’s energy requirements increase, additional turbines can be added, or the existing systems can be upgraded with newer, more efficient technology. This scalability ensures that the power plant can grow with the island while maintaining energy independence and sustainability.
- Technology Upgrades: The steam turbine industry continues to evolve with new advancements in efficiency, fuel types, and hybrid technologies. Staying up-to-date with the latest developments ensures that the island’s power systems remain efficient, cost-effective, and environmentally friendly.
- Emerging Technologies: Over the next few decades, private islands can leverage emerging technologies to further enhance the sustainability and efficiency of their energy systems.
- Hydrogen Power: With the growing interest in green hydrogen, steam turbines could potentially be adapted to run on hydrogen, produced through electrolysis using renewable energy. Hydrogen could serve as both an energy storage medium and a clean fuel source for steam turbines, further decarbonizing the energy mix.
- Carbon Capture and Storage (CCS): For islands using biomass or waste-to-energy systems, implementing carbon capture and storage could be an option to reduce the environmental impact of power generation. By capturing carbon emissions from the combustion process and storing them underground or using them for other purposes, islands could achieve net-zero emissions from their power systems.
- Economic Viability and ROI: Over time, the combination of reduced energy costs, renewable energy integration, and potentially new revenue streams from carbon credits, eco-tourism, or even energy exports (if feasible) can lead to a high return on investment (ROI) for steam turbine-based power plants.
- Cost Recovery through Energy Savings: In the long term, the savings generated by reduced dependence on fossil fuels and improved operational efficiency lead to a faster return on investment. As fuel prices fluctuate and external supply lines become more unpredictable, the stability of a local power system provides a cost-effective energy solution.
- Tourism and Branding: As sustainability and green technologies continue to be significant selling points in the travel industry, private islands can attract eco-conscious tourists by showcasing their energy self-sufficiency. This can position the island as a destination that not only offers luxury and privacy but also provides an environmentally responsible vacation experience.
Conclusion
In conclusion, small-scale steam turbine power plants for private islands represent a sophisticated and sustainable solution for off-grid energy generation. By leveraging advanced technologies, renewable energy integration, energy storage, and waste-to-energy solutions, these plants can provide islands with reliable, clean, and cost-effective power. Future-proofing the system with scalability, emerging technologies like hydrogen and carbon capture, and incorporating automated systems for optimal operation will ensure these power plants remain efficient, resilient, and sustainable for years to come. With growing interest in sustainability and eco-tourism, small-scale steam turbine power systems are poised to be a cornerstone of future energy solutions for private islands, contributing to both energy independence and environmental stewardship.
Small Scale Power Plant for Petrochemical Plants
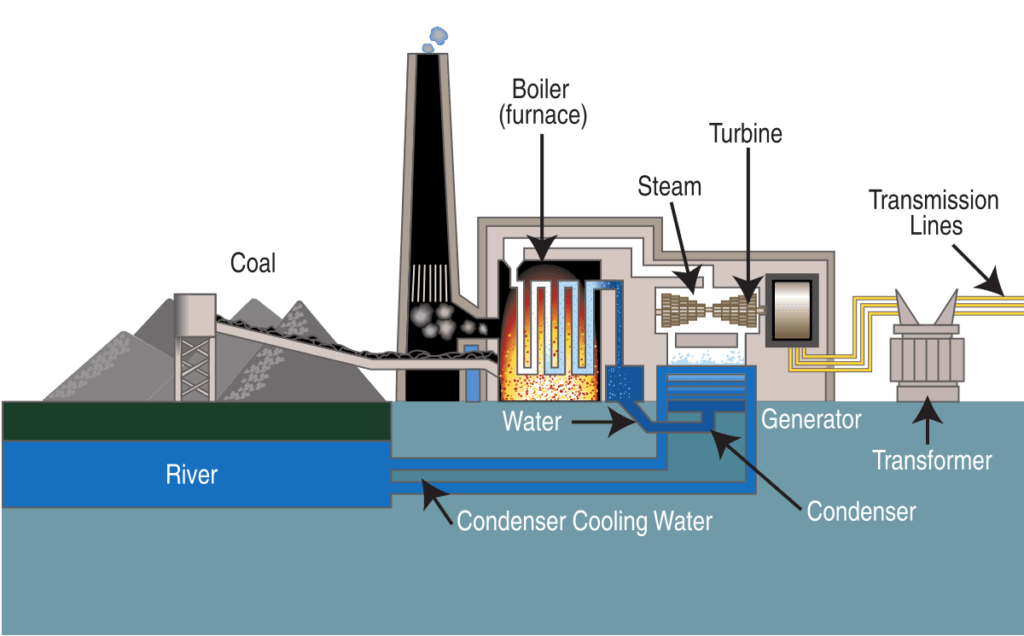
Small-scale power plants for petrochemical plants, particularly those using steam turbines, are vital for ensuring reliable and efficient energy supply in an energy-intensive industry like petrochemicals. These power plants must meet the high demands of the petrochemical production process while minimizing costs, maximizing energy efficiency, and aligning with sustainability goals. Here’s a comprehensive overview of how steam turbine-based power systems can optimize the energy needs of petrochemical plants.
Energy Demand in Petrochemical Plants
Petrochemical plants operate continuously and require substantial amounts of energy for their chemical processing operations, including cracking, distillation, separation, and polymerization. These processes often consume energy in the form of steam, electricity, and heat, making an efficient energy management system a crucial aspect of plant operation.
Role of Steam Turbines in Small-Scale Power Plants
Steam turbines play a central role in ensuring that petrochemical plants can meet their significant energy requirements. They offer a reliable, cost-effective, and scalable solution for generating electricity and providing steam for industrial processes. The integration of a steam turbine power plant into a petrochemical plant’s energy infrastructure can significantly improve efficiency, reduce reliance on external power grids, and contribute to long-term sustainability.
- Cogeneration (Combined Heat and Power, CHP): One of the key advantages of steam turbines in petrochemical plants is their ability to operate in cogeneration mode, where the steam produced by the turbine can be used both for electricity generation and for heating or industrial processes.
- Steam Utilization: In petrochemical plants, steam is often required for a wide range of processes such as distillation, cracking, and dehydration. By integrating steam turbines into the plant’s operations, the steam produced can be directly used in these processes, maximizing the efficiency of the entire energy generation system. This integration of power generation and thermal energy usage reduces waste and optimizes energy consumption.
- Efficiency Gains: Cogeneration systems can reach energy efficiencies of up to 80%, much higher than traditional power plants that only generate electricity. This makes them highly beneficial in the energy-intensive petrochemical industry, where reducing energy consumption is key to improving profitability and sustainability.
- Energy Independence and Cost Reduction: Petrochemical plants are often located in remote areas, where access to the grid may be limited or unreliable. A small-scale steam turbine power plant can provide these plants with energy independence, ensuring a continuous supply of power without reliance on external energy sources.
- Reduced Grid Dependency: A locally generated power system allows petrochemical plants to mitigate risks associated with grid failures or energy price fluctuations. By generating their own electricity through steam turbines, plants can avoid reliance on external grid systems that may be unreliable or subject to pricing volatility.
- Cost Savings: Operating a steam turbine-based power plant reduces the costs associated with purchasing electricity from the grid. Additionally, by utilizing waste heat from the turbine and incorporating renewable energy solutions, the plant can further reduce fuel consumption and improve overall operational cost-efficiency.
- Modular and Scalable Power Generation: Small-scale steam turbine power plants are inherently scalable, which is beneficial for petrochemical plants that may need to expand their production capacity over time.
- Modular Design: The design of small-scale power plants allows for the addition of turbines as the plant’s energy needs grow. This modularity ensures that the plant can adjust its energy generation capacity in response to increases in demand or changes in production volumes.
- Flexible Power Supply: Depending on the plant’s operational requirements, steam turbines can be used to provide both base load and peak load power. This flexibility makes it easier to meet varying levels of energy demand throughout the day, avoiding inefficiencies that arise from overproduction or underproduction.
Integrating Renewable Energy for a Hybrid System
Incorporating renewable energy sources such as solar, wind, or biomass into a small-scale power plant can enhance sustainability and reduce the overall environmental impact of a petrochemical plant. This is particularly relevant as industries increasingly focus on reducing their carbon footprint.
- Solar and Wind Energy Integration: As many petrochemical plants are large facilities with substantial land areas, integrating solar photovoltaic (PV) or wind energy into the power generation mix can provide a significant portion of the energy required by the plant.
- Energy Supplementation: Solar and wind power can supplement the steam turbine power plant, reducing the need for fossil fuels and decreasing carbon emissions. The energy produced during the day by solar panels, for example, can be used to power auxiliary systems in the petrochemical plant, while the steam turbine can focus on providing the main electricity and steam for industrial processes.
- Hybrid Power Generation: By combining renewable energy with a steam turbine power plant, petrochemical plants can create a hybrid energy system that leverages the strengths of both sources. The flexibility of the steam turbine allows it to balance out the intermittent nature of solar and wind energy, providing reliable power generation when renewable sources are unavailable due to weather or time of day.
- Biomass or Waste-to-Energy Systems: Biomass is another renewable energy source that can be used to fuel steam turbines, providing a sustainable and low-carbon option for energy generation. Petrochemical plants that produce organic waste or have access to biomass resources can use this material as a feedstock for their power plants.
- Waste Utilization: Biomass or waste-to-energy systems can turn plant waste, such as plastic by-products, agricultural residues, or other organic materials, into energy. This waste-to-energy approach not only provides a renewable energy source but also helps reduce the environmental impact of the petrochemical plant by diverting waste from landfills and reducing greenhouse gas emissions.
- Energy from Waste Heat: In addition to biomass, steam turbines can also capture and use waste heat from chemical processes. The integration of waste heat recovery systems can significantly improve the overall efficiency of the power plant, further reducing the need for additional fuel.
Optimizing Plant Operations with Smart Technology
- Automation and Control Systems: Advanced automation and control systems are essential for optimizing the operation of small-scale steam turbine plants in petrochemical facilities. These systems monitor and adjust turbine output, heat generation, and power distribution in real-time to ensure maximum efficiency.
- Predictive Maintenance: Predictive analytics can help monitor the health of the steam turbine, alerting plant operators to potential issues before they lead to failure. By reducing unplanned downtime, the plant can operate more efficiently and avoid costly repairs.
- Energy Management Software: Integrating energy management software (EMS) allows for better forecasting, scheduling, and optimization of energy use. The software can track energy consumption patterns and adjust operations to balance power production from steam turbines and renewable sources, ensuring that the plant runs at peak efficiency.
- Integration with Process Control Systems: In petrochemical plants, power systems must be closely integrated with the plant’s process control systems to ensure that energy is being used efficiently and in sync with production demands.
- Dynamic Load Adjustment: The steam turbine’s output can be dynamically adjusted based on the energy requirements of the petrochemical plant’s operations. For instance, when energy demand spikes during certain phases of production, the steam turbine can ramp up its output accordingly.
- Real-Time Monitoring: By continuously monitoring key parameters like pressure, temperature, and flow rate, advanced sensors and control systems ensure that steam generation and electricity production remain at optimal levels.
Environmental and Sustainability Considerations
- Carbon Capture and Storage (CCS): Petrochemical plants, especially those relying on fossil fuels, have significant carbon emissions. Integrating carbon capture technologies with the small-scale steam turbine power plant can help reduce the plant’s environmental impact by capturing and storing CO2 emissions.
- CCS Technologies: By implementing CCS technologies, petrochemical plants can capture carbon dioxide generated by the steam turbine and other plant processes, reducing their overall greenhouse gas emissions. This aligns with both corporate sustainability goals and international regulatory frameworks for emissions reduction.
- Sustainable Industrial Processes: Through the use of clean and renewable energy sources (such as biomass or waste-to-energy), along with the adoption of CCS, petrochemical plants can significantly reduce their carbon footprint and contribute to more sustainable industrial practices.
- Efficient Water Use: Steam turbines in petrochemical plants often rely on water cooling systems, which can consume large amounts of water. Utilizing more efficient cooling technologies, such as air-cooled condensers or dry cooling, can help minimize water usage and reduce the plant’s environmental impact, particularly in water-scarce regions.
- Water Reuse: Petrochemical plants can implement water recycling or reuse systems that allow water used in the steam turbine cooling process to be purified and used again, reducing the plant’s water consumption and minimizing waste.
Long-Term Benefits and Future Prospects
- Cost-Effectiveness: The long-term cost savings offered by small-scale steam turbine power plants, particularly through cogeneration, waste heat recovery, and renewable energy integration, make them an attractive solution for petrochemical plants looking to reduce operational costs.
- Sustainability Goals and Eco-Branding: As the petrochemical industry faces increasing pressure to adopt more sustainable practices, having a small-scale steam turbine power plant integrated with renewable energy sources can significantly enhance a company’s reputation. Petrochemical plants can market themselves as leaders in sustainable industrial practices, drawing attention from investors, regulators, and consumers who prioritize environmental responsibility.
- Future Scalability: As global energy systems transition toward renewable energy, small-scale steam turbine power plants can be adapted to run on green hydrogen, biofuels, or other low-carbon fuels. This flexibility ensures that petrochemical plants can remain aligned with future energy trends and continue to reduce their carbon emissions over time.
Conclusion
Small-scale steam turbine power plants offer an effective and sustainable solution for the energy needs of petrochemical plants. Through cogeneration, integration with renewable energy sources, and the use of advanced automation systems, these plants can optimize energy efficiency, reduce costs, and minimize environmental impact. With a growing focus on sustainability and carbon reduction, steam turbine power plants provide petrochemical companies with a robust energy solution that supports both operational goals and long-term sustainability initiatives.
Building on the previous points, further elaboration on the integration of small-scale steam turbine power plants for petrochemical facilities can help identify specific areas of focus, such as fuel flexibility, energy storage, and regulatory alignment. These factors will enhance the plant’s operational flexibility, economic feasibility, and environmental compliance, ensuring long-term success and growth.
Fuel Flexibility and Fuel Management
- Alternative Fuels and Hybrid Systems: Petrochemical plants often face fluctuating fuel prices and supply issues, so using a steam turbine that can operate on different fuels provides valuable flexibility. In addition to traditional fuels like natural gas or diesel, steam turbines can be adapted to utilize biofuels, hydrogen, or syngas produced from waste materials or biomass.
- Biofuels: By incorporating biofuels into the steam turbine’s fuel mix, petrochemical plants can reduce their reliance on fossil fuels, lower carbon emissions, and tap into renewable energy sources. Biofuels, such as plant-based oils or algae-derived fuels, can be used as substitutes for conventional petroleum products, making them a sustainable and economically viable option.
- Hydrogen and Syngas: As the energy sector transitions to cleaner fuels, steam turbines can be adapted to use hydrogen or syngas produced from renewable sources (e.g., waste biomass, electrolysis). These fuels help reduce the carbon intensity of the power plant and align with global sustainability goals.
- Waste-to-Energy: Petrochemical plants with access to significant amounts of waste materials—such as industrial by-products, plastics, or agricultural waste—can use waste-to-energy systems that convert these materials into fuel for steam turbines. This reduces landfill waste while providing a renewable source of energy.
- Fuel Storage Solutions: For facilities operating in remote areas or dealing with fuel supply uncertainties, the integration of fuel storage systems becomes crucial. Having dedicated fuel reserves for steam turbines ensures uninterrupted power generation even during supply disruptions.
- Strategic Fuel Reserves: Establishing on-site fuel reserves allows petrochemical plants to operate independently during times of fuel shortage or supply chain disruption. This strategy ensures that the plant remains operational without significant interruptions, especially when dealing with time-sensitive processes in the petrochemical production chain.
Energy Storage and Grid Integration
- Energy Storage Systems (ESS): Integrating energy storage systems with a steam turbine power plant can provide additional reliability and cost savings, especially in facilities that integrate renewable energy sources like solar and wind. Energy storage helps balance fluctuating power generation with consistent demand.
- Battery Storage: Battery energy storage can store excess electricity generated during off-peak hours or during periods of high renewable energy output. This stored energy can then be used when renewable generation is low or during peak demand hours, helping stabilize energy supply and reduce reliance on grid power or backup generators.
- Thermal Storage: In some cases, thermal energy storage systems, such as molten salt storage, can be used to store excess heat produced by the steam turbine. This stored thermal energy can later be converted back into electricity or used directly in industrial processes, further enhancing efficiency.
- Grid Integration and Flexibility: While small-scale steam turbine power plants can operate off-grid, some petrochemical plants may still need to be integrated with the local or national power grid. In such cases, it is essential to have an energy management system (EMS) that can optimize the interaction between the power plant and the grid.
- Grid Support and Stability: Small-scale steam turbine plants can provide grid stabilization services by adjusting their output based on demand or by feeding excess power into the grid during periods of high generation. This adds value to the local grid by helping balance supply and demand.
- Demand Response: In regions with dynamic pricing or grid stability concerns, steam turbine plants can be equipped with demand response systems that automatically adjust power production or consumption to align with grid needs, reducing costs and contributing to grid stability.
Regulatory Compliance and Environmental Impact
- Environmental Regulations: Petrochemical plants are subject to stringent environmental regulations regarding emissions, waste disposal, and resource utilization. A small-scale steam turbine power plant can help the facility comply with these regulations while minimizing its environmental footprint.
- Emission Controls: Installing emission control technologies such as scrubbers, selective catalytic reduction (SCR) systems, and particulate filters on steam turbines can significantly reduce harmful emissions like sulfur oxides (SOx), nitrogen oxides (NOx), and particulate matter (PM). This helps ensure the plant adheres to local, regional, and international environmental standards.
- Monitoring and Reporting: The integration of real-time emissions monitoring systems ensures that the steam turbine’s output complies with regulatory standards. Automated data logging and reporting enable the petrochemical plant to meet regulatory requirements and avoid penalties.
- Carbon Pricing and Sustainability: With the increasing adoption of carbon pricing mechanisms, such as carbon taxes or cap-and-trade systems, it is crucial for petrochemical plants to reduce their carbon footprint. Steam turbines powered by low-carbon fuels or waste heat can help the plant minimize its exposure to carbon-related costs and potentially even generate carbon credits.
- Carbon Capture and Utilization (CCU): Incorporating carbon capture technologies into the steam turbine setup can enable the petrochemical plant to capture CO2 emissions and either store them underground or repurpose them for use in other industrial processes (e.g., enhanced oil recovery, synthetic fuels). This not only reduces emissions but may also create additional revenue opportunities from carbon credits or the sale of captured CO2.
- Waste Heat Recovery: A significant advantage of using steam turbines in petrochemical plants is their ability to capture and utilize waste heat produced during various chemical processes. By integrating waste heat recovery systems, the plant can significantly reduce its energy demand, lower fuel consumption, and improve overall efficiency.
- Heat Exchangers and CHP: Heat exchangers can capture waste heat from the steam turbine’s exhaust or from industrial processes and transfer it to other systems, such as heating water or powering other equipment. By using the same energy for multiple purposes (electricity generation, heating, or cooling), the plant maximizes the use of available resources and reduces energy costs.
Long-Term Strategic Considerations
- Operational Excellence and Efficiency: To ensure the steam turbine power plant operates at peak performance, regular maintenance and optimization are essential. An effective maintenance strategy involves predictive maintenance, real-time monitoring of key parameters, and regular system audits.
- Predictive Maintenance: Using advanced condition monitoring systems, plant operators can detect signs of wear or malfunction before a critical failure occurs. This allows for preemptive repairs and reduces the risk of unplanned downtime.
- Continuous Improvement: Applying lean principles and continuous improvement methodologies can help streamline operations, reduce waste, and improve efficiency. Implementing data-driven strategies such as Six Sigma or Total Productive Maintenance (TPM) can further enhance operational performance.
- Future-Proofing with Technology Integration: The petrochemical industry is undergoing a transformation, with a growing focus on digitalization and automation. Integrating IoT, big data analytics, and AI-driven decision-making can help optimize the operation of steam turbines and improve the overall efficiency of the power plant.
- Artificial Intelligence (AI): AI and machine learning can optimize power generation by predicting energy demand patterns and adjusting turbine operations accordingly. AI can also help analyze large datasets from the turbine and plant systems to detect inefficiencies or potential issues.
- Digital Twin Technology: Digital twin technology can be used to create a virtual replica of the steam turbine power plant, enabling operators to simulate different scenarios, optimize performance, and test potential upgrades without interrupting actual operations.
Conclusion
Small-scale steam turbine power plants offer a versatile and sustainable solution to meet the high energy demands of petrochemical plants. By integrating renewable energy sources, waste heat recovery, and fuel flexibility, these power systems provide efficiency, reduce operational costs, and enhance environmental sustainability. In a rapidly changing energy landscape, where carbon reduction and technological innovation are key priorities, the ability to incorporate advanced technologies like predictive maintenance, AI, and energy storage ensures that steam turbine plants remain effective, resilient, and future-ready. With careful planning and integration, petrochemical plants can achieve energy independence, comply with environmental regulations, and contribute to long-term sustainability goals, making steam turbine power plants a critical component of the industry’s evolving energy landscape.
Building on the previous section, further insights into the potential for small-scale steam turbine power plants in petrochemical facilities highlight the importance of continual innovation, environmental stewardship, and economic viability. The integration of advanced technologies and strategic energy management practices is vital for maintaining operational efficiency and meeting the evolving regulatory and market demands. Below, we explore additional considerations to maximize the value of steam turbine-based power plants for petrochemical facilities.
Energy Security and Resilience
- Enhanced Grid Resilience: Small-scale steam turbine power plants provide energy security in regions where power supply is unreliable or vulnerable to disruptions. As petrochemical plants often require uninterrupted power for sensitive processes, having a decentralized power generation system can be a critical factor in maintaining operational continuity.
- Microgrid Capabilities: Integrating a steam turbine power plant into a microgrid allows for greater flexibility and resilience. A microgrid can operate autonomously, disconnected from the main grid, during power outages or periods of grid instability. This is particularly valuable for petrochemical plants that need to ensure continuous production without risking downtime.
- Energy Security in Remote Locations: Petrochemical plants located in remote or offshore areas may face challenges in accessing a reliable power grid. In such scenarios, small-scale steam turbine plants can ensure a continuous energy supply without relying on external grid infrastructure, providing energy autonomy and mitigating risks associated with remote energy sourcing.
- Fuel Diversification: Another key advantage of small-scale steam turbine power plants is their ability to use a variety of fuels, offering flexibility and greater energy security. The ability to switch between different fuel sources—such as natural gas, hydrogen, biofuels, and even waste—ensures that the plant can continue to operate even if one fuel source becomes unavailable or expensive.
- Fuel Switching for Reliability: In cases where one fuel source is disrupted or costly, such as in times of geopolitical instability or price volatility, the steam turbine can switch to an alternative fuel to maintain energy supply. This capability enhances the resilience of the petrochemical plant against external market or supply disruptions.
- Fuel Flexibility for Economic Optimization: The flexibility to switch between different fuels enables economic optimization, as the plant can choose the most cost-effective fuel based on market conditions. By utilizing a combination of fossil fuels and renewable sources, such as biomass, the plant can lower operating costs and improve its competitiveness.
Technological Innovations and Future Trends
- Digitalization and Advanced Control Systems: As digitalization continues to impact industries worldwide, petrochemical plants can leverage smart technologies to improve the performance of their steam turbine power plants. Advanced control systems, predictive analytics, and automation can optimize power generation, reduce fuel consumption, and enhance the efficiency of steam turbines.
- Smart Monitoring and Diagnostics: Using advanced sensor technologies and real-time monitoring systems, plant operators can track the performance of each component within the power plant. Sensors can detect temperature, pressure, vibration, and other key metrics to ensure optimal turbine operation and predict potential failures before they occur.
- AI-Driven Optimization: Artificial intelligence (AI) and machine learning can analyze large amounts of data to identify patterns and trends in energy consumption and turbine performance. AI can be used to optimize energy dispatch, anticipate equipment maintenance, and fine-tune the operation of the steam turbine to improve its efficiency and longevity.
- Integration with Green Technologies: With growing global emphasis on green technologies, the ability to integrate steam turbines with carbon-free or low-carbon energy sources will be crucial for meeting sustainability goals and adhering to stricter environmental regulations.
- Hydrogen Power: Hydrogen, as a clean energy carrier, is emerging as a promising fuel for steam turbines. By utilizing green hydrogen produced from renewable energy sources like wind or solar, petrochemical plants can reduce their carbon footprint while ensuring reliable energy production. Hydrogen-fueled steam turbines also align with long-term decarbonization efforts.
- Carbon Capture and Utilization: As discussed earlier, integrating carbon capture systems with steam turbines can significantly reduce CO2 emissions from petrochemical plants. Not only does this help meet regulatory requirements, but it can also position the plant as a leader in carbon management within the industry. Moreover, captured carbon can be utilized in other processes, such as enhanced oil recovery or producing synthetic fuels, creating additional revenue streams for the plant.
- Blockchain and Energy Trading: Blockchain technology is increasingly being used in the energy sector to enable peer-to-peer energy trading and improve the transparency and security of energy transactions. In the context of small-scale steam turbine power plants, blockchain could facilitate energy exchanges between the plant and neighboring facilities or grid operators.
- Decentralized Energy Trading: Petrochemical plants with excess energy from their steam turbine power plants could sell the surplus energy to nearby industries or even the public grid via a blockchain-based platform. This decentralized approach could provide additional revenue streams for the plant and promote more efficient energy distribution within the region.
- Carbon Credit Trading: Blockchain could also streamline the management of carbon credits earned through carbon reduction initiatives like the use of renewable fuels, carbon capture, or waste heat recovery. This provides a transparent and secure way to trade carbon credits, further incentivizing sustainable practices in the petrochemical industry.
Operational Efficiency and Resource Optimization
- Water Conservation and Wastewater Management: In addition to optimizing energy use, petrochemical plants can also focus on efficient water management to further reduce costs and environmental impact. Steam turbines often rely on large amounts of water for cooling, but with advanced cooling technologies, such as air-cooled condensers or dry cooling systems, water consumption can be significantly reduced.
- Water Recycling Systems: By implementing closed-loop water systems and wastewater treatment technologies, petrochemical plants can minimize their reliance on freshwater sources, reduce wastewater discharge, and enhance their sustainability. This is particularly important in regions experiencing water scarcity or where regulations around water use are tightening.
- Zero-Waste Strategies: Integrating steam turbine systems with zero-waste initiatives within the plant, where every by-product or waste stream is either reused or converted into valuable resources, can help achieve higher levels of operational efficiency and sustainability.
- Heat Recovery and Optimization: Waste heat recovery is a key area where operational efficiency can be maximized. As discussed previously, integrating waste heat recovery with steam turbines allows petrochemical plants to recycle heat produced during industrial processes and use it for power generation or heating.
- Heat Integration Networks: By creating an integrated heat recovery network, petrochemical plants can further improve overall energy efficiency. These networks allow the plant to capture and redistribute excess heat to various sections of the plant that require heat for their operations. This reduces the need for additional fuel consumption and lowers operational costs.
- Advanced Heat Exchanger Designs: Improved heat exchanger designs, such as plate heat exchangers or shell-and-tube heat exchangers, can further increase the efficiency of waste heat recovery systems. These systems maximize the amount of heat that can be extracted from the exhaust gases or process streams, reducing energy consumption and improving the plant’s overall performance.
Long-Term Environmental Sustainability
- Circular Economy and Sustainability: A key principle that is gaining traction in the petrochemical industry is the concept of a circular economy—where waste products and by-products from one part of the plant are used as inputs for other processes. Small-scale steam turbine power plants can play a significant role in supporting circular economy practices.
- Utilizing Waste Streams: For example, a petrochemical plant may generate large amounts of waste heat, CO2, or organic by-products during chemical production. By integrating steam turbine power generation with waste heat recovery and carbon capture, the plant can minimize waste and utilize these by-products for energy generation, thereby closing the loop on resource use.
- Sustainable Feedstock: As petrochemical plants move toward more sustainable production processes, small-scale steam turbines can be powered by renewable feedstocks such as biomass, waste oils, or algae. This reduces the carbon footprint of the plant’s operations and supports the transition toward renewable energy sources in the industrial sector.
- Collaborative Industry Standards: Collaborative efforts between governments, regulators, and industry stakeholders will be key to driving the widespread adoption of sustainable energy systems in petrochemical plants. Industry-wide standards for energy efficiency, emissions reduction, and environmental stewardship will ensure that the petrochemical sector collectively works toward achieving its sustainability goals.
- Government Incentives and Policies: Governments around the world are increasingly offering incentives and subsidies to promote sustainable industrial practices. Petrochemical plants that implement energy-efficient steam turbine power systems and renewable energy solutions may benefit from tax credits, grant funding, or carbon credits. These incentives can significantly offset the initial investment in steam turbine systems and renewable technologies.
Conclusion
The integration of small-scale steam turbine power plants in petrochemical plants presents a compelling case for improving energy efficiency, reducing operational costs, and enhancing environmental sustainability. By embracing fuel flexibility, renewable energy integration, energy storage, and advanced digital technologies, petrochemical facilities can ensure a reliable and resilient energy supply while minimizing their environmental impact.
As the industry continues to evolve toward cleaner and more sustainable practices, steam turbines will play a pivotal role in shaping the future of energy production in petrochemical plants. With the potential for fuel diversification, waste heat recovery, and carbon capture technologies, small-scale steam turbine power plants represent a promising solution to meet the energy needs of the petrochemical sector while achieving long-term environmental and economic benefits. By staying ahead of technological advancements and regulatory requirements, petrochemical plants can remain competitive and contribute to a more sustainable and resilient energy landscape.
Continuing from the previous sections, the development and integration of small-scale steam turbine power plants in petrochemical facilities must address not only the immediate energy needs of the plant but also long-term goals surrounding operational excellence, regulatory compliance, technological innovation, and market adaptation. As the energy landscape shifts towards decarbonization and digitalization, petrochemical plants must evolve to meet the challenges of tomorrow.
Financial Considerations and Investment Strategies
- Capital Investment and Payback Period: Investing in small-scale steam turbine power plants requires substantial capital outlay, including costs for purchasing, installing, and integrating the turbine system. The payback period for this investment will depend on several factors, including fuel prices, operational efficiency, and the extent to which the plant can capture cost savings through energy independence and waste heat utilization.
- Long-Term Cost Savings: While the initial investment in a steam turbine power plant might be high, the long-term cost savings can be substantial. The ability to generate power on-site reduces dependence on grid electricity, which can be subject to price fluctuations. Additionally, energy savings derived from improved efficiency and waste heat recovery contribute to cost reductions over time.
- Financial Incentives and Government Programs: Governments may offer financial incentives to support the adoption of energy-efficient technologies. These can include grants, subsidies, tax breaks, or favorable financing options that help offset the capital investment. Petrochemical companies can take advantage of such programs to shorten the payback period and make the investment more financially viable.
- Financing Models: To mitigate the upfront capital burden, financing models such as Power Purchase Agreements (PPAs), Energy-as-a-Service (EaaS), or leasing arrangements can be considered. These models allow for shared risk and may enable the plant to benefit from advanced technologies without bearing the full cost of ownership immediately.
- Operational Efficiency and Productivity Gains: Beyond energy savings, integrating a steam turbine power plant can lead to overall improvements in operational efficiency and productivity. By incorporating advanced monitoring and control systems, petrochemical plants can optimize performance across a range of variables, from turbine load management to the integration of renewable energy sources.
- Data-Driven Insights: With the use of data analytics and real-time monitoring systems, plant operators can gain insights into the operation of the steam turbine, identifying inefficiencies, equipment wear, and potential issues before they result in costly downtime. These systems help improve operational performance, reduce energy wastage, and increase overall productivity.
- Enhanced Maintenance Practices: Integrating predictive maintenance technologies using IoT sensors and AI-driven analytics allows operators to address wear-and-tear issues proactively, reducing unplanned outages and extending the life of the steam turbine. This minimizes downtime and ensures that the plant remains fully operational, contributing to continuous production.
Sustainability and Social Responsibility
- Community Engagement and Environmental Stewardship: With growing attention on corporate social responsibility (CSR) and environmental stewardship, petrochemical plants must be mindful of their broader impact on local communities and ecosystems. Small-scale steam turbine power plants can contribute positively by reducing the carbon footprint of the facility and providing reliable energy with minimal environmental impact.
- Reduction of Greenhouse Gas Emissions: By utilizing cleaner fuels like hydrogen or biofuels, along with the integration of carbon capture technologies, the plant can significantly reduce its greenhouse gas (GHG) emissions. This supports corporate sustainability goals and can enhance the company’s reputation with stakeholders, investors, and the public.
- Energy Access in Remote Communities: In some cases, small-scale steam turbine power plants can also help extend reliable energy access to surrounding communities or smaller operations. By producing excess energy, these systems could be shared with nearby areas that are otherwise disconnected from the grid, contributing to social good and expanding the plant’s positive local impact.
- Corporate Sustainability Reporting: As the global economy becomes increasingly focused on sustainability and ESG (Environmental, Social, and Governance) criteria, petrochemical plants with small-scale steam turbine power plants must focus on sustainability reporting and transparent environmental practices.
- Sustainability Metrics: The plant can track key sustainability metrics, including energy efficiency, water usage, carbon emissions, and fuel consumption, to demonstrate its commitment to environmental stewardship. These metrics can be shared in sustainability reports, helping to strengthen the company’s public image and attracting investors who prioritize ESG performance.
- Third-Party Certifications: Engaging with third-party certifications, such as LEED, ISO 50001 (energy management), or ISO 14001 (environmental management), helps validate the sustainability efforts of the plant. This independent recognition can further enhance the company’s credibility and foster trust among stakeholders.
Strategic Adaptation to Market Changes
- Adaptation to Changing Energy Markets: The energy market is evolving rapidly, with increasing emphasis on renewable energy, decentralized power generation, and carbon pricing mechanisms. Petrochemical plants must stay ahead of these trends to ensure the long-term viability of their energy systems.
- Transition to Renewable Energy: As part of a broader transition to renewables, petrochemical plants can explore hybrid models where steam turbines are integrated with solar panels or wind turbines. This diversification of energy sources helps reduce reliance on fossil fuels and mitigates exposure to fuel price volatility.
- Flexible Power Generation: Small-scale steam turbines, with their ability to run on a variety of fuels, offer the flexibility needed to adapt to changing energy market conditions. This adaptability allows petrochemical plants to take advantage of lower-cost fuels or to switch to cleaner options when renewable energy is abundant, optimizing both cost and environmental impact.
- Navigating Energy Transition Challenges: The transition to a low-carbon future presents challenges for energy-intensive industries like petrochemicals. Small-scale steam turbines can provide a solution by facilitating the adoption of carbon-neutral fuels, such as biofuels and green hydrogen, and by incorporating carbon capture and storage (CCS) solutions.
- Market Competitiveness: By integrating advanced steam turbine systems, petrochemical plants can lower their carbon intensity, align with evolving carbon reduction targets, and stay competitive in markets where carbon emissions are becoming an important factor in bidding, procurement, and market share.
- Adapting to Future Regulations: Governments worldwide are setting increasingly stringent carbon reduction targets and implementing carbon tax schemes. Small-scale steam turbine systems that incorporate clean energy solutions, such as hydrogen or renewable biomass, will help petrochemical plants comply with these regulations, avoid penalties, and take advantage of potential incentives.
Workforce and Skill Development
- Training and Reskilling the Workforce: Implementing new technologies such as steam turbines powered by alternative fuels or coupled with waste heat recovery systems requires skilled labor. Petrochemical plants must invest in workforce training and reskilling to equip employees with the necessary expertise to manage and operate these advanced systems.
- Skilled Technicians: Technicians and engineers must be trained to operate and maintain high-efficiency steam turbines, which may require expertise in advanced combustion technologies, renewable energy systems, and digital control systems. Regular training and certification programs will help ensure that the workforce remains competent and adaptable to new technological advancements.
- Collaboration with Educational Institutions: Petrochemical plants can collaborate with local universities, vocational schools, and training centers to develop tailored programs that equip students with the skills needed in the energy sector. By fostering partnerships with educational institutions, the plant can contribute to the growth of a skilled workforce while addressing the industry’s future talent gap.
- Safety Protocols and System Integration: The integration of small-scale steam turbines into petrochemical facilities requires stringent safety protocols and proper system integration to mitigate risks associated with high-pressure systems, hazardous materials, and fuel handling. Workers need to be trained in advanced safety measures, emergency response plans, and troubleshooting techniques to ensure the safe operation of the plant.
- Advanced Safety Systems: The implementation of smart safety technologies that monitor pressure, temperature, and system conditions in real-time can provide an additional layer of protection for the workforce. Additionally, digital twins and AI-driven predictive analytics can identify potential safety hazards before they escalate, ensuring a proactive approach to hazard prevention.
Conclusion: A Future-Oriented Approach
Small-scale steam turbine power plants are becoming an essential part of the energy mix for petrochemical plants, particularly as the industry moves toward cleaner, more sustainable energy sources. By investing in flexible, efficient, and resilient steam turbine systems, petrochemical plants can improve their energy security, reduce costs, comply with evolving regulatory requirements, and enhance their overall environmental sustainability.
As technology advances and market dynamics shift, the integration of digital technologies, renewable energy sources, and carbon reduction solutions will be crucial in optimizing the performance of steam turbine systems. By aligning with these trends and leveraging innovative technologies, petrochemical plants can remain competitive, economically viable, and environmentally responsible, ensuring they are well-positioned for future growth and success in a rapidly changing global energy landscape.
Small Scale Power Plant for Forestry Operations
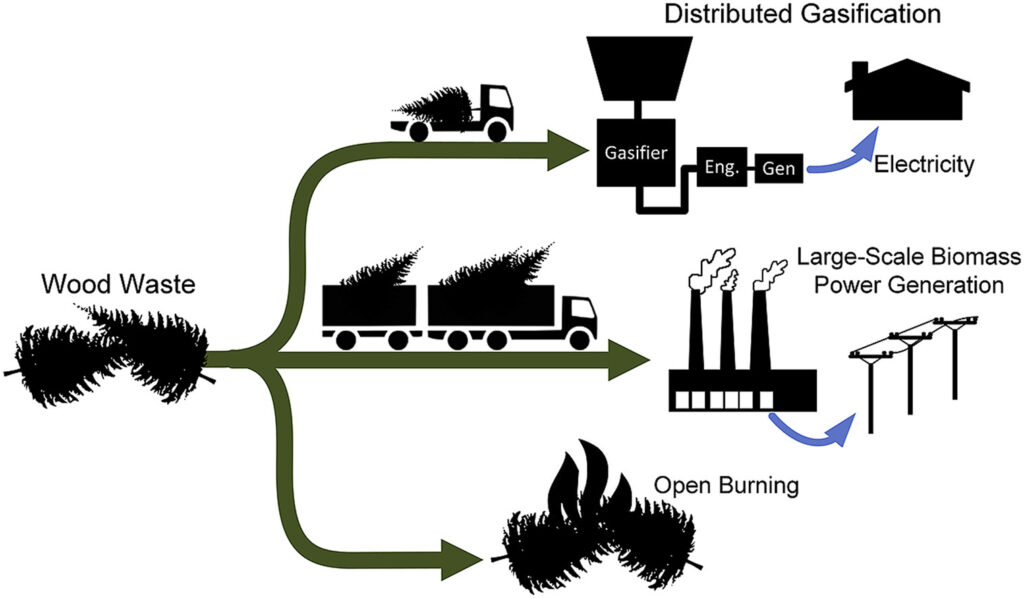
Small-scale power plants for forestry operations present a unique opportunity to create self-sustaining energy systems in remote areas while contributing to environmental sustainability. These systems, typically utilizing steam turbines, can harness biomass and waste products from forestry activities to generate power. By doing so, they can reduce dependence on external power sources, lower operational costs, and minimize the carbon footprint of forestry operations.
Key Features of Small-Scale Power Plants for Forestry Operations
- Biomass Fuel Sources: The primary fuel source for small-scale power plants in forestry operations is often biomass. Forestry by-products, such as wood chips, sawdust, bark, and even forestry residues, provide a renewable source of energy. Utilizing these materials to generate electricity reduces waste and supports a more sustainable operation by closing the loop on the use of natural resources.
- Wood Waste Utilization: Wood chips and sawdust generated during logging, milling, and other forestry activities can be converted into fuel for steam turbines. This reduces the need to transport waste materials off-site, cutting down on transportation costs and the associated carbon emissions.
- Carbon-Neutral Energy Production: Biomass is considered a carbon-neutral energy source because the CO2 emitted during combustion is offset by the carbon absorbed by the trees during their growth. This makes biomass an attractive option for forestry operations seeking to minimize their environmental impact.
- Power Generation Efficiency: Steam turbine-based power plants in forestry operations are typically designed for high thermal efficiency. The heat generated by burning biomass fuels is used to produce steam, which drives a turbine to generate electricity. This power can then be used to run the plant’s operations, including sawmills, drying kilns, and other machinery.
- Combined Heat and Power (CHP) Systems: Many small-scale steam turbine power plants are designed to operate as CHP systems, where both electricity and useful heat are generated. The heat produced in the process can be used for drying wood, heating buildings, or other applications within the forestry operation, further improving the system’s overall efficiency.
- Off-Grid Energy Solutions: Forestry operations are often located in remote areas, where access to the central power grid may be limited or non-existent. In these cases, small-scale power plants provide a reliable and cost-effective off-grid energy solution.
- Energy Independence: A dedicated power plant allows forestry operations to reduce reliance on external energy suppliers, which can be particularly valuable when grid electricity is unreliable, costly, or unavailable.
- Microgrid Capabilities: Integrating the power plant with a microgrid enables the forestry operation to maintain stable electricity supply in isolated areas. A microgrid can also improve operational resilience by providing backup power in case of grid failures or extreme weather conditions.
- Local Job Creation and Economic Development: The establishment of small-scale power plants within forestry operations can create new local jobs, contributing to regional economic development. These jobs may include roles in plant operation, maintenance, and fuel procurement, as well as ancillary services related to the power generation process.
- Skilled Labor: As with any industrial energy system, the operation of a small-scale steam turbine power plant requires skilled technicians and operators. Training local workers to manage these plants can enhance the sustainability and self-sufficiency of forestry operations, while also boosting the local economy.
- Local Supply Chain Benefits: By utilizing local biomass materials, forestry operations can create a circular economy that benefits local suppliers, including logging companies and wood product manufacturers. This fosters economic growth and strengthens the regional economy.
- Environmental Benefits: Small-scale power plants for forestry operations can contribute significantly to environmental sustainability by promoting the use of renewable resources and reducing waste. Key environmental benefits include:
- Reduction in Landfill Waste: Many forestry operations produce waste materials such as sawdust and bark that would otherwise be discarded. By converting these materials into energy, forestry operations can minimize waste sent to landfills and reduce the environmental impact associated with waste disposal.
- Ecosystem Preservation: By utilizing local biomass and reducing dependence on fossil fuels, small-scale power plants can help preserve ecosystems. The use of biomass reduces the environmental degradation associated with extracting and burning fossil fuels and helps promote a cleaner, greener energy future.
- Sustainable Resource Management: Forestry operations that integrate small-scale power plants can promote sustainable resource management practices by ensuring that biomass is harvested and used efficiently. This reduces the environmental impact of the entire operation and helps the company meet sustainability goals.
Technological and Operational Considerations
- Fuel Supply and Logistics: Efficient biomass procurement and transportation are essential for the smooth operation of small-scale power plants in forestry operations. Forestry companies must establish reliable supply chains for biomass fuels to ensure a consistent and cost-effective fuel supply.
- Local Biomass Sourcing: Forestry operations often have ready access to biomass materials, such as wood chips and sawdust, making them ideal candidates for utilizing local resources. To maintain a sustainable fuel supply, companies may need to develop long-term contracts with nearby suppliers or create a process for collecting and processing waste materials into fuel.
- Storage and Handling: Efficient biomass storage systems are necessary to ensure that fuel remains dry and easily accessible for combustion. Technologies like bunker storage or silage bags can be employed to manage biomass inventory, minimizing fuel waste and maximizing plant performance.
- Steam Turbine Design and Optimization: The design of the steam turbine system is crucial to maximizing the efficiency and reliability of the power plant. Key considerations include turbine size, fuel input, steam pressure, and heat recovery systems.
- Customizable Turbine Solutions: Small-scale steam turbines for forestry operations should be tailored to meet the specific energy needs and available fuel sources of the operation. The right turbine capacity and configuration ensure that the plant can generate sufficient power while maintaining high efficiency.
- Heat Recovery Systems: Incorporating waste heat recovery systems into the design allows the plant to use excess heat for other processes, such as drying wood, providing hot water, or heating buildings. This can improve the overall efficiency of the system and reduce operational costs.
- Environmental Compliance and Emissions Control: Small-scale power plants in forestry operations must comply with local and national environmental regulations, including those related to air quality and emissions.
- Emissions Control Technologies: Biomass combustion releases certain emissions, such as particulate matter, NOx, and CO2. To minimize the impact of these emissions, forestry operations can incorporate advanced flue gas cleaning systems such as electrostatic precipitators, scrubbers, or baghouse filters. Additionally, biomass gasification or pyrolysis technologies can be explored to lower emissions and improve energy efficiency.
- Grid Integration and Energy Export: In some cases, small-scale power plants for forestry operations can be integrated with the local electricity grid, enabling them to export excess energy.
- Power Purchase Agreements (PPAs): Forestry operations can negotiate PPAs with local utilities or government agencies to sell excess power generated by their steam turbine plants. This creates additional revenue streams and further reduces the costs of operating the power plant.
- Exporting Clean Energy: The use of renewable biomass fuels makes small-scale power plants attractive for utilities looking to meet renewable energy targets. Forestry operations that produce excess clean energy could play a role in the region’s renewable energy landscape, helping to reduce the carbon intensity of the grid.
Challenges and Mitigation Strategies
- Fuel Availability and Cost Variability: One of the main challenges of using biomass as a fuel source is the variability in fuel availability and cost, depending on seasonal factors and market fluctuations.
- Seasonal Supply Management: Forestry operations can mitigate these challenges by developing efficient biomass procurement strategies that ensure consistent supply throughout the year. This could involve storing excess fuel during peak harvesting periods and using it during leaner months.
- Diversified Fuel Options: While wood residues and forestry waste are ideal, small-scale power plants can also use other biomass sources, such as agricultural waste or even dedicated energy crops, to ensure a stable fuel supply.
- Initial Capital Investment: The cost of setting up a small-scale steam turbine power plant, including the cost of equipment, installation, and infrastructure, can be significant.
- Financing Options: Forestry operations can explore various financing models, including green financing and carbon credit markets, to offset initial costs. Government incentives for renewable energy projects can also help alleviate the financial burden of setting up a small-scale power plant.
- Partnerships and Collaboration: Collaborating with technology providers, engineering firms, and financial institutions can also help mitigate upfront costs and ensure the project’s success.
- Maintenance and Operational Costs: Like any energy system, a small-scale power plant requires regular maintenance to ensure optimal performance and longevity.
- Preventive Maintenance: Implementing predictive maintenance practices using sensors and AI can help identify potential issues before they cause downtime, minimizing operational disruptions and costs.
- Training and Skill Development: The operation and maintenance of steam turbines require specialized knowledge. Training local workers and establishing a skilled workforce are essential for ensuring long-term plant reliability and efficiency.
Conclusion
Small-scale steam turbine power plants for forestry operations offer a promising solution for energy independence, environmental sustainability, and economic growth. By harnessing locally available biomass resources, these plants can generate renewable power, reduce waste, and provide reliable energy for remote operations. With the right technologies and strategic planning, forestry operations can create a sustainable energy model that supports both operational needs and environmental goals.
The integration of small-scale power plants in forestry can also contribute to local job creation, the economic development of rural communities, and the advancement of clean energy initiatives. Despite challenges related to fuel supply, capital investment, and operational maintenance, the long-term benefits of using biomass for power generation in the forestry sector outweigh the potential downsides. By carefully managing these factors, forestry operations can thrive in a future where sustainable energy practices are increasingly prioritized.
Continuing the discussion on small-scale power plants for forestry operations, there are several aspects to consider when optimizing the integration of steam turbines within forestry energy systems, particularly in terms of technological advancements, operational excellence, and long-term sustainability goals.
Advanced Technological Developments
- Hybrid Power Generation Systems: As the energy landscape evolves, forestry operations may benefit from hybrid power generation systems that combine traditional biomass steam turbines with renewable energy sources such as solar power or wind energy. This integration offers several advantages, such as increased energy reliability and stability, especially in regions where biomass supply can fluctuate due to seasonal variations.
- Renewables Integration: Hybrid systems allow forestry operations to complement their biomass power generation with clean, renewable sources. For example, during periods when biomass availability is lower (like after the primary harvesting season), solar or wind power can ensure the continuity of operations. Additionally, when biomass is abundant, excess energy produced by solar or wind can be used to charge energy storage systems or be fed into the local grid.
- Energy Storage Solutions: Coupled with renewable sources, energy storage systems, such as batteries or thermal storage units, can store excess energy generated during off-peak times. This stored energy can be used during peak demand periods or when renewable generation drops, enhancing the overall resilience of the power system.
- Decentralized Energy Networks: Small-scale power plants, especially when deployed in isolated or off-grid areas, are often integrated into decentralized energy networks. These networks use advanced digital grid technologies to efficiently distribute and balance energy across multiple sources within the operation.
- Smart Grids and IoT Integration: Using Internet of Things (IoT) technologies, sensors, and smart meters, forestry operations can better monitor and control energy consumption and generation in real-time. This data-driven approach allows operators to optimize the operation of the steam turbine power plant, adjust for energy demand fluctuations, and reduce inefficiencies. A smart grid can also provide better integration with renewable energy sources, facilitating smooth power sharing between turbines and solar panels or wind farms.
- Microgrids: In remote areas where connection to the main electricity grid is challenging or impractical, forestry operations may consider microgrids. These are localized energy systems that can operate independently or in conjunction with the grid. Microgrids allow for better energy management and ensure that critical operations, such as sawmills or processing equipment, continue to function even if there are grid disruptions.
Operational Efficiency and Optimization
- Advanced Combustion Technology: Innovations in combustion technology can enhance the performance of small-scale steam turbines used in forestry operations. For example, fluidized bed combustion or circulating fluidized bed (CFB) boilers can be used to improve the combustion of low-grade biomass fuels, which are commonly found in forestry waste.
- Higher Combustion Efficiency: These advanced combustion systems are designed to burn a variety of biomass fuels at higher efficiency rates, producing more energy with less fuel. This reduces operational costs by optimizing fuel use, increasing the overall energy output, and extending the lifespan of the steam turbine.
- Reduced Emissions: Newer combustion technologies often come with lower emissions than traditional methods, improving the environmental footprint of the forestry operation. Lower emissions of particulate matter and NOx contribute to cleaner air quality around the plant, helping companies meet regulatory compliance and sustainability goals.
- Automation and Control Systems: The integration of advanced automation and control systems in steam turbine-based power plants is essential for optimizing performance. Using real-time data analysis and AI-powered decision-making, operators can monitor various system parameters such as fuel quality, steam pressure, turbine speed, and exhaust temperature.
- Predictive Maintenance: With the help of AI and machine learning, the power plant can implement predictive maintenance systems. These systems use data gathered from sensors installed in critical components like turbines and boilers to predict potential failures before they occur. Predictive analytics help schedule maintenance only when needed, reducing unnecessary downtime and ensuring that the plant operates at peak performance.
- Performance Monitoring: Automated systems allow for continuous performance monitoring and optimization. For example, a software-based energy management system can adjust turbine operations based on demand and fuel availability, ensuring efficient energy generation without overloading the system. This can significantly extend the operational life of the plant and improve the overall return on investment.
- Waste Heat Recovery: Incorporating waste heat recovery systems into small-scale power plants can significantly boost overall energy efficiency. The heat generated by the steam turbine can be captured and repurposed for other energy needs within the forestry operation, such as drying timber, heating facilities, or generating hot water.
- Cogeneration and Trigeneration: Many small-scale steam turbine plants in forestry operations are designed as cogeneration systems, where electricity and useful heat are produced simultaneously. This maximizes the utilization of the energy generated and further reduces operational costs. In some cases, trigeneration systems may also be employed to produce cooling in addition to heat and electricity, further expanding the range of applications for the recovered energy.
- Sustainable Resource Use: Heat recovery systems can also aid in minimizing environmental impact by reducing the need for additional fossil fuel-based heating sources, such as oil or gas. By utilizing waste heat, forestry operations reduce their carbon footprint, contributing to broader sustainability initiatives.
Environmental Impact and Regulatory Compliance
- Sustainable Biomass Sourcing: Sustainable sourcing of biomass is crucial for minimizing the environmental impact of a small-scale power plant in forestry operations. Ensuring that the biomass used in power generation comes from sustainably managed forests or waste materials is essential for meeting environmental standards and supporting broader conservation efforts.
- Certification Programs: Forestry companies can seek certification under programs like Forest Stewardship Council (FSC) or Programme for the Endorsement of Forest Certification (PEFC) to demonstrate their commitment to sustainable practices. These certifications ensure that biomass is harvested responsibly, protecting biodiversity, soil health, and the integrity of ecosystems.
- Minimizing Land Use Impact: Ensuring that biomass production does not compete with food production or contribute to deforestation is critical. Small-scale power plants should prioritize the use of residues and waste biomass over direct harvesting of live trees, thus reducing pressure on natural forests.
- Emission Reduction Strategies: Small-scale steam turbine plants for forestry operations must adhere to stringent environmental regulations regarding emissions. Implementing state-of-the-art emissions control technologies, such as scrubbers or electrostatic precipitators, can help minimize the release of harmful pollutants into the atmosphere.
- Carbon Emissions Offsets: In some regions, forestry operations may be eligible for carbon credits by reducing their carbon footprint through the use of biomass for power generation. Carbon credits can be sold in carbon markets, providing an additional revenue stream and promoting sustainability.
- Monitoring and Reporting: Forestry operations must maintain transparent emissions monitoring systems to ensure compliance with regulatory standards. Data from emission sensors can be transmitted to regulatory agencies, ensuring that the plant operates within the required limits. This can also help prevent fines or operational shutdowns due to non-compliance.
- Water Conservation: Water usage in power plants, particularly in steam turbines, is a significant environmental concern. Small-scale power plants for forestry operations can reduce water consumption by employing closed-loop water systems, where water used for cooling is recirculated rather than discharged after one use.
- Water-Efficient Technologies: Integrating technologies such as dry cooling systems or air-cooled condensers can help minimize water consumption, especially in regions where water resources are scarce. These systems use ambient air to cool the steam, eliminating the need for extensive water use in cooling towers.
Economic Considerations and Future Potential
- Cost Savings and ROI: The use of small-scale power plants in forestry operations offers the potential for significant cost savings over time. By generating their own electricity from biomass, forestry operations can lower their energy bills and avoid the volatility of external electricity prices. Additionally, using waste materials for power generation reduces the need for waste disposal or the purchase of external fuels, further contributing to savings.
- Reduced Energy Costs: One of the most immediate financial benefits of operating a small-scale steam turbine power plant is the ability to generate electricity on-site. The savings from reduced grid electricity purchases can be used to offset the initial capital costs of the power plant and contribute to a faster payback period.
- Revenue from By-Products: Depending on local market conditions, forestry operations may also be able to generate revenue by selling excess energy to the grid through Power Purchase Agreements (PPAs). These agreements ensure a stable source of income, contributing to the long-term economic viability of the plant.
- Potential for Expansion and Replication: Small-scale steam turbine power plants can be replicated and scaled up within the forestry sector or adapted for other rural and remote industries. The model could be extended to agricultural operations, mining activities, or small communities in need of reliable, sustainable energy. By demonstrating the feasibility of such systems in forestry, the model could be adapted to other industries, offering further economic opportunities.
- Supporting Policy and Incentives: Governments and international organizations increasingly recognize the value of small-scale renewable energy projects, including those in forestry. Financial incentives, grants, and carbon trading schemes can provide critical support for these initiatives, helping to make them financially viable. By leveraging these incentives, forestry operations can accelerate the adoption of small-scale power plants and maximize their impact on both the environment and the economy.
Conclusion
Small-scale steam turbine power plants are a compelling option for forestry operations, offering multiple benefits ranging from cost savings and energy independence to environmental sustainability and local job creation. By leveraging locally sourced biomass and incorporating advanced technologies such as hybrid power systems, waste heat recovery, and predictive maintenance, forestry operations can optimize energy production while minimizing their environmental impact.
As the world moves toward a more sustainable energy future, small-scale power plants offer a versatile solution that can be adapted to meet the unique challenges faced by industries in remote or off-grid areas. For the forestry sector, investing in these energy systems not only provides reliable power but also supports broader sustainability goals, contributing to both economic resilience and environmental stewardship.
Continuing with small-scale power plants for forestry operations, there are additional facets of this system that contribute to its optimization and long-term success, focusing on efficiency improvements, community engagement, and market opportunities.
Efficiency Improvements and Technological Advancements
- Biomass Preprocessing Technologies: The quality of the biomass used in steam turbine power plants plays a significant role in determining the efficiency of power generation. To optimize fuel utilization, forestry operations can invest in biomass preprocessing technologies, such as pelletization and torrefaction. These processes enhance the fuel properties by making the biomass more uniform in size, energy-dense, and easier to handle.
- Pelletization: Biomass pellets are made by compacting wood chips, sawdust, or other organic materials into small, uniform cylinders. This process improves the energy content per unit of fuel and reduces moisture levels, leading to more efficient combustion. Pelletized biomass can be burned more effectively in steam turbines, leading to higher power generation with less fuel.
- Torrefaction: This is a process where biomass is heated to high temperatures in the absence of oxygen, producing a fuel that has similar characteristics to coal. Torrefied biomass has higher energy density, lower moisture content, and is easier to store and transport, making it an ideal fuel for small-scale power plants. The process also reduces the volume of waste produced, making it a more efficient energy resource for forestry operations.
- Advanced Turbine Technologies: As technology evolves, so do the steam turbines used in small-scale power plants. Innovations in turbine design, materials, and efficiency mechanisms continue to improve the overall performance of these systems.
- High-Efficiency Steam Turbines: Newer steam turbines offer improved performance with higher thermal efficiency, allowing forestry operations to extract more power from the same amount of fuel. Advanced materials like superalloys and ceramic composites are being used to increase turbine performance by allowing them to operate at higher temperatures and pressures. This results in more efficient power generation and reduced fuel consumption.
- Flexible Operations: Modern steam turbines are increasingly designed to be flexible, capable of adjusting to fluctuations in fuel supply and varying power demands. This is particularly beneficial for forestry operations that may experience seasonal variations in biomass availability or changes in energy needs. The ability to ramp turbine operations up or down efficiently allows for better integration of renewable energy sources and improved energy security.
- Optimization of System Integration: The integration of small-scale steam turbines with other components of the forestry operation, such as heating systems, can further improve energy efficiency. By designing the system as a closed-loop energy network, energy losses can be minimized, and waste heat can be used productively.
- Heat Recovery for Drying Processes: In forestry operations, wood drying is one of the most energy-intensive processes. By integrating waste heat recovery systems with drying kilns, the excess heat from the steam turbine can be used to dry wood, thereby reducing the need for additional fuel sources and improving energy efficiency across the operation.
- Integrated Energy Systems: In addition to electricity and heating, small-scale steam turbine systems can be integrated with other energy systems, such as biogas or waste-to-energy technologies, to create a hybrid power plant that efficiently uses available resources. By designing an integrated system that can adapt to the dynamic needs of forestry operations, companies can maximize both energy production and cost savings.
Community Engagement and Socioeconomic Benefits
- Job Creation and Skill Development: The implementation of a small-scale power plant in forestry operations not only creates jobs within the plant itself but can also stimulate the local economy by creating new opportunities in support industries.
- Local Employment: Power plant operations, maintenance, and fuel procurement provide significant employment opportunities, particularly in rural or remote areas. Skilled labor is required for plant operation, turbine maintenance, and fuel processing, which can directly benefit local communities.
- Training and Skill Development: Investing in workforce training programs ensures that local workers are equipped with the technical skills needed to operate and maintain advanced power generation systems. These skills can also be transferred to other sectors, further contributing to the region’s overall economic growth. By developing a highly trained workforce, forestry operations can ensure that their power plants operate efficiently and remain competitive in the energy market.
- Strengthening Local Economies: By generating power locally, forestry operations can create a more resilient and self-sufficient local economy. In addition to reducing energy costs, the economic benefits extend to the wider community, as the power plant can support local businesses by providing affordable, stable energy.
- Economic Diversification: Small-scale power plants can encourage economic diversification by fostering the development of ancillary businesses that support plant operations. These businesses can include logistics providers for biomass fuel, maintenance service providers for turbine equipment, and local construction companies that assist with infrastructure development.
- Support for Rural Communities: In remote rural areas where energy access may be limited, the development of small-scale power plants provides an opportunity to improve the living standards of local residents. Reliable electricity can power homes, schools, and medical facilities, enhancing the overall quality of life and supporting community development.
- Enhancing Sustainability through Local Resources: By using locally sourced biomass, forestry operations support a circular economy model that ensures that waste materials from the forestry process are repurposed into valuable energy. This reduces the need for external energy imports and strengthens the connection between the forestry operation and the local ecosystem.
- Local Biomass Sourcing: Forestry operations can source biomass locally from nearby forests, waste materials, or agricultural by-products. This not only supports the local economy but also reduces transportation costs and the environmental impact of fuel sourcing. By relying on locally sourced biomass, the carbon footprint associated with fuel transport is minimized.
- Waste Minimization: In addition to biomass, other waste products from forestry activities—such as tree bark, leaves, and other organic materials—can be utilized in the power generation process, minimizing the amount of waste sent to landfills. The integration of waste-to-energy systems can make the entire operation more efficient and environmentally responsible.
Market Opportunities and Financial Considerations
- Revenue Generation through Power Sales: Forestry operations that generate excess power from their small-scale steam turbines can take advantage of Power Purchase Agreements (PPAs) with local utilities or private companies. These agreements allow the forestry operation to sell excess energy to the grid, creating an additional revenue stream and improving the overall financial feasibility of the project.
- Renewable Energy Certificates (RECs): In many regions, renewable energy generation is incentivized through programs that allow operators to earn Renewable Energy Certificates (RECs) for producing clean, renewable energy. These certificates can be sold to other companies or entities that need to meet renewable energy mandates, further enhancing the financial viability of the plant.
- Government Incentives: Governments around the world offer tax credits, subsidies, and grants to support the development of small-scale renewable energy projects. Forestry operations can leverage these incentives to reduce the upfront capital costs of power plant installation and improve the overall financial return on investment.
- Carbon Credit Markets: As part of global efforts to mitigate climate change, carbon credit markets have grown, offering companies the opportunity to generate revenue by reducing their carbon footprint. Small-scale power plants that use biomass as fuel can participate in carbon trading programs and sell carbon credits to businesses looking to offset their emissions.
- Carbon Offsets: The biomass combustion process in steam turbines can qualify as a carbon-neutral energy source, where the carbon released during combustion is offset by the carbon absorbed by the plants during their growth. By selling carbon credits, forestry operations can generate additional income, while also contributing to global climate goals.
- Green Certification: Gaining certification under sustainable energy programs can improve the marketability of the forestry operation’s products and services. Customers increasingly prefer businesses with green certifications, and this can provide a competitive edge in the marketplace.
- Energy Independence and Risk Mitigation: By investing in small-scale steam turbine power plants, forestry operations can improve their energy security and reduce the risks associated with rising energy costs or supply disruptions.
- Mitigating Energy Price Fluctuations: Fossil fuel-based energy prices are often volatile, which can impact the profitability of operations. By producing their own energy, forestry operations can protect themselves from these fluctuations and gain more control over their energy expenses.
- Operational Resilience: Having a reliable, locally generated power source can improve the operational resilience of forestry operations. This is especially important in remote areas where power outages from the grid could disrupt operations, leading to costly downtime. Small-scale power plants offer a reliable backup solution to ensure continuity.
Future Trends and Opportunities
- Energy Innovation: As renewable energy technologies evolve, small-scale power plants will continue to benefit from new innovations that improve efficiency, reduce costs, and lower environmental impact. Advances in turbine design, fuel processing, and energy storage will open new opportunities for forestry operations to adopt the latest technologies and remain competitive.
- Increasing Market Demand for Renewable Energy: The global shift toward renewable energy is driving increased demand for clean power sources. As policies and regulations become more stringent regarding carbon emissions, forestry operations that invest in renewable energy technologies will be well-positioned to meet regulatory requirements and attract environmentally conscious investors and customers.
- Circular Economy and Sustainability: The growing emphasis on sustainability and circular economy principles will continue to drive the demand for integrated renewable energy systems in sectors like forestry. Small-scale power plants that utilize waste materials and provide energy to support the entire operation will align with these trends and demonstrate long-term viability.
Conclusion
Small-scale power plants utilizing steam turbines present a compelling solution for forestry operations, offering numerous environmental, economic, and operational benefits. By embracing innovations in biomass processing, turbine design
, energy integration, and sustainability practices, forestry operations can significantly enhance their energy independence, reduce operational costs, and contribute to local economic growth. The combination of advanced technologies, community engagement, and market opportunities positions small-scale power plants as a sustainable and viable energy solution for the forestry sector.
Key Takeaways for Forestry Operations
- Energy Security and Self-Sufficiency: One of the most notable advantages of implementing a small-scale steam turbine power plant is the increased energy security it provides. By generating electricity on-site, forestry operations become less reliant on external power sources, which can be especially beneficial in remote or off-grid locations where energy access is unreliable. This self-sufficiency allows for better control over operational costs, mitigating the risk of price volatility and supply disruptions from the national or regional grid.
- Economic Viability: Beyond reducing energy costs, small-scale power plants can open up new revenue streams through the sale of excess electricity, renewable energy certificates (RECs), and carbon credits. By participating in carbon credit markets or entering into power purchase agreements (PPAs), forestry operations can generate additional income, which can help offset the capital investment in the power plant. Furthermore, efficient biomass processing and advanced combustion technologies reduce operational costs, ensuring a high return on investment over time.
- Sustainability and Environmental Responsibility: By harnessing locally sourced biomass and implementing energy-efficient technologies, small-scale steam turbine plants help forestry operations meet sustainability goals while contributing to broader environmental efforts. Reducing greenhouse gas emissions through the use of renewable biomass fuels, minimizing waste through energy recovery, and incorporating low-emission technologies all contribute to a cleaner, more sustainable energy solution. These initiatives support the circular economy by repurposing waste products into valuable energy, promoting responsible resource management.
- Community Development and Local Benefits: Small-scale power plants create a variety of social and economic benefits for local communities, particularly in rural or underserved areas. These plants provide job opportunities in energy production, maintenance, and biomass processing, which can help alleviate unemployment in remote areas. Moreover, the provision of reliable electricity can improve living standards by powering homes, schools, and medical facilities, supporting broader community development efforts.
- Technological Advancements and Future Trends: As technology continues to evolve, the efficiency and capabilities of small-scale steam turbine plants will only improve. Innovations in turbine design, biomass fuel processing, energy storage systems, and emissions control will allow forestry operations to optimize their energy production and reduce costs further. Additionally, the growing global shift toward renewable energy and sustainability will create further incentives for forestry operations to invest in clean, renewable energy sources.
- Resilience to External Factors: In addition to energy security, small-scale steam turbine plants offer resilience against unpredictable factors such as natural disasters, climate change, and supply chain disruptions. The ability to generate electricity locally ensures that forestry operations can maintain continuity even in the face of external challenges. This is especially important in the context of a changing climate, where the availability of external resources like fossil fuels may become more uncertain.
The Road Ahead: Strategic Considerations for Forestry Operations
As the demand for renewable energy grows and sustainability becomes a more significant factor in business operations, the strategic advantages of small-scale power plants in the forestry industry are clear. For successful implementation, forestry operations should consider the following factors:
- Feasibility Studies and Site Assessment: Before moving forward with a small-scale steam turbine power plant, forestry operations should conduct thorough feasibility studies and site assessments. These studies will evaluate the biomass availability, energy needs, environmental impacts, and local market conditions, ensuring that the project aligns with both technical and financial goals.
- Investment in Research and Development: Continuous investment in research and development (R&D) can help forestry operations stay ahead of technological advancements and refine their energy systems over time. Collaboration with technology providers, universities, and research institutions can lead to the adoption of cutting-edge solutions that improve efficiency and lower costs.
- Partnerships with Local Stakeholders: Collaborating with local communities, governments, and energy providers is crucial to the success of small-scale power plant projects. Establishing strong partnerships can help secure funding, gain regulatory approvals, and ensure that the project aligns with regional development goals. Additionally, these partnerships can help foster community buy-in and support for renewable energy initiatives.
- Long-Term Sustainability Planning: Forestry operations should view small-scale steam turbine power plants not as short-term investments but as long-term sustainability assets. A well-designed and properly maintained power plant can provide reliable, cost-effective energy for decades, contributing to the operation’s overall profitability and resilience. By incorporating ongoing maintenance, technological upgrades, and monitoring systems, forestry operations can ensure that their energy systems remain efficient and effective for the long term.
- Expanding to Other Markets and Applications: Once the model has been successfully implemented in forestry, there is potential for expanding small-scale power plants to other industries and sectors. The lessons learned from forestry operations can be applied to other rural or remote areas, such as agricultural operations, mining projects, or isolated communities, further enhancing the potential for clean, locally generated energy.
Conclusion
Small-scale steam turbine power plants represent a powerful tool for forestry operations looking to increase energy independence, reduce costs, and contribute to environmental sustainability. By leveraging locally sourced biomass, advanced technology, and innovative energy integration strategies, these power plants provide a reliable and efficient source of energy for forestry operations and their surrounding communities. Moreover, as the global push for cleaner, renewable energy intensifies, the adoption of such systems will play a crucial role in achieving broader sustainability goals and securing a cleaner, more resilient energy future for the forestry industry.
As technological advancements continue to improve the efficiency, affordability, and scalability of small-scale power plants, their role in transforming the energy landscape for forestry operations and other industries will become even more pivotal. With the right planning, investment, and community engagement, small-scale steam turbine power plants can provide a sustainable energy solution that meets the operational needs of the forestry sector while driving positive economic, social, and environmental change.
Small Scale Power Plant for Recycling Centers
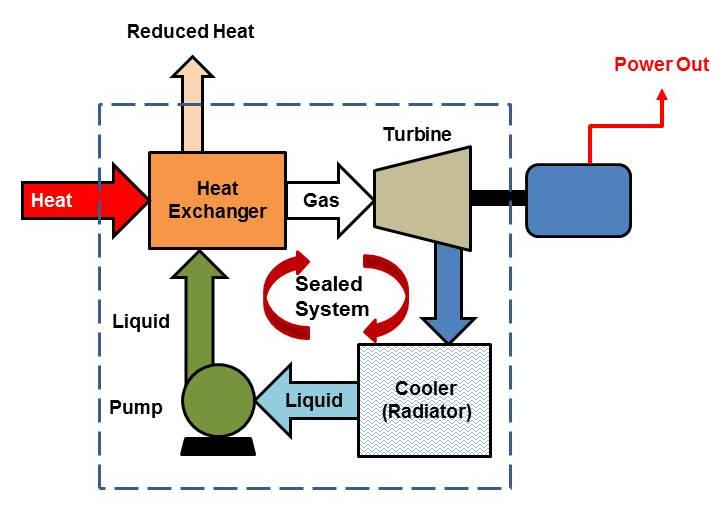
A small-scale power plant for recycling centers offers a unique and sustainable solution to energy generation while supporting the recycling industry. Recycling centers often deal with significant amounts of waste materials, much of which can be repurposed for energy production. The integration of small-scale power plants, especially those powered by steam turbines, into recycling operations can help to create an efficient and environmentally friendly energy ecosystem.
Recycling centers face several challenges, such as managing large quantities of waste, maintaining operational efficiency, and minimizing their environmental footprint. Small-scale steam turbine power plants can provide a significant advantage by turning waste materials into a valuable energy source, reducing operational costs, and making the recycling process more energy-efficient.
Key Considerations for Implementing Small Scale Power Plants in Recycling Centers
- Energy Generation from Waste Materials: One of the most significant benefits of using small-scale steam turbine power plants in recycling centers is the ability to convert waste into energy. Various types of waste materials—such as paper, plastics, and organic matter—can be processed into biomass fuel or directly used to generate heat and electricity through combustion.
- Biomass Fuel Production: Recycling centers can collect organic waste like wood, food scraps, and agricultural by-products, process them into biomass pellets or briquettes, and use these as fuel in the steam turbine power plant. By doing so, recycling centers can reduce their reliance on external power sources while providing a local, renewable energy supply.
- Energy from Non-Recyclable Materials: Non-recyclable materials, such as certain plastics or contaminated paper products, can be used as fuel in waste-to-energy processes. These materials can undergo thermal treatment processes like pyrolysis or gasification to generate energy, reducing the overall waste sent to landfills and converting it into usable electricity.
- Operational Efficiency and Cost Savings: By generating energy onsite, recycling centers can reduce their energy costs, ensuring more efficient operations. The energy savings from a small-scale power plant can offset the initial investment and ongoing maintenance costs of the plant, providing long-term financial benefits.
- Reduced Energy Expenses: Recycling centers often operate 24/7, consuming large amounts of electricity for machinery, lighting, and sorting processes. By generating their own power, these facilities can lower their dependence on external energy suppliers, stabilizing their energy expenses.
- Operational Flexibility: Small-scale power plants allow recycling centers to adjust energy production according to their needs. For instance, when there is an increase in the volume of recyclable materials, the plant can ramp up power generation to meet the demand. This flexibility ensures the center can continue its operations smoothly without disruptions due to power shortages or rising energy prices.
- Waste Management and Environmental Impact: A primary concern for recycling centers is the environmental impact of the waste they process. Small-scale power plants powered by steam turbines can help recycling centers improve their waste management strategies and lower their environmental footprint.
- Reduction of Landfill Waste: Many materials processed at recycling centers, especially plastics and other non-biodegradable items, can be challenging to dispose of sustainably. Converting waste to energy through combustion or gasification allows these materials to be used productively instead of contributing to landfill buildup.
- Lower Carbon Emissions: Recycling centers can choose biomass fuels that have a lower carbon footprint compared to fossil fuels. The steam turbine systems can run on organic matter that absorbs carbon dioxide during growth, ensuring that the energy generation process is carbon-neutral or low in emissions. This reduces the overall greenhouse gas emissions associated with the recycling operation and contributes to a more sustainable energy model.
- Energy Integration and Efficiency: Small-scale power plants can be integrated with various systems within a recycling center, including heating, lighting, and drying equipment. This system integration allows for better energy optimization, which can improve overall energy efficiency.
- Combined Heat and Power (CHP) Systems: Many small-scale steam turbine power plants are designed as combined heat and power (CHP) systems. CHP systems generate both electricity and useful heat in a single process, which can be used for purposes like drying materials, heating the facility, or powering machinery. This integrated approach maximizes the use of available energy, increasing the overall efficiency of the recycling center.
- Waste Heat Recovery: In a recycling center, certain processes like shredding or material sorting can generate excess heat. This heat can be captured and used in the power plant’s steam turbine system, reducing the need for additional fuel and further enhancing the facility’s energy efficiency.
- Community and Economic Benefits: The implementation of a small-scale power plant for recycling centers can provide substantial benefits to local communities and the economy at large. These plants help stimulate local economies by providing reliable energy, creating jobs, and supporting regional sustainability initiatives.
- Job Creation: The development, operation, and maintenance of small-scale power plants create job opportunities in areas like energy management, machinery maintenance, and biomass fuel production. Additionally, local businesses that supply fuel or services to the plant can benefit from the increased demand.
- Economic Resilience: By producing energy locally, recycling centers become less reliant on external power grids and suppliers, insulating them from price fluctuations and potential disruptions. This energy resilience strengthens the overall economic stability of the area.
- Supporting Local Sustainability: Using waste as an energy source supports a circular economy model, where the materials are continuously reused, reducing reliance on new resources and encouraging sustainable practices. This aligns with the broader environmental goals of reducing waste and promoting green energy solutions.
- Technology and Innovation: Technological advancements in small-scale power generation, waste-to-energy systems, and steam turbines will continue to evolve, offering further improvements in efficiency and environmental performance. By adopting cutting-edge technology, recycling centers can future-proof their energy systems and ensure optimal operation for years to come.
- Improved Biomass Conversion: Innovations in biomass conversion technologies, such as advanced gasification or fast pyrolysis, allow recycling centers to process a wider variety of materials more efficiently. These processes convert waste into gas or oil that can be used in turbines or engines for power generation, offering greater flexibility in fuel options.
- Smart Grid Integration: As the energy landscape becomes more digitized, small-scale power plants can be integrated into smart grids that allow for better management of energy production, distribution, and consumption. This integration improves the reliability and stability of the energy supply, enabling recycling centers to track and optimize their energy usage in real time.
Challenges and Solutions
While the benefits of small-scale power plants for recycling centers are considerable, there are also challenges that need to be addressed for successful implementation:
- Initial Capital Investment: The upfront cost of building a small-scale power plant can be significant, especially for recycling centers that are already operating under tight budgets. However, through careful financial planning and by seeking incentives like government subsidies, grants, or loans for renewable energy projects, the capital burden can be eased.
- Fuel Availability and Supply Chain: Ensuring a consistent and reliable supply of biomass or other waste materials for fuel can be a logistical challenge, particularly in urban areas where space for fuel storage is limited. Partnering with local waste collection services or exploring waste-to-energy options like industrial by-products can help ensure a stable supply of fuel.
- Regulatory Compliance and Environmental Standards: Waste-to-energy systems and small-scale power plants must adhere to stringent environmental regulations. Recycling centers must comply with local emissions standards, waste management policies, and health and safety guidelines. Working with environmental experts and regulatory bodies can help ensure the plant operates within legal requirements and minimizes environmental impact.
- Technological Integration: Integrating a small-scale steam turbine plant with existing recycling center operations may require significant upfront planning to ensure compatibility and optimal performance. Recycling centers may need to invest in upgrading infrastructure, training staff, or incorporating additional systems to manage power generation and energy consumption effectively.
Conclusion
Small-scale power plants powered by steam turbines offer recycling centers an effective, sustainable, and economically viable solution for energy generation. These systems not only provide a renewable source of power but also contribute to waste management by converting waste materials into valuable energy. By improving energy efficiency, reducing environmental impact, and supporting local economies, these power plants align with the growing demand for greener, more resilient energy solutions.
As the recycling industry continues to grow and evolve, integrating small-scale power plants can help create a more sustainable and energy-efficient ecosystem, supporting both the recycling centers’ operational needs and broader environmental goals. With continued innovation and a focus on sustainable practices, small-scale steam turbine power plants have the potential to revolutionize the way recycling centers operate, creating a cleaner, more sustainable future for the industry and the communities they serve.
Small Scale Power Plant for Recycling Centers
The integration of small-scale power plants into recycling centers is an exciting and innovative way to enhance both operational efficiency and environmental responsibility. Moving forward, the potential for growth in this area is immense, with advancements in technology and increased demand for sustainable solutions driving the transition to more energy-independent and eco-friendly recycling operations.
Advancements in Technology for Enhanced Efficiency
As technology continues to evolve, recycling centers can benefit from improvements in energy generation and waste processing technologies. Some of these advancements include:
- Advanced Combustion Technologies: New combustion technologies can help optimize the use of biomass waste, allowing recycling centers to burn a wider variety of materials more efficiently. Innovations such as fluidized bed combustion or circulating fluidized bed (CFB) systems allow for more consistent energy output while minimizing emissions, offering an even more sustainable approach to waste-to-energy systems.
- Biogas Production and Integration: In addition to steam turbines, small-scale power plants could also integrate biogas production systems. By processing organic waste through anaerobic digestion, recycling centers can generate biogas, which can then be used to fuel gas turbines or combined with other forms of energy generation, such as CHP systems. This technology would add another layer of sustainability, offering flexibility in fuel use and enhancing overall plant efficiency.
- Energy Storage Systems: To mitigate fluctuations in energy demand and ensure consistent power generation, energy storage systems (ESS), such as batteries or thermal energy storage, can be integrated into small-scale power plants. This would allow recycling centers to store excess energy during periods of low demand and release it when needed, reducing the reliance on external energy sources and ensuring a continuous power supply.
- Smart Sensors and Automation: Automation and Internet of Things (IoT) technologies can further improve the efficiency of small-scale power plants by enabling real-time monitoring of energy production, consumption, and equipment performance. Smart sensors can provide data on key metrics like temperature, pressure, and emissions, allowing operators to fine-tune processes and identify inefficiencies or potential issues before they lead to system failures.
Impact of Small Scale Power Plants on Circular Economy and Sustainability
The transition to renewable energy through small-scale power plants in recycling centers directly contributes to the circular economy by promoting the reuse and repurposing of materials. This aligns perfectly with the sustainability goals of recycling centers, as they focus on reducing waste, reprocessing materials, and minimizing environmental impact. Small-scale power plants powered by waste not only help reduce landfill use but also:
- Conserve Natural Resources: By using waste materials as energy sources, recycling centers avoid extracting and using raw materials that are required for conventional power generation, such as coal or natural gas.
- Reduce Transportation Emissions: Recycling centers that process local waste into energy reduce the need for transportation of raw fuels or external energy sources, which results in a smaller carbon footprint.
- Enhance Waste Diversion: Through waste-to-energy processes, recycling centers can divert materials from landfills or incinerators and convert them into valuable resources. This increases the overall recycling rate and decreases environmental pollution.
Scalability and Replicability of Small Scale Power Plants
One of the major advantages of small-scale power plants for recycling centers is their scalability. These systems can be tailored to suit facilities of various sizes and capacities, making them ideal for implementation in both large-scale recycling centers and smaller, local operations. In some cases, as the recycling center grows, the power plant can be expanded or upgraded to increase its energy production without a complete overhaul of the system.
- Modular Systems: Many modern steam turbine systems are modular, which means they can be customized and scaled according to the specific needs of the recycling center. As a result, smaller centers with limited energy requirements can start with a smaller plant and expand as they scale their operations.
- Replicability in Other Locations: The successful implementation of a small-scale power plant at one recycling facility can serve as a model for other recycling centers, both within the same region and across different locations. This makes the technology highly replicable, allowing for widespread adoption and contributing to the development of a more sustainable waste-to-energy infrastructure.
Conclusion
Small-scale power plants powered by steam turbines offer a dynamic and highly sustainable solution for recycling centers looking to reduce energy costs, improve waste management practices, and contribute to a cleaner environment. The ability to convert waste materials into energy aligns perfectly with the principles of the circular economy, making recycling centers more energy-independent and environmentally responsible.
By leveraging advancements in combustion technologies, biogas production, and energy storage systems, recycling centers can further enhance the efficiency of their operations while reducing their carbon footprint. The scalability and flexibility of small-scale power plants make them a viable solution for both large and small recycling facilities, ensuring that energy needs can be met effectively across a range of operations.
Moreover, as the global demand for sustainable waste management solutions continues to grow, the integration of renewable energy generation in recycling centers will play an increasingly important role in driving the transition to a low-carbon economy. Through strategic investments, technological innovation, and a focus on community and environmental benefits, recycling centers can position themselves at the forefront of the green energy revolution while ensuring long-term operational success and contributing to a more sustainable world.
Future Outlook and Strategic Considerations for Small Scale Power Plants in Recycling Centers
The integration of small-scale power plants in recycling centers offers significant opportunities for growth and sustainability, but as with any technological investment, careful consideration is needed to ensure their success. Below are several future outlook points and strategic considerations for recycling centers looking to incorporate these systems:
1. Financial Incentives and Policy Support
The financial viability of small-scale power plants can be bolstered by governmental incentives, grants, and favorable policies aimed at promoting renewable energy adoption and waste management improvements. Recycling centers can explore funding opportunities provided by both local and national governments to offset the initial capital investment for these projects.
- Tax Incentives and Subsidies: Governments may offer tax credits or subsidies for renewable energy initiatives, including waste-to-energy systems. These incentives can significantly reduce the upfront costs associated with installing a small-scale power plant and improve the financial return on investment over time.
- Renewable Energy Certificates (RECs): As part of green energy regulations, recycling centers can earn RECs for generating renewable energy from waste. These certificates can be traded or sold in renewable energy markets, providing an additional revenue stream for the recycling center and offsetting operational costs.
- Public-Private Partnerships (PPPs): Forming strategic alliances with private investors or utilities may provide an additional layer of financial security for the development of small-scale power plants. Such partnerships can enable recycling centers to access both financial and technical expertise while reducing their financial burden.
2. Integration with Smart Waste Management Systems
The future of waste management is likely to be heavily influenced by digital technologies. Recycling centers that integrate smart waste management systems with their small-scale power plants can optimize energy generation, reduce waste, and streamline operations.
- Smart Sensors and Data Analytics: The use of IoT sensors and data analytics will allow recycling centers to monitor waste streams in real-time, optimizing energy production. These systems can track the types of materials being processed, adjust combustion settings in the power plant, and even identify opportunities for further waste reduction or recycling.
- Automated Waste Sorting: As part of a broader waste management strategy, automation technologies, such as robotic sorting systems, can be incorporated to improve waste separation. By using automated systems, recycling centers can ensure that only suitable materials are sent to the power plant for energy generation, improving fuel efficiency and reducing operational waste.
3. Expansion into Circular Economy Initiatives
Small-scale power plants that utilize waste materials for energy generation also position recycling centers as key players in the circular economy. As demand for circular economy solutions grows, these plants can provide a more efficient way to recycle and repurpose resources.
- Collaborative Waste-to-Energy Projects: Recycling centers can collaborate with other industries, such as agriculture, food processing, or manufacturing, to collect waste materials that would otherwise go to landfills. By integrating these materials into the small-scale power plant’s energy production, recycling centers can further contribute to waste diversion efforts and become integral to regional circular economy initiatives.
- Energy Recovery from E-Waste: One particularly growing area within recycling is the processing of electronic waste (e-waste), which contains valuable metals and materials. A small-scale power plant could potentially use non-recyclable e-waste to generate energy, provided the proper technologies are in place to safely process these materials.
4. Scalability to Meet Future Demand
As recycling centers scale their operations to accommodate growing volumes of recyclable materials, small-scale power plants can grow alongside them. Scalability is a crucial factor in ensuring that power generation capacity aligns with the future needs of the facility.
- Modular Expansion: Small-scale steam turbine systems are typically modular, meaning that as the recycling center processes more materials and generates more waste, the power plant’s capacity can be expanded without disrupting operations. This modularity ensures that the plant remains adaptable to future growth.
- Geographic Expansion: Larger recycling facilities with established waste-to-energy systems can consider branching out and setting up satellite plants or expanding services into nearby regions. This can help create a network of small-scale power plants that service different geographic areas, helping other local facilities reduce energy costs and support broader sustainability efforts.
5. Technological Innovation in Waste Conversion
Innovations in waste conversion technologies will continue to drive improvements in the efficiency and environmental performance of small-scale power plants. Recycling centers should keep an eye on emerging technologies that could enhance their operations.
- Thermochemical Conversion: New methods for converting waste to energy—such as pyrolysis, gasification, and plasma arc technology—can provide higher efficiency in generating energy from mixed waste streams. These methods can convert a wider variety of waste into syngas, which can be used in gas turbines or combined heat and power systems.
- Advanced Biomass Processing: Improvements in biomass pre-treatment methods, such as torrefaction (a process that makes biomass more energy-dense), will make it easier for recycling centers to use lower-grade waste materials, like agricultural by-products, and convert them into high-efficiency fuel sources.
6. Consumer and Public Awareness
As more consumers and businesses become aware of the environmental impacts of waste and energy consumption, recycling centers that implement small-scale power plants can leverage this trend to enhance their reputation and attract more customers. Public awareness campaigns that highlight the center’s renewable energy initiatives and waste-to-energy practices can build trust and loyalty with the community and promote sustainable behaviors.
- Corporate Social Responsibility (CSR): By implementing green energy solutions like waste-to-energy power plants, recycling centers can highlight their commitment to sustainability and CSR initiatives. This can improve their public image and attract environmentally conscious customers and investors.
- Engagement with Stakeholders: Recycling centers can work closely with local governments, educational institutions, and NGOs to raise awareness about the environmental benefits of small-scale power plants. By engaging with local communities, they can foster a broader culture of sustainability and environmental stewardship.
Conclusion: The Future of Small-Scale Power Plants in Recycling Centers
The future of small-scale power plants in recycling centers is promising, with numerous opportunities for growth, innovation, and environmental benefits. These systems offer a way to reduce energy costs, improve waste management, and contribute to the circular economy. With ongoing advancements in technology, financial incentives, and increased public awareness, recycling centers have the opportunity to become leaders in sustainable energy generation while also supporting broader environmental goals.
As the demand for renewable energy, waste reduction, and sustainable waste management practices grows, small-scale steam turbine power plants will play an increasingly pivotal role in transforming recycling operations. By adopting these systems, recycling centers can not only improve their bottom line but also create a positive impact on the environment and local communities.
The key to success lies in continued investment in technology, collaboration with stakeholders, and a commitment to building more efficient and sustainable operations. With these principles in mind, recycling centers can become vital contributors to the green economy, pushing forward solutions that balance the needs of the environment, industry, and society as a whole.
The Role of Policy and Industry Collaboration in Advancing Small-Scale Power Plants in Recycling Centers
For small-scale power plants in recycling centers to thrive, it is essential that both governmental policies and industry collaborations provide the necessary framework to support their development and operation. Below are a few critical aspects that will shape the future success of these systems:
1. Governmental Regulations and Incentives
Government policies will be instrumental in shaping the adoption and growth of small-scale power plants in recycling centers. Regulatory frameworks can provide the necessary push for recycling centers to invest in renewable energy solutions by creating incentives and regulations that make such investments financially viable and operationally sustainable.
- Waste Diversion Mandates: Many countries and municipalities have set ambitious targets for waste diversion, such as aiming to divert a certain percentage of waste away from landfills. Small-scale power plants powered by waste materials are a direct way for recycling centers to meet these mandates while generating renewable energy. Governments could further incentivize this by offering financial support or streamlined permitting processes for centers investing in waste-to-energy technologies.
- Renewable Energy Standards and Goals: Many governments have implemented renewable energy standards (RES), which require utilities and businesses to meet specific renewable energy production quotas. Recycling centers with small-scale power plants can qualify as renewable energy generators, allowing them to fulfill part of these quotas and potentially benefit from government-backed renewable energy credits or tax incentives.
- Emissions Reduction Programs: Small-scale power plants contribute to emissions reduction by utilizing waste and biomass, which would otherwise decay and release greenhouse gases. Governments can support these systems by providing incentives tied to carbon credits and offering financial compensation for reducing greenhouse gas emissions. Such incentives can offset the costs of adopting renewable energy systems in recycling centers.
- Research Grants and Technical Assistance: Governments can further promote the adoption of renewable energy in recycling centers by offering research grants, funding for pilot projects, and providing technical assistance. This support can help mitigate the risks and challenges associated with the adoption of new technologies, such as small-scale steam turbines and advanced waste-to-energy systems.
2. Collaboration with the Private Sector and Technology Providers
Public-private partnerships (PPPs) will play a crucial role in advancing the integration of small-scale power plants in recycling centers. These collaborations can provide recycling facilities with access to the latest technologies, financial resources, and expertise needed to design and implement energy solutions.
- Technology Providers: Collaborating with technology providers specializing in steam turbines, waste-to-energy solutions, and energy storage systems will ensure that recycling centers have access to the latest, most efficient technologies. Working directly with manufacturers and energy consultants can help tailor solutions to the specific needs of the facility, optimizing both energy production and waste processing.
- Energy Suppliers and Utilities: Recycling centers can form partnerships with local energy suppliers and utilities to sell excess energy generated from their power plants. By entering into power purchase agreements (PPAs), recycling centers can secure long-term contracts for the sale of renewable energy, further improving the financial viability of their investments. In turn, energy suppliers gain access to clean, locally produced energy that contributes to their renewable energy portfolios.
- Waste Management Companies: Collaborating with waste management companies can improve the efficiency of waste-to-energy systems. These partnerships can ensure a steady supply of waste materials for the power plants and allow for better sorting and preparation of waste to optimize its energy generation potential.
3. Educating Stakeholders and Public Engagement
As small-scale power plants in recycling centers are an innovative approach to waste management, it will be important to educate stakeholders, including employees, community members, investors, and policymakers, on the benefits of these systems.
- Employee Training: Training employees on the operation and maintenance of small-scale power plants will ensure that systems are run efficiently and safely. Recycling centers can offer continuous professional development programs for staff to ensure they are knowledgeable about emerging technologies, regulatory changes, and the integration of renewable energy into daily operations.
- Community Outreach: Public engagement campaigns can raise awareness about the environmental benefits of small-scale power plants. By showcasing the positive impact of waste-to-energy initiatives, recycling centers can gain the support of local communities and stakeholders, reinforcing the importance of sustainability and renewable energy in everyday practices.
- Industry Knowledge Sharing: Industry associations and networks can facilitate knowledge sharing between recycling centers, technology providers, government bodies, and other stakeholders. Conferences, workshops, and online platforms can provide a space for exchanging best practices, new research, and lessons learned from implementing small-scale power plants, ensuring that all parties are up to date with the latest developments in the field.
4. Addressing Public Concerns and Perceptions
One challenge in implementing small-scale power plants in recycling centers is addressing public concerns about potential environmental impacts. These concerns may include emissions from combustion, the potential for hazardous waste, and other operational risks. Transparent communication and careful planning will be key to overcoming these challenges.
- Transparency and Monitoring: Recycling centers should commit to environmental transparency by publishing regular reports on emissions, waste processing, and energy production. These reports can provide data on air quality, emissions levels, and other critical metrics that demonstrate the environmental benefits of the facility.
- Clean Technology Solutions: As combustion technologies evolve, new solutions that minimize emissions and optimize fuel use will help mitigate public concerns. Technologies such as carbon capture and storage (CCS), advanced filtration systems, and cleaner combustion methods can be integrated into small-scale power plants to reduce air pollution and make them more environmentally friendly.
5. Long-Term Sustainability and Impact
The success of small-scale power plants in recycling centers will ultimately depend on their long-term sustainability and ability to deliver continuous positive impacts. These impacts extend beyond operational efficiency and cost savings to include broader social and environmental benefits.
- Job Creation and Local Economic Development: The implementation and operation of small-scale power plants in recycling centers can create new jobs, particularly in areas such as plant operation, maintenance, waste processing, and energy management. This can contribute to local economic growth while helping to build a green economy in the community.
- Long-Term Environmental Impact: Over time, the cumulative effects of waste diversion, reduced carbon emissions, and renewable energy generation can contribute to significant environmental improvements. Recycling centers that invest in small-scale power plants are not only helping to reduce waste but are also playing an active role in mitigating climate change and promoting the transition to a sustainable energy future.
Conclusion: Unlocking the Potential of Small-Scale Power Plants in Recycling Centers
The integration of small-scale power plants in recycling centers offers a wealth of opportunities to advance sustainability in waste management. From the technological innovations that improve energy generation to the financial incentives that make such projects more feasible, small-scale power plants are well-positioned to drive significant improvements in both operational efficiency and environmental performance.
To fully realize the potential of these systems, recycling centers must work in partnership with government agencies, private sector technology providers, and local communities. Collaboration across these sectors will provide the support, knowledge, and financial backing necessary to overcome challenges and ensure the long-term success of waste-to-energy initiatives.
As we continue to move toward a more sustainable future, small-scale power plants in recycling centers will be at the forefront of the waste-to-energy revolution, helping to reduce waste, conserve natural resources, and generate clean energy. By investing in these systems today, recycling centers can ensure a greener tomorrow—one where waste is no longer seen as a burden but as a valuable resource that contributes to the sustainable energy landscape.
Small Scale Power Plant for Sawmills
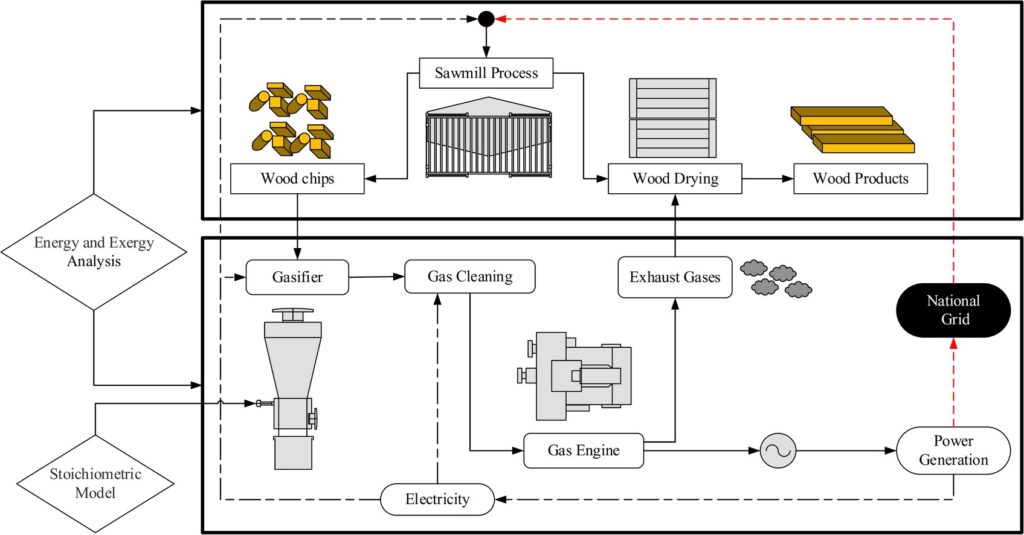
Sawmills are a critical part of the forestry industry, providing essential services in the processing of wood into lumber and other products. However, sawmills also produce significant amounts of waste, including wood chips, sawdust, bark, and other by-products. These materials often present disposal challenges and can contribute to environmental pollution if not handled properly. One solution to both reduce waste and generate renewable energy is the integration of small-scale power plants that use steam turbines.
The integration of a small-scale power plant powered by sawmill by-products, such as biomass, offers a sustainable way to generate energy while addressing waste management issues. This system not only helps reduce operational costs but also supports the sawmill’s environmental sustainability goals by utilizing the by-products that would otherwise be discarded.
1. Energy Production from Sawmill By-Products
Sawmills generate large amounts of waste material, including wood chips, bark, and sawdust. Rather than sending these by-products to landfills or incinerators, small-scale power plants can convert them into valuable energy. These biomass materials can be burned in a combustion system or processed through gasification or pyrolysis technologies to produce heat and electricity.
- Biomass Combustion: Biomass is the most straightforward and widely used method for small-scale power generation in sawmills. In a steam turbine setup, the biomass is combusted in a boiler to generate heat. The heat turns water into steam, which drives a steam turbine to generate electricity. The use of sawmill waste in this process significantly reduces the need for external fuel sources, allowing the sawmill to become more energy self-sufficient.
- Gasification: Instead of direct combustion, gasification involves heating biomass in a controlled environment to produce a flammable gas (syngas). This syngas can then be used to generate electricity through a gas turbine or an internal combustion engine. Gasification has the advantage of generating more energy from lower-grade biomass materials and producing fewer emissions compared to traditional combustion methods.
- Pyrolysis: Pyrolysis is a thermal process that breaks down biomass at high temperatures without the presence of oxygen. The resulting bio-oil can be used as a fuel for steam turbines or other energy generation technologies. Pyrolysis can also create biochar, a carbon-rich by-product that can be used in soil enhancement, further promoting sustainability.
2. Benefits of Small Scale Power Plants for Sawmills
Integrating a small-scale power plant into sawmill operations offers a range of benefits, from cost savings to environmental advantages:
- Reduced Energy Costs: A significant portion of a sawmill’s operating expenses goes toward purchasing electricity or fuel to run machinery, lighting, and other facilities. By using waste materials as fuel, a small-scale power plant can reduce or eliminate the need to purchase energy from external sources, leading to substantial cost savings.
- Waste Reduction: Rather than transporting wood chips, sawdust, and bark to landfills or incinerators, the power plant can use these by-products to generate electricity and heat. This approach not only reduces waste disposal costs but also helps reduce the environmental footprint of the sawmill.
- Carbon Neutrality: Biomass is considered a renewable energy source because the carbon dioxide released during combustion is offset by the carbon absorbed by the plants during their growth. Using sawmill waste as fuel for energy production can help the mill move toward carbon neutrality by reducing the overall emissions from the facility.
- Sustainable Energy Supply: By generating its own energy, a sawmill can become more energy independent. This reduces reliance on grid electricity, which may be subject to price fluctuations or interruptions. The ability to produce energy locally can also help sawmills maintain stable operations during power outages or energy crises.
- Job Creation and Local Economic Development: The establishment of a small-scale power plant creates jobs, including positions for plant operators, maintenance workers, and engineers. Additionally, the use of local wood waste as fuel supports regional economies and promotes the development of green jobs.
3. Environmental and Operational Considerations
While small-scale power plants powered by sawmill by-products offer clear environmental benefits, there are operational considerations that must be addressed to ensure the system functions efficiently and sustainably.
- Emissions Control: The combustion of biomass can produce particulate matter, nitrogen oxides (NOx), sulfur oxides (SOx), and carbon monoxide (CO). Therefore, proper emissions control systems, such as baghouse filters, scrubbers, and selective catalytic reduction (SCR) systems, must be installed to ensure compliance with environmental regulations and minimize air pollution.
- Fuel Preparation and Quality: Biomass fuels such as sawdust and wood chips vary in moisture content, size, and density. These variations can impact the efficiency of the power plant. Therefore, proper fuel handling and preparation processes, such as drying and sorting, should be implemented to ensure consistent and efficient combustion. The quality of the biomass fuel will directly affect the energy output and operational costs of the plant.
- Thermal Efficiency: The efficiency of energy conversion in small-scale power plants is crucial. Improvements in boiler efficiency, steam turbine performance, and heat recovery systems can all contribute to more effective power generation. Additionally, combining the power plant with a combined heat and power (CHP) system can enhance overall efficiency by capturing and using waste heat for other processes in the sawmill.
- Regulatory Compliance: The sawmill must ensure that the small-scale power plant complies with all local environmental and safety regulations. This includes obtaining necessary permits, adhering to air quality standards, and monitoring emissions. Regulatory compliance ensures that the plant operates legally and minimizes any potential negative impact on the surrounding community.
4. Financial Considerations and Incentives
The financial viability of integrating a small-scale power plant into sawmill operations will depend on several factors, including the upfront capital investment, operational costs, and potential for energy savings. The capital investment typically covers the cost of the biomass combustion system, steam turbine, boilers, and emissions control equipment. However, this investment can be offset by several financial incentives:
- Government Incentives: Many governments offer financial incentives to encourage the adoption of renewable energy systems, including tax credits, grants, or low-interest loans. Sawmills can take advantage of these incentives to reduce the initial cost of implementing a small-scale power plant.
- Renewable Energy Credits (RECs): Sawmills can potentially generate Renewable Energy Credits (RECs) for producing renewable energy from biomass. These credits can be sold or traded, generating additional revenue to offset operational costs and improve the financial viability of the project.
- Cost Savings from Waste Utilization: By utilizing sawmill waste to generate power, sawmills can reduce or eliminate the costs of waste disposal and fuel purchases. This provides a significant return on investment, especially when the energy generated is used on-site to power sawmill operations.
- Energy Sales: In some cases, sawmills with small-scale power plants may generate more energy than needed for their operations. In such cases, they can sell excess electricity to the local grid or to nearby industries, further increasing the financial benefits of the system.
5. Scalability and Future Growth
One of the main advantages of small-scale power plants is their scalability. Sawmills can begin with a modest system and expand it as their energy needs grow or as waste production increases. This scalability allows sawmills to manage initial costs and grow their energy generation capacity gradually.
- Modular Power Systems: Many small-scale power plant systems are modular, which means they can be expanded or upgraded over time. If the sawmill’s waste generation or energy demand increases, additional modules or units can be added to the existing system without requiring a complete overhaul of the infrastructure.
- Adapting to New Waste Streams: Sawmills may also diversify their waste streams over time as new types of wood by-products or alternative biomass sources become available. A small-scale power plant can be adapted to process different forms of biomass, allowing the sawmill to continue generating energy from waste regardless of changes in the type of material available.
6. Conclusion
Small-scale power plants powered by biomass from sawmill waste provide an effective and sustainable solution for energy generation while addressing waste disposal challenges. These systems offer sawmills the opportunity to reduce energy costs, improve waste management practices, and contribute to environmental sustainability by utilizing renewable energy sources.
With the ability to generate energy locally, sawmills can reduce their dependence on external power sources, stabilize energy costs, and potentially sell excess electricity to the grid. Furthermore, by converting sawmill waste into energy, these systems help reduce the environmental footprint of sawmills and contribute to the broader goal of reducing carbon emissions and fostering a circular economy.
As technology advances, small-scale power plants will continue to become more efficient and cost-effective, making them an increasingly attractive option for sawmills looking to improve their sustainability practices and operational efficiency. By integrating small-scale power plants, sawmills can not only reduce their environmental impact but also build a more resilient and sustainable business model for the future.
Future Prospects and Technological Innovations for Small-Scale Power Plants in Sawmills
The future of small-scale power plants in sawmills is promising, as technological innovations continue to improve the efficiency and environmental performance of these systems. The adoption of advanced technologies, along with evolving market dynamics and environmental policies, can further enhance the viability and impact of power plants within the sawmill sector. Below are key areas where technological developments could play a significant role in the future of small-scale power plants for sawmills:
1. Advanced Biomass Conversion Technologies
As the demand for more efficient and environmentally friendly energy production grows, sawmills can benefit from the integration of advanced biomass conversion technologies. These technologies can improve the energy output, reduce emissions, and optimize the use of available biomass resources.
- Improved Combustion Systems: Advances in combustion technology, such as fluidized bed combustion and bubbling bed systems, can help sawmills increase the efficiency of biomass burning. These technologies allow for more consistent and controlled combustion, leading to better heat recovery and reduced emissions. Additionally, low-emission burners are being developed to minimize pollutants like particulate matter and nitrogen oxides (NOx), which can be a concern in biomass combustion.
- Gasification Enhancements: Gasification technology continues to evolve, with new processes emerging that enhance the efficiency of converting biomass into syngas. Innovations in high-temperature gasification and partial oxidation processes can increase the energy density of the produced syngas, allowing sawmills to generate more power from the same amount of biomass. Moreover, the use of catalytic converters can further improve the quality of syngas by removing harmful contaminants before it is used in power generation.
- Pyrolysis Process Innovations: Pyrolysis is gaining attention as a way to convert biomass into biochar, bio-oil, and syngas. With new developments in the pyrolysis process, sawmills may be able to optimize the energy production from their wood waste while also creating valuable by-products, such as bio-oil, which can be used as an alternative fuel for heating or power generation. Furthermore, biochar can be used for soil enrichment or carbon sequestration, adding another layer of sustainability to the process.
2. Integration with Renewable Energy and Storage Systems
Integrating small-scale power plants in sawmills with other renewable energy technologies and energy storage systems can provide a more reliable and efficient energy solution. These systems not only enhance the energy generation capabilities of sawmills but also allow them to operate more flexibly, meeting fluctuating demand and contributing to grid stability.
- Hybrid Renewable Systems: Small-scale biomass power plants can be integrated with other renewable energy sources, such as solar or wind power, to form a hybrid renewable energy system. During times when biomass availability or energy demand is low, solar or wind power can supplement energy production, while biomass can continue to provide power when renewable conditions are not optimal. This hybrid system can improve the overall reliability and efficiency of the energy supply, allowing sawmills to achieve greater energy independence.
- Energy Storage Solutions: The integration of energy storage systems (such as batteries or thermal storage) can help sawmills store excess energy produced during peak generation periods. The stored energy can then be used when power demand is high or when biomass availability is lower. Advanced battery technologies, such as lithium-ion and flow batteries, are becoming more cost-effective and can help sawmills optimize their energy use by storing and discharging electricity as needed.
- Demand Response Systems: Demand response technologies allow sawmills to adjust their energy consumption based on real-time signals from the grid or their own internal energy management systems. By participating in demand response programs, sawmills can reduce energy costs, provide grid services, and help balance supply and demand in the energy market. This technology can be integrated with small-scale power plants to optimize energy use and improve the overall energy efficiency of the sawmill.
3. Smart Grids and Automation
The integration of smart grid technology and automation in sawmills can enhance the performance and flexibility of small-scale power plants. A smart grid allows for better communication and control over energy generation and consumption, enabling the sawmill to optimize its energy use, reduce waste, and increase the overall efficiency of its operations.
- Real-Time Monitoring and Control: Advanced sensor technology and Internet of Things (IoT) devices can enable real-time monitoring of biomass feedstock, combustion efficiency, and emissions. This data can be used to optimize the combustion process, manage fuel supplies, and adjust energy generation based on current needs. By integrating this data into a centralized energy management system, sawmills can gain a better understanding of their energy consumption patterns and make data-driven decisions to improve performance.
- Predictive Maintenance and Optimization: Predictive maintenance, enabled by IoT sensors and data analytics, allows sawmills to identify potential issues with equipment before they lead to failures. For example, sensors can monitor the health of steam turbines, boilers, and other critical components of the small-scale power plant, helping to schedule maintenance only when necessary. This can reduce downtime, extend the lifespan of equipment, and lower operational costs.
- Automated Energy Management: Energy management systems (EMS) powered by artificial intelligence (AI) and machine learning can optimize the operation of small-scale power plants by continuously analyzing data from various sources, including weather forecasts, biomass availability, and energy demand. These systems can automatically adjust operations to ensure that the sawmill’s energy needs are met in the most cost-effective and environmentally friendly manner.
4. Policy and Market Trends Influencing Growth
Policy support and market trends are critical factors that will influence the growth of small-scale power plants in sawmills. As governments worldwide continue to push for decarbonization and energy independence, sawmills are likely to benefit from favorable regulations and incentives.
- Carbon Pricing and Emissions Trading: As carbon pricing mechanisms and emissions trading schemes are increasingly adopted, sawmills with small-scale power plants can potentially benefit from carbon credits generated through the use of biomass as a renewable fuel. These credits can be sold on carbon markets, providing additional revenue streams for sawmills while helping to offset their operational costs.
- Sustainability Certifications and Green Labeling: As consumers and businesses place greater value on sustainability, sawmills with renewable energy systems powered by waste materials may qualify for sustainability certifications or green labeling. These certifications can provide a marketing advantage, attracting environmentally conscious customers and opening up new market opportunities.
- Government Subsidies and Tax Incentives: Many governments offer tax credits, subsidies, and incentives for the installation of renewable energy systems, particularly those that utilize waste biomass. These incentives can help reduce the initial capital investment required for a small-scale power plant and improve the financial attractiveness of such projects.
- Circular Economy Initiatives: The growing focus on the circular economy promotes the idea of using waste materials as resources rather than disposing of them. Small-scale power plants that use sawmill by-products for energy generation align with these principles, helping sawmills become more sustainable and reduce their environmental impact. This alignment with circular economy initiatives can create new opportunities for sawmills to gain access to funding, partnerships, and market incentives.
5. Conclusion
The future of small-scale power plants for sawmills is bright, driven by advancements in technology, growing market demand for renewable energy, and supportive policy frameworks. The continued development of more efficient biomass conversion technologies, energy storage systems, and automation tools will enhance the performance and cost-effectiveness of these systems, making them more viable for sawmills across the globe.
By integrating renewable energy production into their operations, sawmills can not only reduce their environmental impact but also improve their financial sustainability and energy independence. The development of innovative solutions, including hybrid systems, smart grids, and predictive maintenance technologies, will ensure that small-scale power plants in sawmills continue to evolve and thrive in an increasingly sustainable energy landscape.
As the demand for green energy and sustainable production practices grows, sawmills that invest in small-scale power plants powered by biomass will be well-positioned to lead the way toward a more sustainable and energy-efficient future for the forestry industry.
Ongoing Challenges and Considerations for Small-Scale Power Plants in Sawmills
Despite the promising benefits of integrating small-scale power plants in sawmills, there are still several ongoing challenges and considerations that must be addressed for these systems to operate effectively and sustainably. These challenges revolve around technical, financial, regulatory, and operational aspects that may hinder the seamless implementation of such systems.
1. Technical Challenges
While small-scale power plants powered by sawmill by-products can offer great promise, there are several technical issues that may need to be addressed to ensure optimal performance.
- Fuel Variability and Handling: The composition of biomass feedstock from sawmills can vary significantly in terms of moisture content, size, and density. Variations in these factors can affect combustion efficiency, energy production, and emissions. For optimal performance, it’s essential that biomass fuels be processed and prepared consistently. This requires efficient fuel handling systems, such as drying and sorting technologies, to ensure that the biomass is of uniform quality. However, these systems add to the upfront cost and operational complexity of the small-scale power plant.
- Boiler and Turbine Optimization: Biomass combustion, while reliable, tends to be less efficient than natural gas or coal. The efficiency of the boiler and steam turbine system is critical to achieving the desired energy output and economic viability. While modern steam turbines can achieve high efficiency, the operation of the boiler at varying loads (based on biomass availability and demand) can lead to fluctuating performance. Sawmills may need to invest in advanced boiler designs or hybrid systems to ensure consistent and efficient energy production.
- Emissions Control: Biomass combustion produces emissions, including particulate matter, volatile organic compounds (VOCs), carbon monoxide (CO), and nitrogen oxides (NOx). These emissions must be controlled to meet local air quality standards. Installing and maintaining efficient emissions control technologies such as scrubbers, filters, and selective catalytic reduction (SCR) systems can be costly and require regular maintenance. Additionally, ensuring the proper disposal of ash and other by-products generated by combustion can create logistical challenges.
2. Financial Considerations
Small-scale power plants in sawmills can require significant capital investment, which can be a major hurdle for some operators. Several financial considerations need to be factored into the planning and implementation of these projects.
- High Initial Capital Investment: The cost of designing, installing, and commissioning a small-scale power plant can be substantial. This includes purchasing equipment such as boilers, turbines, emissions control systems, and storage tanks for biomass. The financial burden of these investments can be a barrier for smaller sawmills that may not have the available capital to make such an investment upfront. However, financing options, such as loans, grants, or government incentives, may be available to offset these costs.
- Return on Investment (ROI): The return on investment for small-scale power plants can take several years to materialize, depending on energy savings, operational efficiency, and revenue from excess power sales. Some sawmills may be hesitant to make long-term investments in renewable energy technologies if the financial benefits are not immediately clear. However, careful financial planning and cost-benefit analysis can help sawmills assess the potential for ROI based on factors such as biomass fuel costs, energy savings, and government incentives.
- Operational and Maintenance Costs: Beyond the initial investment, operating and maintaining a small-scale power plant can incur ongoing costs. Fuel costs (if biomass is not fully sourced from waste), labor for operation and maintenance, and regular servicing of boilers, turbines, and emissions control systems are all factors that need to be accounted for. These costs can impact the overall financial sustainability of the power plant, especially if energy production is inconsistent or lower than expected.
- Financial Incentives: Despite the high initial costs, there are financial incentives that can help mitigate some of the capital expenses. Governments in many regions provide renewable energy subsidies, tax credits, and grants for businesses adopting clean energy technologies. Programs such as feed-in tariffs (FiTs) or power purchase agreements (PPAs) can also help sawmills secure a stable revenue stream by selling excess energy back to the grid. These incentives are essential to making small-scale power plants financially feasible for sawmills.
3. Regulatory and Policy Barriers
Sawmills seeking to integrate small-scale power plants powered by biomass must navigate a complex regulatory landscape. Adhering to both environmental and energy regulations is essential, but policy barriers can sometimes present significant challenges.
- Permitting and Compliance: The installation of a biomass power plant often requires multiple permits and approvals from local, regional, and national authorities. These include environmental permits related to air quality, water usage, emissions, and waste disposal. The permitting process can be time-consuming, costly, and may involve compliance with stringent emissions limits that may require expensive upgrades to technology, such as advanced filtration systems.
- Grid Access and Connection: Selling excess electricity to the grid is a key component of the financial viability of many small-scale power plants. However, sawmills may face challenges when attempting to connect their power plants to the local grid. Grid operators may have specific technical or operational requirements that must be met before a small-scale power plant can be connected. Additionally, there may be fees for grid connection, which can further add to the cost of the project.
- Sustainability Regulations: As governments continue to place greater emphasis on reducing carbon emissions and promoting renewable energy, sawmills may face increasing pressure to meet sustainability targets. While biomass is considered a renewable resource, there are still concerns regarding deforestation, land use change, and the carbon footprint associated with harvesting biomass. Sawmills must ensure that their biomass feedstocks are sustainably sourced to avoid potential regulatory issues or reputational damage.
- Policy Support for Biomass: The level of policy support for biomass energy can vary greatly depending on the country or region. In some places, there may be strong incentives for renewable energy projects, while in others, the policy framework may not be as supportive. For instance, in regions where the government is reducing subsidies for biomass power generation or prioritizing other renewable energy sources such as solar or wind, the financial feasibility of small-scale biomass plants may be compromised. Lobbying for favorable policies or aligning with trade associations that support biomass energy could help sawmills navigate these challenges.
4. Operational Risks and Challenges
While small-scale power plants for sawmills offer potential long-term benefits, several operational risks and challenges must be managed to ensure the success of the project.
- Reliability of Biomass Supply: The reliability of the biomass supply is a critical factor in the continuous operation of the power plant. If a sawmill relies on external suppliers of biomass (such as wood chips or waste from other industries), disruptions in the supply chain could affect the plant’s ability to generate power. Sawmills that rely on their own by-products have more control over the fuel supply but must still deal with fluctuations in the availability of waste materials, depending on production levels.
- Energy Demand Fluctuations: Sawmills experience fluctuating energy demands based on operational cycles. During periods of high production, the demand for energy may exceed the power generated by the small-scale power plant, necessitating reliance on grid electricity. Conversely, during low production periods, excess energy may need to be stored or sold to the grid. Predicting these fluctuations and ensuring that the plant has sufficient capacity to meet peak demand is a key operational consideration.
- Long-Term Maintenance: Maintenance is a critical part of ensuring that the small-scale power plant operates efficiently and reliably. Boilers, turbines, and other equipment are subject to wear and tear, and if not properly maintained, they can lead to costly repairs, unplanned downtimes, and reduced efficiency. A well-defined maintenance schedule, along with monitoring systems that track equipment performance, is essential to minimize operational disruptions.
- Technological Advancements: As new technologies emerge, sawmills will need to continually evaluate whether their small-scale power plants require upgrades or adjustments to remain competitive and efficient. This might include integrating newer combustion technologies, improving emissions control systems, or adopting more efficient power generation methods. Staying current with technological advancements will require ongoing investment and operational adjustments, but these changes can lead to improved performance and cost savings over time.
5. Conclusion: Overcoming Challenges for Long-Term Success
While integrating small-scale power plants into sawmills offers significant environmental and financial benefits, overcoming the technical, financial, regulatory, and operational challenges will be essential for ensuring their long-term success. By focusing on advanced biomass conversion technologies, ensuring reliable fuel supply, and adhering to regulatory requirements, sawmills can harness the full potential of these systems. Financial incentives, along with the growing market demand for renewable energy, will further support the implementation and expansion of small-scale power plants in sawmills.
Ongoing research and development in the biomass sector, as well as innovations in energy storage and automation, will continue to improve the performance and sustainability of these power plants. With careful planning, investment in the right technologies, and adherence to evolving regulations, sawmills can successfully integrate small-scale power plants into their operations, helping them become more energy self-sufficient, economically competitive, and environmentally responsible.
Sustainability and Environmental Considerations for Small-Scale Power Plants in Sawmills
As sawmills continue to explore the integration of small-scale power plants, there is an increasing focus on sustainability and environmental considerations. This is particularly important in the context of rising awareness around climate change, resource depletion, and the environmental impact of industrial operations. By ensuring that their small-scale power plants are designed and operated with sustainability in mind, sawmills can not only meet regulatory requirements but also improve their corporate image, reduce operating costs, and contribute to global environmental goals.
1. Carbon Neutrality and Net Zero Targets
One of the key benefits of small-scale power plants in sawmills is their potential to contribute to carbon neutrality. Biomass energy, when sourced from renewable materials such as wood waste or sawdust, is considered carbon neutral over the long term. This means that the amount of carbon dioxide (CO2) emitted during combustion is offset by the carbon absorbed by the biomass during its growth. For sawmills, this can significantly reduce the carbon footprint of their operations compared to conventional fossil fuel-based power generation.
To align with global net-zero targets and address growing concerns about carbon emissions, sawmills can also implement additional measures to reduce their overall carbon footprint, such as:
- Carbon Capture and Storage (CCS): Some sawmills may consider adopting CCS technologies to capture and store CO2 emissions produced during biomass combustion. Though CCS is still an emerging technology, it has the potential to further reduce the environmental impact of biomass power generation.
- Carbon Offset Programs: By participating in carbon offset programs, sawmills can further reduce their net carbon emissions by investing in renewable energy projects, forest conservation, or other environmental initiatives. This can complement their efforts to operate a carbon-neutral power plant and support broader climate change mitigation efforts.
2. Circular Economy and Waste Minimization
The principles of the circular economy are highly applicable to small-scale power plants in sawmills. The circular economy emphasizes the efficient use of resources, reducing waste, and reusing by-products wherever possible. In sawmills, the integration of a small-scale power plant can help turn wood waste, sawdust, and other by-products into valuable energy, contributing to a more sustainable and efficient operation.
Sawmills can achieve waste minimization by implementing the following strategies:
- Waste-to-Energy Integration: As mentioned, biomass residues such as sawdust, wood chips, and bark can be utilized to generate power through combustion, gasification, or pyrolysis. This waste-to-energy approach reduces the need to dispose of waste materials, which would otherwise end up in landfills. This approach not only minimizes waste but also reduces landfill emissions, such as methane, which is a potent greenhouse gas.
- By-Product Utilization: In addition to generating energy, small-scale power plants can produce valuable by-products like biochar, bio-oil, or syngas. Biochar, for instance, can be used as a soil amendment, contributing to improved soil quality and carbon sequestration. The sale of these by-products can also provide additional revenue streams for sawmills, making the overall operation more financially sustainable.
- Zero-Waste Operations: Sawmills can work towards achieving zero-waste goals by creating closed-loop systems where all wood waste is utilized either as a fuel source or as a by-product for other industries. This model helps sawmills reduce the need for external waste disposal services and prevents the environmental costs associated with waste management.
3. Emission Reductions and Air Quality Improvements
While biomass combustion is considered more sustainable than fossil fuels, it still generates emissions that need to be controlled to ensure that air quality is not adversely affected. Advances in emissions control technologies can help sawmills meet environmental regulations while reducing the impact of their operations on local air quality.
Key measures for reducing emissions include:
- Advanced Combustion Technologies: New combustion technologies, such as fluidized bed combustion and circulating fluidized bed (CFB) systems, offer better efficiency and lower emissions compared to conventional biomass boilers. These technologies can achieve more complete combustion, resulting in fewer particulate emissions and a reduction in the formation of harmful compounds such as NOx and sulfur oxides (SOx).
- Air Pollution Control Systems: To meet air quality standards and reduce pollutants, sawmills can install advanced air pollution control technologies such as scrubbers, electrostatic precipitators, and baghouse filters. These systems can capture particulate matter, VOCs, and other pollutants produced during combustion, preventing them from being released into the atmosphere. Additionally, selective catalytic reduction (SCR) and low-NOx burners can be used to reduce nitrogen oxide emissions, which contribute to smog and acid rain.
- Utilizing Cleaner Biomass Feedstocks: The quality of the biomass feedstock used in power generation plays a significant role in emissions levels. Sawmills can improve air quality by sourcing higher-quality biomass that has lower moisture content, as wet biomass tends to produce more smoke and particulate matter. Pre-processing biomass to remove contaminants, such as bark, dirt, or chemicals, can also help reduce the environmental impact.
4. Water Conservation and Wastewater Management
In addition to air quality, water consumption and wastewater management are important environmental factors for sawmills with small-scale power plants. Biomass power plants often require water for cooling and steam generation, and poor management of water resources can lead to inefficiencies or negative environmental impacts.
Sawmills can improve their water management practices by implementing the following strategies:
- Closed-Loop Cooling Systems: To reduce water consumption, sawmills can use closed-loop cooling systems that recirculate water rather than discharging it. These systems minimize the need for fresh water intake and reduce the thermal pollution of nearby water sources.
- Efficient Steam Cycle Design: Small-scale power plants that use steam turbines can optimize the steam cycle to maximize energy generation and minimize water usage. Technologies such as regenerative feedwater heaters can pre-heat water before it enters the boiler, reducing the need for large amounts of makeup water.
- Wastewater Treatment and Reuse: Any wastewater produced during the operation of the power plant, such as from ash removal or cooling processes, should be treated and potentially reused for non-potable purposes. Implementing an on-site wastewater treatment system can help sawmills reduce their environmental impact and lower the costs associated with water discharge fees.
5. Biodiversity and Ecosystem Impact
The sourcing of biomass from forests for energy generation can have implications for biodiversity and ecosystem health. To ensure that small-scale power plants in sawmills contribute to sustainability rather than causing harm to ecosystems, careful sourcing and forest management practices are required.
- Sustainable Sourcing of Biomass: Sawmills should ensure that their biomass feedstocks come from sustainably managed forests and certified sources such as those recognized by the Forest Stewardship Council (FSC). This includes avoiding biomass sourced from primary forests or ecologically sensitive areas. By adhering to sustainable sourcing standards, sawmills can ensure that their biomass supply does not contribute to deforestation or habitat loss.
- Agroforestry and Land Restoration: Sawmills can support biodiversity by sourcing biomass from agroforestry projects or lands that are being restored. These projects combine forestry with agricultural practices, providing habitat for wildlife while ensuring the long-term health of ecosystems. Additionally, sawmills can invest in reforestation and afforestation programs that contribute to carbon sequestration and ecosystem regeneration.
- Lifecycle Assessments (LCA): Sawmills should conduct lifecycle assessments (LCA) of their biomass power generation systems to evaluate the environmental impact from the cradle to the grave. This can help identify opportunities for reducing the environmental footprint of the entire system, from the sourcing of biomass to the end-of-life disposal of by-products.
6. Conclusion: Enhancing Environmental Performance for Long-Term Sustainability
As small-scale power plants in sawmills become more widespread, it is essential to prioritize sustainability and environmental performance. Through the adoption of advanced technologies for emission control, fuel efficiency, water management, and biomass sourcing, sawmills can significantly reduce their environmental impact and contribute to global sustainability goals.
By aligning with circular economy principles, minimizing waste, and improving energy efficiency, sawmills can make a positive contribution to both their bottom line and the planet. Furthermore, regulatory pressures and market demand for sustainable practices will likely continue to drive innovation in the biomass energy sector, ensuring that small-scale power plants in sawmills remain a viable and environmentally responsible energy solution.
Sawmills that invest in cleaner, more efficient technologies and align with sustainability goals will be well-positioned to thrive in the evolving energy landscape, providing renewable energy while minimizing environmental harm and promoting sustainable forestry practices.
Small Scale Power Plant for Off-the-Grid Homes
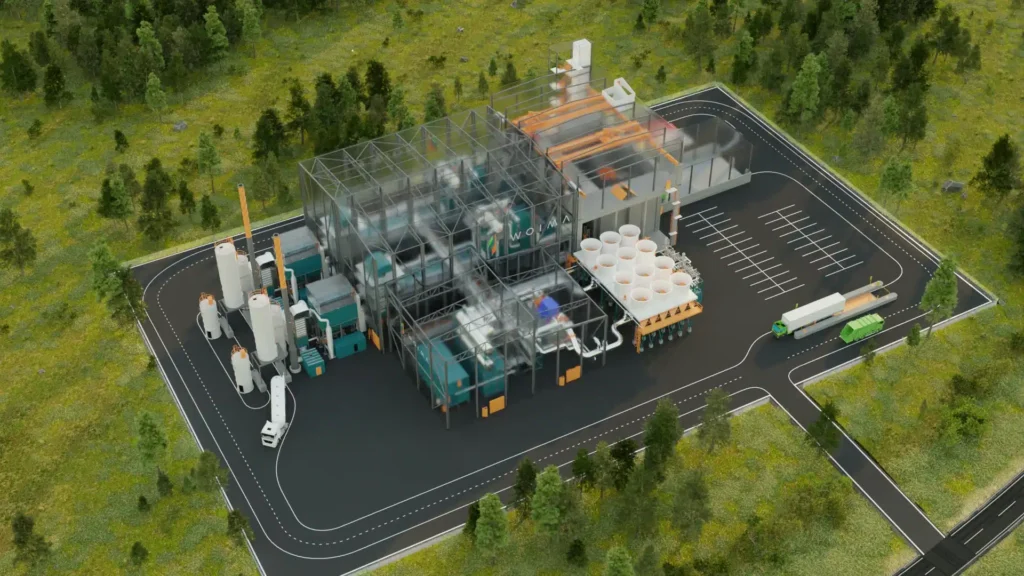
In many rural and remote areas where access to centralized power grids is limited or nonexistent, small-scale power plants are proving to be a highly effective solution for off-the-grid homes. These power plants can generate energy from renewable sources such as solar, wind, biomass, and even small-scale hydroelectric systems, providing an autonomous and sustainable energy supply for homeowners in isolated locations. Such systems not only offer energy security but also contribute to reducing reliance on fossil fuels and lowering carbon footprints.
1. Energy Requirements and Planning
The first step in designing a small-scale power plant for off-the-grid homes is to determine the energy requirements of the home. These energy needs are influenced by factors such as the number of people living in the home, the type of appliances and devices used, heating and cooling requirements, and any other specific demands for energy.
For example:
- Basic Load: Includes lighting, refrigeration, communication devices, and basic electrical appliances.
- High-Load Devices: Includes heating, air conditioning, water pumping systems, and power-hungry appliances such as washing machines or dryers.
- Peak Demand: This is the highest energy demand at any given time, which needs to be factored in to avoid overloads or power interruptions.
Designing a system that accounts for all these variables ensures the power plant can meet the home’s energy needs at all times. The sizing of the energy system must balance between providing enough power during the day and storing excess energy for use at night or during periods of low generation.
2. Renewable Energy Sources for Off-the-Grid Homes
Several renewable energy sources are well-suited to power off-the-grid homes. The choice of source depends on geographical location, available resources, climate, and the home’s energy requirements. These sources include solar energy, wind energy, micro-hydro systems, and biomass.
- Solar Power: Solar energy is one of the most widely used and accessible renewable energy sources for off-the-grid homes. Photovoltaic (PV) panels can be installed on rooftops or nearby land, where sunlight exposure is optimal. Solar power systems are relatively easy to maintain, and battery storage can be used to store excess energy produced during the day for use at night or on cloudy days. The simplicity and scalability of solar power systems make them a popular choice for off-the-grid homes.
- Hybrid Solar-Wind Systems: In areas with inconsistent sunlight but strong winds, combining solar panels with small wind turbines can help ensure a more stable energy supply. Wind turbines generate electricity when wind speeds are adequate, and solar panels provide energy during the day. Hybrid systems can be designed with energy storage solutions (batteries) to balance production and consumption.
- Wind Power: Wind energy can be harnessed through small wind turbines. These systems are ideal in areas with consistent wind, such as coastal regions or open plains. Wind turbines generate electricity by capturing the kinetic energy of the wind, which can be stored in batteries for later use. Wind power systems require careful consideration of wind speed, site location, and turbine capacity.
- Micro-Hydro Power: For off-the-grid homes near streams or rivers, micro-hydro power systems can provide a continuous and reliable energy supply. These systems generate electricity by harnessing the energy of flowing water through a small turbine connected to a generator. Micro-hydro systems are ideal in areas with consistent water flow but can be costly and require careful planning to ensure minimal environmental impact and proper water rights management.
- Biomass: In regions where wood or organic waste is readily available, biomass energy systems can be used to generate heat or electricity. Biomass can be burned in a small-scale biomass boiler to produce steam, which powers a steam turbine connected to a generator. Alternatively, biomass can be converted into biogas through anaerobic digestion, which can then be used to generate electricity. Biomass systems are highly efficient and sustainable, especially in areas where agricultural residues or wood waste are abundant.
3. System Configuration and Integration
The integration of renewable energy sources into a small-scale power plant for off-the-grid homes requires careful planning of the energy generation and storage systems. Key components of these systems include:
- Energy Generation: This involves the power production unit (solar panels, wind turbines, micro-hydro turbine, or biomass boiler), which converts the renewable resource into electrical energy. Depending on the selected power generation technology, homeowners may choose to use a single source or combine multiple sources for hybrid systems.
- Energy Storage: To ensure a continuous power supply when the renewable source is not producing electricity (e.g., at night for solar, during periods of low wind or low water flow), energy storage is crucial. Batteries such as lithium-ion, lead-acid, or saltwater batteries are commonly used in off-the-grid systems. These batteries store excess energy produced during times of peak generation, making it available when demand exceeds generation.
- Battery Sizing: Proper battery sizing is essential for ensuring the system has enough capacity to meet the energy needs during times of low generation. Sizing the batteries too small may result in insufficient energy during periods of low production, while oversized batteries may incur unnecessary costs.
- Inverters and Charge Controllers: Inverters are used to convert the DC (direct current) electricity generated by renewable sources (like solar panels or wind turbines) into AC (alternating current) electricity for use by standard household appliances. Charge controllers ensure that the batteries are charged safely and do not exceed their voltage limits, preventing damage and prolonging their lifespan.
- Backup Generators: In some cases, a backup generator powered by fossil fuels (gasoline, diesel, or propane) may be used as a backup power source when renewable generation is insufficient. While this adds to the operational cost, it ensures that the home has a reliable power supply even during extended periods of adverse weather conditions or low energy production.
4. Energy Management and Automation
Small-scale power plants for off-the-grid homes often include energy management systems (EMS) that help monitor and optimize the use of generated electricity. These systems can:
- Monitor Energy Consumption: EMS can track the amount of electricity consumed by various appliances, enabling homeowners to identify high-energy devices and manage usage more efficiently.
- Optimize Energy Generation and Storage: An EMS can help optimize the charging and discharging cycles of batteries, ensuring that energy storage is used efficiently and preventing overcharging or deep discharge, which could damage the batteries.
- Automate Power Distribution: Energy management systems can automatically adjust power distribution based on the availability of renewable energy and the storage state. For instance, during periods of low solar production, the system can prioritize the use of battery power or switch to a backup generator if necessary.
5. Economic Feasibility and Cost Considerations
While the initial installation of a small-scale power plant for off-the-grid homes can be expensive, it can offer long-term financial benefits, especially in areas with high energy costs or unreliable grid access.
- Upfront Investment: The cost of installing a renewable energy system includes the purchase of renewable energy generation units (solar panels, wind turbines, biomass systems, etc.), energy storage systems (batteries), inverters, charge controllers, wiring, and installation services. Depending on the system size, location, and selected technologies, the upfront cost can range from several thousand to tens of thousands of dollars.
- Operational Costs: Once installed, the ongoing operational costs for off-the-grid power plants are relatively low, especially for systems that rely solely on renewable resources like solar, wind, or micro-hydro. Maintenance costs typically include occasional cleaning of solar panels, turbine servicing, battery maintenance or replacement, and possibly generator fuel costs. Biomass systems also require fuel sourcing (wood chips, agricultural waste, etc.), which can add to operational costs.
- Return on Investment (ROI): The ROI of a small-scale off-the-grid power plant depends on the initial investment, available incentives, and the savings achieved by avoiding monthly grid connection fees. In regions where grid electricity is expensive or unreliable, off-the-grid solutions can provide significant savings over time, often within 5-10 years.
- Government Incentives: Many governments offer incentives, grants, or subsidies for renewable energy projects, making off-the-grid solutions more financially accessible. These incentives can significantly reduce the upfront costs and improve the ROI of renewable energy systems for homeowners.
6. Environmental and Sustainability Benefits
Off-the-grid power plants are inherently more sustainable than conventional grid-based systems, as they rely on renewable resources, reduce greenhouse gas emissions, and minimize environmental impact.
- Reduction in Carbon Footprint: By replacing fossil fuel-based power sources with renewable energy, off-the-grid homes can significantly reduce their carbon footprint. Solar, wind, and micro-hydro systems produce little to no greenhouse gas emissions during operation, making them environmentally friendly options for energy generation.
- Reduced Environmental Impact: Small-scale power plants help reduce the environmental impact associated with traditional grid power, which often relies on coal, natural gas, or oil, all of which have significant negative environmental effects, such as air pollution, habitat destruction, and resource depletion.
- Resource Efficiency: By utilizing local, renewable resources, off-the-grid homes contribute to resource conservation and the sustainable management of natural resources, such as sunlight, wind, and water.
7. Conclusion
Small-scale power plants for off-the-grid homes offer a sustainable and reliable energy solution for remote areas where grid connectivity is unavailable or unreliable. By harnessing renewable energy sources such as solar, wind, biomass, or micro-hydro, homeowners can reduce their reliance on fossil fuels, lower their carbon footprint, and achieve energy independence. With careful planning, proper system integration, and consideration of economic feasibility, off-the-grid power plants can provide long-term environmental and financial benefits, making them an attractive option for individuals seeking a self-sufficient and sustainable lifestyle.
Future Trends and Innovations in Small-Scale Power Plants for Off-the-Grid Homes
As technology continues to evolve, small-scale power plants for off-the-grid homes are expected to undergo significant advancements that will improve efficiency, cost-effectiveness, and integration with other smart home technologies. These trends not only promise to make off-the-grid living more accessible but also enhance the overall sustainability and performance of renewable energy systems.
1. Advanced Energy Storage Solutions
One of the biggest challenges for off-the-grid power systems is ensuring a reliable energy supply when renewable sources are not producing power, such as during cloudy days for solar systems or calm periods for wind energy. Advances in energy storage technology will play a crucial role in overcoming this issue by improving the capacity, cost, and lifespan of storage systems.
- Solid-State Batteries: Solid-state batteries are emerging as a potential game-changer in energy storage. Unlike traditional lithium-ion batteries, solid-state batteries use a solid electrolyte instead of a liquid one, making them safer, more efficient, and capable of holding more energy in a smaller space. This technology promises to significantly reduce the size and cost of energy storage systems for off-the-grid homes.
- Flow Batteries: Flow batteries are another promising energy storage technology that could be ideal for off-the-grid applications. These batteries use two liquid electrolytes stored in separate tanks and pump them through a reactor to generate electricity. Flow batteries have the advantage of being easily scalable and long-lasting, making them suitable for homes that require large amounts of energy storage.
- Long-Duration Energy Storage: As the demand for clean and reliable energy grows, technologies for long-duration energy storage (LDES) are being developed. These systems can store energy for days or even weeks, which is essential for regions with extended periods of low sunlight or wind. By providing longer energy storage capacities, LDES could help eliminate the need for fossil fuel backup generators and increase the resilience of off-the-grid power systems.
2. Smart Grids and Demand Response
The integration of smart grids and demand response systems into off-the-grid homes is expected to revolutionize the way energy is managed and consumed. These systems allow for real-time monitoring, communication, and control of energy production, storage, and consumption.
- Smart Metering: Smart meters can track energy usage in real time, providing homeowners with detailed insights into their consumption patterns. This data can be used to optimize energy usage and identify opportunities for savings, such as shifting energy-intensive activities to times of day when renewable generation is at its peak (e.g., during midday for solar).
- Energy Management Systems (EMS): Advanced EMS platforms can automatically control and optimize the distribution of energy across different sources (solar, wind, batteries) to ensure the most efficient and cost-effective operation. These systems can balance energy demand and supply dynamically, making adjustments as needed to prevent shortages or waste.
- Automated Demand Response: Demand response systems can help off-the-grid homes automatically adjust their energy consumption in response to external signals, such as weather patterns or energy supply fluctuations. For example, if a solar system is producing excess energy, the system might automatically switch on non-essential appliances to store more energy in the batteries.
- Decentralized Energy Trading: As renewable energy production becomes more decentralized, peer-to-peer (P2P) energy trading platforms are being developed to allow off-the-grid homes to sell surplus energy to others. Homeowners with excess solar energy can trade it with nearby off-the-grid homes or even contribute to a community energy grid. This not only helps to make the most of available renewable resources but also creates a potential source of income for homeowners.
3. Integration with Electric Vehicles (EVs)
Electric vehicles (EVs) are rapidly becoming an integral part of the renewable energy ecosystem, especially in off-the-grid communities. As the number of EVs grows, homeowners can take advantage of their large battery storage capacity to further enhance the reliability and resilience of their small-scale power systems.
- Vehicle-to-Grid (V2G) Technology: V2G technology allows energy to flow both ways between EVs and the home’s power system. This means that EVs can act as additional energy storage units, feeding power back into the home or the grid when needed. For off-the-grid homes, this technology could serve as a backup power source during periods of low renewable generation, making it easier to balance energy demand.
- Mobile Power Supply: In the future, EVs could provide off-the-grid homes with mobile power supply during emergencies or power outages. Homeowners could charge their EVs when renewable generation is plentiful and then use the vehicle’s stored energy for backup during periods of high demand or low generation. This would eliminate the need for additional backup generators or reliance on external energy sources.
4. Increased Use of Artificial Intelligence (AI) and Machine Learning (ML)
The role of artificial intelligence (AI) and machine learning (ML) is set to expand in the management and optimization of off-the-grid power plants. AI and ML can help automate energy management systems, predict energy needs, and optimize the integration of renewable energy sources.
- Predictive Analytics: AI and ML algorithms can analyze historical data from solar and wind production, weather patterns, and energy consumption to predict future energy needs. This enables off-the-grid homes to better plan their energy storage and usage, ensuring they always have enough power available while minimizing energy waste.
- Autonomous Energy Management: AI-driven systems can autonomously adjust the power generation and storage process based on real-time data. For example, if an AI system detects a period of low sunlight or wind, it could optimize the use of stored energy or adjust the load of electrical appliances to reduce demand.
- Energy Efficiency Optimization: AI and ML can also be used to monitor energy consumption patterns and recommend energy-saving practices. Homeowners may receive tailored suggestions to reduce energy consumption, such as optimizing the use of appliances, adjusting heating/cooling schedules, or improving insulation. Over time, these adjustments can lead to significant cost savings and a reduction in energy consumption.
5. Modular and Scalable Systems
The trend toward modular and scalable power systems is expected to grow in popularity for off-the-grid homes. These systems allow homeowners to start small and scale up their energy generation and storage capacity as needed, making them more affordable and adaptable to changing needs.
- Modular Solar Power Systems: Homeowners can begin by installing a small solar system and gradually expand it by adding additional panels as their energy demands increase. Modular systems are easy to install and expand, providing flexibility as off-the-grid homes grow or as energy needs change.
- Scalable Wind and Hydro Systems: Similarly, small wind and hydro systems can be installed in stages. Homeowners can start with a small wind turbine or micro-hydro setup and expand it as resources permit or as their energy demands increase. This scalability allows for a cost-effective and customizable solution for off-the-grid living.
- Integrated Solutions for Multiple Energy Sources: Companies are also developing integrated systems that combine multiple renewable energy sources (e.g., solar, wind, biomass) into a single modular unit. These systems are designed to be easily scaled to meet the energy needs of off-the-grid homes, ensuring a more reliable and balanced power supply throughout the year.
6. New Financing Models
The upfront cost of installing a small-scale power plant can be a barrier for many homeowners, especially those in rural or off-the-grid locations. As the renewable energy industry grows, new financing models are emerging to make off-the-grid power systems more accessible to a wider range of people.
- Solar Leasing and Power Purchase Agreements (PPAs): Solar leasing and PPAs allow homeowners to install solar systems with little or no upfront cost. Under these agreements, homeowners pay for the electricity generated by the system rather than owning the system itself. This makes renewable energy more accessible to people who might otherwise be unable to afford the initial investment.
- Community Energy Programs: Community energy initiatives allow groups of homeowners to pool resources and invest in shared off-the-grid power systems. This approach reduces individual costs and enables communities to collectively benefit from renewable energy. It can also promote energy independence on a larger scale by enabling rural or remote communities to become self-sufficient in terms of energy production.
- Green Financing: As the demand for renewable energy grows, financial institutions are increasingly offering green loans and environmentally responsible financing options for off-the-grid power systems. These financing models often come with favorable terms and lower interest rates for projects that contribute to environmental sustainability.
7. Conclusion: A More Sustainable Future for Off-the-Grid Homes
The future of small-scale power plants for off-the-grid homes is bright, with a combination of new technologies, innovative financing models, and increased integration of renewable resources making it easier and more affordable for homeowners to live off the grid.
As energy storage improves, renewable energy systems become more efficient, and new technologies like AI and electric vehicles help optimize energy usage, off-the-grid homes will become more self-sufficient, reliable, and sustainable. The ongoing evolution of these systems is expected to pave the way for a future where off-the-grid living is not only viable but also a mainstream choice for homeowners seeking energy independence and sustainability.
These advancements will play a critical role in reducing our reliance on fossil fuels, mitigating climate change, and promoting a sustainable energy future for generations to come.
Challenges and Considerations for Small-Scale Power Plants for Off-the-Grid Homes
While small-scale power plants offer significant advantages for off-the-grid homes, there are several challenges and considerations that need to be addressed to ensure that these systems are reliable, efficient, and cost-effective. These challenges span across technological, economic, and environmental factors and must be carefully managed to achieve the desired outcome.
1. Initial Capital Investment
The upfront capital required to install a small-scale power plant can be significant, especially for homeowners who want to adopt a complete renewable energy system that includes energy generation (solar panels, wind turbines, etc.), energy storage (batteries), and additional components like inverters and charge controllers. The cost of these systems varies depending on factors such as location, system size, and technology choice.
- Solar Power Systems: The initial cost of solar panels and the necessary installation can be high, especially if the home is large or located in an area with low sunlight. However, solar energy systems can pay for themselves over time through energy savings, making them a long-term investment.
- Wind and Hydro Power Systems: Wind and micro-hydro systems, though often providing higher and more consistent energy generation than solar, can also be expensive to install. Wind turbines, for example, require significant installation costs for both the equipment and the infrastructure needed to support it, such as towers and wiring. Micro-hydro systems require access to a water source, which may limit their feasibility in some locations.
- Energy Storage: Batteries are one of the highest costs in off-the-grid power systems. High-quality batteries, such as lithium-ion, are efficient and long-lasting, but they come with a hefty price tag. Over the long term, battery costs have been decreasing, but they still represent a significant portion of the total system cost.
While the financial burden of initial investments can be eased through various financing options (e.g., green loans, leasing models, and subsidies), the upfront cost remains a challenge for many homeowners looking to adopt off-the-grid energy systems.
2. Energy Production and Weather Variability
One of the main challenges with renewable energy systems, particularly for off-the-grid homes, is the variability of energy production due to weather conditions. Solar, wind, and hydro systems all depend on natural resources, which can fluctuate based on seasonal changes, local weather patterns, and unforeseen events.
- Solar Variability: Solar panels produce energy based on sunlight, which can be affected by cloud cover, fog, and seasonal changes. Areas with less consistent sunlight, such as regions with long winters or frequent overcast weather, may find it more challenging to rely solely on solar energy for power generation.
- Wind Variability: Wind energy production is highly dependent on wind conditions, which can be inconsistent and unpredictable. While some regions experience constant wind, others may have periods of low wind speeds, limiting energy generation from wind turbines.
- Hydro Variability: Micro-hydro systems depend on water flow, which can fluctuate based on rainfall, snowmelt, and other environmental factors. In areas with seasonal water availability or drought conditions, maintaining a steady supply of energy from micro-hydro systems can be difficult.
These fluctuations in energy generation require energy storage to ensure consistent and reliable power. Battery storage systems must be sized appropriately to meet the home’s energy demand during periods when renewable generation is low.
3. Maintenance and System Longevity
Another challenge for small-scale off-the-grid power plants is the ongoing maintenance required to ensure systems operate efficiently over time. Unlike grid-connected systems, off-the-grid power plants operate independently, which means the homeowner is responsible for managing system upkeep, troubleshooting, and repairs.
- Solar Panels: Solar panels are relatively low-maintenance, but they need to be cleaned periodically to maintain efficiency. Dust, dirt, and snow buildup can reduce the amount of sunlight absorbed by the panels. In addition, older solar panels may lose efficiency over time, requiring replacement or upgrades.
- Wind Turbines: Wind turbines require regular maintenance to keep the blades and moving parts in good condition. Bearings, controllers, and electrical connections may wear out over time, requiring attention. In addition, turbine performance can degrade if the wind speeds are inconsistent, leading to lower-than-expected energy production.
- Micro-Hydro Systems: Micro-hydro systems require periodic inspection to ensure that the turbine, pipes, and other components are functioning properly. Additionally, sediment buildup in water sources, debris, or flooding can damage the equipment, leading to higher maintenance needs.
- Battery Systems: Batteries require careful management to maximize their lifespan. Deep discharges and overcharging can degrade battery health. Batteries also degrade over time, losing capacity and efficiency. Regular maintenance and replacement of aging batteries are necessary to keep the system running smoothly.
Proper maintenance schedules and regular system checks are essential for ensuring that off-the-grid systems provide reliable and efficient energy. Failure to do so can lead to performance degradation, energy shortages, and increased costs.
4. Site Location and Infrastructure
Choosing the right location for a small-scale power plant is crucial for maximizing the efficiency and effectiveness of the system. For off-the-grid homes, factors like terrain, access to renewable resources, and infrastructure availability play a major role in determining the success of the power system.
- Solar Location: Solar panels need to be installed in areas with optimal exposure to sunlight, which is influenced by geographical location, local weather conditions, and shading from trees, buildings, or other structures. A home located in a shaded area may not be ideal for solar power generation unless significant efforts are made to clear the area or install a ground-mounted system.
- Wind Location: Wind turbines require consistent wind speeds, typically in the range of 10–20 mph (16–32 km/h), to generate power efficiently. Homes located in areas with insufficient wind may not be able to benefit from wind turbines unless they are situated in elevated locations or near coastal areas where wind patterns are more predictable.
- Hydro Location: Micro-hydro systems require access to flowing water. This can be a major limitation in dry areas or regions where rivers or streams are seasonal. The feasibility of installing a micro-hydro system depends on the availability of a suitable water source with a steady flow, as well as local regulations regarding water rights and environmental protection.
- Infrastructure: Off-the-grid homes may face challenges when it comes to installing infrastructure for energy systems. For example, running electrical wiring for solar or wind power from a remote location can be costly. Micro-hydro systems may require the construction of channels or pipelines, which can be complex and expensive.
Ensuring that the location is ideal for the chosen renewable energy technology is critical to maximizing energy production and avoiding costly infrastructure investments. Homeowners may need to assess site conditions, consider alternatives, or invest in professional advice to optimize their system.
5. Regulatory and Legal Issues
Off-the-grid power systems are often subject to a range of local, state, or national regulations and legal considerations, particularly when it comes to the installation of renewable energy technologies.
- Permitting and Zoning: In some areas, homeowners may need permits to install solar panels, wind turbines, or micro-hydro systems. Local zoning laws may restrict the size, placement, or type of renewable energy systems allowed on residential properties. Additionally, environmental permits may be required for systems that affect water bodies (e.g., micro-hydro systems) or wildlife (e.g., wind turbines).
- Grid Connection and Backup Systems: In some regions, even off-the-grid homes may need to comply with regulations related to grid connection, especially if they use backup generators or participate in community energy trading. Homeowners must understand the legal implications of their energy systems and whether they are allowed to sell excess power to the grid or to neighboring homes.
- Safety Standards: Renewable energy systems must meet certain safety standards to ensure that they operate safely for both the homeowner and the surrounding community. Electrical systems, batteries, and generators must be installed according to local building codes and fire safety regulations. Non-compliance with these standards can lead to legal complications, fines, and safety hazards.
Understanding and navigating the regulatory landscape is essential for homeowners who want to build small-scale power plants for off-the-grid homes. Professional assistance from legal experts or renewable energy consultants can help streamline the process and ensure compliance.
6. Technological Integration and Compatibility
As off-the-grid homes become more reliant on multiple sources of renewable energy, integration of different technologies becomes an important factor in system efficiency. Ensuring that solar panels, wind turbines, batteries, and other components work together seamlessly is critical to the performance of the power plant.
- Compatibility of Components: When designing an off-the-grid power system, it’s important to select compatible components that can efficiently communicate with each other. For example, inverters must be matched with the type of renewable energy source to convert energy correctly from DC to AC. Battery systems must be compatible with the energy generation source and the home’s energy management system.
- System Integration: Integrating renewable energy sources into a unified system can be complex, especially when using hybrid systems that combine multiple technologies (e.g., solar, wind, biomass). Homeowners may need specialized control systems to balance the power input and optimize energy usage based on the availability of each resource.
- Smart Technologies: As off-the-grid homes become more technologically advanced, the integration of smart technologies like home automation systems, AI-driven energy management, and advanced monitoring solutions will become more common. These technologies allow homeowners to track energy production, storage, and consumption in real time, but they also require a certain level of technical expertise to install, operate, and maintain.
7. Conclusion
Small-scale power plants for off-the-grid homes offer a promising path to energy independence and sustainability, but they come with a variety of challenges and considerations that need to be carefully addressed. From high initial costs to weather variability and regulatory issues, homeowners must carefully plan and invest in systems that meet their energy needs and ensure reliable operation over time.
By taking a proactive approach to system design, site selection, and ongoing maintenance, off-the-grid homeowners can overcome these challenges and enjoy the benefits of clean, renewable energy. As technological advancements continue to improve the efficiency and affordability of renewable energy systems, the future of off-the-grid living looks brighter than ever.
Small Scale Power Plant for Off-the-grid Homes
8. Energy Efficiency and Load Management
For off-the-grid homes, ensuring efficient energy usage is essential to minimizing waste, reducing the size of the required energy system, and maximizing the available energy generated. Homes that rely on small-scale power plants must have efficient energy consumption practices in place to avoid exceeding their generation capacity, especially when relying on intermittent renewable sources like solar or wind.
- Energy-Efficient Appliances: Off-the-grid homes often use energy-efficient appliances that consume less electricity while providing the same functionality. Energy-efficient lighting (LED bulbs) and appliances (e.g., refrigerators, washers, dryers) are crucial in ensuring that the energy generated by the small-scale power plant is used effectively. In particular, LED lights consume significantly less power than traditional incandescent bulbs, allowing homes to run multiple lights without draining the power supply.
- Insulation and Building Design: The design and insulation of the home can drastically reduce the need for heating and cooling, which often accounts for a significant portion of a home’s energy usage. Proper insulation and passive solar design reduce energy demand and improve the overall energy efficiency of the building. Off-the-grid homes often incorporate natural ventilation, thermal mass, and the use of materials that help maintain a comfortable temperature without the need for excessive electrical heating or cooling.
- Load Management: Load management systems can help off-the-grid homes monitor and control energy usage, ensuring that power is available when it’s needed most. These systems manage when high-demand appliances are turned on (e.g., water heaters, washing machines) to avoid overloading the power generation system. Load management systems can also prioritize the most critical systems, such as refrigeration or medical equipment, ensuring that they always have access to power.
- Energy Usage Awareness: Homeowners need to be aware of their energy usage patterns, adjusting their consumption based on the availability of power. For instance, off-the-grid homes may rely on solar panels to generate energy during the day, but they may need to conserve energy in the evenings when solar energy is unavailable. Having smart energy management systems can help provide real-time feedback and help users adjust accordingly.
9. Hybrid Systems for Enhanced Reliability
While a single renewable energy source might suffice for some off-the-grid homes, hybrid systems that combine multiple energy generation technologies often offer enhanced reliability and resilience. These systems typically involve two or more energy sources, such as solar and wind, combined with energy storage, to ensure a continuous power supply regardless of the vagaries of weather or seasonal changes.
- Solar and Wind Hybrid Systems: A combination of solar panels and wind turbines can provide a more reliable energy supply. Solar energy typically peaks during daylight hours, while wind energy may be more available in the evening or nighttime. By integrating both, the system ensures that energy is continuously available, even if one source is temporarily unavailable. In some cases, wind turbines can even produce energy when solar production is low due to overcast or rainy conditions.
- Solar and Micro-Hydro Hybrid Systems: In areas with access to flowing water, micro-hydro systems can be combined with solar power to create a hybrid system. Solar panels provide energy during the day, while the micro-hydro system offers a steady and reliable power supply around the clock, as long as the water source is consistent. In regions where water flow remains constant throughout the year, a micro-hydro system can significantly reduce the reliance on solar and wind, making the off-the-grid home even more energy independent.
- Energy Storage in Hybrid Systems: Energy storage systems, such as batteries, are essential for hybrid systems, as they store excess energy generated during periods of high production for use during low-production times. In hybrid systems, battery storage can help balance the energy inputs from the various renewable sources, ensuring that power is available even during low-production times or peak demand. Battery technology has improved significantly over recent years, with options such as lithium-ion batteries offering long life and high efficiency.
- Backup Generators: While not considered part of the renewable energy generation system, backup generators can be used in conjunction with hybrid systems to ensure power supply during long periods of low renewable generation (e.g., days of continuous rain or windless weather). These generators are typically fueled by diesel, propane, or natural gas and can be automatically turned on when battery levels are low. However, the use of backup generators should be minimized to reduce reliance on fossil fuels and maintain the sustainability of the off-the-grid system.
10. Community and Shared Energy Systems
In some cases, off-the-grid homes may find it beneficial to join a larger community energy system, where multiple homes share resources and infrastructure for energy generation and storage. This concept, sometimes referred to as microgrids or community-based renewable energy systems, allows homes to pool their energy resources and share the benefits of large-scale renewable energy generation, without needing to connect to the traditional utility grid.
- Microgrids: A microgrid is a local energy system that can operate independently or in parallel with the grid. In off-the-grid communities, microgrids allow several homes or buildings to combine their renewable energy resources, such as solar panels or wind turbines, into a shared power system. This creates a more reliable and consistent energy supply by pooling resources and enabling energy-sharing between homes. Microgrids also allow excess energy to be stored collectively in batteries and used by other homes when needed.
- Community Solar Projects: In regions where individual homeowners may not have the space or resources for large solar installations, community solar projects can provide a shared solar power system that multiple households can access. By installing solar panels in a central location, such as a shared rooftop or a nearby field, several homes can benefit from the clean energy generated by the system. The generated energy can be distributed to individual homes based on their consumption or through a subscription model.
- Shared Energy Storage: In communities with multiple off-the-grid homes, shared energy storage solutions can help maximize energy reliability and reduce costs. Large-scale battery systems or other forms of energy storage can be used to store excess energy generated during the day, and the stored energy can be shared with the community when demand peaks or generation falls. Shared storage systems allow for better utilization of renewable resources and ensure that energy is available even during long periods of low generation.
- Grid Independence for Communities: These community-based systems are often designed to operate without relying on the central grid, ensuring that all homes in the community are energy independent. However, in some cases, the community microgrid may still be connected to the grid, providing an emergency backup or enabling homes to sell excess power back to the grid during periods of high production. This hybrid approach offers the benefits of renewable energy while maintaining the security of the grid when needed.
11. Future Trends in Small-Scale Power Plants for Off-the-grid Homes
As technology continues to evolve, the potential for small-scale power plants for off-the-grid homes is expanding. Several trends are likely to shape the future of off-the-grid energy systems, making them more efficient, affordable, and widely adopted.
- Advances in Energy Storage: Energy storage technology is one of the most significant areas of development for off-the-grid systems. The cost of batteries is steadily decreasing, while their efficiency and lifespan continue to improve. Solid-state batteries, flow batteries, and other emerging storage technologies may further increase the efficiency of energy storage and reduce the cost for homeowners. These advancements could make off-the-grid living more feasible for a larger number of households.
- Smart Grid Integration: As smart grid technology advances, off-the-grid homes may be able to more effectively integrate with larger grids while still maintaining their energy independence. Smart grids use digital technology to improve the efficiency of electricity distribution, allowing homes to monitor and adjust energy usage in real-time. Off-the-grid homes that connect with the smart grid could sell excess energy back to the grid or take advantage of grid power during low-generation periods, creating a more flexible and sustainable energy system.
- Blockchain for Energy Trading: The rise of blockchain technology offers the potential for decentralized energy trading among off-the-grid homes and small-scale power plants. Homeowners could use blockchain-based platforms to sell excess energy directly to other homes or communities, cutting out traditional utilities and intermediaries. This technology could offer a way for off-the-grid homeowners to earn income from their excess power generation while contributing to a decentralized, sustainable energy future.
- Increased Affordability and Accessibility: As renewable energy technologies become more mainstream and manufacturing scales up, the cost of components such as solar panels, wind turbines, and batteries will continue to fall. As a result, off-the-grid systems are likely to become more affordable and accessible to a broader range of people. Government incentives, subsidies, and tax credits will also play a crucial role in making these systems more economically viable for homeowners in both developed and developing regions.
12. Conclusion
Small-scale power plants for off-the-grid homes offer a sustainable and flexible way to provide reliable energy without relying on traditional utility grids. By leveraging renewable energy sources such as solar, wind, hydro, and bioenergy, these systems can reduce homeowners’ dependence on fossil fuels and promote environmental stewardship. However, the successful implementation of small-scale power plants requires careful planning, significant upfront investment, and ongoing management to ensure that they provide continuous, reliable power.
In the future, advances in energy storage, smart grid technology, and community-based energy systems will make it easier for homeowners to enjoy the benefits of off-the-grid living. With the right combination of technology, planning, and awareness, off-the-grid homes can play a crucial role in creating a more sustainable, self-reliant energy future.
13. Environmental Benefits and Sustainability
One of the main driving forces behind the adoption of small-scale power plants for off-the-grid homes is their positive impact on the environment. By utilizing renewable energy sources such as solar, wind, and biomass, these systems offer a significant reduction in the reliance on fossil fuels, which are the primary contributors to climate change. Off-the-grid power systems help mitigate the environmental harm caused by traditional energy production and foster a more sustainable energy model.
- Reduction in Carbon Emissions: Fossil fuel-powered electricity generation is one of the largest sources of carbon dioxide (CO2) emissions worldwide. By adopting renewable energy solutions, off-the-grid homes can drastically reduce or even eliminate their carbon footprint. Solar and wind energy, for instance, generate zero emissions during operation, and even micro-hydro systems emit negligible greenhouse gases. Homes that rely on small-scale power plants contribute to lowering global emissions, which is vital for tackling climate change.
- Resource Conservation: Off-the-grid systems, especially those based on solar, wind, and hydropower, make use of natural, abundant resources that are renewable and not subject to depletion. Unlike fossil fuels, which take millions of years to form and can become exhausted, the energy from the sun, wind, and water is replenished daily and sustainably. This makes off-the-grid power generation a far more reliable and eco-friendly option for meeting energy needs in the long run.
- Waste-to-Energy Solutions: In some off-the-grid communities, waste-to-energy systems can be integrated to further reduce environmental impacts. Biomass from agricultural waste, forestry by-products, or even municipal waste can be converted into biogas or used as a fuel source in small-scale power plants. This helps reduce the volume of waste that would otherwise go to landfills, while providing a valuable source of energy for off-the-grid homes.
- Promoting Biodiversity: Renewable energy technologies, particularly solar and wind power, have a lower environmental footprint compared to traditional fossil fuel-based energy generation. They do not involve destructive mining processes or the release of harmful pollutants into the air and water. As a result, these systems help preserve natural habitats and ecosystems, promoting biodiversity by reducing pollution levels and the need for large-scale industrial energy infrastructure.
14. Social and Economic Impact
Small-scale power plants not only benefit the environment but can also positively impact the social and economic fabric of communities that rely on them. By providing a reliable source of energy to off-the-grid homes, these systems can improve the quality of life, create new economic opportunities, and promote energy independence.
- Energy Independence: One of the most significant advantages of off-the-grid power systems is energy independence. Homeowners who generate their own energy are not subject to rising electricity prices, power outages, or the economic volatility associated with centralized utilities. This financial freedom allows off-the-grid homes to be more self-sustaining, reducing the economic burden that utility bills place on families. Additionally, energy independence provides peace of mind during periods of natural disasters or geopolitical instability that may disrupt centralized power supplies.
- Job Creation and Local Economy Growth: The growing demand for off-the-grid power solutions presents an opportunity for job creation, particularly in the renewable energy sector. The design, installation, and maintenance of small-scale power plants require skilled workers, including solar panel technicians, electricians, engineers, and energy consultants. In rural or remote areas, local businesses can benefit from the installation and upkeep of these systems, boosting the local economy. Training programs in renewable energy can help create skilled workers in the renewable energy field, leading to a workforce that is well-equipped for the jobs of tomorrow.
- Empowering Remote Communities: For communities located in remote or rural areas where grid infrastructure is lacking or unreliable, off-the-grid power solutions provide a pathway to greater autonomy and empowerment. These systems allow homes and villages to develop without being dependent on distant power stations or utility companies. Off-the-grid energy systems are often the only viable solution in these areas, and they can play a pivotal role in reducing energy poverty and improving overall living conditions.
- Affordable Access to Energy: In developing regions, particularly in areas without reliable access to electricity, small-scale power plants provide an affordable and sustainable alternative. Solar and wind technologies, in particular, can be scaled to meet the specific energy needs of off-the-grid households, and they require little to no ongoing fuel costs. In these areas, the initial investment in renewable energy systems may be higher, but over time, the savings on fuel and electricity costs make them a far more economical choice than relying on traditional power sources, such as diesel generators.
- Community Resilience and Disaster Recovery: Off-the-grid power systems can also enhance community resilience by ensuring a reliable energy supply during natural disasters. In remote or disaster-prone areas, centralized power grids are often the first to fail during storms, earthquakes, or floods. Off-the-grid homes, equipped with their own energy sources, are less likely to face blackouts, and they can act as critical energy hubs for surrounding communities in the aftermath of a disaster. Additionally, mobile solar or wind power systems can be deployed to areas in need of temporary energy supply, contributing to disaster recovery efforts.
15. Challenges and Solutions
While small-scale power plants for off-the-grid homes offer numerous benefits, there are challenges that need to be addressed to ensure their success. Some of the key challenges include:
- Upfront Costs: The initial investment for small-scale power systems can be significant, particularly for solar, wind, or micro-hydro installations. The cost of solar panels, batteries, and other equipment can be a barrier for many homeowners, especially in developing regions where financial resources may be limited. To address this, governments, NGOs, and private companies are increasingly offering subsidies, incentives, and financing options to make renewable energy systems more accessible. Over time, the falling costs of renewable energy technologies and the savings on energy bills can offset the upfront costs.
- Energy Storage and Reliability: One of the key challenges of off-the-grid systems is ensuring a consistent energy supply, particularly when energy demand exceeds generation capacity or when renewable resources are intermittent. Battery storage technology plays a critical role in managing this issue, but storage solutions can be expensive and have limited lifespans. Advances in battery technology, along with the use of hybrid systems and load management strategies, are helping to mitigate these issues and improve the reliability of off-the-grid power systems.
- Maintenance and Technical Expertise: Off-the-grid systems require regular maintenance to ensure they remain operational, and some technologies may require technical expertise for troubleshooting and repair. In remote areas, where access to technical support may be limited, this can pose a challenge. However, the growth of local training programs and the increasing availability of remote monitoring systems can help address these issues by enabling homeowners to maintain their systems effectively or seek support when needed.
- Environmental Impact of Materials: While renewable energy technologies are far less harmful to the environment than fossil fuels, the production of equipment such as solar panels and batteries still has an environmental impact. The mining of raw materials for these technologies, such as lithium for batteries or silicon for solar cells, can cause environmental degradation if not managed responsibly. However, efforts are underway to improve the sustainability of the entire life cycle of these technologies, from manufacturing to disposal. Recycling programs for solar panels and batteries are growing, helping to reduce the environmental footprint of renewable energy systems.
16. Conclusion
Small-scale power plants for off-the-grid homes are an essential part of the transition to a sustainable, decentralized energy future. These systems allow homeowners to generate clean, renewable energy and reduce their reliance on fossil fuels. With the right combination of technology, financial support, and energy management practices, off-the-grid homes can provide a model for sustainable living that benefits individuals, communities, and the planet.
As the cost of renewable energy technologies continues to fall and new innovations emerge, small-scale power plants will become more affordable, efficient, and accessible to homeowners around the world. By embracing these technologies, off-the-grid homes can help to build a more resilient, equitable, and environmentally friendly energy system for the future.
17. Technological Innovations Shaping Off-the-grid Power Solutions
As the demand for small-scale power plants for off-the-grid homes continues to rise, technological advancements are significantly enhancing the viability, efficiency, and affordability of these systems. Several innovations in renewable energy generation, storage, and management are poised to make off-the-grid living even more accessible and sustainable.
- Next-Generation Solar Panels: Solar technology is evolving rapidly, with next-generation solar panels offering higher efficiency and lower costs. New materials, such as perovskite solar cells, have the potential to drastically reduce the cost per watt of solar power while improving efficiency levels beyond current silicon-based panels. These advanced solar cells can capture more sunlight in a smaller area, making them ideal for off-the-grid homes where space for solar installations might be limited. Additionally, improvements in solar panel durability and lifespan will reduce the need for frequent replacements, further decreasing the long-term cost of solar power.
- Bifacial Solar Panels: Bifacial solar panels are an emerging technology that captures sunlight from both the front and back of the panel, significantly increasing energy production. By reflecting sunlight off surrounding surfaces, such as snow or rooftops, bifacial panels can capture more light and generate additional energy compared to traditional panels. These panels are particularly effective in areas with high reflectivity, making them well-suited for off-the-grid homes located in remote or rural areas where maximizing energy production is crucial.
- Wind Turbine Advancements: Small-scale wind turbines are becoming more efficient, quieter, and easier to install. Innovations in turbine blade design, aerodynamics, and materials have led to the development of compact wind turbines that can operate efficiently in low-wind conditions, making them more suitable for a broader range of geographic locations. In addition, vertical-axis wind turbines (VAWTs) are gaining popularity in residential applications due to their lower height, reduced noise, and ability to operate in turbulent wind conditions. VAWTs also have a more compact design, making them ideal for small off-the-grid homes with limited space.
- Micro-Hydro Power: Micro-hydro systems are also benefiting from technological advancements that make them more efficient and affordable. Modern turbines are designed to work in a wider range of water flow rates, and some can even operate in areas with lower water head, enabling homes to take advantage of smaller water sources. With efficient turbine designs and the ability to store excess power in batteries, micro-hydro systems offer a consistent and reliable energy supply for off-the-grid homes, especially in areas with access to flowing water.
- Smart Inverters and Energy Management Systems: Smart inverters and energy management systems are essential tools for optimizing the performance of small-scale power plants. Inverters convert the DC electricity generated by solar panels or wind turbines into usable AC electricity, and smart inverters allow homeowners to monitor, control, and optimize their energy use in real time. Energy management systems can automatically adjust the load distribution between different energy sources, manage battery storage, and prioritize energy use based on demand, ensuring the system runs efficiently without overloading. These systems can also be integrated with smartphone apps for remote monitoring, giving homeowners greater control over their energy consumption.
- Energy as a Service (EaaS): One of the newer business models emerging in the renewable energy sector is Energy as a Service (EaaS), where off-the-grid homeowners can access renewable energy systems through subscription or pay-per-use models rather than making a large upfront investment. In this model, companies provide, install, and maintain small-scale power plants for off-the-grid homes, including solar panels, wind turbines, and batteries. Homeowners pay for the energy generated by the system on a regular basis, similar to paying for utility service. EaaS can lower the financial barrier for homeowners and make off-the-grid energy solutions more accessible, especially for those in remote regions or developing countries.
- Battery Storage Innovations: Advances in battery technology are crucial to the success of off-the-grid power systems. Energy storage is essential for storing excess power generated during sunny or windy days to be used during low-generation periods. Lithium-ion batteries, which have become the standard for home energy storage, are continually improving in terms of energy density, cost, and lifespan. However, newer alternatives like solid-state batteries and flow batteries promise even greater performance. Solid-state batteries, in particular, offer higher energy density, faster charging times, and longer lifespans, making them an ideal solution for off-the-grid homes that require reliable energy storage. Flow batteries, which store energy in liquid form, offer a longer discharge cycle and the ability to scale easily for larger energy storage needs.
- Energy-Positive Buildings: The future of off-the-grid homes is also being shaped by the concept of energy-positive buildings—homes that generate more energy than they consume. Through a combination of highly efficient energy systems, renewable generation technologies, and advanced building designs (including passive solar features, super-efficient insulation, and smart heating/cooling systems), these buildings produce surplus energy that can be shared with the community or fed back into the grid. This trend could revolutionize off-the-grid living by turning energy consumers into energy producers, contributing to the broader adoption of sustainable energy solutions.
18. Policy and Regulatory Support for Off-the-grid Solutions
Governments and regulatory bodies play a crucial role in promoting the adoption of small-scale power plants for off-the-grid homes. Through a combination of policies, incentives, and regulations, governments can reduce the financial burden of renewable energy systems, ensure equitable access to clean energy, and foster an environment that supports innovation and development in the off-the-grid energy sector.
- Financial Incentives and Subsidies: Many governments offer financial incentives, such as tax credits, grants, and subsidies, to encourage the adoption of renewable energy systems. In countries like the United States, tax credits like the Investment Tax Credit (ITC) allow homeowners to deduct a portion of the cost of installing solar power systems from their federal taxes. Similarly, in Europe, feed-in tariffs and green certificates offer compensation for excess energy produced by small-scale power plants. By lowering the upfront costs of renewable energy technologies, financial incentives make off-the-grid living more financially feasible for homeowners.
- Net Metering and Energy Trading: In some regions, homeowners with small-scale renewable energy systems can participate in net metering programs, where they can sell excess power back to the grid or share energy with other households. These programs enable homeowners to offset the cost of their energy systems and create additional income streams. By participating in energy trading platforms, off-the-grid homeowners can engage in decentralized energy markets and sell energy to others in need, further promoting sustainability and energy independence.
- Building Codes and Standards: Building codes and regulations are essential for ensuring the safety and efficiency of off-the-grid power systems. Governments often provide guidelines for the installation of renewable energy technologies and require compliance with safety standards, such as those set by the National Electrical Code (NEC) in the United States. These regulations ensure that off-the-grid homes are equipped with the necessary infrastructure to prevent hazards like electrical fires, while also ensuring that systems are properly integrated and optimized for long-term use.
- Rural Electrification and Development Programs: In many developing countries, rural electrification programs are focused on providing reliable and affordable energy to remote communities. These programs often prioritize the installation of small-scale power plants, such as solar mini-grids, which can be expanded as demand grows. Governments and international organizations provide funding, technical support, and capacity-building efforts to ensure that off-the-grid solutions are successfully implemented in rural areas. These programs not only address the energy needs of remote populations but also contribute to economic development by fostering local industries, education, and health improvements.
- Environmental Regulations: To ensure the long-term sustainability of small-scale power plants, governments and regulatory bodies often implement environmental regulations that govern the production, disposal, and recycling of renewable energy technologies. These regulations can help minimize the environmental impact of the manufacturing process, as well as promote the recycling of materials used in solar panels, wind turbines, and batteries. By promoting the sustainable life cycle of renewable energy systems, these regulations help ensure that off-the-grid solutions contribute to a more circular economy and reduce overall environmental harm.
19. Conclusion: The Future of Off-the-grid Power Plants
Small-scale power plants for off-the-grid homes represent a transformative shift towards sustainable, decentralized energy solutions. With advancements in renewable energy technologies, battery storage, and energy management systems, off-the-grid homes are becoming more reliable, efficient, and cost-effective. The ongoing development of new innovations and policies will continue to shape the future of off-the-grid living, allowing more households to transition to energy independence while reducing their environmental impact.
As society embraces these technologies, off-the-grid power systems can play a key role in addressing energy inequality, reducing dependence on fossil fuels, and mitigating climate change. The future of energy is increasingly decentralized, and off-the-grid homes are at the forefront of this shift, providing a sustainable, self-sufficient, and resilient model for the generations to come.
20. The Role of Community-Based Off-the-grid Solutions
As off-the-grid power solutions continue to evolve, the concept of community-based renewable energy systems is gaining traction. These systems enable groups of off-the-grid homes or small villages to collectively generate, store, and manage their own energy resources. By leveraging economies of scale, community-based systems can enhance the reliability and affordability of renewable energy, while providing a model for collaborative sustainability.
- Community Solar and Wind Projects: One of the most common forms of community-based off-the-grid solutions is the shared solar or wind power system. These projects enable multiple households or small communities to pool resources and install larger renewable energy systems, such as solar farms or small-scale wind turbines. The energy generated by these systems is then distributed among participating homes, reducing costs and improving access to clean energy. The benefits of community solar and wind projects include reduced installation costs, better maintenance efficiency, and enhanced resilience to external energy supply disruptions.
- Microgrids: A microgrid is a small-scale energy system that operates independently or in coordination with a larger grid. Microgrids can include various renewable energy technologies, such as solar panels, wind turbines, and energy storage systems. They provide energy to a localized area, such as a village, neighborhood, or industrial park, and can function autonomously during grid outages. Microgrids have become increasingly popular for off-the-grid homes and small communities, as they allow for greater control over energy production, distribution, and consumption. In addition, microgrids can be integrated with energy storage systems to provide reliable power during periods of low renewable generation, ensuring that energy demands are met even in remote areas with limited access to centralized power.
- Local Energy Cooperatives: In some regions, local energy cooperatives are emerging as a solution for off-the-grid communities. These cooperatives are formed when a group of individuals or organizations come together to collectively own and operate renewable energy systems. By sharing the costs, risks, and rewards, energy cooperatives enable off-the-grid communities to achieve greater energy independence while benefiting from shared infrastructure and resources. These cooperatives can generate electricity from renewable sources and distribute it among members, often at a lower cost than traditional utilities. Additionally, cooperatives can reinvest any profits into further renewable energy projects or local community initiatives.
- Peer-to-Peer Energy Trading: Peer-to-peer (P2P) energy trading platforms are a new and innovative way for off-the-grid homeowners to exchange surplus energy with their neighbors or the wider community. These digital platforms allow individuals to sell or share excess renewable energy generated by their solar panels, wind turbines, or other systems. By facilitating energy trading within local communities, P2P platforms create new opportunities for income generation and help balance energy supply and demand. P2P trading encourages greater energy independence and can help stabilize the community’s energy system by allowing homes to access affordable, locally-produced power when they need it most.
- Collaborative Consumption Models: Collaborative consumption, sometimes referred to as the “sharing economy,” is an approach in which individuals or communities share access to resources. In the context of off-the-grid power solutions, this could include sharing renewable energy generation equipment, such as solar panels or wind turbines, or even sharing access to energy storage systems. By pooling resources, communities can reduce individual costs and increase the overall efficiency of their energy systems. For example, a group of off-the-grid homeowners could collectively invest in a battery storage system that can store excess energy generated by their solar panels during the day and distribute it among the group during nighttime or cloudy periods. This collaborative approach ensures that the community benefits from a shared renewable energy infrastructure, which can improve overall energy security and sustainability.
- Shared Knowledge and Capacity Building: As off-the-grid power systems grow in popularity, sharing knowledge and building local capacity is crucial for long-term success. In community-based renewable energy projects, participants can share their knowledge and expertise in areas such as installation, maintenance, energy management, and troubleshooting. This not only helps to create a sustainable, self-sufficient community but also empowers individuals by building skills that can be used for future renewable energy initiatives. Additionally, local workshops, training programs, and educational outreach can help demystify renewable energy technologies and provide the tools necessary for communities to take ownership of their energy systems.
21. The Global Expansion of Off-the-grid Power Solutions
The global expansion of off-the-grid power systems is fueled by a combination of factors, including increasing demand for clean energy, advancements in renewable energy technologies, and the growing recognition of the benefits of decentralized energy generation. Countries and regions around the world are adopting off-the-grid solutions as part of their broader energy transition strategies, seeking to improve energy access, reduce reliance on fossil fuels, and strengthen energy resilience.
- Developing Countries and Rural Electrification: Off-the-grid power systems have the potential to transform energy access in developing countries, particularly in rural areas that lack reliable grid infrastructure. In many regions of sub-Saharan Africa, South Asia, and Latin America, off-the-grid renewable energy solutions are being deployed to bring electricity to rural communities. Solar home systems, mini-grids, and wind power solutions are all playing a role in providing clean energy to millions of people who would otherwise remain without access to electricity. These systems not only improve living standards but also foster economic development by powering essential services, such as schools, hospitals, and agricultural enterprises.
- International Development and Aid: International organizations and development agencies are increasingly focusing on off-the-grid energy solutions as part of their efforts to address energy poverty and promote sustainable development. Initiatives such as the United Nations’ Sustainable Development Goal (SDG) 7, which aims to ensure universal access to affordable, reliable, sustainable, and modern energy, are driving investments in renewable energy systems for off-the-grid communities. Donor-funded projects often provide the necessary financial support, technical expertise, and training for implementing renewable energy solutions in underserved areas. By partnering with local governments, NGOs, and businesses, international aid efforts are helping to expand the reach of off-the-grid power systems and improve energy access in remote regions.
- Technological Leapfrogging: One of the most exciting aspects of off-the-grid power solutions is the potential for technological leapfrogging. In many developing countries, off-the-grid communities are skipping over the traditional, centralized energy infrastructure in favor of decentralized renewable energy technologies. This leapfrogging allows countries to bypass the environmental and financial costs of fossil fuel-based energy generation and directly adopt clean, sustainable energy solutions. By deploying small-scale solar, wind, and other renewable energy systems, these countries can achieve energy independence while reducing their carbon emissions.
- Private Sector Involvement: The private sector is also playing a key role in the global expansion of off-the-grid power solutions. Renewable energy companies, technology providers, and financing institutions are increasingly targeting off-the-grid markets, recognizing the potential for growth in these regions. Companies are offering innovative products, such as portable solar generators, off-grid battery storage systems, and mini-grid solutions, to meet the needs of remote communities. Additionally, the private sector is exploring new financing models, such as pay-as-you-go systems and mobile money solutions, to make renewable energy systems more affordable and accessible to off-the-grid homeowners.
- Smart Grid Integration: While off-the-grid systems are designed to function independently, they can also be integrated into larger regional or national grids to support a broader energy transition. Smart grids, which incorporate digital technology to manage energy distribution, can enable off-the-grid homes to exchange energy with the larger grid when available or necessary. This integration allows for a more flexible and resilient energy system, where off-the-grid homes contribute to the grid’s stability and are able to access backup power when required. In regions with intermittent renewable energy production, such as wind or solar, off-the-grid systems can provide valuable support by helping balance supply and demand.
22. The Path Forward: Building a Sustainable Off-the-grid Future
The future of small-scale power plants for off-the-grid homes is bright, with continued advancements in technology, policy, and business models paving the way for widespread adoption. In order to build a sustainable off-the-grid future, several steps need to be taken:
- Increased Investment: Governments, international organizations, and the private sector must continue to invest in renewable energy technologies, energy storage solutions, and infrastructure that supports off-the-grid living. Increased investment will accelerate the development of new technologies, reduce costs, and ensure that off-the-grid power systems are accessible to communities worldwide.
- Collaboration and Partnerships: Collaboration between governments, NGOs, the private sector, and local communities will be essential to the successful implementation of off-the-grid solutions. By working together, stakeholders can pool resources, share expertise, and create innovative solutions that meet the energy needs of off-the-grid homes and communities. Partnerships with local organizations can also ensure that off-the-grid projects are culturally appropriate and responsive to the unique needs of each community.
- Policy Support: Governments must continue to provide policies, regulations, and incentives that support the adoption of renewable energy systems for off-the-grid homes. This includes offering financial incentives, facilitating access to affordable energy storage, and creating favorable regulatory environments that enable innovation and growth in the off-the-grid sector.
- Public Awareness and Education: Raising public awareness about the benefits of off-the-grid power systems and providing education on renewable energy technologies is key to driving adoption. By informing individuals and communities about the advantages of renewable energy, energy efficiency, and energy independence, more people will be empowered to make informed decisions about their energy consumption and embrace sustainable alternatives.
In conclusion, small-scale power plants for off-the-grid homes represent a crucial part of the global transition to sustainable, decentralized energy solutions. With continued technological innovation, supportive policies, and global collaboration, off-the-grid power systems will play a vital role in meeting the energy needs of the future, creating resilient, self-sufficient communities, and reducing the environmental impact of traditional energy systems. The future of energy is off the grid, and it is bright, sustainable, and full of potential.
Small Scale Power Plant for Cottage Resorts
Cottage resorts, especially those located in remote or rural areas, often face unique challenges when it comes to energy supply. These locations typically lack access to the central grid, and their energy needs are highly variable, often depending on peak seasons. Establishing a small-scale power plant for cottage resorts provides a solution for energy independence, reducing the reliance on diesel generators or costly grid connections. Moreover, incorporating renewable energy sources into such plants offers an eco-friendly alternative, appealing to guests who prioritize sustainability.
1. Energy Demand in Cottage Resorts
Cottage resorts have varying energy demands, which can fluctuate based on occupancy, weather conditions, and operational activities. Energy requirements for these resorts typically include lighting, heating, cooling, hot water, refrigeration, and cooking, all of which are essential for providing guests with a comfortable stay. In addition, leisure facilities such as pools, spas, and entertainment areas require considerable power, especially during peak tourist seasons.
However, the seasonal nature of most cottage resorts means that energy consumption peaks during specific times of the year, such as summer or winter holidays, and is significantly lower during off-seasons. A well-designed small-scale power plant for cottage resorts must be capable of handling this variability and providing a consistent power supply year-round, even during low-demand periods.
2. Renewable Energy Solutions for Off-the-grid Resorts
Cottage resorts are prime candidates for small-scale renewable energy solutions due to their relatively low energy demands and the opportunity to harness local natural resources. Various renewable energy technologies can be combined to create an integrated power system for these resorts. The most common renewable energy options include:
- Solar Power: Solar energy is a highly effective solution for cottage resorts located in sunny regions. Solar panels can be installed on rooftops or in open areas to capture sunlight and convert it into electricity. Solar energy can be particularly useful for powering lighting, water heating, and other essential services. During sunny periods, excess energy can be stored in batteries to ensure continuous supply during cloudy days or nighttime.
- Wind Power: If the resort is situated in a windy area, wind turbines can be a valuable addition to the energy mix. Small-scale wind turbines can provide a reliable source of energy to supplement solar power, especially in remote or isolated locations where other energy options are less viable. Wind power can be integrated with battery storage systems, allowing resorts to harness energy during high-wind periods for use when the wind dies down.
- Hydropower (Micro-Hydro Systems): If the resort is located near a flowing water source, a micro-hydro system can be an excellent choice for generating power. A micro-hydro plant harnesses the kinetic energy of flowing water to turn a turbine, which generates electricity. Hydropower is highly reliable, providing a steady source of energy throughout the year, as long as there is sufficient water flow. For resorts located near rivers, streams, or waterfalls, micro-hydro systems can deliver a consistent energy supply, reducing reliance on other renewable sources.
- Biomass: For resorts located in areas with abundant organic waste, biomass energy can be an effective way to generate power. Biomass systems can convert wood, agricultural waste, or other organic materials into heat or electricity. This solution is particularly suitable for resorts surrounded by forests or agricultural lands. Biomass systems also have the benefit of being highly efficient and capable of operating continuously, providing a steady source of energy for heating, hot water, and cooking.
- Geothermal: In areas with significant geothermal activity, geothermal energy can be harnessed for both heating and electricity generation. Geothermal heat pumps can be used to provide space heating and hot water, while geothermal power plants can generate electricity. This energy source is particularly valuable in regions with consistent geothermal activity, providing year-round access to renewable energy.
3. Hybrid Power Systems
For small-scale power plants serving cottage resorts, hybrid energy systems that combine multiple renewable energy sources often provide the most reliable and cost-effective solution. A hybrid system may combine solar, wind, and storage technologies, or a combination of solar and micro-hydro power, to meet the resort’s energy needs. These systems are designed to optimize energy generation based on local resource availability, ensuring a steady supply of power even when one energy source is less productive.
- Solar-Wind Hybrid Systems: A solar-wind hybrid system takes advantage of the complementary nature of solar and wind energy. Solar power tends to be most effective during sunny days, while wind power is more reliable during the night or in windy conditions. By combining the two energy sources, a resort can benefit from a more consistent energy supply throughout the day and night.
- Solar-Hydro Hybrid Systems: Combining solar and micro-hydro systems can be particularly effective for resorts located near water sources. Solar panels can provide power during the day when sunlight is abundant, while the micro-hydro system can generate energy continuously, ensuring a consistent power supply. In cases of low water flow or seasonal variations in sunlight, the hybrid system will balance energy generation and consumption.
- Energy Storage Solutions: Batteries play a crucial role in hybrid power systems, storing excess energy during periods of high generation for use when the renewable sources are not producing enough power. High-capacity batteries, such as lithium-ion or flow batteries, can store energy efficiently and release it when demand peaks, ensuring a constant energy supply for the resort. Energy storage also helps stabilize the grid in off-the-grid situations, preventing disruptions during peak loads or inclement weather.
4. Benefits of a Small-Scale Power Plant for Cottage Resorts
- Energy Independence: A small-scale power plant reduces the resort’s dependence on external energy sources, such as diesel generators or the electrical grid. This is especially important for resorts in remote locations, where grid access may be unreliable or unavailable.
- Cost Savings: While the initial investment in renewable energy systems may be significant, the long-term operational costs are usually lower compared to diesel fuel or grid electricity, especially in areas where energy prices are high. Over time, the resort can save money on energy bills, allowing for a faster return on investment.
- Sustainability and Eco-friendliness: Many guests choose cottage resorts for a relaxing and nature-filled experience. By using renewable energy sources, the resort enhances its eco-friendly appeal, which can attract environmentally-conscious travelers. Reducing greenhouse gas emissions and the carbon footprint of resort operations aligns with global sustainability goals and can contribute to a greener future.
- Resilience: Small-scale renewable energy systems, when properly designed and integrated, can make cottage resorts more resilient to power outages or fuel shortages. With energy independence, the resort is better equipped to handle natural disasters, energy supply disruptions, or rising fuel costs.
5. Considerations for Implementing Small-Scale Power Plants
- Site Assessment: The first step in establishing a small-scale power plant is to assess the local energy resources. Factors such as sunlight hours, wind patterns, water flow, and the availability of biomass must be carefully evaluated to determine the most suitable combination of energy technologies for the location.
- Energy Demand Forecasting: Accurate forecasting of energy demand is essential for sizing the system appropriately. Overestimating the demand could lead to over-investment in infrastructure, while underestimating it could result in insufficient power during peak times. Understanding the resort’s usage patterns and designing a system that can handle seasonal variations in demand is critical.
- Maintenance and Monitoring: Small-scale power plants require regular maintenance to ensure optimal performance. For solar panels, this includes cleaning and inspecting for damage, while wind turbines may require occasional maintenance to keep the blades and components in working condition. Battery storage systems also need monitoring to ensure their capacity and performance remain reliable. Resort management must plan for the costs and logistics of maintenance and repairs, either through on-site staff or remote monitoring services.
- Integration with Resort Operations: The power plant must be integrated into the resort’s overall energy management strategy. This includes coordinating the use of renewable energy with heating, cooling, and lighting systems to optimize consumption and minimize waste. A smart grid or energy management system (EMS) can be implemented to monitor and control energy use, balancing supply and demand in real-time.
6. Future Trends and Opportunities
- Smart Resort Integration: The trend of smart homes is extending to the hospitality industry, with an increasing number of cottage resorts incorporating smart technology into their operations. By integrating renewable energy systems with smart technologies, resorts can optimize energy consumption, manage guest experiences, and automate routine tasks such as lighting, heating, and cooling. Smart energy systems can also help resorts monitor and control their energy use remotely, ensuring efficiency and reducing waste.
- Energy Sharing Models: In the future, resort owners could explore energy-sharing models with nearby communities or other resorts. By participating in microgrid systems or energy exchange networks, resorts can sell excess energy to other properties or share resources during times of peak demand, further increasing the financial sustainability of their renewable energy systems.
- Eco-Tourism Incentives: As eco-tourism continues to grow, more governments and private organizations are providing incentives for businesses, including cottage resorts, to adopt renewable energy technologies. These incentives, such as tax breaks or grants, can reduce the financial burden of installing small-scale power plants, making them more accessible to resort owners.
7. Conclusion
Small-scale power plants for cottage resorts offer a sustainable and reliable solution to the energy challenges faced by these remote, seasonal operations. By integrating renewable energy sources such as solar, wind, hydro, and biomass, cottage resorts can reduce their environmental impact, lower operating costs, and enhance their energy independence. Hybrid systems and energy storage solutions ensure that energy is available year-round, regardless of fluctuations in demand or resource availability. With the ongoing advancement of energy technologies, small-scale power plants for cottage resorts will continue to improve, providing eco-friendly and resilient energy solutions for the tourism industry.
A small-scale power plant for cottage resorts is more than just a utility—it’s a crucial component of sustainable operations. Given the increasing demand for environmentally conscious travel and the growing concern about energy consumption in remote areas, implementing such systems can be transformative for the hospitality industry. Beyond the initial energy generation benefits, these systems can have a broader positive impact on both the environment and local communities.
8. Long-Term Sustainability and Impact
For cottage resorts, the long-term benefits of adopting renewable energy systems extend beyond mere cost savings. The environmental advantages of reducing fossil fuel consumption, lowering greenhouse gas emissions, and minimizing reliance on non-renewable resources are significant. As public awareness of climate change and sustainability continues to grow, eco-conscious tourists are becoming more inclined to support businesses that align with their values.
Environmental Impact:
- Reduced Carbon Footprint: By transitioning to renewable energy sources such as solar, wind, hydro, and biomass, the resort significantly reduces its carbon footprint. The reliance on diesel generators and grid power, often fueled by fossil fuels, is one of the primary sources of carbon emissions in remote resorts. By substituting these energy sources with cleaner alternatives, the resort contributes to global climate action and helps preserve the surrounding natural beauty.
- Conservation of Natural Resources: Renewable energy systems harness the natural resources available in the resort’s environment, whether it’s sunlight, wind, or flowing water. By utilizing these locally available sources, resorts not only minimize their reliance on external energy supply chains but also help conserve finite natural resources. For instance, reducing the consumption of diesel fuel means fewer shipments and infrastructure required to transport fuel, thus lowering the ecological impact of transportation.
- Waste Reduction: Renewable systems like biomass also provide a solution to organic waste, turning it into useful energy instead of sending it to landfills. This aspect supports circular economy principles and aligns with the resort’s sustainability goals, further enhancing its eco-friendly reputation.
9. Engaging Guests with Sustainability
Modern travelers increasingly value sustainability, and they appreciate when businesses, including resorts, embrace green initiatives. Guests staying at a cottage resort powered by renewable energy are likely to feel more connected to nature, knowing they are part of an eco-friendly operation. Moreover, educating guests about the energy systems in place—whether through informational brochures, guided tours of the energy plant, or signage highlighting sustainability efforts—can improve the guest experience while fostering a deeper appreciation for the environment.
- Guest Awareness: Resorts can use their renewable energy systems as a marketing tool. Highlighting their commitment to clean energy can differentiate the resort in a competitive market. For example, providing guests with the option to take part in sustainability tours or showing them how the power plant works can elevate the guest experience.
- Sustainable Amenities: Resorts can further enhance their eco-friendly image by offering amenities that promote sustainable living. For example, offering electric vehicle (EV) charging stations powered by renewable energy can attract eco-conscious guests with electric vehicles. Additionally, offering locally sourced, organic food, reducing single-use plastics, and providing water conservation initiatives can all complement the resort’s green energy efforts.
- Eco-Tourism Certifications: Many international and local tourism organizations offer eco-certifications or awards to businesses that adopt sustainable practices. By implementing a small-scale renewable energy plant, a cottage resort may qualify for these prestigious certifications, further enhancing its marketability to eco-conscious travelers.
10. Economic Opportunities for Local Communities
Another key benefit of adopting small-scale renewable energy solutions is the positive impact on local communities. Often, cottage resorts are located in remote areas where access to energy infrastructure is either limited or costly. By establishing a small-scale power plant, these resorts can not only meet their own energy needs but also provide energy solutions to local communities, creating economic opportunities and fostering collaboration.
- Job Creation: The installation and ongoing maintenance of renewable energy systems can create local jobs. From the construction phase, where local labor can be employed for installation, to ongoing maintenance and operational roles, renewable energy systems contribute to local employment. Additionally, the resort may choose to hire local workers to manage and operate the power plant, fostering a sustainable local workforce.
- Energy Sharing and Community Benefits: In certain cases, cottage resorts with small-scale power plants may have the capacity to share surplus energy with neighboring villages or communities. This can help stabilize the local energy supply and reduce the reliance on expensive or unreliable energy sources. In regions where energy is scarce, this energy-sharing model can be a valuable way to support local infrastructure while reducing the resort’s operational costs.
- Investment in Local Infrastructure: The establishment of a renewable energy system can also spur investment in local infrastructure. In some cases, building a renewable power plant may necessitate improvements to roads, water supplies, or communication networks. As resorts expand their energy independence, their economic impact on surrounding areas grows, creating a beneficial relationship between the resort and the local community.
11. Challenges and Considerations
While the benefits of small-scale renewable power plants for cottage resorts are clear, several challenges must be addressed to ensure successful implementation. These include:
- Initial Capital Investment: The upfront costs for installing renewable energy systems, particularly for technologies like solar or micro-hydro, can be high. However, the long-term savings in energy bills and the potential for increased guest satisfaction through a green energy initiative often justify these costs. Financing options, such as grants, loans, or partnerships with energy developers, can help resorts manage the initial investment.
- Technical Expertise: The design, installation, and maintenance of small-scale power plants require specialized technical expertise. Resorts may need to partner with renewable energy providers or consultants to ensure the systems are correctly designed and integrated into the resort’s energy needs. Long-term maintenance of the system is also crucial for ensuring reliability and minimizing downtime, which may require specialized staff or external contractors.
- Energy Storage: Even with renewable energy systems, some resorts may experience fluctuating power supply, especially in areas with seasonal changes or inconsistent renewable resources. The integration of energy storage systems, such as lithium-ion batteries or other advanced storage solutions, is essential to ensure a stable power supply when production is low. Ensuring the storage capacity is sufficient for the resort’s needs is critical, particularly during off-peak seasons when solar or wind generation may be minimal.
- Regulatory Compliance: Before setting up a small-scale power plant, resorts must navigate local regulations regarding energy production, grid connections, and environmental impact assessments. In some regions, permits or licenses may be required to install renewable energy systems. Resorts must work with local authorities to ensure all legal and environmental guidelines are met.
12. Conclusion
Small-scale power plants for cottage resorts provide a highly effective and sustainable solution to energy challenges in remote or off-the-grid locations. By leveraging renewable energy sources like solar, wind, hydro, and biomass, resorts can create a resilient, cost-effective, and environmentally friendly energy system that supports both their operational needs and sustainability goals. Beyond the operational benefits, these systems also contribute to the resort’s appeal to eco-conscious travelers, foster positive relationships with local communities, and contribute to global efforts to combat climate change. With careful planning, implementation, and ongoing maintenance, small-scale power plants can be a game-changer for cottage resorts looking to reduce costs, enhance guest experiences, and operate sustainably.
13. Advanced Technologies for Power Generation
In addition to the foundational renewable energy systems, advancements in energy generation and storage technologies are opening up even more possibilities for cottage resorts looking to enhance their energy independence. Incorporating these cutting-edge technologies can improve the efficiency, reliability, and flexibility of small-scale power plants.
- Microgrids: A microgrid is a localized power system that can operate independently or in conjunction with the main power grid. Microgrids are ideal for remote cottage resorts because they can integrate multiple energy sources (solar, wind, biomass, etc.) and optimize energy consumption. During periods when renewable energy generation is insufficient, a microgrid can connect to a larger grid (if available) or rely on backup systems like diesel generators. This flexibility ensures continuous power, even in isolated areas. Furthermore, microgrids can incorporate energy storage systems to manage fluctuations in renewable energy generation.
- Energy Management Systems (EMS): Advanced EMS can optimize energy generation, storage, and consumption across the entire resort. These systems monitor and control various energy sources in real-time, ensuring the most efficient use of power. For example, EMS can prioritize solar energy when available, store excess energy in batteries, and switch to wind or biomass power during low solar generation periods. By using EMS, resorts can reduce waste, ensure a steady energy supply, and lower operating costs. Additionally, EMS can provide valuable data for further optimizing energy use and identifying areas for efficiency improvements.
- Advanced Battery Storage Solutions: Traditional battery systems used in small-scale power plants are typically lithium-ion or lead-acid batteries. However, new advancements in battery storage technology are improving efficiency and lifespan. Flow batteries, for example, offer long-term storage capabilities with minimal degradation, making them ideal for large-scale energy storage needs. Another promising technology is solid-state batteries, which provide higher energy densities and faster charging times, making them ideal for remote locations where energy demand is highly variable.
- Combined Heat and Power (CHP) Systems: In resorts with access to biomass or other thermal energy sources, CHP systems can provide both electricity and thermal energy (heat or hot water). These systems are highly efficient because they capture the waste heat from electricity generation and repurpose it for heating or other uses. For example, excess heat from a biomass plant can be used for space heating in cottages or for heating water for guests, reducing the need for separate heating systems.
14. Long-Term Investment and Return on Investment (ROI)
Investing in a small-scale power plant for a cottage resort requires significant upfront costs, but the return on investment (ROI) can be substantial over time. Several factors influence ROI, including energy savings, government incentives, and guest interest in sustainable practices.
- Energy Savings: As resorts reduce their dependence on costly diesel generators or expensive grid electricity, they can realize significant savings over the long term. In regions where fuel prices are high or the electrical grid is unreliable, this can lead to a rapid ROI. Additionally, if the resort generates more power than needed, they could potentially sell excess energy back to the grid or share it with nearby communities, further increasing their ROI.
- Incentives and Subsidies: Many governments and private organizations offer financial incentives for businesses that invest in renewable energy systems. These incentives can significantly lower the initial investment costs, making renewable energy solutions more affordable for cottage resorts. Tax credits, grants, and rebates are common in many regions, as they help offset installation costs and speed up the payback period.
- Branding and Marketability: Eco-friendly practices can also enhance the resort’s brand image, attracting more guests who prioritize sustainability. As a result, resorts that adopt renewable energy solutions can often charge a premium for their services, especially if they market their energy systems as part of their eco-tourism offerings. For example, the appeal of staying in a cottage resort powered entirely by renewable energy is likely to attract guests who are willing to pay more for a sustainable experience.
- Operational Efficiencies: Over time, renewable energy systems become more efficient, especially as the technology improves. For example, solar panels, which have become increasingly efficient and affordable, can generate more power at a lower cost. Wind turbines are also becoming quieter and more efficient, reducing maintenance needs and operating costs. As these technologies continue to evolve, the operational costs of the power plant will decrease, further improving ROI.
15. Future-Proofing the Resort’s Energy Needs
One of the most important aspects of a small-scale power plant for a cottage resort is ensuring that the energy system remains future-proof. The global transition toward renewable energy is accelerating, and staying ahead of these trends can offer long-term benefits for resort operations.
- Scalability: A well-designed small-scale power plant should be scalable, meaning it can be easily expanded or modified to accommodate future energy needs. As resorts grow and expand, they may need to increase their energy generation capacity. A scalable system can accommodate additional solar panels, wind turbines, or storage batteries without requiring a complete overhaul of the system. This ensures that the power plant can continue to meet the energy demands of the resort as it evolves over time.
- Adaptation to Technological Advances: Energy technology is rapidly evolving, and small-scale power plants need to stay adaptable. For instance, integrating emerging technologies like artificial intelligence (AI) for predictive energy management can help optimize energy generation and storage. AI algorithms can forecast energy production based on weather patterns, adjust settings for maximum efficiency, and provide maintenance alerts before issues arise. Keeping the power plant infrastructure flexible allows for seamless integration of future advancements in renewable energy technology.
- Long-Term Contracts and Partnerships: As the renewable energy market matures, opportunities for long-term partnerships may arise. Resorts could explore power purchase agreements (PPAs) or joint ventures with energy companies that specialize in renewable energy. These agreements may offer favorable pricing on energy generation equipment, maintenance services, and energy storage solutions, providing further stability and cost predictability for the resort’s power needs.
- Sustainability Certifications and Future Branding: Over time, eco-certifications and green branding can become even more valuable. As global demand for sustainable tourism increases, resorts with eco-friendly credentials will have a competitive edge. By staying ahead of sustainability trends, cottage resorts can position themselves as leaders in the green tourism market, appealing to guests who are willing to pay a premium for responsible travel experiences.
16. Conclusion: A Green Future for Cottage Resorts
Small-scale power plants for cottage resorts represent a transformative solution for energy needs in remote or off-the-grid locations. By adopting renewable energy technologies such as solar, wind, hydro, biomass, and hybrid systems, resorts can achieve energy independence, reduce operational costs, and offer an eco-friendly experience that resonates with modern travelers.
The integration of advanced energy management systems, energy storage, and scalable solutions ensures that these power plants remain reliable, efficient, and future-proof. Moreover, the long-term economic benefits, including reduced energy costs, government incentives, and enhanced branding opportunities, make small-scale power plants a wise investment for the sustainable growth of cottage resorts.
As renewable energy technologies continue to improve and the demand for eco-friendly tourism grows, cottage resorts that invest in small-scale power plants today will be well-positioned to meet the challenges of tomorrow’s energy landscape while offering guests a unique and sustainable stay. With careful planning, the right technologies, and a commitment to sustainability, small-scale power plants can power the future of eco-conscious hospitality.
17. Operational and Maintenance Considerations
While implementing a small-scale power plant can provide significant benefits for cottage resorts, it’s essential to consider the operational and maintenance aspects to ensure long-term success and cost-effectiveness. A well-maintained power system will ensure reliability, efficiency, and minimal downtime, all of which are crucial for the resort’s operations.
- Routine Maintenance: Regular maintenance is vital for keeping renewable energy systems in optimal condition. Solar panels typically require periodic cleaning to ensure maximum efficiency, as dirt and debris can obstruct sunlight. Wind turbines and other mechanical systems need periodic inspections to check for wear and tear, including lubricating moving parts and replacing any components that have reached the end of their lifespan. Biomass plants also need routine maintenance for fuel handling systems, combustion areas, and emissions controls. While these maintenance needs are often minimal, regular servicing helps avoid unexpected breakdowns and ensures systems remain cost-effective.
- Monitoring Systems: Many small-scale power plants come with built-in monitoring systems that track the performance of the energy system in real-time. These systems can identify potential issues, track energy production levels, and detect inefficiencies before they become significant problems. By using advanced monitoring technologies, resorts can proactively address issues, reducing the risk of costly repairs and ensuring that the power plant operates at peak performance.
- Local Expertise and Partnerships: In remote areas, it’s important for resorts to either develop or partner with local technical expertise to handle power plant maintenance. Training local staff to monitor and maintain systems can not only ensure that there are no delays in addressing issues but can also reduce the resort’s dependency on external service providers. Resort management should establish strong partnerships with local technicians or service companies that specialize in renewable energy equipment, ensuring the power plant remains in good working condition over the long term.
- Spare Parts and Backup Systems: For critical systems like power generation plants, it’s important to have access to necessary spare parts and backup systems. Remote resorts, especially those with unique or custom renewable energy solutions, need to ensure that replacement parts are easily accessible. Creating a stock of essential parts or having agreements with suppliers that guarantee rapid delivery can prevent long downtimes caused by supply chain delays. Additionally, backup systems such as diesel generators or battery storage can provide a failsafe during system maintenance or unexpected downtime.
18. Scaling the Renewable Energy Solution
As cottage resorts grow and expand, there may be a need to scale up their power systems. Scaling renewable energy solutions requires careful planning to ensure the system can handle the increased demand while maintaining efficiency and reliability.
- Modular Systems: Many renewable energy technologies are inherently scalable. Solar panel arrays, for example, can be expanded by adding additional panels as the resort’s energy needs grow. Wind turbines can also be added incrementally. Modular systems allow the resort to start small and gradually expand as demand increases, without the need to replace the entire system. This flexibility is particularly valuable for growing resorts with fluctuating seasonal demand.
- Hybrid Power Systems: In cases where solar, wind, or biomass systems alone might not provide sufficient energy, hybrid systems can be used to integrate multiple energy sources. Hybrid power systems combine technologies like solar with wind or solar with biomass to provide a more balanced and reliable energy supply. By using a mix of renewable energy sources, resorts can ensure stable power generation regardless of seasonal variations in weather or available resources. This kind of system can also increase energy production in areas where one source may be more intermittent than the other.
- Energy Storage Expansion: As energy needs grow, the amount of energy that needs to be stored will also increase. Expanding the energy storage system can help ensure that energy is available when it is needed, even if generation from renewable sources is low. New technologies in storage systems, such as flow batteries or advanced lithium-ion batteries, are becoming more cost-effective and are essential for accommodating growing energy needs without sacrificing the resort’s energy security.
- Integrating New Technologies: As new technologies in renewable energy become available, resorts can integrate them into their existing systems. Innovations like floating solar panels, enhanced turbine designs, or even ocean-based tidal energy systems could potentially offer new ways to generate power for resorts located in coastal areas. Staying ahead of technological trends and adapting systems as necessary ensures that resorts continue to benefit from the most advanced and efficient energy solutions.
19. Resilience to Climate Change
Given the increasing frequency of extreme weather events and climate variability, it’s critical for small-scale power plants to be resilient to changing environmental conditions. Resort managers should consider how their energy systems will perform under different weather conditions, such as storms, floods, heatwaves, and more.
- Weather-Resilient Infrastructure: Renewable energy infrastructure should be designed to withstand harsh weather conditions. For example, solar panels should be installed with durable materials and mounting systems that can withstand high winds and heavy rains. Wind turbines should be robust enough to operate in regions with high winds or extreme storms. Biomass and hydro systems also need to be designed to handle the potential impacts of flooding, droughts, or fire risks, depending on the location of the resort.
- Energy Security: Climate change-induced weather disruptions can affect the availability and reliability of energy generation, particularly from sources like solar or wind. This highlights the importance of having a diverse mix of energy sources and storage options. By ensuring that energy generation is not overly reliant on one source, resorts can safeguard against power shortages in the event of unfavorable weather conditions. Additionally, energy storage provides a buffer, allowing the resort to continue operating even during periods of reduced energy production.
- Adaptability to Future Conditions: With the unpredictability of climate change, resort management should invest in technologies and designs that can easily adapt to future changes in environmental conditions. This might involve upgrading systems as needed or adding more flexible energy solutions, like hybrid systems or cloud-based energy management, that allow for remote adjustments. A forward-thinking approach can ensure that the resort remains energy-independent and operational, regardless of the challenges posed by climate change.
20. Collaboration with Local Governments and Organizations
Collaboration with local governments and environmental organizations can play an important role in the successful deployment and operation of small-scale power plants. Governments may provide policy support, financial incentives, and regulatory guidance that can ease the implementation process. Additionally, local organizations that focus on environmental sustainability or energy innovation may be valuable partners in promoting the resort’s eco-friendly initiatives.
- Policy Support and Incentives: Many governments offer financial incentives or grants to support the transition to renewable energy, including tax credits, subsidies, or low-interest loans. By collaborating with local and national governments, resorts can take advantage of these incentives to reduce the capital required for installing renewable energy systems. Additionally, working with local authorities can ensure that all regulatory requirements are met, such as zoning laws, environmental assessments, and permits.
- Environmental Partnerships: Collaborating with local environmental organizations or community groups can increase the resort’s credibility and expand its sustainability network. These partnerships can help improve the resort’s environmental footprint by providing guidance on best practices, waste reduction strategies, and conservation initiatives. Partnering with organizations that focus on eco-tourism or sustainability can also open up opportunities for joint marketing campaigns or promotional activities that emphasize the resort’s commitment to responsible tourism.
- Community Engagement: Beyond regulatory and environmental support, involving the local community in the power plant project can strengthen the resort’s relationship with its neighbors. Engaging the community in the planning and operation of the power plant, such as offering educational tours or involving them in the development process, can increase local buy-in and support. Communities that see the direct benefits of renewable energy projects, such as job creation and improved energy access, are more likely to embrace and support the resort’s efforts.
21. Conclusion: Empowering Cottage Resorts for a Sustainable Future
Incorporating a small-scale power plant into a cottage resort provides a powerful solution to energy needs in remote or off-the-grid areas. With careful planning, the use of renewable energy systems, and ongoing maintenance, these power plants offer a reliable, sustainable, and cost-effective means of power generation. By leveraging advanced technologies like microgrids, energy management systems, and energy storage, resorts can enhance energy efficiency, improve operational reliability, and create a more resilient energy system.
As environmental concerns continue to drive consumer behavior, resorts that adopt renewable energy solutions can gain a competitive edge by attracting eco-conscious travelers and positioning themselves as leaders in sustainable tourism. With long-term economic, environmental, and social benefits, small-scale power plants for cottage resorts offer a clear path to achieving energy independence, reducing costs, and contributing to a more sustainable future.
Small-scale power plants for non-grid connected communities offer a transformative approach to meeting the energy needs of remote or isolated areas. These communities often face significant challenges, including unreliable or absent access to the central power grid, high energy costs, and environmental degradation from reliance on non-renewable energy sources like diesel generators. A small-scale power plant, particularly one based on renewable energy technologies, can provide a reliable, sustainable, and cost-effective energy solution.
The most commonly used renewable energy systems for non-grid connected communities are solar, wind, hydro, and biomass power. Each of these energy sources has its unique advantages, and selecting the right combination of technologies is crucial for optimizing energy production and meeting the specific needs of the community. Hybrid systems that combine two or more renewable sources are often deployed to ensure a more consistent energy supply, balancing fluctuations in generation due to weather patterns or seasonal variations.
Solar power is one of the most widely adopted options for small-scale power plants, particularly in sunny regions. Photovoltaic (PV) panels are installed to capture sunlight and convert it into electricity. The energy generated can be used immediately or stored in batteries for later use. Battery storage systems are essential for ensuring a reliable power supply, particularly in regions where sunlight is intermittent or during the nighttime. Advances in solar technology have led to more efficient panels that generate more power at lower costs, making solar energy an increasingly affordable solution for remote communities.
Wind energy is another important source of renewable power for non-grid connected communities. Wind turbines, particularly small-scale models, can generate electricity even in areas with moderate wind speeds. Wind is a highly reliable energy source in many regions, and in combination with solar power, it can provide a complementary solution for communities that may experience variable weather patterns. Wind energy systems typically require minimal maintenance, and when installed in the right locations, they can generate power consistently.
Hydropower, or small-scale hydroelectric systems, is an ideal solution for communities located near rivers, streams, or other water bodies with consistent water flow. These systems use the kinetic energy of flowing water to turn turbines and generate electricity. Small-scale hydroelectric plants can provide a steady, reliable energy supply with little environmental impact, making them an excellent choice for communities with access to water resources. However, the installation of such systems requires careful planning to avoid disrupting local ecosystems and ensure that the power plant operates efficiently.
Biomass power generation, using organic materials like wood, agricultural waste, or even dedicated energy crops, is another viable option for non-grid connected communities. Biomass plants can convert waste products into electricity, providing both a renewable energy source and a solution for managing local waste. This system is particularly valuable in agricultural or forested regions, where biomass materials are abundant. It also has the added benefit of reducing reliance on fossil fuels, contributing to lower carbon emissions.
One of the key advantages of small-scale power plants is their ability to operate independently of the main power grid. This feature is particularly valuable in areas where the grid is either unavailable or unreliable, and traditional energy infrastructure is expensive or impractical. For non-grid connected communities, this independence not only ensures a continuous energy supply but also reduces dependency on fuel imports, which can be costly and subject to price fluctuations.
In addition to energy generation, microgrids play a significant role in optimizing power distribution in off-grid communities. A microgrid is a localized system that integrates various energy sources, including renewables, storage, and backup generators, to provide a consistent and reliable energy supply. Microgrids can operate independently or be connected to the main grid when available, ensuring flexibility in energy management. These systems often include energy management systems (EMS) that monitor and control energy flows, ensuring that power is used efficiently and that excess energy is stored for future use.
Energy storage is a critical component of small-scale power plants, especially for intermittent renewable energy sources like solar and wind. Battery storage systems, such as lithium-ion or flow batteries, store excess energy generated during peak production periods and release it when demand exceeds generation capacity. These systems help ensure a continuous power supply, even when energy production is low. Additionally, advances in battery technology have made energy storage more affordable and efficient, further enhancing the viability of renewable energy systems for off-grid communities.
The economic viability of small-scale power plants for non-grid connected communities is closely tied to the costs of installation, operation, and maintenance. While the initial capital costs for renewable energy systems may be higher than traditional fossil-fuel-based power sources, the long-term benefits are significant. Renewable energy systems have low operational costs, require minimal fuel inputs, and have longer lifespans compared to diesel generators. Over time, these systems can provide substantial cost savings, especially when considering the rising costs of fuel and the environmental benefits of reducing carbon emissions.
Furthermore, many governments and international organizations offer financial incentives, grants, or subsidies to encourage the adoption of renewable energy technologies in off-grid communities. These incentives can significantly reduce the upfront costs of installing renewable energy systems, making them more accessible for communities with limited financial resources. In some cases, community-based organizations or local businesses may also play a role in financing and operating small-scale power plants, further ensuring that these systems are financially sustainable.
Beyond financial benefits, small-scale power plants also contribute to social and environmental improvements in non-grid connected communities. Reliable access to electricity enables improvements in education, healthcare, and communication. For example, solar-powered lighting and energy-efficient appliances can extend the hours during which children can study and provide lighting for medical facilities, enhancing the overall quality of life. Additionally, the use of clean energy reduces air and water pollution caused by burning fossil fuels and can help mitigate the effects of climate change by reducing greenhouse gas emissions.
The environmental benefits of renewable energy extend beyond the reduction of emissions. For example, by generating electricity from locally sourced biomass, small-scale power plants can help manage waste and reduce the risk of wildfires or other environmental hazards caused by the accumulation of organic materials. Moreover, the implementation of renewable energy systems in non-grid connected communities helps preserve natural resources and ecosystems by reducing the need for resource-intensive, polluting energy infrastructure.
One challenge that non-grid connected communities face is the lack of local technical expertise to install and maintain renewable energy systems. To address this, training programs and capacity-building efforts are essential to ensure that local communities can manage and maintain their energy systems effectively. By developing local skills in renewable energy technologies, communities not only gain the ability to maintain their power plants independently but also foster economic opportunities through the creation of green jobs.
In conclusion, small-scale power plants for non-grid connected communities offer a sustainable, reliable, and cost-effective solution to the energy challenges faced by remote or isolated areas. By harnessing renewable energy sources like solar, wind, hydro, and biomass, these communities can achieve energy independence, reduce their reliance on expensive and polluting fossil fuels, and improve their quality of life. While initial costs can be a barrier, long-term savings, environmental benefits, and the potential for financial incentives make renewable energy systems an increasingly attractive option. With the right planning, technology, and support, small-scale power plants can play a crucial role in powering the future of non-grid connected communities.
Small-scale power plants for non-grid connected communities have the potential to unlock significant benefits beyond just providing electricity. When designed and implemented effectively, these systems can improve the overall resilience of the community, empower local economies, and support sustainable development goals.
The transition to renewable energy solutions also reduces the vulnerability of non-grid connected communities to the price volatility of fossil fuels. Communities that rely on diesel generators, for example, face fluctuating fuel prices that can make energy costs unpredictable and unaffordable. By shifting to renewables like solar, wind, or biomass, these communities can mitigate the impacts of fuel price hikes and reduce the financial burden on households and businesses. Moreover, these systems allow for the long-term stabilization of energy costs, ensuring that future generations have access to affordable energy.
For communities located in areas where natural resources are abundant—such as regions with strong winds, abundant sunlight, or ample water sources—small-scale power plants offer a particularly fitting solution. The ability to leverage local resources for power generation reduces the need for expensive imports and further enhances the resilience of the community. Additionally, utilizing renewable resources for energy generation helps build a sustainable local economy that is less reliant on external factors, leading to more self-sufficiency and greater economic stability.
The energy generated from small-scale power plants can also contribute to improving agricultural productivity in rural areas. For example, electricity from renewable sources can be used to power irrigation systems, enabling year-round crop production and enhancing food security. Energy availability also facilitates the use of modern agricultural equipment and machinery, improving efficiency in farming and supporting the development of value-added agricultural products.
One of the often-overlooked benefits of small-scale renewable energy systems is their role in fostering educational and social development within non-grid connected communities. With access to reliable electricity, schools can stay open longer and provide access to modern teaching tools, such as computers, lighting, and educational videos. Students can study in the evenings, helping to level the playing field for children in rural areas. This improved access to education has the potential to break cycles of poverty and lead to better economic prospects in the future.
Beyond education, healthcare services in remote communities also stand to benefit from the availability of renewable energy. Reliable electricity allows health centers to operate refrigeration for vaccines and medications, power medical equipment, and ensure continuous lighting, especially in emergencies. The ability to maintain cold chains for vaccines and medicines is especially critical in rural and isolated areas, where transport and storage infrastructure may be lacking. In areas where emergency medical services are essential, access to electricity can make the difference between life and death.
In addition to improving public services, small-scale power plants can also create new employment opportunities. The installation, operation, and maintenance of renewable energy systems often require a skilled workforce, which can drive job creation in local communities. These jobs can range from technical positions, such as electricians and engineers, to administrative and managerial roles. Furthermore, when a community becomes more energy self-sufficient, it can attract outside investments and businesses, thereby fostering economic growth and diversification.
The environmental impact of small-scale renewable energy systems cannot be overstated. By shifting away from fossil fuel-based power generation, non-grid connected communities can significantly reduce their carbon footprint. In many cases, small-scale power plants using clean energy help restore the balance of natural ecosystems that may have been affected by conventional energy infrastructure, such as large-scale hydroelectric dams or coal-fired power plants. By using local, renewable resources responsibly, these communities can set an example for sustainable development that others can follow.
To make small-scale renewable energy systems a success, communities must prioritize energy efficiency and sustainability. Implementing energy-saving practices, such as the use of energy-efficient appliances, LED lighting, and proper insulation, can help reduce the overall energy demand and ensure that power is used effectively. Proper system design and sizing are critical to ensuring that the energy needs of the community are met without overburdening the system. Additionally, efficient energy storage solutions, such as advanced batteries or pumped hydro storage, are necessary to manage energy availability and ensure a steady supply during peak demand periods.
While small-scale power plants offer a wealth of benefits, the implementation process can still present challenges. One of the biggest obstacles is securing financing for the upfront costs of installation and infrastructure. Many non-grid connected communities may lack access to traditional financing mechanisms, making it difficult for them to fund renewable energy projects. To address this challenge, governments, non-governmental organizations, and development agencies can provide financial support, grants, and low-interest loans to help communities access renewable energy technology. Furthermore, public-private partnerships can play a critical role in bringing together resources and expertise to successfully implement and sustain these projects.
Another challenge is ensuring the continued operation and maintenance of renewable energy systems. While these systems generally require less maintenance than traditional fossil-fuel-based power plants, periodic inspections, repairs, and upgrades are necessary to maintain optimal performance. Training local community members in the maintenance and management of renewable energy systems can foster a sense of ownership and ensure the sustainability of the projects. Additionally, it reduces dependence on external service providers, which can be costly and logistically challenging, particularly in remote areas.
To foster long-term success, it is essential to involve the community in the planning and decision-making processes. This ensures that the energy system meets the unique needs of the community and has local buy-in. Engaging community members in the design and operation of the energy systems not only improves the chances of success but also empowers individuals and groups to take an active role in shaping their energy future. Community participation can also help identify potential issues early on, creating solutions that are both effective and culturally appropriate.
The integration of renewable energy solutions in non-grid connected communities represents a significant step toward addressing global energy access challenges. These systems help mitigate the negative impacts of conventional energy generation, provide opportunities for economic and social development, and contribute to environmental sustainability. By investing in small-scale power plants and empowering local communities to become energy-independent, we move closer to a more sustainable and equitable energy future for all.
Expanding on the benefits and potential of small-scale power plants for non-grid connected communities, it is important to consider the broader implications these systems have on local development, technological innovation, and resilience to climate change.
The decentralization of energy production via small-scale power plants can act as a catalyst for broader regional development. In many remote communities, energy access is a prerequisite for attracting investment in local industries, businesses, and infrastructure projects. With a stable energy supply, local entrepreneurs can establish businesses such as small manufacturing units, food processing plants, or retail operations that rely on electricity. These ventures not only create jobs but can also help reduce migration to urban areas, as people see opportunities to live and work in their communities, rather than seek work in larger cities.
Technological innovation is another area that benefits from the introduction of small-scale power plants. In non-grid connected communities, the adoption of renewable energy technologies encourages local innovation, particularly in the fields of energy storage, microgrid design, and energy management systems. These innovations can help address the unique challenges of operating in remote environments, such as energy transmission losses, harsh weather conditions, or limited access to technical expertise. In some cases, communities may develop their own solutions or partner with research institutions and private companies to improve the performance and efficiency of renewable energy systems. These innovations not only benefit the community but can also have broader applications in other remote or off-grid areas globally.
Small-scale power plants can also play an important role in enhancing the resilience of non-grid connected communities in the face of climate change. Many remote communities are located in areas that are vulnerable to the impacts of climate change, such as extreme weather events, droughts, or rising sea levels. Conventional energy infrastructure, such as long-distance power transmission lines, is particularly vulnerable to such events. In contrast, small-scale power plants—especially those based on renewable energy—are more resilient to climate disruptions because they are decentralized and can be designed to withstand extreme conditions. For example, solar panels can be designed to be storm-resistant, and wind turbines can be installed to function in low- or high-wind conditions, depending on the local climate. This resilience ensures that communities can continue to receive electricity even during or after extreme weather events, reducing the need for emergency power supplies and making it easier to recover from disasters.
Another important aspect of small-scale power plants is their role in promoting local environmental stewardship. When communities are empowered to manage their own energy resources, they are more likely to prioritize sustainability and conservation. For instance, in areas with abundant biomass, communities may choose to establish sustainable fuel sources, such as energy plantations or waste-to-energy systems, to ensure that their power plants remain viable in the long term. Moreover, by reducing reliance on fossil fuels, small-scale power plants decrease local air pollution, improve health outcomes, and preserve the natural environment. These benefits align with global sustainability goals and contribute to a cleaner, healthier environment for future generations.
The role of policy and regulatory frameworks is also pivotal in ensuring the success of small-scale power plants in non-grid connected communities. Governments play a key role in facilitating the deployment of renewable energy technologies by providing incentives, reducing regulatory barriers, and ensuring that policies are in place to support both the development and maintenance of these systems. In some countries, governments have established programs to promote off-grid energy solutions, offering subsidies or tax breaks to businesses and communities that invest in renewable energy systems. In others, policymakers are working to create more favorable conditions for microgrid development and the integration of energy storage solutions.
The integration of small-scale renewable power plants into national or regional energy strategies is essential to achieving energy access for all. Policymakers can create frameworks that enable these systems to be included in the broader energy mix, ensuring that they complement centralized grid infrastructure where available. By supporting small-scale power plants through favorable regulations, funding mechanisms, and technical assistance, governments can help bridge the energy access gap and encourage innovation in the energy sector.
Furthermore, global organizations, development agencies, and international financial institutions can play a key role in financing small-scale renewable energy projects in non-grid connected communities. These entities can provide concessional loans, grants, and technical assistance to support the installation of renewable energy systems in rural and isolated areas. Their support can help overcome the initial capital costs and make renewable energy systems financially viable for communities that may otherwise struggle to afford them. International collaboration and funding mechanisms ensure that energy access becomes part of the global agenda and that the benefits of renewable energy reach the communities that need it most.
As small-scale power plants become more prevalent in non-grid connected communities, they can also serve as a model for broader energy transitions. These systems demonstrate how decentralized energy production can reduce the reliance on fossil fuels, improve energy access, and contribute to environmental sustainability. The lessons learned from these projects can be applied to urban and industrial energy systems, where decentralized renewable energy technologies are increasingly seen as a viable alternative to traditional grid-based infrastructure. In this way, small-scale renewable energy solutions help pave the way for a larger-scale energy revolution, driving global efforts to reduce carbon emissions, promote sustainability, and create more resilient communities.
In conclusion, the adoption of small-scale power plants in non-grid connected communities offers a wide range of benefits that extend beyond energy provision. From fostering local economic development and technological innovation to enhancing resilience against climate change and improving environmental sustainability, these systems have the potential to transform communities in meaningful ways. With the right support from governments, international organizations, and local stakeholders, small-scale power plants can provide a pathway to a more sustainable, equitable, and resilient energy future for remote and underserved populations worldwide.
In the evolving landscape of energy access, small-scale power plants for non-grid connected communities not only address immediate energy needs but also create a foundation for long-term growth and prosperity. These systems offer solutions to many of the challenges that remote and underserved communities face, including environmental sustainability, social equity, and economic development. By leveraging local renewable energy resources, communities can build self-sufficiency, reduce their dependency on external energy sources, and improve the overall quality of life for their residents.
An essential aspect of ensuring the continued success and scalability of small-scale power plants is integrating them into broader regional and national development strategies. As renewable energy technologies continue to advance, small-scale power plants can serve as a stepping stone toward a more interconnected and decentralized energy system. By connecting these off-grid systems with microgrids or integrating them into national energy grids when available, communities can enjoy the best of both worlds—local energy autonomy and access to centralized power during periods of high demand or energy shortfall.
In many cases, small-scale power plants provide a unique opportunity to create hybrid energy systems that maximize energy security. For example, in areas prone to seasonal weather changes, a combination of solar, wind, and battery storage can provide a consistent and reliable energy supply. In regions with fluctuating water resources, hybridizing hydropower with biomass or solar power can help balance generation during dry seasons or low-water periods. This approach increases resilience and ensures communities are not vulnerable to power shortages due to the limitations of any one energy source.
Another key advantage of small-scale renewable energy systems is their ability to offer flexibility and customization. Unlike traditional large-scale power plants, which may require significant infrastructure and are often limited by the geographic and logistical challenges of their location, small-scale power plants can be tailored to meet the specific energy demands and environmental conditions of a community. This customizability allows for more efficient use of resources and ensures that energy generation is aligned with local needs. For example, a village near a river may rely on a small hydroelectric system, while a community in a sunny region may prioritize solar power. Similarly, biomass-based systems may be better suited to areas with significant agricultural or forestry activity.
Importantly, the deployment of small-scale power plants can be a catalyst for local entrepreneurship and innovation. With energy access comes the opportunity to expand small businesses, promote the development of new technologies, and create local job opportunities. Small-scale power plants themselves can provide local employment in areas such as system installation, operation, and maintenance. Beyond this, electricity can stimulate new industries that rely on a stable energy supply, such as small-scale food processing, woodworking, or textile production. The creation of such industries fosters economic diversification and ensures that local communities are not overly reliant on any single sector.
As these systems begin to provide reliable energy, other aspects of community life begin to improve as well. Schools, healthcare facilities, and small businesses benefit from the reliable electricity generated by small-scale power plants, leading to better outcomes in education, health, and economic well-being. Additionally, access to clean, renewable energy improves the overall standard of living. For example, cooking with electricity or efficient cookstoves powered by renewable energy can significantly reduce indoor air pollution, a major cause of respiratory issues in rural communities. Similarly, lighting and electricity for appliances make everyday life more comfortable and productive.
One of the most significant impacts of small-scale power plants is their role in addressing global energy access inequality. According to the International Energy Agency (IEA), approximately 770 million people still live without access to electricity, with a disproportionate number of these individuals located in sub-Saharan Africa and parts of South Asia. Small-scale power plants, particularly those based on renewable energy, offer a feasible solution to bridging this energy access gap. They provide a cost-effective way to bring electricity to communities that have been left behind by traditional grid infrastructure, offering an equitable path to development.
Moreover, small-scale renewable energy systems can play an essential role in achieving international climate targets. As more communities transition to clean, renewable energy, global greenhouse gas emissions from fossil fuels decrease, contributing to climate change mitigation efforts. These local energy solutions help to decentralize energy production, reducing transmission losses and minimizing the need for long-distance energy transport, which often leads to additional emissions. By investing in small-scale renewable systems, countries can meet their climate goals while also providing essential services to their citizens.
The impact of small-scale power plants is not limited to local communities; it has the potential to influence national and global energy policies. As these systems proliferate, governments are encouraged to review and revise their energy policies to accommodate decentralized power generation. Many countries are already adopting favorable policies, such as feed-in tariffs, power purchase agreements, and tax incentives, which encourage the development of renewable energy projects. In turn, these policies help reduce the overall cost of renewable energy technologies, making them more accessible and affordable for off-grid communities.
The financial sustainability of small-scale power plants is a critical factor for their long-term success. While the initial capital required for the installation of renewable energy systems can be high, the operational and maintenance costs are generally low. Over time, the financial benefits become evident, as communities experience reduced energy costs, greater energy security, and the ability to reinvest savings into other areas of development. Additionally, the use of renewable resources reduces the need for costly fuel imports, creating savings that can be used for other community priorities, such as education, healthcare, or infrastructure.
Financing models that support small-scale renewable energy systems vary across regions but typically involve a combination of public and private sector investments. Governments can help by providing subsidies, low-interest loans, or grants to offset the initial costs of installation. Microfinance institutions, impact investors, and development banks also play an important role in funding small-scale energy projects, especially in countries with limited access to traditional financial services. Additionally, innovative financing models, such as pay-as-you-go systems or energy-as-a-service platforms, enable communities to pay for energy generation and storage infrastructure in smaller, more manageable installments, making it easier to afford these systems.
One of the challenges to scaling up small-scale power plants is the lack of adequate training and technical expertise in remote communities. To ensure that energy systems are properly maintained and managed, capacity-building efforts are crucial. Training local technicians, engineers, and energy managers can empower communities to take control of their energy resources, increasing system reliability and ensuring that issues are addressed quickly. Furthermore, these local technicians can play a role in educating their communities about energy efficiency practices and the benefits of renewable energy.
In conclusion, small-scale power plants for non-grid connected communities present a powerful tool for tackling the world’s energy access challenges. These systems offer reliable, renewable, and affordable energy solutions that drive social, economic, and environmental benefits. By fostering energy independence, promoting local innovation, and supporting sustainable development, small-scale power plants help pave the way for a more equitable and resilient future. With the right policies, financing, and technical support, these systems can transform lives and contribute to the global transition to a sustainable energy future.
Small Scale Power Plant for Plastic Recycling Plants
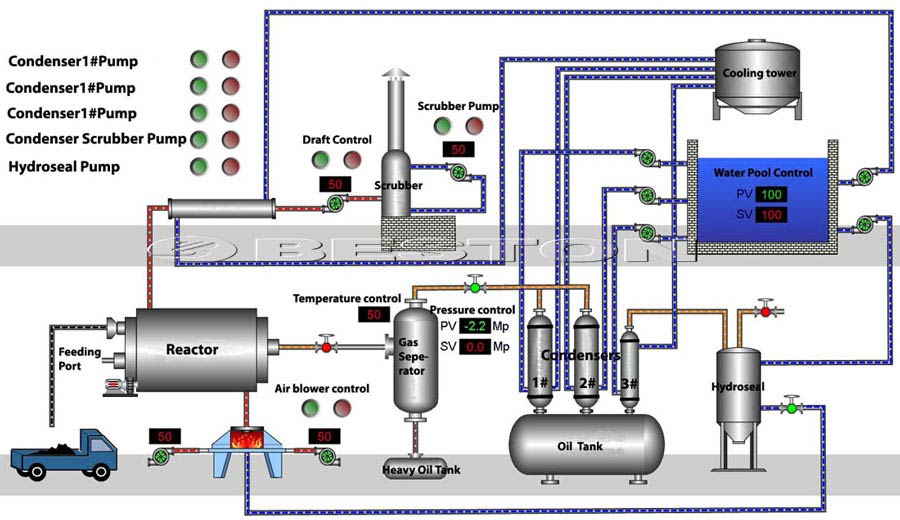
Small-scale power plants for plastic recycling plants offer a highly effective and sustainable solution to meet the energy demands of recycling operations while addressing the growing challenge of plastic waste management. As the world grapples with the environmental impact of plastic pollution, innovative solutions like these can help tackle both energy supply and waste disposal issues simultaneously. By harnessing local resources and renewable energy sources, small-scale power plants can power plastic recycling facilities in an environmentally friendly and cost-effective way, contributing to a circular economy where materials are reused rather than disposed of in landfills or oceans.
Plastic recycling plants often require significant energy for processes like shredding, washing, and melting plastics, as well as operating machinery that sorts and processes the materials. Traditional power sources for such operations are often fossil fuel-based, which not only increases carbon emissions but also makes these facilities vulnerable to fluctuations in energy prices. Small-scale power plants, especially those that rely on renewable energy sources, offer a viable alternative. Renewable energy technologies such as solar, wind, biomass, or waste-to-energy systems can provide a reliable and cost-effective energy supply to these plants, reducing their environmental impact and promoting sustainability.
One of the primary advantages of small-scale power plants for plastic recycling is that they can be integrated with waste-to-energy technologies, which allow for the generation of power from plastic waste itself. Waste-to-energy systems, such as pyrolysis or gasification, break down plastic materials into usable energy forms, such as electricity or heat, by using high-temperature processes. This approach not only addresses the plastic waste issue but also provides a continuous energy supply to the recycling plant. Additionally, these systems reduce the need for external energy sources, helping to lower operational costs and reduce the facility’s carbon footprint.
For example, a plastic recycling plant that uses waste-to-energy systems can convert low-value, non-recyclable plastics (such as mixed plastic packaging or contaminated plastics) into energy. These materials are typically difficult to process through conventional recycling methods but can be transformed into fuel through pyrolysis or gasification. This energy can then be used to power the recycling plant, further reducing its dependence on traditional energy sources and minimizing waste. Furthermore, the carbon emissions produced by these processes are typically much lower than those generated by fossil fuel-based power plants, making this an environmentally friendly approach to both recycling and energy generation.
Biomass is another renewable energy source that can be utilized in small-scale power plants for plastic recycling facilities. If the recycling plant is located in an area with access to agricultural or forest waste, this biomass can be used to generate energy through combustion or anaerobic digestion. For example, wood chips, agricultural residues, or organic waste can be burned in a biomass boiler to produce heat and electricity, which can be used to power the plant. This type of system helps recycle organic materials while also providing a sustainable energy source to support the plastic recycling process.
Solar and wind energy technologies can also play a critical role in powering small-scale plastic recycling plants. Solar panels installed on the roofs of recycling facilities or in nearby areas can provide a clean, renewable energy source for day-to-day operations. Likewise, wind turbines can be installed in suitable locations to harness the power of the wind, especially in regions with high wind potential. Both solar and wind energy are particularly well-suited for areas where traditional energy infrastructure may be lacking or unreliable, helping to ensure a steady and uninterrupted power supply.
In terms of energy efficiency, small-scale power plants designed for plastic recycling facilities can incorporate advanced energy management and storage systems to optimize energy use. Energy storage solutions, such as batteries or thermal storage, can help balance the supply and demand for energy by storing excess energy produced during periods of high generation and releasing it when needed. This ensures that the recycling plant can operate continuously without interruptions, even when renewable energy sources like solar or wind are not producing electricity. Additionally, energy management systems can monitor and control energy consumption, helping to reduce waste and improve overall efficiency.
The use of small-scale power plants also offers economic benefits for plastic recycling plants. By reducing reliance on external electricity sources, recycling facilities can lower their operating costs and make their operations more cost-effective in the long run. In some cases, the savings generated from using renewable energy may allow these plants to expand their capacity or invest in more advanced technologies to improve their recycling processes. Moreover, small-scale power plants provide a more stable and predictable energy supply, which is crucial for optimizing the performance and output of recycling machinery. Predictable energy costs and availability can also make recycling plants more attractive to investors, enabling further growth in the sector.
Another significant advantage of small-scale power plants for plastic recycling plants is their contribution to sustainability and the circular economy. By using renewable energy sources and integrating waste-to-energy technologies, these plants help reduce greenhouse gas emissions, minimize landfill waste, and promote the reuse of materials. The circular economy aims to keep products, materials, and resources in use for as long as possible, and small-scale power plants aligned with this principle can ensure that plastic waste is managed efficiently and sustainably. Moreover, reducing the reliance on fossil fuels helps decrease the overall carbon footprint of the recycling industry.
Local communities also benefit from the deployment of small-scale power plants for plastic recycling plants. In many areas, recycling plants are located in rural or industrial zones, where access to reliable electricity may be limited. By providing renewable energy to these plants, small-scale power plants can help improve local energy access, create jobs, and stimulate economic development. The construction, operation, and maintenance of renewable energy systems can generate employment opportunities for local workers and promote skills development in areas like engineering, electrical maintenance, and energy management.
As the plastic recycling industry continues to grow and evolve, it is crucial for recycling facilities to adopt sustainable and efficient energy solutions. Small-scale power plants that utilize renewable energy sources and waste-to-energy technologies offer a promising way to address both the energy needs of these facilities and the environmental challenges of plastic waste. By reducing the environmental footprint of recycling plants, creating local economic opportunities, and contributing to the global effort to manage plastic waste, small-scale power plants can play a key role in advancing sustainability in the recycling sector.
The implementation of small-scale power plants for plastic recycling plants is not without challenges, however. One of the primary obstacles is the initial capital investment required for the installation of renewable energy systems and waste-to-energy technologies. The cost of solar panels, wind turbines, and biomass boilers can be significant, and many recycling facilities may not have the financial resources to invest in these systems upfront. However, there are a variety of financial mechanisms available, including government incentives, grants, and private sector financing, which can help offset these costs and make renewable energy technologies more accessible.
Another challenge is the need for appropriate technical expertise to design, install, and maintain small-scale power plants. Recycling plants may require specialized knowledge to integrate renewable energy solutions into their existing infrastructure and optimize energy use. Training and capacity-building programs for local technicians and engineers are essential to ensure that these systems can be installed and operated effectively, ensuring long-term sustainability.
Despite these challenges, the benefits of small-scale power plants for plastic recycling plants far outweigh the obstacles. With careful planning, investment, and the right technological solutions, these power plants can transform the way plastic recycling facilities operate, making them more sustainable, cost-effective, and resilient in the long term. By combining waste management with renewable energy generation, small-scale power plants not only contribute to the circular economy but also help build a cleaner, more sustainable future for communities and industries around the world.
Expanding on the role of small-scale power plants for plastic recycling plants, it’s important to highlight the critical nature of these systems in advancing the efficiency and sustainability of the recycling sector. As the demand for more responsible waste management and resource conservation grows, integrating renewable energy technologies into recycling operations allows facilities to meet these demands while ensuring their energy supply is both environmentally friendly and economically viable.
Another critical aspect is the role of innovation in enhancing the potential of small-scale power plants. As technological advancements in renewable energy systems continue to evolve, power plants can become more efficient and cost-effective. For example, improvements in solar panel efficiency, energy storage systems, and waste-to-energy technologies can help reduce the overall cost of energy production, making it more accessible for small-scale recycling facilities. Furthermore, new solutions such as energy optimization software can help maximize the output from renewable energy sources, ensuring that recycling plants are operating at peak efficiency.
Additionally, there is a growing opportunity for plastic recycling plants to diversify their energy sources. While waste-to-energy systems may be a primary source of power, integrating multiple renewable energy technologies can further optimize energy generation. For instance, solar power can supplement the energy needs of recycling plants during the day, while biomass or wind energy could provide power during the night or during periods when other renewable sources are not generating sufficient energy. This hybrid approach not only increases energy reliability but also maximizes the use of available local resources, reducing the facility’s overall reliance on external energy.
Incorporating energy efficiency practices into the design and operation of plastic recycling plants can also help reduce overall energy consumption. By upgrading machinery, using more energy-efficient equipment, and implementing better insulation and heat recovery systems, recycling plants can lower their energy demand and improve operational costs. Small-scale power plants can be tailored to meet these optimized energy needs, ensuring that each plant has a highly customized energy solution that aligns with its specific processes.
From an economic standpoint, the integration of small-scale power plants can be seen as an investment in long-term sustainability. As energy prices fluctuate and demand for cleaner energy sources increases, recycling facilities with renewable energy systems are positioned to stay ahead of market shifts. In addition to reducing energy costs over time, these plants can also benefit from various government policies and incentives that encourage renewable energy use. Feed-in tariffs, carbon credits, tax incentives, and green energy subsidies are examples of policy mechanisms that can help lower the capital investment required to implement renewable energy systems, making them more accessible for small-scale recycling operations.
Furthermore, adopting renewable energy solutions can improve the overall public image and corporate social responsibility (CSR) of recycling companies. As consumers, investors, and governments place increasing importance on sustainability, facilities that demonstrate a commitment to green practices by integrating renewable energy into their operations will be seen as leaders in the circular economy. This enhanced reputation can help attract new customers, investors, and partners who value environmental responsibility, providing a competitive edge in an increasingly eco-conscious market.
From a policy perspective, governments around the world are beginning to prioritize waste-to-energy and renewable energy systems as part of their broader environmental and energy strategies. Small-scale power plants for plastic recycling facilities can play a key role in meeting national and international climate goals by reducing the carbon footprint of the recycling industry and decreasing the reliance on fossil fuels. As part of global initiatives to reduce greenhouse gas emissions and limit the impacts of climate change, incentivizing the integration of renewable energy into recycling operations aligns with efforts to promote cleaner industrial practices.
The implementation of small-scale renewable energy systems in plastic recycling plants also contributes to broader sustainability objectives by fostering resource efficiency and reducing waste. In a circular economy, the focus is on keeping resources in use for as long as possible, reducing the need for raw material extraction and minimizing environmental pollution. By utilizing local renewable energy resources and reducing waste through the conversion of plastic materials into energy, small-scale power plants help create a more sustainable, efficient, and resilient recycling system.
At the community level, the adoption of renewable energy systems in plastic recycling facilities can have significant socio-economic benefits. As renewable energy becomes more widespread, local communities may benefit from cleaner air and water, reduced pollution, and improved health outcomes due to the decrease in toxic emissions from fossil fuel-based power generation. Additionally, the expansion of the recycling sector through small-scale power plants creates jobs in the renewable energy industry, such as in the installation, operation, and maintenance of renewable energy systems, as well as in recycling operations. These jobs can help stimulate economic development, particularly in regions with high unemployment or underdeveloped industries.
The collaborative potential between plastic recycling and small-scale power plants opens up new possibilities for both industries. These systems can lead to innovative solutions in energy generation, waste management, and resource optimization. By partnering with energy providers, waste management companies, and government agencies, recycling facilities can expand their capacity, adopt new technologies, and secure long-term energy supply while also contributing to global sustainability efforts.
In conclusion, small-scale power plants for plastic recycling plants are a transformative tool that addresses both the environmental and energy challenges faced by recycling operations. By integrating renewable energy sources such as waste-to-energy, biomass, solar, and wind, these plants reduce dependence on fossil fuels, lower operational costs, and promote sustainability. The potential for energy optimization, job creation, and enhanced community development further underscores the importance of small-scale power plants in the circular economy. As technological innovations continue to advance and renewable energy becomes more affordable, small-scale power plants will be integral in driving the growth of the plastic recycling industry, enabling facilities to meet the rising demand for efficient, sustainable waste management solutions.
Continuing with the discussion on small-scale power plants for plastic recycling plants, it’s essential to explore the practical challenges and potential solutions that could enhance their effectiveness. While the benefits of integrating renewable energy solutions into plastic recycling are numerous, some technical, economic, and logistical hurdles must be addressed to ensure long-term success.
Technical Challenges and Solutions: One of the key challenges in implementing small-scale power plants in plastic recycling facilities is the integration of renewable energy technologies into existing infrastructure. Many recycling plants operate in environments with established systems that rely on traditional energy sources, such as the grid or diesel generators. Converting these plants to operate with renewable energy systems can require significant modifications to their infrastructure and processes. For example, energy storage systems, grid connection equipment, and energy management software must be installed to allow for seamless integration between renewable power sources and the plant’s operations.
To address this, partnerships with experienced energy developers and engineers can help ensure the successful design and integration of renewable energy solutions. Specialized companies that focus on retrofitting industrial plants with energy-efficient technologies can conduct detailed feasibility studies to determine the best renewable energy mix based on the plant’s location, size, and energy needs. These partnerships also provide access to expertise in designing systems that are scalable, adaptable, and efficient, ensuring the long-term sustainability of the plant.
Another technical challenge is the intermittency of renewable energy sources, such as solar and wind. These energy sources are not constant and may not align with the plant’s energy demand, leading to periods of low or no energy production. To overcome this, plastic recycling plants can incorporate hybrid energy systems that combine multiple renewable sources (e.g., wind and solar) with energy storage systems, like batteries or thermal storage. These storage solutions can store excess energy produced during periods of high generation and release it when energy demand exceeds supply. This hybrid approach ensures that the recycling plant has a continuous and reliable energy source, even when solar or wind production fluctuates.
Economic Considerations: While renewable energy systems offer long-term cost savings and sustainability, the initial investment required for installing small-scale power plants can be a barrier for many plastic recycling facilities. The costs associated with purchasing and installing solar panels, wind turbines, waste-to-energy technologies, and associated infrastructure (such as energy storage systems) can be significant, particularly for small or medium-sized recycling operations.
To mitigate this, governments and financial institutions can play a critical role in facilitating access to funding and financing options. Green financing mechanisms, such as low-interest loans, subsidies, or tax incentives, can help reduce the upfront capital costs for recycling facilities. In many countries, government programs support the adoption of renewable energy and waste-to-energy technologies, providing financial assistance for projects that contribute to sustainability and carbon reduction goals.
In addition to government incentives, private sector investments from environmentally conscious investors or venture capitalists can help support the development of small-scale renewable energy systems for recycling plants. Partnerships with energy service companies (ESCOs) that specialize in renewable energy projects can also offer financing options, including performance-based contracts, where the plant pays for the energy services provided by the renewable systems over time, thus avoiding the initial capital investment burden.
From a cost perspective, it is also important to consider the operational savings generated by the implementation of renewable energy systems. While the initial investment may be high, the long-term savings on energy bills, reduced reliance on fossil fuels, and decreased exposure to fluctuating energy prices can provide a significant return on investment (ROI). By reducing energy costs, recycling plants can improve their bottom line and reinvest savings into further technological upgrades or capacity expansion.
Operational Challenges and Optimization: Once renewable energy systems are integrated into plastic recycling facilities, ongoing maintenance and optimization become crucial for ensuring their efficient operation. Maintenance of energy infrastructure, including solar panels, wind turbines, biomass boilers, and energy storage systems, requires specialized knowledge and skilled personnel. To address this, training programs and certification courses for local technicians can ensure that there is a steady supply of qualified workers who can maintain, repair, and optimize renewable energy systems.
Moreover, the use of advanced monitoring and control systems can help improve the efficiency and performance of these systems. Energy management software can be employed to track energy generation, consumption, and storage levels in real time, allowing plant operators to make informed decisions about energy usage. For example, the software could automatically adjust energy flows from solar, wind, or waste-to-energy systems based on real-time demand, ensuring that the plant uses energy most efficiently.
Regular performance audits and system assessments are also essential to ensure that the renewable energy infrastructure is functioning at optimal levels. By conducting periodic assessments of the energy systems, recycling plants can identify any issues that may arise (such as decreased energy production from solar panels due to dirt or debris accumulation) and address them promptly to maintain high efficiency.
Logistical Considerations and Supply Chain Challenges: Small-scale power plants for plastic recycling plants often depend on locally sourced renewable energy inputs, such as biomass or waste materials for energy generation. One of the logistical challenges that may arise is the reliable and consistent supply of these raw materials. Biomass, for example, may be sourced from agricultural or forest waste, but it requires a stable supply chain to ensure that energy production remains consistent.
To address this challenge, recycling plants can establish local partnerships with farmers, forestry operations, or waste management companies to create a reliable supply chain for biomass or other renewable energy inputs. Collaborative efforts between recycling plants and local communities can also help establish sustainable waste management practices that support energy production while addressing environmental concerns.
Additionally, the transport of materials to the plant can be a challenge, especially for remote locations. Efficient supply chain management practices, including bulk purchasing, local sourcing, and optimized logistics, can help ensure a steady supply of renewable energy materials at a reasonable cost. Developing multi-year contracts with suppliers or forming cooperatives between multiple recycling facilities can help stabilize the supply chain and reduce the risk of energy shortages.
Regulatory and Policy Landscape: Government policies and regulations play a significant role in shaping the success of small-scale power plants for plastic recycling plants. In some regions, there are specific regulations governing the use of renewable energy systems and waste-to-energy technologies, which may include permits, environmental assessments, and emission controls. Recycling facilities need to navigate these regulatory frameworks to ensure compliance and avoid legal or financial penalties.
On the other hand, favorable policies that promote renewable energy adoption, such as feed-in tariffs, tax credits, or carbon pricing schemes, can create a more favorable environment for small-scale power plants. By providing incentives for using clean energy and reducing greenhouse gas emissions, these policies encourage recycling plants to adopt renewable energy systems and improve their environmental footprint.
As the global push for sustainability and carbon neutrality continues, governments worldwide are likely to increase support for industries that contribute to circular economies, such as recycling plants. Recycling facilities that integrate renewable energy systems not only align with national climate goals but also contribute to the transition toward a more sustainable, low-carbon economy.
Conclusion: Small-scale power plants for plastic recycling plants represent a promising avenue for addressing the dual challenges of energy supply and plastic waste management. By leveraging renewable energy sources like waste-to-energy, biomass, solar, and wind, these plants can reduce their environmental impact, lower energy costs, and promote sustainability within the recycling sector. Though challenges such as capital investment, technical integration, and supply chain logistics remain, the long-term benefits—ranging from operational cost savings to increased energy reliability—make small-scale power plants a worthwhile investment.
As renewable energy technologies continue to improve and become more affordable, and as policies evolve to support green energy initiatives, small-scale power plants are poised to play a key role in the future of plastic recycling. By embracing innovative, sustainable energy solutions, plastic recycling plants can enhance their operations, reduce their carbon footprint, and contribute to global efforts in managing plastic waste more effectively.
Building on the long-term vision for small-scale power plants in plastic recycling facilities, several additional aspects warrant attention to fully realize their potential within the recycling and waste management sectors.
Technological Innovations: As renewable energy technologies continue to evolve, the integration of new innovations into small-scale power plants for plastic recycling will enhance efficiency and cost-effectiveness. For example, advances in energy storage technologies, such as next-generation batteries, will provide better solutions for managing the intermittent nature of renewable energy sources like solar and wind. These innovations will help recycling plants better balance energy production with consumption, ensuring a constant power supply even during off-peak generation periods. Furthermore, the development of more efficient waste-to-energy conversion technologies, such as advanced gasification systems or pyrolysis units, will allow recycling facilities to generate more energy from the same amount of plastic waste, improving overall energy output.
Similarly, improvements in energy optimization software and smart grid technology can help recycling plants achieve better energy management. By using real-time data and predictive analytics, energy consumption can be adjusted based on demand patterns, preventing energy waste and ensuring that production runs smoothly. These technologies can also assist in identifying energy-saving opportunities, such as adjusting heating and cooling systems or utilizing waste heat recovery systems to improve plant efficiency.
Circular Economy and Extended Producer Responsibility: The concept of a circular economy plays a pivotal role in shaping the future of small-scale power plants for plastic recycling. In a circular economy, the goal is to minimize waste, maximize resource reuse, and reduce the need for virgin raw materials. Small-scale power plants integrated with recycling facilities contribute to this model by enabling the conversion of plastic waste into valuable energy, which can power the recycling processes themselves or even supply energy to nearby communities.
The growing importance of Extended Producer Responsibility (EPR) programs, in which manufacturers are held accountable for the lifecycle of their products—including post-consumer waste—also supports the development of small-scale power plants for recycling. As more plastic manufacturers are encouraged or required to fund the recycling and proper disposal of their products, the demand for efficient, sustainable recycling solutions will increase. Small-scale power plants will be key to helping these facilities meet their energy needs while promoting the responsible disposal and repurposing of plastic materials.
Moreover, EPR programs can encourage innovation within the recycling sector, prompting the development of new technologies and business models that incorporate renewable energy. By creating a financial incentive for manufacturers to reduce plastic waste and promote recycling, these programs can drive investments in small-scale power plants that integrate renewable energy generation into the recycling process, further closing the loop in the circular economy.
Decentralization of Waste Management and Energy Systems: As decentralized energy generation systems become more widespread, small-scale power plants for plastic recycling have the potential to become a core component of localized waste management solutions. In many regions, particularly rural or off-the-grid communities, access to centralized power grids is limited or non-existent. For these communities, plastic waste can be a valuable energy resource, helping to meet local energy needs while simultaneously addressing waste disposal issues.
Small-scale power plants can be deployed in isolated regions, such as remote villages or islands, where they can independently supply both energy and waste management services. These systems can serve as a resilient and self-sufficient solution for areas that struggle with waste management and energy supply challenges. By utilizing plastic waste as a resource for generating energy, off-grid communities can reduce their reliance on imported fossil fuels, lower energy costs, and create local employment opportunities in both the recycling and energy sectors.
In addition, the growing trend toward distributed energy generation allows for a more flexible and resilient energy grid. Small-scale power plants for recycling plants can contribute to a decentralized energy system that is less vulnerable to disruptions and more adaptable to local energy needs. These systems can be interconnected to form microgrids, where multiple small power plants work together to create a robust and flexible energy network that can respond to changes in demand, weather patterns, and other variables.
Synergies with Other Industrial Sectors: Beyond the direct benefits to plastic recycling plants, small-scale power plants can create synergies with other industries that contribute to the waste management and recycling ecosystem. For example, industries like automotive manufacturing, electronics production, and construction generate large amounts of plastic waste that can be processed and converted into energy at recycling facilities.
Small-scale power plants located near such industries can form collaborative partnerships, allowing for shared energy resources and infrastructure. This could reduce the overall cost of energy for both industries while simultaneously addressing waste management challenges. Additionally, industries that produce large quantities of plastic waste may be incentivized to invest in small-scale power plants to handle their waste more sustainably, either by purchasing energy directly from these plants or through partnership arrangements.
This collaborative approach can foster a circular economy within local industrial hubs, where waste from one industry becomes a resource for another. The integration of small-scale power plants into the broader industrial ecosystem can increase recycling rates, lower costs, and reduce overall environmental impact.
Public Awareness and Consumer Engagement: As sustainability continues to gain traction as a global priority, public awareness and consumer engagement in recycling efforts will be crucial for the success of small-scale power plants for plastic recycling. Educating the public about the value of recycling and the role that renewable energy plays in the process can foster greater community participation and support for recycling programs.
In addition, as consumers increasingly demand products and services from environmentally responsible companies, businesses that adopt small-scale renewable energy systems for their recycling operations can differentiate themselves in the market. This consumer-driven pressure will encourage more companies to invest in clean energy solutions for their recycling processes, leading to a broader adoption of small-scale power plants.
Consumer engagement can also help promote behavior changes, such as reducing plastic consumption and encouraging more sustainable packaging and recycling practices. As consumer demand shifts toward products made from recycled materials and produced using renewable energy, the plastic recycling industry will be incentivized to adopt cleaner, more efficient technologies, including small-scale power plants.
Policy and Regulatory Support: Government policies will continue to play a critical role in the success of small-scale power plants in plastic recycling. As the importance of reducing plastic waste and advancing clean energy grows, policymakers can support the integration of renewable energy into recycling operations by providing grants, tax incentives, and subsidies. These incentives could encourage both the installation of small-scale power plants and the adoption of waste-to-energy technologies, making it financially viable for smaller businesses and local governments to implement these systems.
Additionally, local and regional policies that promote waste reduction, recycling, and renewable energy use can help drive demand for integrated energy and recycling solutions. For example, bans on single-use plastics and mandates for higher rates of plastic recycling can increase the supply of plastic waste available for energy generation, creating a sustainable resource stream for small-scale power plants. Combined with energy policies that prioritize the expansion of clean energy sources, these regulations can create an environment that supports the growth of small-scale power plants.
Conclusion: Small-scale power plants for plastic recycling represent a dynamic and innovative solution to the challenges of waste management and energy production. By combining renewable energy technologies with waste-to-energy systems, these plants not only reduce the environmental impact of plastic waste but also provide a sustainable and cost-effective energy solution for recycling facilities. Technological advancements, economic incentives, regulatory support, and synergies with other industries will all contribute to the continued success and expansion of these systems.
As the circular economy continues to evolve, small-scale power plants will play an increasingly important role in driving sustainability within the recycling industry. By turning plastic waste into valuable energy, these plants create a more resilient, efficient, and sustainable waste management system. With the right mix of policy support, technological innovation, and consumer engagement, small-scale power plants can help lead the way toward a cleaner, greener, and more circular future.
Small Scale Power Plant for Military and Police Stations
Small-scale power plants for military and police stations represent a critical and practical solution to enhance the energy resilience, operational efficiency, and sustainability of these facilities, particularly in remote or off-grid locations. Military and police operations often require a reliable and continuous power supply to ensure safety, security, and operational readiness. With the growing emphasis on sustainability and reducing dependence on traditional grid-based power, integrating renewable energy solutions in the form of small-scale power plants can offer significant advantages.
Energy Reliability and Security: Military and police stations often operate in environments where energy reliability is paramount. These facilities need a constant power supply to ensure that critical operations, such as communications, surveillance, lighting, and power for weapons and other equipment, are uninterrupted. In remote locations, grid power may not be available, or it may be unreliable due to weather conditions, natural disasters, or damage to the grid infrastructure.
Small-scale power plants can serve as an independent energy source, ensuring that military and police stations are not dependent on external power grids. By using renewable energy technologies such as solar, wind, or biomass, these facilities can generate their own power locally, reducing their vulnerability to power outages or disruptions. Furthermore, the use of energy storage systems, such as batteries or thermal storage, can help ensure that there is always a reliable power supply, even when renewable generation is low (e.g., during cloudy weather or at night).
Having an independent energy source also improves energy security, which is particularly important for military and police stations involved in national security or critical law enforcement activities. In times of crisis or conflict, access to reliable power is essential for communication, surveillance, and the operation of other essential equipment. A small-scale power plant can help safeguard against energy supply disruptions caused by geopolitical tensions or sabotage of infrastructure.
Environmental Sustainability and Cost Reduction: Integrating small-scale renewable energy systems in military and police stations also aligns with the growing global focus on environmental sustainability. Military and law enforcement agencies are increasingly being tasked with reducing their carbon footprints and adopting green technologies. Small-scale power plants that use clean energy sources, such as solar panels, wind turbines, or waste-to-energy systems, can help these facilities reduce their reliance on fossil fuels, lower greenhouse gas emissions, and contribute to national or global sustainability goals.
The long-term cost savings associated with renewable energy can also be a significant advantage for military and police stations. While the initial capital investment required for installing renewable energy systems can be high, the ongoing operational costs are generally much lower than traditional energy sources. By reducing the need to purchase fuel or pay for electricity from the grid, these facilities can reduce their operating costs over time. In addition, renewable energy technologies are becoming more affordable as they mature, making them an increasingly attractive option for budget-conscious organizations.
Self-Sufficiency and Off-Grid Operations: For military bases, police stations, and other facilities located in remote or off-grid locations, small-scale power plants provide a level of self-sufficiency that is crucial for operational success. In some cases, these facilities are located in areas far from the nearest power grid or have only limited access to external energy sources. In such cases, a small-scale power plant becomes the sole energy provider, ensuring that operations can continue without interruption.
For example, military bases in remote desert or mountain regions, or police stations in rural or conflict zones, may face challenges in obtaining a reliable supply of fuel or electricity. Installing small-scale power plants that harness renewable energy from local resources—such as solar power in sunny regions, wind power in windy areas, or biomass from organic waste—allows these stations to operate independently, reducing the logistical challenges of fuel transport and ensuring consistent power supply.
This self-sufficiency also extends to the emergency response capacity of these stations. Whether it’s for disaster response, military operations, or national security efforts, having a local energy source means that stations can operate during critical times without worrying about energy shortages or delays in fuel deliveries. By relying on locally available renewable resources, small-scale power plants enhance both the flexibility and autonomy of military and police stations.
Mobility and Temporary Installations: In some situations, military units or police forces may need to deploy temporary installations in the field, such as forward operating bases or mobile command posts. For these situations, small-scale power plants can provide a portable and reliable energy source. Mobile renewable energy solutions, such as solar generators or wind turbines, can be quickly deployed and set up in various locations to support military operations or police missions, providing power for essential systems such as communications, lighting, medical equipment, and surveillance.
These mobile power systems are particularly valuable in conflict zones, disaster-stricken areas, or remote locations where traditional energy infrastructure is either absent or unreliable. The ability to deploy a renewable energy system that is both compact and efficient can dramatically improve the effectiveness of military and police missions, ensuring that energy demands are met without relying on fuel convoys or vulnerable energy infrastructure.
Hybrid Systems for Energy Resilience: While small-scale power plants focused on renewable energy sources can significantly improve energy security, many military and police stations require even greater resilience, particularly in areas with harsh environmental conditions or where energy demand is particularly high. In such cases, hybrid systems that combine multiple energy sources—such as solar, wind, and backup generators—can provide the necessary flexibility and resilience.
A hybrid system can be designed to use renewable energy as the primary source of power, with backup generators or battery storage systems kicking in when the renewable sources are insufficient. For example, during periods of low sunlight or wind, the system could switch to backup generators powered by diesel or gas. On the other hand, during times of excess renewable energy production, the system could store the surplus energy in batteries for later use.
This hybrid approach ensures that the station remains fully operational under all circumstances. Even in situations where weather conditions or high energy demands temporarily impact the renewable energy generation, the station will still have access to power through the backup system, offering continuous support for critical operations.
Integration with Smart Grid and Monitoring Systems: Small-scale power plants for military and police stations can also benefit from integration with smart grid technologies and advanced monitoring systems. These systems allow for real-time monitoring of energy production, consumption, and storage, enabling the optimization of energy use and ensuring that energy flows are efficiently managed. By incorporating smart grid technology, military and police stations can automatically adjust energy usage based on available power supply, prioritizing essential operations when energy is limited and reducing non-critical consumption during peak demand times.
Furthermore, energy management software can provide valuable data and insights into energy usage patterns, helping these facilities make informed decisions about energy consumption and identifying areas for improvement. This data can also help identify when maintenance is needed for renewable energy systems or when the system needs to be adjusted to better match operational requirements.
Economic and Geopolitical Considerations: For military and police stations, reducing dependence on external energy sources has broader economic and geopolitical implications. Fuel and energy imports can be costly, and in times of political instability or conflict, access to fuel supplies can be disrupted. By investing in small-scale renewable energy systems, military and law enforcement agencies can mitigate these risks, ensuring that they remain self-sufficient regardless of external circumstances.
Renewable energy systems also provide an opportunity for cost savings, as they reduce the need for fuel supply contracts, transportation, and other logistics associated with traditional energy sources. This is particularly relevant for operations in conflict zones or remote areas where the cost of transporting fuel is high. By adopting renewable energy, military and police stations can reduce operating expenses while enhancing their operational capabilities and energy independence.
Conclusion: Small-scale power plants for military and police stations represent a forward-thinking solution that enhances energy reliability, operational efficiency, and sustainability. By integrating renewable energy technologies like solar, wind, and biomass, these stations can become more self-sufficient, resilient, and less reliant on external energy sources. Whether in remote locations or on temporary deployments, renewable energy can provide a constant and secure power supply, ensuring that military and law enforcement operations remain effective in all circumstances.
The benefits of small-scale power plants extend beyond energy resilience; they also contribute to environmental sustainability, cost savings, and geopolitical stability. As renewable energy technologies continue to evolve, the potential for small-scale power plants to enhance military and police operations will only grow, helping to meet the demands of modern security challenges while reducing the carbon footprint of these critical facilities.
In further exploring the role of small-scale power plants for military and police stations, it’s essential to consider a few additional factors that can enhance the practicality, scalability, and overall effectiveness of such systems.
Resilience to Natural Disasters: Natural disasters, such as hurricanes, floods, and earthquakes, pose significant risks to energy infrastructure, particularly in vulnerable regions. Military and police stations in disaster-prone areas must have a reliable and resilient energy source that can continue functioning even when external power systems are compromised. Small-scale power plants, especially those using renewable energy sources, offer an effective way to ensure that these stations remain operational during emergency situations.
For instance, during hurricanes or floods, where grid infrastructure can be damaged or taken offline, solar or wind-powered plants can keep essential operations running, such as communication with other units, coordination with emergency responders, and powering critical systems like medical equipment and lighting. In some cases, small-scale plants can be equipped with weather-resistant and disaster-proof components, further improving their ability to function in extreme conditions. By decentralizing energy production and relying on locally available renewable resources, military and police stations can reduce their vulnerability to power disruptions during critical times.
Quick Deployment and Scalability: One of the key advantages of small-scale power plants for military and police stations is their ability to be rapidly deployed in emergency or conflict situations. As mentioned earlier, mobile energy solutions, such as portable solar panels, wind turbines, and backup generators, can be quickly set up to support a temporary military base, police station, or command post.
This capability is especially valuable in situations where energy demands are unpredictable, such as disaster relief efforts, peacekeeping missions, or military operations in unfamiliar or hostile environments. Small-scale plants can also be easily scaled up or down depending on the specific energy needs of the operation. In areas where additional power is required, the capacity of the plant can be increased by adding more renewable energy sources or connecting multiple plants, creating a flexible and adaptable solution.
Enhanced Collaboration and Partnerships: Small-scale power plants for military and police stations also present opportunities for collaboration between different sectors and stakeholders. In some cases, local governments, private contractors, or even non-governmental organizations (NGOs) can play a role in the development and implementation of renewable energy systems. These partnerships can help offset initial installation costs and provide additional expertise in renewable energy technologies.
For example, local authorities might partner with military or police organizations to install a shared small-scale renewable energy system that can supply power to both the military facility and surrounding communities. By collaborating with energy providers or technology manufacturers, these organizations can access specialized equipment, training, and maintenance services, which can reduce operational costs and improve the long-term sustainability of the power plant.
Training and Skill Development: Another important aspect of integrating small-scale power plants into military and police stations is the potential for training and skill development. The personnel operating these power plants can be trained in renewable energy technologies, energy management, and system maintenance. This knowledge can improve their ability to manage and troubleshoot energy systems independently, ensuring the continued reliability of the power supply.
Furthermore, personnel trained in renewable energy technologies can contribute to the development of similar systems in other military or police installations or in local communities. This capacity-building approach not only improves the operational efficiency of individual stations but also promotes wider adoption of renewable energy solutions across the sector.
Energy Independence for Tactical Operations: In tactical operations, such as military engagements, peacekeeping missions, or police actions in remote locations, energy independence is vital. Deploying small-scale power plants in these scenarios ensures that military units or police teams are not reliant on external fuel supplies or vulnerable grid infrastructure.
For instance, when military forces are deployed in hostile or conflict zones, energy security becomes a key strategic advantage. Not having to rely on fuel convoys—often targets for sabotage or attack—can significantly enhance operational effectiveness and reduce logistical risks. By providing portable, renewable energy sources that can operate autonomously, small-scale power plants increase the tactical flexibility of military and police units, enabling them to maintain communication, surveillance, and other essential operations without energy supply interruptions.
Cost-Effectiveness in Long-Term Operations: While the initial investment in a small-scale power plant may be high, the long-term savings in operational costs can be substantial. Renewable energy sources, such as solar and wind, have low maintenance costs and produce little to no ongoing fuel expenditure once installed. This cost savings is particularly advantageous for military and police stations with limited budgets or those operating in remote areas where fuel transportation costs are high.
Furthermore, the growing trend of energy efficiency—such as the use of energy-saving equipment and advanced energy management systems—can further reduce operational costs. By adopting renewable energy sources and improving energy efficiency, military and police stations can allocate more resources toward mission-critical activities, ensuring that budgets are better managed and operational capabilities are enhanced.
Carbon Footprint Reduction and Environmental Stewardship: In addition to the economic benefits, adopting small-scale power plants for military and police stations aligns with environmental stewardship goals. Governments worldwide are setting ambitious carbon reduction targets, and military and law enforcement agencies are increasingly being held accountable for reducing their environmental impact.
By adopting renewable energy solutions, military and police stations can significantly lower their carbon footprints, contribute to national emissions reduction goals, and demonstrate a commitment to sustainability. This not only helps in addressing global environmental concerns but also sets a positive example for other sectors to follow, encouraging a wider adoption of clean energy technologies.
Conclusion: Small-scale power plants for military and police stations are an innovative and sustainable solution that enhances energy security, operational efficiency, and environmental responsibility. With a focus on renewable energy sources such as solar, wind, and biomass, these systems provide critical infrastructure resilience, ensuring that military and police operations remain uninterrupted even in remote or off-grid locations.
The advantages of small-scale power plants extend beyond energy independence—they also improve cost-effectiveness, enhance tactical capabilities, and contribute to broader sustainability and environmental goals. By incorporating these systems, military and police agencies can improve operational readiness, reduce logistical risks, and play a vital role in promoting the global transition toward renewable energy.
As renewable energy technologies continue to advance, the potential for small-scale power plants to transform military and law enforcement operations will only increase. Whether used for stationary facilities or mobile deployments, these systems are poised to become an integral part of modern military and police operations, ensuring that energy needs are met in a reliable, cost-effective, and environmentally responsible manner.
Building on the previously discussed benefits of small-scale power plants for military and police stations, there are several additional considerations and emerging trends that could further enhance the utility and adoption of these systems.
Integration with Hybrid Energy Solutions: As energy needs for military and police stations become more complex, the integration of hybrid energy systems could significantly improve the operational efficiency and flexibility of these small-scale power plants. A hybrid energy solution typically involves combining multiple energy sources, such as solar, wind, batteries, and backup generators, to meet the varied and dynamic energy demands of military and police facilities.
For example, during periods of abundant renewable energy generation, such as sunny days or windy conditions, the system could prioritize renewable energy for immediate consumption while storing excess energy in batteries. During periods when renewable energy generation is low, such as cloudy days or at night, the system could seamlessly switch to backup power sources such as diesel generators or gas turbines. This hybrid approach allows military and police stations to reduce their reliance on non-renewable energy while ensuring that critical operations continue even when renewable resources are insufficient.
Hybrid energy solutions can also help reduce fuel consumption, as the backup generators only operate when absolutely necessary, minimizing wear and tear. Additionally, hybrid systems can be equipped with smart energy management technologies to optimize energy flow between various components, ensuring the most efficient use of available resources. This approach increases the resilience of energy supply in military and police operations, which is particularly important in volatile or unpredictable environments.
Smart Grid Integration for Better Energy Management: Incorporating smart grid technology into small-scale power plants for military and police stations is another promising development. Smart grids allow for real-time monitoring, control, and optimization of energy generation and distribution. With the help of advanced sensors and communication technologies, smart grids enable operators to detect fluctuations in energy demand, anticipate potential disruptions, and make adjustments to maintain a stable and efficient energy supply.
For military and police stations, this can be particularly advantageous in managing power use during peak demand times, such as during large-scale operations or emergencies. Smart grids can prioritize critical systems, such as communications, lighting, and security systems, ensuring they remain powered even during energy shortages or system disruptions. By optimizing energy distribution, smart grids reduce waste and help lower overall energy consumption, contributing to long-term cost savings and reducing the environmental impact of operations.
Moreover, the integration of smart grids with renewable energy sources like solar or wind ensures that power generation is more predictable and reliable. By anticipating energy demand and adjusting power generation accordingly, smart grids enhance the overall efficiency and autonomy of small-scale power plants, allowing military and police stations to operate with greater energy independence and less reliance on backup power.
Customization and Scalability for Diverse Operational Needs: Military and police stations come in various sizes and have different energy requirements depending on their specific operations. For example, a forward operating base may have much higher energy demands than a stationary police station. One of the key benefits of small-scale power plants is their scalability and the ability to customize systems to meet the unique needs of each facility.
For smaller stations or temporary installations, smaller renewable energy systems, such as compact solar panels or portable wind turbines, may be sufficient to meet power needs. These systems can be easily deployed and scaled up or down based on changing energy requirements. For larger or more permanent facilities, more robust systems combining multiple energy sources, such as a combination of solar, wind, and biomass, may be necessary to ensure consistent power generation.
The modularity of small-scale power plants makes them versatile and adaptable to different operational contexts. Additionally, renewable energy systems can be integrated with energy storage solutions like batteries, which allow military and police stations to store excess energy for use during periods of high demand or when renewable resources are not available. The combination of these systems provides a reliable and flexible energy solution that can evolve with the changing needs of military and police operations.
Cost-Benefit Analysis and Long-Term Financial Sustainability: In many cases, military and police agencies face budget constraints that can affect their ability to invest in renewable energy solutions. While the initial capital costs of installing small-scale power plants may seem high, the long-term financial benefits should not be overlooked. With energy costs often being one of the largest operational expenses for military and police stations, switching to renewable energy can lead to significant savings over time.
The financial sustainability of small-scale power plants can be evaluated through a cost-benefit analysis that compares the installation and maintenance costs with the ongoing savings on fuel and electricity. As mentioned earlier, renewable energy systems, particularly those utilizing solar, wind, or biomass, have lower operational costs compared to traditional fossil fuel-based energy sources. Additionally, renewable energy systems require minimal fuel expenditures once installed, which results in reduced reliance on expensive fuel supply chains.
In regions with frequent fuel price volatility or challenges in fuel supply, small-scale power plants can shield military and police stations from the uncertainty of fuel markets, providing more predictable energy costs. Over time, the savings generated by renewable energy systems can offset the upfront costs of installation, making them a cost-effective solution for long-term operations. Additionally, tax incentives or government subsidies for renewable energy adoption may help reduce initial capital expenditures, further improving the financial feasibility of small-scale power plants.
Community Engagement and Local Benefits: The adoption of small-scale power plants for military and police stations can also have positive impacts on surrounding communities. In some cases, military and police stations with renewable energy systems can share excess power with nearby civilian communities, particularly in remote or underserved areas. This can provide a dual benefit—helping to meet local energy demands while also fostering positive relationships between the military, police, and local residents.
For example, a solar-powered military base located in a rural area could supply power to nearby homes or community centers, reducing the community’s reliance on expensive and less reliable grid electricity. This collaborative approach can build goodwill with local populations and create more sustainable, self-reliant energy solutions for both military operations and civilian life.
Additionally, the establishment of small-scale power plants can support local economic development by creating jobs in the renewable energy sector. Local contractors, technicians, and workers can be trained to install, maintain, and operate renewable energy systems, contributing to the local economy and helping build local expertise in sustainable energy solutions.
Adapting to Future Technological Advances: As renewable energy technology continues to evolve, small-scale power plants for military and police stations are likely to become even more efficient, cost-effective, and adaptable. Emerging technologies, such as advanced energy storage systems, more efficient solar panels, and next-generation wind turbines, will further enhance the performance of small-scale renewable energy systems.
For example, improvements in battery storage technology can increase the amount of energy that can be stored and used during times of low renewable energy generation. More efficient solar panels can provide higher energy yields from smaller installations, while the development of lightweight and portable wind turbines may expand the potential applications of small-scale power plants, making them even more suitable for mobile or temporary deployments.
By staying ahead of technological trends and continuously adapting renewable energy systems to emerging innovations, military and police stations can ensure that their energy infrastructure remains cutting-edge, efficient, and capable of meeting evolving operational needs.
Conclusion: The integration of small-scale power plants for military and police stations is an innovative and forward-thinking approach to energy management that offers a host of operational, environmental, and economic benefits. By utilizing renewable energy sources, these power plants enhance energy resilience, reduce reliance on external fuel supplies, and contribute to sustainability goals. Whether deployed in remote locations, off-grid environments, or during tactical operations, small-scale power plants ensure that military and police stations have a reliable, independent, and cost-effective power supply.
As the technologies supporting these systems continue to evolve, the potential for small-scale renewable energy solutions to revolutionize military and police operations will only increase. Hybrid energy solutions, smart grid technologies, and advancements in energy storage and efficiency will further improve the adaptability, scalability, and cost-effectiveness of small-scale power plants, ensuring that military and police stations are well-equipped to meet the energy demands of the future. By embracing these technologies, military and police agencies can improve operational readiness, reduce energy costs, and play a pivotal role in advancing the global transition to renewable energy.
Continuing with the exploration of small-scale power plants for military and police stations, there are several more emerging trends, benefits, and challenges that need to be addressed to ensure these systems’ ongoing success in military and law enforcement operations.
Energy Security and Geopolitical Considerations: Energy security is a critical concern for military and police operations, especially in conflict zones or politically unstable regions. Dependence on centralized power grids and external fuel supplies can leave military and police stations vulnerable to sabotage, attacks, or blockades. Small-scale, renewable energy power plants provide an effective solution by reducing dependency on external energy sources and ensuring that these stations have a reliable power supply, even in the face of political or security risks.
In conflict zones, for instance, military bases or police stations can use locally generated renewable energy to maintain operations and avoid reliance on fuel convoys that can be targeted or disrupted. This can also reduce the environmental footprint of military and law enforcement activities, as well as mitigate the impact of energy supply disruptions due to geopolitical instability. In addition, using renewable energy helps to reduce logistical burdens related to fuel transport, which can be costly, vulnerable to theft, and a logistical challenge in remote areas.
In areas where energy supply chains are heavily influenced by political tensions, local renewable energy solutions can empower military and police stations to function independently, reducing their vulnerability to international energy politics. These renewable solutions can serve as a way to enhance operational autonomy and protect strategic assets from external pressures.
Improved Operational Efficiency through Microgrids: Microgrids are localized energy systems capable of operating independently from the main grid and are an excellent fit for small-scale power plants deployed at military or police stations. These systems can be integrated into renewable energy plants to ensure reliable power even when the broader grid is unavailable or unreliable.
Microgrids allow military and police facilities to optimize their energy consumption, manage energy resources in a more efficient manner, and ensure critical operations are always powered. They enable efficient energy distribution within the facility, prioritizing power for mission-critical systems, such as communications, security, medical devices, and other essential equipment, while reducing power usage for non-essential systems.
Additionally, microgrids can be connected to larger grids when available, allowing military and police stations to contribute energy back to the grid, further improving the economic viability of the system. These systems can also enhance grid resilience by decentralizing energy production and reducing the strain on central power systems, which can be particularly beneficial in regions where grid infrastructure is prone to failures or interruptions.
Robust Backup Systems for Emergency Situations: While small-scale renewable energy systems offer substantial benefits, it is important to address their intermittency—renewable sources like wind and solar can be unpredictable depending on weather conditions and time of day. This makes the integration of robust backup power systems crucial for military and police stations.
Backup power systems, such as battery storage or supplementary diesel generators, ensure that these stations can continue operating even when renewable energy sources are insufficient. For instance, battery storage systems can store excess energy generated during the day and discharge it during the night or when there is insufficient renewable generation. This ensures the continuity of critical operations without disruption. Alternatively, diesel generators can serve as a secondary power source when energy demand exceeds the capacity of renewable systems or during long periods of low generation from renewable sources.
The key to optimizing these backup systems is creating a well-designed energy management strategy that balances the use of renewable energy and backup resources while minimizing fuel consumption and operational costs. This approach not only ensures that power is always available when needed but also minimizes the environmental impact of relying on non-renewable backup systems.
Mobile and Tactical Energy Solutions for Deployed Forces: Mobile energy solutions are a significant consideration for military and police forces deployed in the field, especially in combat or disaster relief operations. Small-scale power plants can be designed to be portable, providing mobile units with the ability to generate energy on the go. These mobile energy systems can power equipment, vehicles, or even temporary command centers that are set up in remote areas.
For military and law enforcement agencies, tactical energy solutions can be critical for operational success. For example, lightweight solar panels can be rapidly deployed to power communication systems, radar equipment, and other essential electronics used during tactical operations. Similarly, portable wind turbines can be set up in areas with high wind potential to generate energy in real-time, reducing dependence on fuel supplies or battery-operated equipment.
These mobile solutions are invaluable in forward-operating bases or temporary installations, where traditional grid power is not available. Furthermore, they can enhance operational flexibility by providing energy independence in dynamic and ever-changing environments. Mobile renewable energy solutions help military and police units focus on their primary missions while minimizing energy-related logistical challenges.
Energy Efficiency and Smart Technology Integration: To maximize the performance of small-scale power plants, the integration of smart technologies can improve energy efficiency and ensure optimized power management. Advanced technologies such as artificial intelligence (AI) and the Internet of Things (IoT) can play a crucial role in this.
AI-powered energy management systems can predict energy usage patterns and adjust power generation or consumption in real-time. For example, AI can forecast the energy requirements of different parts of the facility based on operational schedules, weather forecasts, or other environmental factors. This allows the power plant to proactively adjust its energy output, balancing renewable generation with backup power systems to ensure stable, uninterrupted energy supply.
IoT-based sensors and smart meters can provide detailed data on energy consumption at a granular level, allowing operators to track and control the use of power throughout the facility. These sensors can detect issues like energy inefficiency, leaks, or equipment malfunctions, enabling quick interventions and reducing energy waste. Over time, the data collected through IoT systems can be analyzed to identify areas for energy optimization and future improvements in system design and operation.
Environmental and Social Impact: In addition to the operational benefits of small-scale power plants, military and police stations must also consider the broader environmental and social impact of their energy consumption. As organizations that operate on behalf of national or international security, military and police stations are increasingly expected to set an example for sustainable practices, particularly as global concerns about climate change intensify.
By adopting renewable energy systems, military and police stations can significantly reduce their carbon footprint, contribute to international efforts to combat climate change, and improve their public image. Reducing greenhouse gas emissions through the use of solar, wind, and biomass energy also supports the broader transition toward sustainable energy solutions in society.
Furthermore, renewable energy systems have positive social impacts by creating green jobs and promoting energy independence. As renewable energy systems become more widely adopted, the skills and expertise needed to design, install, and maintain these systems will lead to job creation, training opportunities, and economic growth in local communities. Military and police agencies can play a key role in driving this transition and fostering long-term sustainability within their operations and surrounding areas.
Strategic Partnerships and Knowledge Transfer: Lastly, small-scale power plants present an opportunity for strategic partnerships between military and law enforcement agencies, private companies, and local communities. Through partnerships with renewable energy firms, local governments, and technology providers, military and police stations can access the latest innovations in renewable energy, financing models, and operational best practices.
Additionally, these partnerships can lead to knowledge transfer, where military and police personnel gain expertise in renewable energy technologies and energy management practices. This knowledge can be applied to other projects within the defense or law enforcement sectors, as well as in broader efforts to promote clean energy solutions in civilian applications.
Collaborations with non-governmental organizations (NGOs) or international agencies can further extend the reach of these initiatives, particularly in developing countries or areas with limited access to reliable energy. By sharing knowledge and resources, military and police stations can contribute to the global transition to renewable energy while strengthening their own operational resilience and efficiency.
Conclusion: The integration of small-scale power plants for military and police stations is an essential step toward ensuring energy security, operational efficiency, and sustainability. From tactical deployments in remote areas to long-term energy independence, renewable energy systems offer a diverse range of benefits for military and law enforcement operations.
By leveraging hybrid systems, microgrids, smart technologies, and mobile energy solutions, military and police agencies can improve their operational flexibility, reduce costs, and contribute to environmental goals. These small-scale renewable energy solutions empower stations to remain operational in challenging conditions, enhance their resilience to external threats, and provide critical services during emergencies or crises.
As technology continues to evolve, small-scale power plants will play an increasingly significant role in ensuring that military and police operations are sustainable, self-sufficient, and effective. The future of energy for military and law enforcement agencies lies in harnessing the power of renewable sources to drive their operations forward in a clean, efficient, and secure manner.
Small Scale Power Plant for Breweries
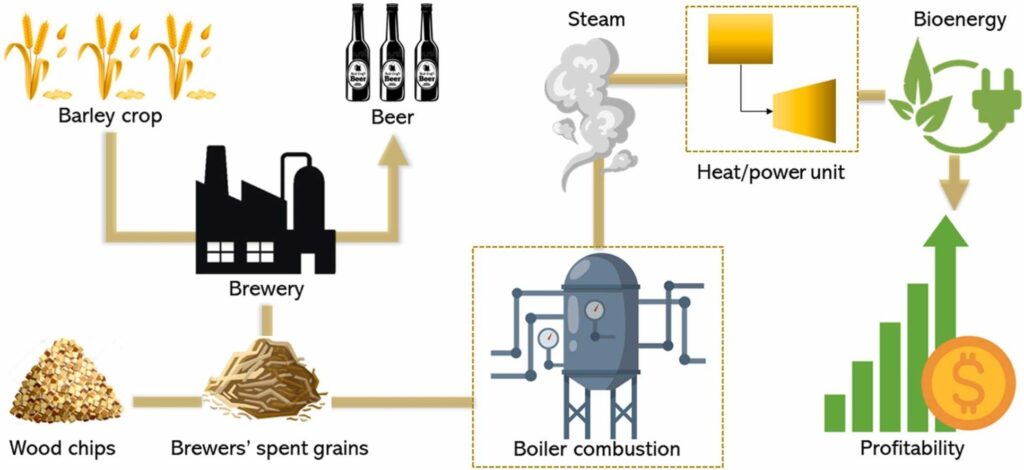
Small-scale power plants for breweries present a unique opportunity to enhance energy efficiency, reduce operating costs, and promote sustainability in an industry that requires significant energy for production processes. Breweries rely heavily on energy for activities such as heating water, boiling wort, fermentation, refrigeration, and packaging. Given the high energy demand, transitioning to small-scale renewable energy systems can help breweries reduce their reliance on external power sources, lower their carbon footprint, and improve their overall environmental and financial sustainability.
Energy Requirements and Challenges in Breweries
Breweries are energy-intensive operations due to the various stages involved in brewing beer. The most energy-consuming processes in a brewery include:
- Heating and Boiling: A significant amount of energy is required for mashing (heating the malted barley), boiling the wort (the liquid extracted from the mash), and pasteurizing beer. These processes typically use heat, often provided by gas or electricity.
- Cooling and Refrigeration: After boiling, the wort must be cooled rapidly to the desired fermentation temperature, which requires substantial energy, especially in larger breweries. Refrigeration is also necessary to maintain the appropriate temperatures during fermentation and storage.
- Packaging and Transportation: The packaging process (including bottling, canning, and labeling) also demands considerable energy for machinery and conveyor belts. Additionally, energy is required for logistics, which can be addressed by utilizing renewable energy for electric vehicles and transportation systems.
Breweries are often located in regions with reliable renewable resources such as solar, wind, or biomass. Therefore, utilizing small-scale power plants for energy generation, especially in combination with energy storage and efficient energy management systems, can help mitigate some of these challenges.
Advantages of Small-Scale Power Plants for Breweries
1. Energy Independence and Reliability: Small-scale power plants, such as those using solar panels, wind turbines, or biomass generators, can provide breweries with a reliable, on-site source of power. This is especially important for breweries located in remote areas or regions with unreliable power grids, where the risk of power outages can disrupt production. By generating their own electricity, breweries can reduce their dependence on the grid and avoid the cost and uncertainty of fluctuating energy prices. Additionally, using on-site power generation ensures consistent power supply, which is critical for maintaining operations, particularly in energy-sensitive brewing processes like fermentation and cooling.
2. Cost Savings and Financial Benefits: The initial investment in a small-scale power plant can be offset by long-term energy savings. Breweries typically have high energy costs, so reducing these expenses over time can have a significant impact on the bottom line. By producing their own electricity, breweries can minimize reliance on expensive grid power and the associated costs. Furthermore, surplus energy generated during periods of low demand (e.g., sunny days for solar power) can be stored in batteries or sold back to the grid, creating an additional revenue stream. Government incentives, tax credits, and grants for renewable energy adoption can further reduce the upfront investment and accelerate the financial payback period.
3. Carbon Footprint Reduction and Environmental Sustainability: With the growing consumer demand for environmentally conscious products, many breweries are turning to renewable energy sources to reduce their environmental impact. By utilizing solar, wind, or biomass energy to power operations, breweries can significantly lower their carbon emissions, contributing to sustainability goals and reducing their overall environmental footprint. In addition to reducing greenhouse gas emissions, breweries can also implement water conservation measures and waste-to-energy systems to further improve their sustainability credentials. Many breweries already produce organic waste, such as spent grains, which can be used as feedstock for biomass power generation, further integrating sustainability into their operations.
4. Use of Waste Heat and Biomass: One of the benefits of small-scale power plants for breweries is the potential for waste heat recovery and the use of organic waste in biomass power systems. Brewing processes, especially the boiling stage, generate significant amounts of waste heat. This waste heat can be captured and used for other heating processes, such as mashing, or to pre-heat water, reducing the need for additional energy input.
Similarly, spent grains, which are a byproduct of the brewing process, can be repurposed as biomass for power generation. These grains are typically rich in organic material, making them an ideal source for biomass energy. By converting this waste into energy, breweries can lower both their waste disposal costs and their reliance on external energy sources, promoting a circular economy and reducing waste.
5. Energy Storage for Consistent Supply: Since renewable energy sources like solar and wind are intermittent, energy storage systems such as batteries are an essential component of a small-scale power plant for breweries. These systems store excess energy generated during periods of high renewable energy production and provide power during periods when renewable energy generation is low. This ensures that breweries have a continuous, stable energy supply even when the sun isn’t shining or the wind isn’t blowing.
Battery storage also allows breweries to shift energy consumption to times when renewable energy is plentiful, optimizing the use of clean energy and minimizing reliance on backup generators or grid power. This contributes to both cost savings and environmental sustainability.
6. Community Engagement and Local Economic Benefits: By adopting renewable energy systems, breweries can also positively impact local communities. For example, solar or wind projects often create jobs in installation, maintenance, and operations, boosting the local economy. Additionally, as breweries become energy self-sufficient, they can contribute excess energy back to the local grid, supporting the energy needs of nearby communities. By becoming a local energy generator, breweries can strengthen their ties with the community and contribute to regional energy security.
Moreover, if a brewery is located in a rural or remote area, a small-scale power plant can be a key part of local infrastructure development. The brewery can serve as a model for other businesses and industries in the area, showing how renewable energy can be utilized effectively to reduce costs and promote sustainability.
Key Considerations for Small-Scale Power Plants in Breweries
1. Initial Capital Investment: The upfront cost of setting up a small-scale power plant can be substantial, and breweries must consider their ability to finance the project. While the long-term savings are significant, it may take several years for a brewery to recover the initial investment, especially if the facility is smaller or located in a region with less renewable energy potential. However, the availability of financial incentives, subsidies, and financing options can help mitigate these costs and make the project more feasible.
2. Integration with Existing Infrastructure: The integration of small-scale power plants with existing brewery infrastructure is an important consideration. Breweries typically have complex energy requirements, and the power generation system must be designed to meet these needs efficiently. For example, a brewery may require a combination of electricity for machinery and heat for various processes. A hybrid system that integrates both electricity generation (e.g., solar or wind) and heat recovery (e.g., biomass or waste heat) may be necessary to meet these dual energy demands. Properly sizing the power plant and ensuring that it can be seamlessly integrated with existing systems is crucial for maximizing energy efficiency and minimizing costs.
3. Maintenance and Technical Expertise: Maintaining a small-scale power plant requires technical expertise, particularly for systems that involve renewable energy sources like solar panels, wind turbines, or biomass systems. Breweries may need to invest in training for staff or contract with external service providers to ensure the plant is operating at optimal efficiency. The complexity of the technology may vary depending on the type of energy generation being used, so it is important for breweries to assess the level of expertise required for operation and maintenance.
4. Regulatory Compliance: Breweries must also be aware of local regulations related to energy generation, waste management, and environmental impact. Different regions may have varying regulations for renewable energy systems, especially when it comes to power generation, emissions, and waste disposal. Breweries will need to navigate these regulations and ensure that their small-scale power plants are in compliance with all local laws and environmental standards.
5. Long-Term Viability and Technological Advancements: As renewable energy technologies continue to evolve, breweries should also consider the long-term viability of their small-scale power plant. Technological advancements, such as improvements in solar panel efficiency, wind turbine designs, or energy storage solutions, can further enhance the performance of renewable energy systems. It is important for breweries to evaluate future technology trends and potential upgrades when planning their energy systems to ensure they remain at the forefront of energy innovation.
Conclusion
Small-scale power plants for breweries offer a range of benefits, from cost savings and energy independence to environmental sustainability and community engagement. By integrating renewable energy solutions such as solar, wind, or biomass systems, breweries can reduce their reliance on external power sources, cut operating costs, and contribute to their sustainability goals. Through smart energy management, hybrid systems, and energy storage, breweries can optimize energy use while ensuring consistent and reliable power for their operations.
The transition to small-scale renewable energy systems in the brewing industry not only benefits the breweries themselves but also contributes to local economies, strengthens communities, and supports global efforts to reduce carbon emissions. As the renewable energy landscape continues to evolve, breweries will have increasing opportunities to leverage new technologies, further enhancing their operational efficiency and sustainability.
Continuing with small-scale power plants for breweries, there are additional advantages and considerations that breweries should evaluate when implementing renewable energy systems.
Integration of Local Resources and Circular Economy: One of the most compelling aspects of small-scale power plants for breweries is the opportunity to integrate local resources into the energy generation process. Many breweries are located in regions where they can access locally sourced materials, such as agricultural waste or forestry products, for use in biomass systems. By using local biomass, breweries can not only reduce their reliance on external energy sources but also contribute to the local economy by creating a market for these materials.
Breweries can establish a circular economy where waste products from the brewing process, such as spent grains, hops, and yeast, are used to generate energy. In this way, breweries effectively “close the loop” on their resource usage, generating power from the very waste products they produce. This approach can significantly reduce disposal costs and transform waste into valuable resources, creating both financial and environmental benefits. Additionally, the use of renewable biomass can provide a continuous and reliable energy source, especially in cases where solar or wind energy may be less consistent.
Supporting Carbon Neutrality and Eco-friendly Branding: As sustainability becomes a growing focus for consumers, many breweries are using renewable energy as part of their eco-friendly branding. Being able to market a brewery’s carbon neutrality or low carbon footprint can resonate strongly with environmentally conscious consumers. By utilizing small-scale renewable energy plants, breweries can reduce their emissions and strengthen their brand’s appeal to an eco-conscious market segment. The adoption of solar, wind, and biomass energy further allows breweries to position themselves as leaders in sustainability within the food and beverage industry.
With increasing demand for carbon-neutral products, breweries that embrace clean energy production can potentially attract new customers, particularly as sustainability becomes an essential consideration for consumers in making purchasing decisions. A brewery’s commitment to reducing its carbon emissions and overall environmental impact can become a selling point, distinguishing it in an increasingly competitive market.
Energy Flexibility and Resilience: Energy flexibility and resilience are especially crucial for breweries that operate in areas with unreliable power grids or frequent power interruptions. For breweries that rely heavily on energy for brewing, refrigeration, and packaging, a loss of power can significantly disrupt production schedules and lead to financial losses. Small-scale power plants, including those with integrated energy storage systems, can provide backup power during grid outages, ensuring that critical systems remain operational.
Furthermore, renewable energy systems such as solar panels and wind turbines offer the advantage of scalability, enabling breweries to adjust their energy generation capacity based on operational growth or changes in energy consumption patterns. For example, a brewery might start with a small solar array to power basic operations, and as demand grows or energy requirements increase, it can expand its system to meet these needs. This flexibility allows breweries to align their energy infrastructure with their growth trajectory and avoid over-investment in energy systems before they are required.
Reducing Operating Costs and Improving Profit Margins: Breweries can significantly lower operating costs by generating their own electricity and reducing the need for purchased power. This cost savings can have a substantial impact on a brewery’s profit margins. While the upfront capital investment for a small-scale power plant can be significant, the long-term cost savings typically make the system a worthwhile investment, particularly for larger breweries with high energy consumption.
In addition to lower energy costs, renewable energy systems offer predictable pricing compared to conventional energy sources. Energy markets can be volatile, with price fluctuations due to factors like fuel supply and demand, geopolitical tensions, or natural disasters. By investing in renewable energy systems, breweries can lock in their energy prices and avoid being subject to the rising costs of fossil fuels. The financial predictability provided by renewable energy sources can enhance long-term financial planning and help breweries manage their cash flow more effectively.
Opportunities for Innovation and Collaborative Initiatives: Small-scale power plants also provide opportunities for breweries to innovate with new technologies and collaborate with other local businesses and communities. Breweries can explore partnerships with local farmers, energy providers, or waste management companies to create synergies that improve energy efficiency and reduce costs. For example, a brewery might collaborate with a local agricultural farm to use organic waste (such as crop residues) for biomass energy production, reducing waste and providing a reliable energy source.
Moreover, by participating in larger regional or national renewable energy initiatives, breweries can support the transition to a sustainable energy future while benefiting from shared resources and knowledge. Joining cooperative energy projects, such as community solar programs or renewable energy co-ops, can help breweries access new funding opportunities, share best practices, and collectively contribute to the renewable energy transition.
Expanding Consumer Engagement through Sustainability: As sustainability becomes a core focus for many consumers, breweries can use their renewable energy adoption as a tool to engage with their customer base. Breweries can educate consumers about the benefits of clean energy through marketing campaigns, product labels, and community outreach. Sharing information about the brewery’s renewable energy efforts, such as producing beer using solar or wind power, allows consumers to make more informed purchasing decisions and helps to build customer loyalty.
In addition to offering a product, breweries that adopt sustainable practices can foster stronger connections with their customers by aligning their values with those of their target market. Offering eco-friendly products or promoting sustainability as part of the brewery’s mission not only enhances customer engagement but also builds a sense of community and responsibility around shared values of environmental stewardship.
Remote and Mobile Brewery Applications: In some cases, small-scale power plants can be used in mobile brewery setups or remote brewery locations. For breweries operating in remote areas or on a smaller scale (such as microbreweries or pop-up operations), access to the grid may not be feasible or affordable. In these situations, portable solar panels, wind turbines, or even small-scale biogas generators can be used to provide power for brewing operations.
For example, a microbrewery located in a remote area may use a combination of solar power and a small biomass system to generate electricity and heat. These mobile energy solutions offer flexibility, enabling breweries to operate independently of the grid while still maintaining high-quality production. Additionally, mobile energy systems can be scaled based on demand and easily relocated if the brewery expands to new locations or faces operational changes.
Smart Metering and Energy Management: Implementing energy management systems is crucial to the effective operation of small-scale power plants in breweries. Smart meters, sensors, and automated control systems allow breweries to monitor their energy usage and optimize consumption across different processes. These systems help breweries track energy performance, identify inefficiencies, and make data-driven decisions to enhance energy management.
Through real-time data collection, breweries can assess the performance of their renewable energy systems and adjust energy usage accordingly. For example, if the brewery produces more energy than needed during daylight hours with solar panels, the system can store the excess energy or redirect it to non-essential equipment during off-peak periods. This type of smart energy management ensures that breweries maximize the effectiveness of their renewable energy systems and minimize waste.
Conclusion:
Small-scale power plants for breweries provide numerous benefits, from energy cost savings to environmental sustainability, and can help breweries increase their operational resilience, reduce their carbon footprint, and engage more meaningfully with eco-conscious consumers. The integration of renewable energy systems, whether through solar, wind, or biomass power, enables breweries to take control of their energy production while minimizing dependence on external power sources.
As breweries continue to grow and demand for sustainable production increases, small-scale power plants offer an innovative solution to meet both operational and environmental goals. With the added benefits of energy independence, reduced operating costs, and improved brand reputation, small-scale power plants represent a valuable investment for breweries aiming to maintain competitiveness in an evolving market that increasingly values sustainability and green practices.
Ultimately, small-scale renewable energy systems empower breweries to optimize their energy consumption, reduce environmental impact, and support the transition to a more sustainable future. By adopting these technologies, breweries can not only improve their bottom line but also contribute to the larger effort to combat climate change and promote clean energy solutions on a global scale.
As breweries explore and implement small-scale power plants, there are several additional aspects to consider that can further enhance the efficiency and sustainability of their energy systems. By incorporating the latest technologies, integrating smart solutions, and creating synergies with other industries, breweries can fully capitalize on the benefits of renewable energy.
Leveraging Advanced Technology for Greater Efficiency: Advancements in renewable energy technologies can significantly increase the efficiency and cost-effectiveness of small-scale power plants for breweries. Solar photovoltaic (PV) technology has evolved, with newer, more efficient panels available that capture more sunlight and generate greater amounts of electricity. Additionally, the development of thin-film solar panels and bifacial solar cells—capable of capturing sunlight from both sides of the panel—can provide increased efficiency, especially in areas with limited space or low sunlight.
Wind turbine technology has also improved, with smaller and more efficient models designed for residential or industrial use. These smaller turbines are perfect for breweries located in areas with adequate wind resources. They offer a viable, low-maintenance, and cost-effective energy solution for supplementing power generation alongside other renewable systems.
For biomass energy, the development of advanced conversion technologies, such as anaerobic digesters and pyrolysis systems, has made it easier for breweries to convert organic waste into high-quality renewable energy. These technologies can further reduce the environmental impact by turning brewery waste into usable heat or electricity, simultaneously reducing waste disposal costs and increasing sustainability.
The growing sophistication of energy storage systems also presents significant opportunities for breweries. Innovations in battery technology, including lithium-ion and flow batteries, have drastically improved energy storage efficiency, enabling breweries to store excess energy generated during peak production times (e.g., sunny days) for use during periods of low renewable generation. This results in a more reliable, round-the-clock energy supply and optimizes the brewery’s renewable energy utilization.
Data-Driven Decision Making with Energy Analytics: Energy analytics and artificial intelligence (AI) can be integrated into the operation of small-scale power plants for breweries to further optimize energy production and consumption. Advanced sensors and monitoring systems can track and analyze real-time energy use across all brewing processes, identifying patterns, inefficiencies, and potential improvements.
For instance, AI algorithms can predict peak energy usage periods, enabling breweries to adjust operations in advance to minimize energy consumption during high-demand times. These systems can also be integrated with weather forecasting tools to predict renewable energy production based on solar radiation or wind speed, allowing breweries to plan and adjust their energy consumption patterns. By leveraging data-driven insights, breweries can not only reduce energy costs but also enhance operational efficiency and minimize energy waste.
Additionally, these energy management systems can be tied into the brewery’s broader sustainability goals, tracking the brewery’s energy consumption, emissions reductions, and waste generation. Such analytics tools can help breweries communicate their sustainability progress with stakeholders and customers, reinforcing their commitment to environmental stewardship.
Hybrid Systems for Energy Diversification: To ensure a steady and reliable energy supply, breweries can adopt hybrid systems that combine multiple renewable energy sources. For example, a brewery located in a region with both strong solar radiation and consistent wind patterns might install both solar panels and small wind turbines to optimize energy production throughout the year.
By integrating a variety of energy sources, breweries can mitigate the variability of any single source. For instance, wind energy may be abundant in the winter, while solar power could be more effective during summer months. Combining multiple sources allows breweries to generate more consistent power and maximize their renewable energy output. Hybrid systems can also provide operational flexibility, allowing breweries to switch between different power generation methods based on real-time energy production and consumption needs.
Moreover, hybrid systems can complement the brewery’s existing infrastructure, reducing the need for backup generators or additional reliance on grid electricity. With the right combination of energy sources and storage solutions, breweries can achieve near-complete energy self-sufficiency, ensuring that they have the power they need without relying on fossil fuels.
Microgrids for Increased Energy Security: Some breweries may find it advantageous to integrate their small-scale power plant into a microgrid, especially if the brewery is located in a remote or off-grid location. A microgrid is a localized energy system that can operate independently or in conjunction with the main grid. By creating a self-sustaining microgrid, breweries can further reduce their reliance on external energy sources, increase energy resilience, and manage their energy production and consumption in a more controlled and efficient manner.
Microgrids allow breweries to integrate various forms of renewable energy generation, including solar, wind, and biomass, along with energy storage systems, into a single, cohesive network. The brewery can then manage energy distribution across the system, optimizing energy flow based on demand and supply, and ensuring continuous operations even during grid outages or power disruptions. For breweries in remote locations, a microgrid can provide a secure, reliable, and cost-effective power solution.
Microgrids also offer the potential for community collaboration. In some cases, a brewery may join forces with neighboring businesses or residential areas to form a local energy network, sharing renewable energy resources and creating a more resilient energy system. Such community-based microgrids can lower costs, increase energy security, and encourage local energy independence.
Partnerships with Research and Development Initiatives: Collaborating with universities, research institutions, or energy innovation hubs can help breweries stay at the forefront of renewable energy technology advancements. Breweries can work with R&D institutions to pilot new energy solutions, test cutting-edge technologies, or participate in research projects aimed at improving the efficiency and sustainability of small-scale power plants.
These partnerships provide breweries with access to the latest innovations, often at reduced costs or with the opportunity to receive funding or grants. For example, breweries could collaborate on projects that explore the integration of new materials for solar panels, develop more efficient energy storage technologies, or experiment with innovative waste-to-energy systems. Being part of these research initiatives also allows breweries to gain early access to emerging technologies, which can provide a competitive advantage in the market.
Engaging in Circular Economy Initiatives: Breweries can extend their renewable energy initiatives by participating in broader circular economy practices. For example, the heat generated by a brewery’s small-scale power plant could be used to support nearby facilities or communities, providing heating or hot water. Similarly, the organic waste generated by the brewery can be used to power local farms, greenhouses, or other agricultural operations. This concept of resource sharing can help breweries further reduce their environmental impact and contribute to regional sustainability.
Breweries may also explore opportunities for collaborating with other industries to create energy synergies. For instance, a brewery might partner with a nearby distillery, food processing plant, or agricultural producer to share energy or use each other’s waste products. In these kinds of partnerships, each business can benefit from the waste-to-energy opportunities provided by the others, further driving efficiency and reducing costs.
Customer Engagement and Transparency: With increasing interest in sustainability, breweries can use renewable energy as part of a larger strategy to engage their customers. Transparency about energy production—such as sharing how much of the beer is brewed using solar or wind power—can be a powerful marketing tool. Breweries can showcase their renewable energy initiatives on product labels, websites, or even through special events or tours.
This level of transparency builds trust with consumers, who are more likely to support companies that prioritize environmental responsibility. Engaging customers with the story of the brewery’s energy generation not only highlights the commitment to sustainability but also invites consumers to be part of a collective effort to protect the environment.
Scalability for Future Growth: One of the key advantages of small-scale power plants is their scalability. As a brewery grows, it can expand its energy systems to meet increasing demand. This scalability ensures that the brewery’s energy infrastructure can evolve alongside its operations, allowing for a smooth transition as production increases.
For example, a small brewery may begin with a solar array that powers its lighting and minor equipment. As the brewery expands, it can add additional solar panels, wind turbines, or even a biomass system to power brewing operations, refrigeration, and other energy-intensive processes. By gradually scaling up the renewable energy system, breweries can align their energy generation with growth while managing the costs and logistical challenges associated with expansion.
Conclusion
The implementation of small-scale power plants in breweries provides numerous benefits, including cost savings, energy independence, sustainability, and a positive environmental impact. By leveraging renewable energy sources such as solar, wind, and biomass, breweries can optimize their energy production, reduce operating costs, and align with the growing demand for eco-friendly products. Advanced technologies, hybrid systems, and energy storage solutions further enhance the effectiveness of these renewable energy systems, ensuring reliable power and greater efficiency.
Small-scale power plants also offer breweries the opportunity to integrate with the broader community, support local economies, and participate in circular economy practices. With the increasing importance of sustainability in the consumer market, breweries that embrace renewable energy can strengthen their brand, reduce their carbon footprint, and build a loyal customer base that values environmental responsibility.
Ultimately, small-scale power plants for breweries represent a win-win opportunity—providing financial savings, increasing operational resilience, and contributing to a more sustainable future. By adopting renewable energy systems, breweries can not only optimize their operations but also play an integral role in the global transition toward clean, renewable energy.
Continuing with small-scale power plants for breweries, we explore additional strategies for enhancing energy systems and building resilience in brewery operations.
Advanced Integration with Energy Networks and Smart Grids: For breweries located in areas with access to a grid, integrating small-scale power plants into a larger energy network can bring added benefits. Advanced energy systems that work in tandem with local smart grids can enhance efficiency, improve the overall power supply reliability, and allow breweries to share energy with the local grid. In some cases, breweries could sell excess energy generated by their solar, wind, or biomass systems back to the grid, creating a source of revenue or offsetting energy costs.
By integrating renewable energy production into a smart grid, breweries can benefit from dynamic pricing, where energy prices fluctuate based on supply and demand. For instance, when energy demand is low, breweries could sell surplus power at higher rates. Conversely, during peak demand, they could draw energy from the grid at more favorable pricing rates. This integration allows breweries to access additional energy sources when needed while ensuring they maximize the value of their own renewable energy production.
Moreover, smart grids equipped with sensors and communication tools allow for real-time monitoring of energy use and grid conditions. For breweries, this means better control over their energy usage and the ability to respond quickly to changes in energy availability or pricing. Energy storage solutions, like batteries or even thermal storage systems, can also be integrated into this setup, enabling breweries to store excess energy for use during low-production periods or during peak times when grid energy costs are higher.
Collaborating with Energy Providers and Incentive Programs: Breweries can also explore opportunities for collaboration with energy providers, particularly those offering renewable energy solutions or government-sponsored incentive programs. Many countries and regions offer financial incentives or tax breaks to businesses that invest in clean energy technologies, including renewable power generation, energy storage systems, and energy efficiency improvements.
Energy providers may also offer special programs for businesses that produce their own energy. These programs may include rebates, technical support, or preferential rates for power purchased from renewable sources. By working with energy providers, breweries can gain access to the latest energy technologies and ensure they are taking advantage of available funding opportunities.
In addition to financial incentives, breweries may also benefit from energy efficiency audits or consultations provided by energy providers. These audits can identify areas for improvement in the brewery’s operations, such as equipment upgrades or changes in energy usage patterns, further reducing energy consumption and increasing overall energy savings.
Customizing Energy Systems to Fit Brewery Needs: Each brewery has unique energy needs based on its size, production scale, location, and type of brewing processes. As such, a one-size-fits-all approach to small-scale power plants may not always be the best solution. A customized energy system tailored to the brewery’s specific requirements ensures the most efficient energy production and consumption.
Energy systems should be designed with the brewery’s current and future needs in mind, taking into consideration its size, energy demands, and growth projections. For example, a small microbrewery might begin with a solar-powered system that generates electricity for lighting and refrigeration, while a large brewery may require a hybrid system with both wind and biomass to meet the power needs of brewing, packaging, and cooling equipment.
Additionally, breweries should consider energy flexibility when designing their systems. Some systems may need to be highly modular, allowing the brewery to scale up or down depending on changes in production. Modular biomass systems, for instance, allow for gradual expansion as more energy is required. Similarly, rooftop solar panels can be easily expanded as the brewery grows or its energy needs change.
Energy Recovery from Brewing Waste Heat: Another way breweries can enhance their energy efficiency is through the recovery and reuse of waste heat generated during the brewing process. Heat recovery systems can capture the excess heat produced during boiling, fermentation, and other brewing operations, which is typically released into the environment as waste.
This recovered heat can be used to power steam turbines or other energy recovery systems that convert thermal energy into usable electricity. Additionally, the recovered heat can be repurposed for other brewing processes, such as heating water for cleaning or sterilizing equipment, reducing the need to generate additional heat through external sources like gas or electric heaters.
Utilizing waste heat reduces energy consumption and further lowers costs associated with energy production, creating a more energy-efficient brewery. This is particularly valuable in breweries that use large amounts of heat for brewing, where even small reductions in energy consumption can lead to significant savings.
Eco-friendly Packaging and Waste Reduction: While not directly related to power generation, breweries can amplify the environmental benefits of small-scale power plants by addressing energy consumption in packaging and waste management. For instance, implementing a circular packaging system, where used bottles and cans are returned, cleaned, and reused, can significantly reduce the brewery’s carbon footprint. Similarly, breweries can adopt recyclable or biodegradable packaging materials to further reduce environmental waste.
Waste reduction strategies such as composting spent grains, hops, and other organic by-products of the brewing process not only minimize landfill waste but also create opportunities for local agricultural producers to use these materials as fertilizers or animal feed. Many breweries are turning their waste streams into valuable products, aligning their operations with the principles of the circular economy.
By minimizing energy use in all parts of the production process, including packaging and waste management, breweries can create a more comprehensive sustainability strategy that extends beyond just the energy production side. These efforts contribute to a brewery’s overall environmental impact, enhancing its reputation as a sustainable business and helping to attract environmentally-conscious consumers.
Empowering Employees and Stakeholders: The shift toward renewable energy also presents an opportunity to engage employees and other stakeholders in the brewery’s sustainability efforts. By educating workers about the benefits of renewable energy and involving them in energy-saving initiatives, breweries can foster a culture of sustainability that extends throughout the organization. Employee participation in energy conservation practices, such as turning off lights when not in use or optimizing energy use in different areas of the brewery, can result in significant energy savings.
Breweries can also involve their customers by offering sustainability-focused tours and demonstrations of their renewable energy systems. Sharing the brewery’s commitment to green energy initiatives with visitors can help spread awareness about renewable energy and inspire other businesses to adopt similar practices.
Additionally, breweries can host sustainability events, such as energy efficiency workshops or community clean-up days, to engage with the local community and reinforce their commitment to renewable energy and environmental stewardship.
Future Trends in Brewery Energy Solutions: The future of small-scale power plants for breweries is exciting, with several emerging trends that will further improve energy generation, storage, and efficiency. One such trend is the increased use of artificial intelligence and machine learning in energy management. AI can predict energy usage patterns more accurately, adjust energy systems in real time, and integrate data from different renewable energy sources to optimize efficiency.
Energy storage technology is also advancing, with developments in solid-state batteries, hydrogen storage, and thermal storage providing new ways to store and manage energy. For breweries that use intermittent renewable energy sources like solar or wind, these advanced storage technologies could make it easier to ensure a reliable power supply without relying on traditional backup generators or grid power.
In addition, advances in decentralized energy systems, including blockchain technology, will likely play a role in the future of energy management for breweries. Blockchain could enable transparent, secure transactions between breweries, energy producers, and consumers, facilitating peer-to-peer energy exchanges and improving the overall energy ecosystem.
As breweries continue to embrace these trends, small-scale power plants will play an even greater role in shaping the industry’s energy future. By adopting the latest energy technologies and integrating them into their operations, breweries can further reduce their environmental impact, increase efficiency, and enhance the sustainability of their products.
Final Thoughts:
The transition to renewable energy through small-scale power plants offers significant benefits to breweries, both in terms of operational efficiency and environmental responsibility. By incorporating solar, wind, biomass, and other renewable energy sources into their operations, breweries can lower energy costs, reduce their carbon footprint, and position themselves as leaders in sustainability. Advanced energy technologies, smart grids, energy storage, and waste-to-energy systems provide breweries with the tools they need to optimize energy generation and consumption.
As breweries continue to grow and evolve, their energy systems must also adapt to meet changing demands. With energy recovery, hybrid energy systems, and smart energy management solutions, breweries can ensure a reliable, sustainable energy supply that supports their long-term growth. Through collaboration with energy providers, investment in energy efficiency, and a commitment to reducing environmental impact, breweries can build resilience and contribute to the larger goal of a clean, sustainable energy future.
The journey towards energy self-sufficiency and sustainability will continue to provide breweries with opportunities for innovation, cost savings, and consumer engagement. As the brewing industry increasingly embraces renewable energy, it will play an essential role in the global transition toward cleaner, greener energy solutions.
Small-scale power plants for mining operations are essential to ensure reliable and cost-effective electricity supply, particularly in remote or off-grid locations. These plants are designed to provide sufficient energy to power mining equipment, processing facilities, and supporting infrastructure. Mining operations require a stable and continuous power supply due to the energy-intensive nature of activities such as drilling, blasting, hauling, crushing, grinding, and material separation. The choice of power generation technology depends on factors such as fuel availability, environmental considerations, capital and operational costs, and regulatory requirements.
Diesel generators are commonly used in small-scale mining power plants due to their reliability and ability to provide immediate power. Diesel fuel is often accessible, and generators can be scaled according to demand. However, diesel-based power generation has high fuel costs and environmental concerns, particularly related to greenhouse gas emissions and fuel transportation logistics. In some cases, hybrid systems combining diesel generators with renewable energy sources like solar or wind can reduce fuel consumption and operating costs.
Gas-powered plants, particularly those using natural gas or liquefied natural gas (LNG), provide a cleaner alternative to diesel. Natural gas power plants have lower emissions and can be cost-effective in areas where gas supply is available. LNG can be transported to remote locations, offering flexibility for mining operations far from gas pipelines. Gas engines and microturbines are commonly used in small-scale mining applications to generate electricity efficiently while reducing operational costs.
Renewable energy integration is gaining popularity in mining power solutions. Solar photovoltaic (PV) systems can be deployed to supplement energy needs, especially in regions with high solar irradiation. Wind turbines are another viable option for locations with consistent wind patterns. Hybrid renewable systems, often combined with battery storage, enhance energy reliability by mitigating fluctuations in renewable energy generation. By reducing dependence on fossil fuels, mining operations can lower emissions and improve sustainability.
Hydropower is an attractive option for mining operations near rivers or water sources with adequate flow rates. Small-scale hydroelectric plants, including run-of-river systems, can provide a steady and renewable energy supply. While hydropower requires initial infrastructure investment, it offers long-term benefits such as low operational costs and minimal fuel dependency.
Waste heat recovery and cogeneration systems improve overall efficiency in mining power plants. Combined heat and power (CHP) systems utilize excess heat from power generation to support heating, drying, or steam applications within mining processes. This enhances energy efficiency and reduces fuel consumption. Steam turbines, often used in cogeneration systems, can convert waste heat into additional electricity, optimizing the overall energy balance of the operation.
Energy storage systems, particularly lithium-ion and flow batteries, enhance the stability of mining power plants by storing excess energy for use during peak demand or low generation periods. Battery storage solutions are particularly useful in hybrid power plants integrating renewable energy sources, ensuring uninterrupted power supply and reducing reliance on backup generators.
Grid connectivity is another factor influencing small-scale power plant design. Some mining sites may have partial grid access, allowing for grid-tied solutions where onsite power generation is supplemented by electricity imports. However, in fully off-grid locations, standalone power systems must be designed to handle varying load demands while maintaining reliability.
Cost considerations play a critical role in power plant selection. Capital expenditures (CAPEX) for power infrastructure, fuel costs, maintenance expenses, and regulatory compliance requirements must be carefully analyzed to optimize overall project economics. Power purchase agreements (PPAs) or leasing arrangements can help mining companies manage upfront costs by outsourcing power generation to independent power producers.
Environmental and regulatory compliance is increasingly important in mining power projects. Governments and environmental agencies impose emissions limits, fuel usage restrictions, and sustainability mandates that influence power plant design. Incorporating cleaner technologies, improving efficiency, and adhering to environmental standards can enhance the social acceptability of mining operations while minimizing ecological impact.
In summary, small-scale power plants for mining operations require a tailored approach based on site-specific conditions, energy demand, and economic feasibility. Diesel generators, gas turbines, renewable energy integration, hydropower, cogeneration, and energy storage solutions each offer advantages and limitations. A well-designed power system ensures operational efficiency, cost-effectiveness, and sustainability, enabling mining operations to function smoothly while mitigating environmental impact.
Another important aspect of small-scale power plants for mining operations is the reliability and redundancy of the system. Mining activities operate around the clock, and power disruptions can lead to significant production losses, equipment damage, and safety risks. Therefore, power plants must incorporate backup generation capacity, automatic switching mechanisms, and predictive maintenance strategies to ensure continuous operation. Redundant power sources, such as a combination of primary and secondary generators or an integrated battery backup system, help mitigate the risks associated with power failures.
Fuel logistics and supply chain management are critical factors in determining the feasibility of different power generation options. In remote mining locations, fuel transportation can be costly and challenging due to inadequate road infrastructure and extreme weather conditions. Diesel fuel, while commonly used, requires a consistent supply chain to avoid shortages. In contrast, natural gas may necessitate the construction of pipelines or LNG storage facilities, adding to the initial investment. Renewable energy sources like solar and wind minimize fuel dependency but require battery storage or supplementary generation to account for variability.
The modularity and scalability of power plants play a key role in their adaptability to changing mining operations. Mining projects often evolve over time, with increasing energy demand as production scales up. Modular power plants, consisting of multiple smaller generation units, provide flexibility by allowing additional capacity to be added as required. Containerized power generation units, for instance, offer a plug-and-play solution that can be quickly deployed, relocated, or expanded based on the mine’s operational needs.
Automation and digital monitoring systems enhance the efficiency and performance of small-scale power plants. Modern mining operations integrate real-time monitoring technologies that track energy consumption, fuel usage, equipment performance, and maintenance needs. Supervisory Control and Data Acquisition (SCADA) systems, combined with Internet of Things (IoT) sensors, enable predictive maintenance, reducing downtime and improving operational efficiency. Artificial intelligence (AI) and machine learning algorithms can further optimize power management by forecasting energy demand and optimizing generation scheduling.
Environmental sustainability and emissions reduction are increasingly prioritized in mining power solutions. Governments and international organizations are implementing stricter environmental regulations, including carbon pricing and emissions reduction targets. Mining companies are under pressure to adopt greener energy solutions, such as carbon capture technologies, hybrid energy systems, and sustainable fuel alternatives. Biofuels, hydrogen fuel cells, and synthetic fuels are emerging as potential alternatives to conventional fossil fuels in mining power applications.
Water usage is another environmental consideration for mining power plants. Some power generation technologies, such as steam turbines and cooling systems, require significant water resources. In water-scarce regions, dry cooling systems, air-cooled condensers, or closed-loop cooling technologies can minimize water consumption while maintaining operational efficiency. Renewable energy sources like solar PV and wind have minimal water requirements, making them advantageous in arid mining locations.
Financial strategies for power plant investment in mining operations include a mix of capital funding, financing agreements, and partnerships with independent power producers (IPPs). Many mining companies opt for build-own-operate-transfer (BOOT) models, where a third-party provider finances, constructs, and operates the power plant, transferring ownership after a defined period. This approach reduces upfront costs for the mining company while ensuring reliable power supply. Power purchase agreements (PPAs) also allow mining operations to secure long-term electricity supply at predetermined rates, reducing exposure to fuel price fluctuations.
Social and community engagement plays a role in the success of mining power projects. In regions where mining operations impact local communities, shared energy infrastructure can provide benefits beyond the mine site. Excess power from the mining plant can be supplied to nearby communities, improving access to electricity and fostering positive relationships with local stakeholders. Community-based renewable energy projects, where solar or wind farms serve both the mine and surrounding areas, contribute to sustainable development and social responsibility.
Security and risk management are additional considerations for mining power plants. Remote mining sites often face security challenges, including theft of fuel or equipment, sabotage, and geopolitical risks. Securing the energy infrastructure involves deploying surveillance systems, physical barriers, and cybersecurity measures to protect digital monitoring and control systems from cyber threats.
Future trends in mining power solutions indicate a shift towards greater electrification, decarbonization, and automation. Battery-electric mining equipment, hydrogen-powered machinery, and microgrid solutions are becoming more prevalent, reducing reliance on traditional diesel-powered equipment. Advancements in battery technology, such as solid-state batteries and flow batteries, will further enhance energy storage capabilities, making renewable-powered mining operations more viable. Smart grid technologies and blockchain-based energy trading systems may also play a role in optimizing power distribution and cost management in mining operations.
Ultimately, small-scale power plants for mining operations must balance cost, reliability, sustainability, and scalability to meet the specific energy demands of each mining site. By leveraging innovative technologies, integrating renewable energy, and adopting flexible financing models, mining companies can achieve long-term operational efficiency while minimizing environmental impact.
The evolution of small-scale power plants for mining operations is increasingly shaped by advances in energy efficiency, sustainability, and digitalization. One of the key challenges mining operations face is optimizing energy usage while minimizing costs and environmental impact. Energy efficiency measures, such as demand-side management and load optimization, help reduce unnecessary power consumption. Advanced variable frequency drives (VFDs) are widely used in mining equipment like pumps, conveyors, and ventilation systems to adjust motor speeds based on real-time demand, reducing overall energy waste.
Mining companies are also exploring microgrid solutions to enhance power reliability and flexibility. A microgrid consists of decentralized energy sources, including a mix of conventional and renewable power generation, storage systems, and smart control technologies that manage power distribution efficiently. These microgrids can operate independently or in conjunction with the main grid, allowing mines to reduce energy costs and improve resilience against power outages. Hybrid microgrids, which combine diesel or gas generators with solar, wind, and battery storage, are particularly effective in remote locations where grid connectivity is unreliable or nonexistent.
The role of artificial intelligence (AI) and big data analytics is becoming increasingly significant in optimizing energy use in mining operations. AI-driven energy management systems can analyze historical and real-time data to predict power demand patterns, optimize generator usage, and reduce fuel consumption. These systems can also detect inefficiencies, such as abnormal equipment behavior, and recommend maintenance actions before failures occur. AI-powered automation extends beyond power generation, contributing to mine-wide optimization in drilling, ore processing, and transportation.
Another critical aspect of small-scale power solutions is the growing integration of hydrogen as an alternative fuel source. Hydrogen fuel cells can provide clean, efficient power for mining sites, particularly in regions where renewable hydrogen production is viable. Some mining operations are piloting hydrogen-powered vehicles and equipment, reducing reliance on diesel and lowering emissions. In addition, hydrogen can be used in hybrid power plants in combination with renewables to enhance energy security and reduce carbon footprints.
Waste-to-energy solutions are also being explored in the mining sector to improve sustainability. Some mines generate significant amounts of organic and industrial waste that can be converted into energy through gasification or anaerobic digestion. This approach not only reduces waste disposal issues but also provides a supplementary energy source for onsite power generation.
The integration of blockchain technology in energy management is an emerging trend that offers new ways to optimize power distribution and cost efficiency in mining operations. Blockchain can facilitate peer-to-peer energy trading within mining microgrids, allowing different units within a mining site—or even multiple mining operations—to share excess power in a secure and transparent manner. This decentralized approach to energy distribution can improve overall efficiency and reduce dependency on external power providers.
Electrification of mining equipment is another factor influencing small-scale power plant design. With the increasing adoption of electric haul trucks, loaders, and drilling rigs, the demand for onsite electricity generation is growing. Electrified mining fleets require reliable charging infrastructure, which can be integrated into the overall power plant system. Fast-charging stations powered by renewables or hybrid systems can support the transition to electric mining vehicles while reducing fuel costs and emissions.
Regulatory frameworks and government incentives play a crucial role in shaping the future of mining power solutions. Many countries are introducing policies to encourage the adoption of renewable energy and low-carbon technologies in mining. Incentives such as tax credits, grants, and carbon pricing mechanisms provide financial support for the transition to cleaner energy sources. Compliance with environmental regulations, such as emissions limits and energy efficiency standards, is becoming a key consideration for mining companies when selecting power generation technologies.
Another emerging development is the adoption of modular nuclear reactors (SMRs) for remote mining power supply. These small-scale nuclear power plants offer a stable and long-term energy solution, particularly for large mining operations that require continuous high power output. While regulatory approvals and public acceptance remain challenges, SMRs could become a viable alternative to diesel and gas generation in the future.
Ultimately, the future of small-scale power plants for mining operations lies in a combination of technological advancements, sustainability initiatives, and strategic planning. By integrating renewable energy, improving energy efficiency, leveraging digitalization, and adopting innovative financing models, mining companies can create more resilient, cost-effective, and environmentally responsible power solutions. The ongoing transition toward decarbonization and electrification will continue to shape the design and operation of mining power plants, ensuring they remain adaptable to evolving industry demands and global sustainability goals.
Small Scale Power Plant for Military Bases
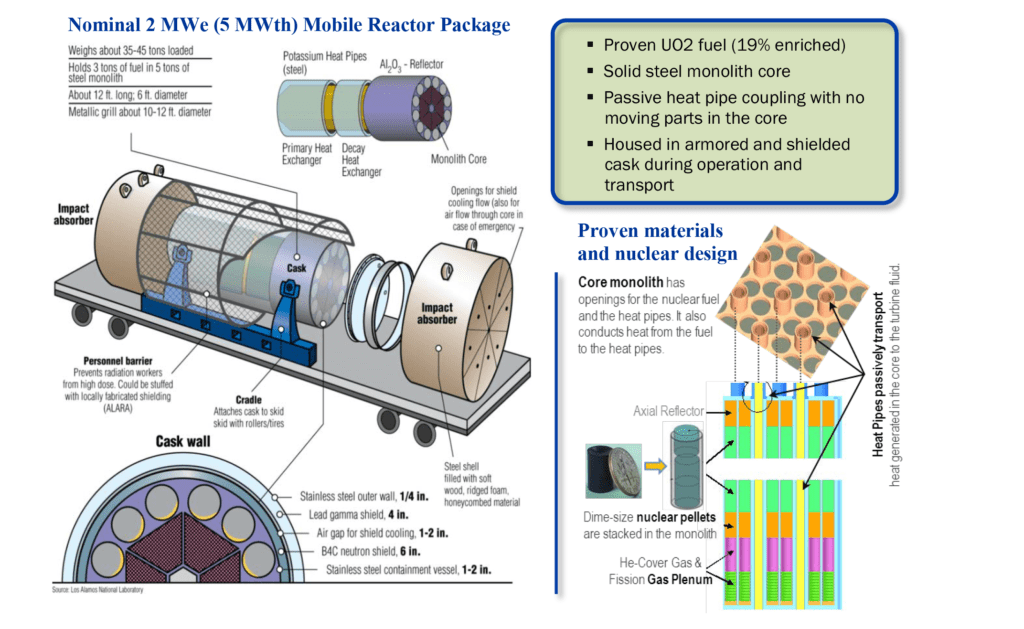
Small-scale power plants for military bases play a crucial role in ensuring energy security, operational readiness, and resilience in both peacetime and combat scenarios. Military installations, whether permanent or forward-operating bases, require reliable and uninterrupted power for mission-critical systems, including communication networks, surveillance equipment, weapon systems, living quarters, and medical facilities. Unlike conventional power plants, military power solutions must be designed with mobility, redundancy, and survivability in mind, often operating in remote or hostile environments where grid connectivity is unavailable or unreliable.
One of the most common power solutions for military bases is diesel generator-based microgrids. Diesel generators provide an immediate and dependable power source, capable of running in extreme conditions. However, diesel fuel logistics pose a major challenge, particularly for forward-deployed bases where fuel convoys are vulnerable to attacks. Fuel resupply operations are costly and dangerous, leading to increased efforts to reduce dependency on diesel through alternative power sources.
Hybrid power systems are gaining traction as a means to improve energy efficiency and reduce reliance on fossil fuels. These systems combine traditional generators with renewable energy sources like solar photovoltaic (PV) panels, wind turbines, and battery storage. Solar power is particularly advantageous in desert deployments, where high solar irradiation enables significant energy generation. Wind energy can also supplement power needs in coastal or high-altitude locations. Hybrid microgrids optimize fuel consumption by using renewable energy during peak sunlight or wind periods while relying on generators only when necessary, thereby extending fuel reserves and minimizing supply chain risks.
Battery energy storage systems (BESS) are increasingly integrated into military microgrids to enhance reliability and efficiency. Advanced lithium-ion and flow batteries store excess renewable energy, allowing bases to maintain power even when solar or wind generation fluctuates. These storage systems also enable silent watch operations, where generators are turned off to reduce acoustic and thermal signatures, making the base less detectable to adversaries.
Nuclear energy is being explored as a long-term solution for military power needs, particularly through small modular reactors (SMRs). These compact, transportable nuclear reactors offer a continuous and high-energy output without the logistical challenges of fuel resupply. SMRs are designed with advanced safety features and passive cooling systems, making them suitable for deployment in isolated locations. While regulatory and security concerns must be addressed, the potential of SMRs to provide reliable, long-term power for remote bases makes them an attractive option for future military energy strategies.
Waste-to-energy (WTE) technologies offer another innovative approach to military power generation. Bases generate significant amounts of organic and inorganic waste, which can be converted into electricity through gasification or anaerobic digestion. WTE systems reduce logistical burdens associated with waste disposal while simultaneously generating supplementary power. These systems are particularly beneficial for expeditionary forces operating in austere environments where conventional fuel sources are scarce.
The increasing electrification of military equipment and vehicles is also reshaping power requirements at bases. Electrified ground vehicles, drones, and aircraft require robust charging infrastructure integrated with the base’s power system. Wireless charging pads, microgrid-connected charging stations, and fast-charging battery solutions are being developed to support next-generation military platforms.
Cybersecurity and resilience are paramount in military power systems. Adversaries may attempt to disrupt base operations through cyberattacks targeting power infrastructure. Secure, encrypted control systems, artificial intelligence-driven threat detection, and decentralized microgrid architectures enhance energy resilience by reducing vulnerabilities. Additionally, EMP (electromagnetic pulse) protection measures are implemented to shield power generation equipment from potential attacks designed to disable electronic systems.
Autonomy and automation are key trends in modern military power plants. AI-driven energy management systems optimize power distribution, predict maintenance needs, and adjust generation based on real-time demand. Automated deployment systems, such as rapidly deployable solar arrays and containerized power units, enhance mobility and adaptability in dynamic operational environments.
Future developments in military power generation will focus on increasing efficiency, reducing emissions, and improving mobility. Advances in hydrogen fuel cells, portable nuclear reactors, and ultra-lightweight energy storage systems will play a critical role in shaping the next generation of military power solutions. By integrating diverse energy sources and adopting smart grid technologies, military bases can enhance self-sufficiency, improve operational effectiveness, and reduce risks associated with fuel dependency in conflict zones.
The development of small-scale power plants for military bases continues to evolve with a focus on energy security, operational efficiency, and sustainability. As military forces become more reliant on high-tech equipment, communication networks, and unmanned systems, the demand for stable and scalable power solutions increases. The integration of smart energy management, modular generation units, and alternative fuels ensures that military bases can operate independently and efficiently, even in the most challenging environments.
One of the most critical aspects of military power infrastructure is redundancy. Military operations cannot afford power outages, as they can disrupt surveillance systems, command centers, and critical defense operations. To address this, modern military bases incorporate multi-layered energy solutions, such as redundant microgrids, distributed energy resources (DERs), and rapid power-switching capabilities. These systems allow seamless transitions between different power sources, ensuring uninterrupted operation in the event of generator failure, cyberattacks, or fuel shortages.
The advancement of deployable power generation units has significantly improved mobility and adaptability in expeditionary operations. Containerized power units, designed for rapid deployment and setup, are essential for forward-operating bases and temporary installations. These units often include a combination of diesel generators, renewable energy sources, and battery storage, enabling self-sufficient power production with minimal logistical support. Some models incorporate flexible solar panels that can be quickly unfolded and deployed alongside battery packs, reducing the need for continuous fuel supply.
Hydrogen fuel cells are emerging as a promising alternative energy source for military bases. Hydrogen provides a clean and efficient power solution with minimal logistical footprint. Fuel cells can be used for both stationary and mobile applications, powering everything from base infrastructure to unmanned aerial vehicles (UAVs) and electric military vehicles. The production of green hydrogen using onsite renewable energy sources further enhances energy independence, reducing the need for fuel convoys and lowering the risk of supply chain attacks.
One of the most significant trends in military energy strategy is the development of self-healing microgrids. These advanced systems use artificial intelligence and machine learning to detect, isolate, and respond to disruptions in real time. Self-healing microgrids can automatically reroute power, prioritize critical loads, and restore functionality after system failures or attacks. The use of blockchain technology in microgrid management is also being explored to enable secure, decentralized energy trading and allocation within a base or between allied forces.
Waste reduction and environmental sustainability are becoming increasingly important for military power systems. The adoption of closed-loop energy systems, which recycle and repurpose waste heat, is gaining traction. Combined heat and power (CHP) systems capture excess heat from power generation and use it for heating, water purification, or secondary energy generation. This not only improves efficiency but also reduces the base’s overall energy consumption and environmental impact.
Cyber and physical security of military power plants remain top priorities. With the increasing threat of cyber warfare, military bases invest heavily in hardened control systems that are resistant to hacking and EMP attacks. Power infrastructure is designed with multiple layers of defense, including backup communication networks, encrypted control protocols, and isolated critical power nodes that ensure mission continuity in the event of cyber or kinetic attacks.
Future advancements in military power solutions will likely involve the expansion of autonomous energy systems. Swarm technology, where multiple small energy units work together in a coordinated manner, could provide scalable and resilient power solutions. Autonomous drones equipped with solar panels and wireless power transmission capabilities may soon be used to provide temporary or emergency power to field units.
Ultimately, the future of small-scale power plants for military bases will be shaped by the need for resilience, efficiency, and sustainability. By integrating cutting-edge technologies such as modular nuclear reactors, hydrogen-based microgrids, and AI-driven energy optimization, military forces can achieve energy independence and operational superiority. The shift toward cleaner, smarter, and more adaptable power systems will not only enhance mission readiness but also reduce logistical burdens and environmental impact, ensuring that modern military operations remain agile and self-sufficient in an increasingly complex global landscape.
Another critical consideration for small-scale power plants in military bases is the growing emphasis on energy efficiency and demand-side management. Military installations, particularly those in remote or hostile regions, require intelligent power distribution to minimize waste and maximize operational uptime. Advanced energy management systems (EMS) are being integrated into base infrastructure to monitor power consumption in real-time, prioritize essential loads, and optimize generator operation based on demand patterns. These systems help reduce unnecessary fuel consumption, extend equipment lifespan, and enhance overall energy resilience.
The shift towards electrification of military assets is another factor driving changes in power plant design. Traditionally, diesel-powered vehicles and equipment have dominated military operations, but there is a growing push toward electric and hybrid military vehicles, including armored personnel carriers, transport trucks, and even combat vehicles. This transition increases the need for high-capacity charging infrastructure within bases, requiring power plants to support fast-charging technologies and energy storage systems. Portable and deployable charging stations powered by solar or hybrid microgrids are being developed to provide energy flexibility for electric vehicles in the field.
Another emerging innovation in military energy strategy is wireless power transmission (WPT). Research into beamed energy, using lasers or microwaves, has the potential to supply power to distant forward operating bases, drones, or remote surveillance units without requiring direct fuel transport. This could significantly reduce the need for risky supply convoys, a major vulnerability in military logistics. Some experimental systems already demonstrate the feasibility of transmitting power wirelessly over several kilometers, and future advancements may enable military bases to receive energy from distant power stations or even from space-based solar arrays.
To further reduce reliance on fossil fuels, military bases are integrating renewable energy storage solutions such as solid-state batteries, supercapacitors, and flywheel energy storage systems. These technologies offer advantages over conventional lithium-ion batteries, including longer lifespan, faster charging, and improved safety. By coupling these advanced storage solutions with microgrids, bases can store excess solar or wind energy for use during periods of low generation or increased demand, improving overall energy reliability.
Another important consideration is logistical and operational scalability. Military bases must be able to adapt their power infrastructure to different mission requirements, from long-term installations to rapidly deployed forward bases. Modular power generation systems—which can be easily transported, assembled, and expanded—are a key innovation. These systems typically consist of prefabricated, containerized power units that can be deployed via aircraft, ships, or land transport and quickly activated upon arrival at a new location. The U.S. Department of Defense, for example, has been developing mobile nuclear reactors under the “Project Pele” initiative, aiming to provide self-sustaining energy in remote areas with minimal logistical support.
Additionally, multi-domain energy integration is becoming a focal point for future military power plants. Modern warfare increasingly involves cyber operations, electronic warfare, and space-based assets, all of which require high-power, uninterrupted energy sources. Military bases must support directed energy weapons (DEWs) such as high-energy lasers (HELs) and microwave-based counter-drone systems, which demand large bursts of power. This requirement is influencing power plant designs to include on-demand high-energy storage capable of delivering short, high-power discharges without affecting overall base operations.
Future small-scale military power plants will continue to evolve in response to the increasing complexity of military operations. Innovations such as artificial intelligence-driven predictive maintenance, self-healing energy grids, and autonomous power distribution systems will enhance operational effectiveness and reduce the manpower required to maintain energy infrastructure. With a strong push toward decarbonization and energy independence, military bases will likely continue to lead the way in advanced microgrid technologies, hybrid energy systems, and next-generation power storage solutions, ensuring sustained mission readiness and security in diverse operational environments.
Small Scale Power Plant for Hospitals
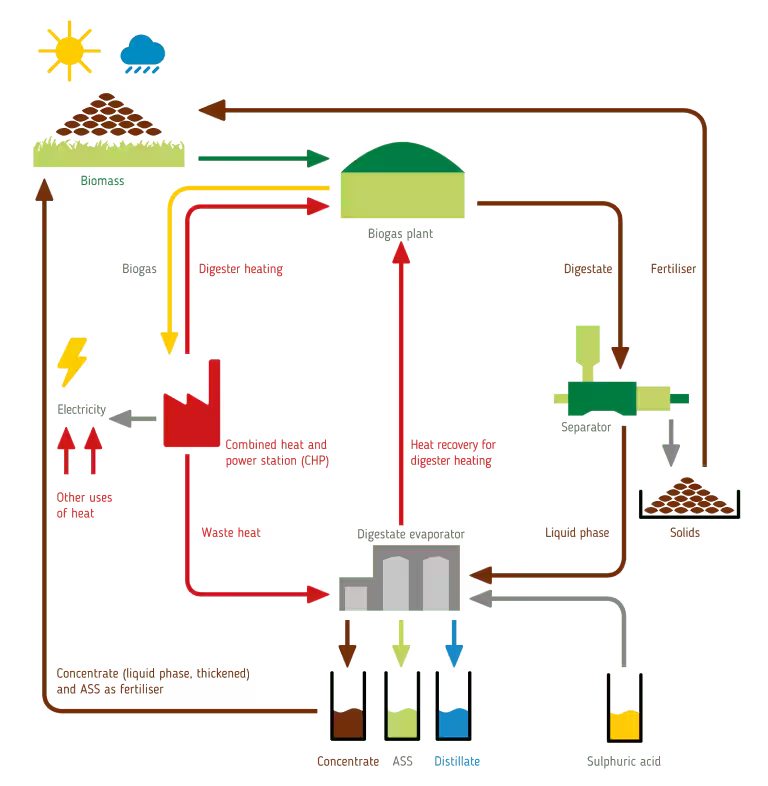
Small-scale power plants for hospitals play a crucial role in ensuring continuous and reliable electricity supply, as healthcare facilities depend on uninterrupted power for life-saving equipment, critical medical procedures, and patient care. Unlike other types of facilities, hospitals cannot afford power failures, as even a brief outage could put patients at risk, disrupt surgeries, or affect critical care units such as intensive care (ICU) and neonatal units. Therefore, power plants serving hospitals must prioritize reliability, redundancy, energy efficiency, and sustainability while integrating advanced energy management systems.
One of the most fundamental elements of hospital power infrastructure is emergency backup power, typically provided by diesel or natural gas generators. These generators are activated within seconds of a grid failure, ensuring that essential medical equipment such as ventilators, life-support systems, and monitoring devices continue to function. Many hospitals use a tiered backup system, with uninterruptible power supplies (UPS) and battery storage covering the transition period until the main backup generators start operating. Advanced UPS systems use lithium-ion or solid-state batteries, which provide instant power without fluctuations, ensuring seamless transitions during outages.
Hospitals are increasingly adopting microgrid solutions to enhance energy resilience. A hospital microgrid consists of multiple distributed energy resources (DERs), such as solar panels, battery storage, and cogeneration units, that work together to provide continuous power even when the main grid is down. These microgrids can operate in an island mode, meaning they can function independently from the larger power grid when necessary. Hospitals in regions prone to extreme weather events, such as hurricanes or wildfires, are investing in microgrid technology to ensure power autonomy during natural disasters.
Cogeneration and trigeneration systems are becoming popular in hospital power plants due to their efficiency. Combined heat and power (CHP) plants generate electricity while capturing waste heat for hospital heating, sterilization, and hot water supply. Trigeneration (CCHP) extends this capability by also providing cooling, which is critical for operating rooms, pharmaceutical storage, and patient comfort. These systems significantly improve energy efficiency and reduce overall energy costs.
Another emerging trend in hospital power infrastructure is the use of renewable energy sources such as solar PV, wind turbines, and bioenergy. Many hospitals are installing rooftop solar panels and integrating them with battery storage to reduce reliance on the grid. While renewables alone may not be sufficient to power an entire hospital, they serve as an excellent supplemental energy source, particularly when paired with energy storage systems. Green hydrogen is also being explored as a long-term clean energy solution for hospitals, especially in regions aiming for carbon neutrality in healthcare operations.
In addition to power generation, smart energy management systems (EMS) are being implemented in hospitals to monitor and optimize energy consumption. These AI-powered systems track real-time energy use, predict peak demand periods, and automatically adjust power distribution to prioritize critical areas like operating theaters and intensive care units. EMS can also integrate demand response strategies, allowing hospitals to shift non-essential energy loads during peak hours, reducing strain on the grid and lowering energy costs.
With the growing demand for medical imaging, data centers, and digital healthcare solutions, hospitals require advanced energy storage solutions to support their expanding power needs. Flow batteries, supercapacitors, and next-generation lithium-ion batteries are being tested in hospital microgrids to provide extended power backup and improve energy reliability. In addition, wireless power transfer (WPT) technology is being explored to reduce reliance on traditional power infrastructure and enable seamless energy distribution in critical care areas.
Cybersecurity and physical security are crucial for hospital power plants, as cyber threats targeting healthcare infrastructure have increased. Hardened energy management systems with secure, encrypted controls help prevent cyberattacks that could disrupt hospital operations. Hospitals also invest in EMP-resistant energy storage and backup systems to safeguard against electromagnetic threats that could compromise electronic medical devices and patient data.
Future developments in hospital power plants will focus on enhancing energy resilience, sustainability, and efficiency. The integration of AI-driven predictive maintenance, blockchain-based energy trading, and grid-independent power solutions will shape the next generation of hospital energy infrastructure. As hospitals continue to adopt net-zero energy goals, the deployment of carbon-neutral microgrids, modular nuclear reactors, and hydrogen-based fuel cells will play a key role in achieving energy self-sufficiency while maintaining the highest standards of patient care and safety.
The future of small-scale power plants for hospitals will continue to evolve in response to growing energy demands, advancements in medical technology, and the need for greater energy resilience in the face of climate change and cyber threats. As hospitals increasingly rely on digital health systems, robotic surgeries, and AI-powered diagnostics, power infrastructure must keep pace by providing stable, uninterrupted, and high-quality electricity while also being adaptable to changing healthcare needs.
One of the most promising advancements in hospital energy solutions is the use of modular power generation. These systems allow hospitals to scale their power generation capacity based on demand, making it easier to integrate new medical technologies that require substantial energy input. Modular nuclear reactors (SMRs) are being explored in some regions as a potential solution for large hospital networks, providing a continuous power supply with zero carbon emissions. While still in the early stages of implementation, SMRs have the potential to revolutionize hospital energy resilience by offering years of uninterrupted power without the need for frequent fuel supply.
Another key development is the integration of artificial intelligence (AI) and machine learning (ML) in hospital power management. AI-driven predictive analytics help hospitals anticipate power demand fluctuations, optimize energy usage, and detect potential failures before they occur. These systems use real-time monitoring to ensure that power is distributed efficiently, prioritizing life-critical equipment in ICUs, emergency rooms, and operating theaters. AI also assists in fault detection and automated grid balancing, allowing hospitals to quickly recover from power disruptions.
On-site hydrogen production and fuel cells are gaining interest as hospitals seek low-emission and highly reliable power sources. Hydrogen can be produced using electrolysis powered by renewable energy, providing a sustainable and storable energy solution. Unlike batteries, hydrogen fuel cells do not degrade over time, making them ideal for long-term hospital energy storage. In addition, fuel cells produce both electricity and heat, which can be used for sterilization, heating, and air purification in hospital environments.
The use of waste-to-energy (WTE) systems is another emerging trend in hospital power plants. Hospitals generate large amounts of medical waste, including organic material, pharmaceuticals, and plastics. Advanced gasification and anaerobic digestion technologies convert this waste into usable electricity, reducing landfill dependency while simultaneously providing an on-site energy source. Some hospitals have already adopted biogas-based CHP systems, using waste from hospital cafeterias and organic materials to generate clean power and heat.
The increasing demand for highly specialized hospital services, such as cryogenic storage, MRI machines, and robotic-assisted surgeries, requires stable voltage and frequency control in power systems. Supercapacitors and solid-state batteries are being tested to ensure hospitals can handle sudden spikes in energy demand without causing fluctuations that could damage sensitive medical equipment.
Another major area of development is the improvement of energy storage systems (ESS) for hospitals. Traditional lead-acid and lithium-ion batteries are now being supplemented with vanadium redox flow batteries (VRFBs), sodium-ion batteries, and graphene-enhanced energy storage solutions. These new battery technologies offer longer lifespan, faster charging, and greater efficiency, ensuring hospitals can operate independently for extended periods in the event of grid failures or natural disasters.
Cybersecurity remains a top priority for hospital power infrastructure. As more hospitals adopt smart grid technology and remote monitoring systems, the risk of cyberattacks targeting hospital microgrids increases. Advanced blockchain-based energy security, zero-trust network architectures, and hardened control systems are being developed to prevent unauthorized access to power management systems.
The growing focus on sustainability in healthcare operations is also shaping the future of hospital power plants. Many hospitals are aiming to achieve carbon neutrality by integrating solar power, geothermal heating, and biofuel-based backup generators into their energy mix. Some hospitals are now part of community energy-sharing networks, where excess energy from hospital microgrids is redistributed to surrounding areas or other critical infrastructure in times of need.
Ultimately, the future of hospital power plants will be characterized by resilient, decentralized, and AI-driven energy solutions that ensure 24/7 uninterrupted power while reducing costs and environmental impact. With continued innovation in renewable integration, energy storage, and autonomous power management, hospitals will be better equipped to handle emergencies, improve patient care, and support the next generation of medical technology.
Another critical aspect of hospital power plants is their ability to withstand and recover from extreme events, such as natural disasters, cyberattacks, and pandemics. Hospitals serve as emergency response hubs, making their energy resilience a matter of life and death. To enhance disaster preparedness, hospitals are increasingly investing in multi-source power redundancy, ensuring that multiple independent power sources, such as solar, diesel, battery storage, and fuel cells, can work together to provide layered backup in case of grid failure.
One of the biggest challenges hospitals face is maintaining continuous operation during grid instability or blackouts. To address this, modern hospitals are adopting self-healing microgrids, which use AI-driven energy management to automatically detect faults, isolate affected areas, and reroute power within the hospital’s network. These smart grids ensure that life-critical equipment and intensive care units (ICUs) always receive priority power, even when other parts of the hospital experience reduced energy availability.
The role of energy storage in hospital power plants is evolving rapidly. Traditional backup diesel generators, while reliable, come with drawbacks such as fuel storage limitations, maintenance requirements, and high emissions. To mitigate these issues, hospitals are now incorporating long-duration battery storage technologies. Vanadium redox flow batteries (VRFBs), sodium-ion batteries, and solid-state batteries offer significant advantages over conventional lithium-ion storage, including longer lifespan, greater thermal stability, and the ability to withstand frequent charge-discharge cycles. These advanced storage solutions ensure hospitals can function independently for extended periods without relying on external fuel supplies.
Another emerging trend is the integration of modular and mobile power solutions. Hospitals in disaster-prone regions or remote locations require rapidly deployable energy solutions that can be installed, scaled, and transported as needed. Mobile containerized power units, equipped with a combination of solar panels, battery storage, and hydrogen fuel cells, are being developed to provide hospitals with temporary or supplemental energy during crises. These units are designed to be airlifted or trucked to affected areas, ensuring hospitals continue operating even in the aftermath of major disasters.
Hospitals are also looking at waste heat recovery and energy recycling to improve efficiency. Combined Heat and Power (CHP) and Trigeneration (CCHP) systems allow hospitals to capture waste heat from power generation and use it for sterilization, heating, and even cooling through absorption chillers. These systems reduce energy waste, lower costs, and improve overall efficiency, making them an attractive option for hospitals aiming to optimize their power plants.
Additionally, hydrogen fuel cells are emerging as a long-term backup power solution for hospitals, particularly in regions shifting toward decarbonized healthcare operations. Hydrogen-powered microgrids offer zero-emission energy storage, and when paired with onsite electrolysis, hospitals can generate their own hydrogen fuel using renewable electricity. This approach reduces reliance on diesel fuel while ensuring that backup power remains scalable and sustainable.
With the increasing reliance on medical AI, telemedicine, and data-intensive applications, hospitals must also account for high-power computing and data center energy requirements. Medical imaging, robotic-assisted surgeries, and AI-powered diagnostics require stable, high-capacity power to function without interruptions. Hospitals are investing in dedicated energy storage and power quality control systems to prevent voltage fluctuations or frequency instability from affecting sensitive medical equipment.
Finally, grid-interactive hospital buildings are being developed to integrate hospitals into the broader energy ecosystem. These smart hospitals can generate, store, and share excess energy with surrounding communities during non-peak hours while ensuring they have ample reserves for emergencies. Hospitals adopting this approach not only reduce operational costs but also contribute to regional energy stability by acting as anchor institutions for local microgrids.
The future of hospital power plants will be shaped by AI-driven automation, advanced energy storage, sustainable microgrid solutions, and decentralized power generation. By combining multiple energy sources, leveraging predictive analytics, and enhancing redundancy, hospitals can achieve unprecedented energy resilience, ensuring they remain fully operational under any circumstances, safeguarding patient lives and advancing the future of sustainable, energy-secure healthcare.
Small Scale Power Plant for hotels and resorts
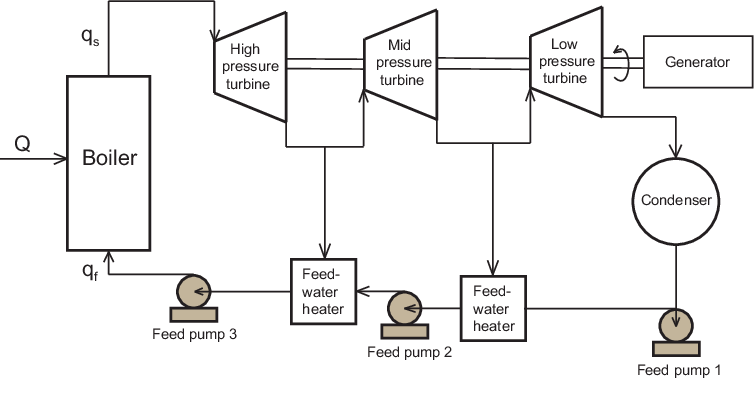
Small-scale power plants for hotels and resorts play a crucial role in ensuring energy reliability, sustainability, and cost efficiency while enhancing guest comfort and operational efficiency. Unlike industrial or medical facilities, hotels and resorts prioritize uninterrupted power supply, optimal climate control, lighting, and water heating, all of which require a well-designed and efficient power generation system. Given the rising demand for eco-friendly tourism and energy independence, many hospitality businesses are now integrating hybrid microgrids, renewable energy sources, and advanced energy management systems to optimize power consumption and reduce their carbon footprint.
One of the most common power solutions for hotels and resorts is the Combined Heat and Power (CHP) system, also known as cogeneration. These systems generate electricity while utilizing the waste heat for applications such as hot water production, swimming pool heating, laundry facilities, and HVAC systems. Some resorts also incorporate Trigeneration (CCHP) systems, which extend this capability to provide cooling using absorption chillers, ensuring energy efficiency and lower utility costs.
Many hotels and resorts, particularly those in remote or off-grid locations, rely on hybrid microgrids that integrate solar PV, wind turbines, bioenergy, and battery storage alongside conventional backup generators. These hybrid power systems allow resorts to maximize renewable energy use while ensuring 24/7 power availability. Resorts in tropical and coastal regions are particularly well-positioned to harness solar energy, while those in mountainous or high-altitude areas benefit from small-scale wind power systems.
Battery energy storage systems (BESS) play a key role in managing hotel power supply, allowing for load balancing and peak shaving, reducing reliance on diesel or grid electricity during high-demand periods. Modern resorts are investing in lithium-ion, vanadium redox flow batteries, and solid-state storage technologies to store excess renewable energy during the day and deploy it during nighttime peak hours. This approach not only enhances energy self-sufficiency but also reduces operational costs and dependence on fossil fuels.
For hotels and resorts seeking carbon neutrality, biogas and biomass energy solutions are gaining traction. Kitchen waste, organic food scraps, and garden trimmings can be converted into biogas through anaerobic digestion, producing electricity while also reducing waste disposal costs. Some resorts even use waste-to-energy (WTE) systems to convert municipal solid waste into power, further enhancing their sustainability efforts.
Another innovative trend in hotel energy infrastructure is geothermal energy utilization. Some high-end resorts located in volcanic or geothermal-active regions are leveraging geothermal heat pumps to provide low-cost, renewable heating and cooling for guest rooms, spa facilities, and heated pools. This high-efficiency, low-maintenance solution significantly reduces the carbon footprint of hotel operations.
Incorporating Artificial Intelligence (AI) and Smart Energy Management Systems (EMS) is becoming a standard for modern hotels and resorts. These AI-driven platforms monitor real-time energy consumption, optimize power distribution, and automatically adjust lighting, temperature control, and HVAC systems based on guest occupancy and usage patterns. Predictive maintenance algorithms also ensure that power generation equipment remains in top condition, minimizing breakdowns and unexpected repair costs.
Additionally, hotels in urban settings are exploring grid-interactive buildings, which allow them to participate in demand response programs and sell excess energy back to the grid. Blockchain-based energy trading platforms are also being piloted in some smart hotels, enabling decentralized energy exchange between hospitality businesses and surrounding communities.
With the increasing demand for luxury experiences, hotels and resorts are also implementing wireless power transmission (WPT) technologies, which allow for seamless energy distribution to smart devices, automated concierge systems, and contactless guest services without visible cables or power outlets.
As the hospitality industry moves toward net-zero energy goals, future hotel power plants will focus on high-efficiency hybrid energy solutions, next-generation energy storage, AI-driven automation, and decentralized renewable power integration. By embracing these innovations, hotels and resorts can ensure uninterrupted, cost-effective, and sustainable energy solutions, providing an unparalleled guest experience while reducing environmental impact.
The future of small-scale power plants for hotels and resorts will continue to evolve as sustainability, resilience, and energy efficiency become top priorities in the hospitality industry. To remain competitive and meet both regulatory requirements and guest expectations, hotels and resorts are integrating cutting-edge technologies that improve energy management, reduce operational costs, and minimize environmental impact.
One of the most significant advancements is the expansion of smart microgrids, which allow hotels to operate independently from the main grid while optimizing energy generation and consumption. These microgrids integrate multiple energy sources—such as solar PV, wind turbines, hydro turbines, biogas, and advanced battery storage—to create a self-sustaining energy ecosystem. Smart microgrids use artificial intelligence (AI) and IoT (Internet of Things) sensors to analyze energy demand in real time, automatically switching between power sources to ensure maximum efficiency and cost savings.
Another emerging trend is the deployment of next-generation energy storage systems (ESS), including graphene-based supercapacitors, sodium-ion batteries, and flow batteries. Unlike traditional lithium-ion batteries, these long-duration storage solutions offer greater thermal stability, faster charging cycles, and a longer lifespan, making them ideal for hotels and resorts that require high energy availability and resilience. These advanced storage technologies help mitigate power fluctuations and ensure a smooth, uninterrupted guest experience, even in regions prone to grid instability or extreme weather events.
For remote resorts located in island destinations, mountains, or desert regions, power reliability remains a critical challenge. Many such properties are fully off-grid and rely on diesel generators, which are costly and environmentally harmful. To address this, hotels are adopting modular renewable energy solutions, such as floating solar farms for island resorts, small-scale hydroelectric plants for mountain lodges, and concentrated solar power (CSP) systems for desert-based hotels. These solutions reduce fuel dependency and significantly cut carbon emissions while ensuring energy security.
Incorporating waste-to-energy (WTE) and circular economy principles is also gaining traction. Some eco-resorts are using advanced gasification, pyrolysis, and anaerobic digestion to convert organic waste, used cooking oil, and wastewater into electricity and heat. This approach not only provides a sustainable energy source but also reduces landfill waste, lowers disposal costs, and promotes environmental responsibility.
Additionally, blockchain-based energy trading platforms are being piloted in smart hotels, enabling resorts to buy and sell excess renewable energy directly within a decentralized energy market. This allows hotels to participate in peer-to-peer energy trading, further enhancing their energy independence and profitability.
Another major development is the use of hydrogen fuel cells and green hydrogen production as an alternative to fossil fuels. Some luxury hotels are now installing hydrogen microgrids, which generate clean power on-site using hydrogen produced from solar-powered electrolysis. This zero-emission energy source is particularly valuable for resorts aiming to achieve carbon neutrality and energy security.
Advanced cooling technologies are also playing a key role in sustainable hotel energy solutions. Since HVAC systems account for a large portion of hotel energy consumption, properties are integrating radiant cooling, solar absorption cooling, and geothermal heat pumps to improve efficiency. Smart air conditioning systems equipped with AI-based predictive controls adjust temperatures based on occupancy, weather patterns, and guest preferences, further reducing energy waste.
Hotels are also leveraging wireless power transmission (WPT) to create a seamless guest experience. Resort suites, conference centers, and luxury villas are now integrating wireless charging stations, smart lighting, and contactless concierge systems powered by electromagnetic resonance and laser-based energy transfer technologies. These advancements eliminate traditional power infrastructure, allowing for aesthetic, clutter-free designs while improving energy distribution.
The integration of grid-interactive buildings is another key development shaping the future of hotel power plants. Smart hotels can now connect with the local energy grid, adjusting their power usage based on grid demand, energy prices, and renewable energy availability. This dynamic energy interaction allows hotels to reduce costs, stabilize local grids, and support community energy networks.
Ultimately, the future of small-scale power plants for hotels and resorts will be defined by decentralized renewable energy, AI-powered automation, advanced energy storage, and sustainable building integration. By embracing these technologies, hotels can achieve energy independence, reduce environmental impact, and enhance guest satisfaction, ensuring they remain at the forefront of the next-generation hospitality industry.
As the hospitality industry continues its shift towards sustainability and energy efficiency, hotels and resorts are embracing innovative energy solutions that not only reduce operational costs but also enhance guest experiences. The future of small-scale power plants for hotels will be characterized by the integration of smart technologies, alternative energy sources, and highly efficient energy management systems.
One of the most transformative innovations is the full automation of hotel energy systems using AI and machine learning algorithms. These intelligent systems can predict power demand fluctuations, optimize energy distribution, and adjust lighting, climate control, and other power-consuming systems in real time. For instance, AI-driven smart HVAC systems can learn guest preferences and adjust room temperatures automatically, ensuring maximum comfort while minimizing unnecessary energy use.
Edge computing and IoT-based sensors are also revolutionizing energy efficiency in hotels. These systems continuously collect and analyze real-time data on room occupancy, weather conditions, and grid energy availability, enabling dynamic load balancing. Hotels equipped with intelligent load management can reduce peak demand charges by automatically shifting non-essential loads—such as laundry operations, water heating, and pool filtration—to times when electricity rates are lower or when renewable energy production is at its peak.
For resorts and hotels aiming to achieve full energy self-sufficiency, modular renewable energy farms are emerging as a viable solution. These self-contained systems combine solar PV arrays, vertical-axis wind turbines, micro-hydro units, and bioenergy generators, all integrated with AI-powered microgrid controllers. These modular setups provide scalability and adaptability, allowing resorts to expand energy capacity as demand grows, without major infrastructure changes.
A major breakthrough in sustainable hospitality energy is the use of thermal energy storage (TES) systems. These innovative storage solutions allow hotels to store excess solar or waste heat energy in phase-change materials (PCMs), molten salts, or underground reservoirs, which can later be used for space heating, hot water production, or cooling through absorption chillers. Resorts in hot climates are increasingly adopting TES systems to reduce dependency on conventional air conditioning, which is a significant energy drain.
Another cutting-edge approach is the implementation of direct air carbon capture (DAC) technology in luxury eco-resorts. This system not only removes CO₂ from the atmosphere but also converts it into renewable synthetic fuels or stores it underground, helping hotels achieve negative carbon emissions while generating clean energy. Paired with algae-based biofuel production, hotels can now create on-site renewable fuel sources for backup generators, shuttle fleets, and cooking appliances.
The shift towards hydrogen economy is also making waves in the hospitality sector. Hotels are increasingly adopting hydrogen fuel cells to generate on-site power, either by storing surplus renewable energy as green hydrogen or by participating in hydrogen microgrid clusters. These systems provide zero-emission power, ensuring energy security and sustainability, especially for resorts in regions with unreliable grid access.
For waterfront resorts and coastal hotels, wave energy converters (WECs) and floating solar farms are becoming viable solutions to harness ocean energy. Some properties are now using artificial lagoons with integrated underwater turbines, generating power from tidal flows and kinetic wave movement while simultaneously creating scenic and recreational water features for guests.
The development of bioelectrogenic systems is another futuristic concept gaining traction. These microbial fuel cells utilize bacteria to generate electricity from organic waste or wastewater, turning a hotel’s own waste streams into a reliable power source. This technology, combined with advanced greywater recycling, allows hotels to operate more sustainably while reducing water and energy consumption.
As hotels move towards carbon neutrality, they are also incorporating regenerative energy systems, such as piezoelectric flooring and kinetic energy harvesting walkways. These systems capture the energy produced by guest footsteps, elevators, and even luggage carts, converting it into usable electricity for lighting, signage, and small appliances. Some high-tech resorts have even introduced fitness equipment-powered generators, where guests can contribute to the hotel’s energy grid by pedaling stationary bikes or running on treadmills.
With the rise of decentralized finance (DeFi) and tokenized carbon credits, blockchain-based green energy marketplaces are allowing hotels to track, trade, and verify their renewable energy contributions in real-time. Some luxury eco-resorts are even launching guest-driven sustainability programs, where visitors can purchase carbon offsets, contribute to reforestation projects, or directly invest in the hotel’s renewable energy expansions through tokenized assets.
Ultimately, the future of small-scale power plants for hotels and resorts will be shaped by intelligent automation, decentralized energy production, and hyper-efficient energy storage solutions. By embracing AI-driven microgrids, alternative energy harvesting methods, and regenerative power sources, hotels will not only achieve greater energy independence but also enhance the guest experience, reduce operating costs, and contribute to a greener, more sustainable world.
Small Scale Power Plant for Commercial Office Buildings
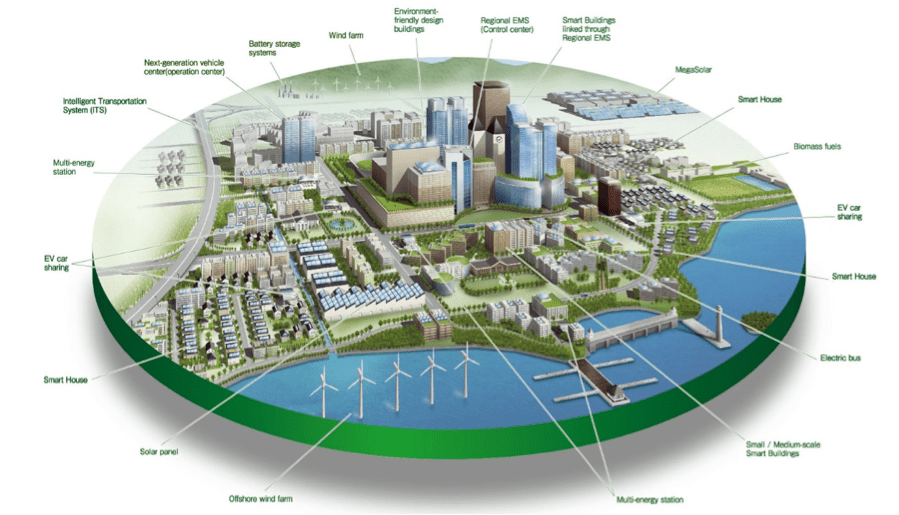
Small-scale power plants for commercial office buildings are evolving rapidly as businesses prioritize energy efficiency, sustainability, and resilience. Modern office buildings require stable, uninterrupted power to support IT infrastructure, HVAC systems, lighting, security, and workplace automation. Additionally, with the rise of remote work, cloud computing, and AI-powered smart offices, commercial buildings are seeing increased electricity demands while striving to reduce operational costs and carbon footprints.
To address these challenges, commercial office buildings are increasingly turning to hybrid microgrids that integrate renewable energy sources, battery storage, and intelligent energy management systems. These decentralized power solutions allow buildings to generate, store, and distribute electricity more efficiently while reducing dependency on the main grid.
One of the most widely adopted solutions is the Building-Integrated Photovoltaics (BIPV) system, where solar panels are seamlessly incorporated into windows, facades, and rooftops. Unlike traditional rooftop solar panels, BIPV technology enables commercial buildings to maximize solar energy generation without compromising aesthetics or usable space. Some office towers are also utilizing transparent solar glass, which allows natural light to pass through while simultaneously generating electricity.
Another major innovation in office building energy systems is the use of Combined Heat and Power (CHP) and Trigeneration (CCHP) systems. These solutions not only generate electricity but also capture and repurpose waste heat for space heating, hot water, and even cooling via absorption chillers. This approach significantly improves overall energy efficiency, reducing utility costs while enhancing sustainability efforts.
To further enhance resilience, office buildings are incorporating battery energy storage systems (BESS), which allow businesses to store excess renewable energy and deploy it during peak hours or grid outages. Advanced storage technologies, such as solid-state batteries, vanadium flow batteries, and graphene-based supercapacitors, provide higher energy density, longer lifespans, and faster charging capabilities, making them ideal for commercial applications.
Additionally, the rise of hydrogen fuel cells and decentralized hydrogen microgrids is providing commercial buildings with a zero-emission backup power alternative to diesel generators. Some office parks are adopting green hydrogen production, where excess solar or wind energy is used to generate hydrogen fuel through electrolysis, which can then be stored and converted back into electricity as needed.
With the increasing adoption of smart buildings and AI-driven automation, office power plants are now incorporating real-time energy management systems (EMS). These platforms use machine learning algorithms and IoT-connected sensors to monitor energy consumption, predict demand fluctuations, and optimize power distribution. For example, AI-driven EMS can automatically adjust lighting, HVAC, and plug loads based on occupancy levels, weather patterns, and grid energy availability—resulting in significant energy savings and improved workplace comfort.
For office buildings aiming for carbon neutrality, biogas and waste-to-energy (WTE) systems are being explored as sustainable alternatives. Organic waste from cafeterias, landscaping, and office operations can be processed through anaerobic digestion or advanced gasification, producing biogas and syngas that can be converted into electricity. This approach not only reduces waste disposal costs but also provides an on-site renewable energy source.
Another growing trend is the implementation of grid-interactive buildings, which allow commercial properties to participate in demand response programs, trade excess energy, and support grid stability. By dynamically adjusting energy consumption based on grid conditions, peak demand pricing, and renewable energy availability, office buildings can lower energy costs while contributing to a more balanced energy ecosystem.
Furthermore, high-efficiency cooling technologies, such as radiant cooling, geothermal heat pumps, and phase-change thermal storage, are helping commercial buildings reduce air conditioning energy consumption, which is a major cost driver. Some smart office towers are now integrating AI-controlled ventilation systems that optimize airflow, humidity, and temperature in real time, improving both energy efficiency and indoor air quality.
Looking toward the future, wireless power transmission (WPT) and inductive charging could revolutionize how commercial offices manage electricity distribution. Emerging technologies in electromagnetic resonance and laser-based power transfer could allow for seamless, cable-free energy distribution to desktops, IoT devices, and even EV charging stations within office premises.
Ultimately, small-scale power plants for commercial office buildings are moving towards decentralized, AI-optimized, and highly efficient energy systems. By integrating renewable energy, energy storage, and intelligent automation, modern office buildings can achieve energy independence, cost savings, and a reduced environmental footprint, making them more resilient, sustainable, and future-ready.
As the adoption of small-scale power plants in commercial office buildings continues to expand, the focus is shifting towards self-sufficient, intelligent, and adaptive energy ecosystems. Modern office buildings are being designed to function as energy hubs, where power generation, consumption, and storage are all seamlessly managed through advanced technologies.
One of the most significant trends is the implementation of AI-powered energy optimization systems. These intelligent platforms analyze historical energy usage, weather forecasts, occupancy levels, and grid conditions to dynamically adjust power distribution, HVAC operations, and lighting schedules. By continuously learning and adapting, AI-driven systems enable real-time energy efficiency improvements, reducing both operational costs and carbon footprints.
Advanced energy storage solutions are also revolutionizing commercial power plants. The latest breakthroughs in solid-state batteries, zinc-air batteries, and iron-flow batteries offer higher energy density, improved safety, and longer lifespan compared to conventional lithium-ion options. These systems store excess renewable energy during off-peak hours and deploy it when demand is high, ensuring a stable and resilient power supply.
To further enhance sustainability, office buildings are integrating Building-Integrated Wind Turbines (BIWTs), which are compact, low-noise vertical-axis wind turbines designed to function in urban environments. These systems capture wind energy at different altitudes and are particularly effective for skyscrapers and high-rise commercial buildings with strong wind exposure.
Hydrogen-based power solutions are also gaining momentum, with some commercial office buildings incorporating hydrogen fuel cells as backup power sources. In addition, buildings are exploring on-site hydrogen production through solar-driven electrolysis, allowing them to store surplus renewable energy as hydrogen fuel for later use. This approach provides a clean, long-term energy storage solution with zero emissions.
For cooling and heating efficiency, advanced phase-change materials (PCMs) are being incorporated into building structures to store and release thermal energy as needed. This passive approach to temperature regulation significantly reduces reliance on traditional HVAC systems while maintaining a comfortable indoor climate.
In addition to power generation, modern office buildings are embracing smart grid connectivity to become active participants in decentralized energy markets. By leveraging blockchain-based energy trading platforms, buildings can sell excess solar or stored energy to neighboring properties, creating localized, peer-to-peer energy networks that increase overall efficiency.
Another transformative innovation is the use of kinetic energy harvesting within office spaces. Floors, staircases, and even ergonomic office furniture can now be embedded with piezoelectric materials that convert foot traffic and movement into usable electricity. This concept is being implemented in high-traffic areas such as lobbies, hallways, and elevators, contributing to the building’s overall energy supply.
Looking ahead, the future of small-scale power plants for commercial office buildings will be defined by hyper-efficient, AI-managed, and decentralized energy systems. Through the combination of on-site renewables, intelligent storage solutions, and real-time energy optimization, office buildings will not only achieve energy independence but also play a crucial role in stabilizing urban energy grids and reducing overall carbon emissions.
As the evolution of small-scale power plants for commercial office buildings continues, the integration of next-generation technologies is shaping a future where buildings are not just energy-efficient but also fully autonomous energy hubs. The convergence of renewable energy, AI-driven management, advanced storage solutions, and smart grid interaction is redefining how office buildings generate, distribute, and consume power.
One of the most revolutionary advancements is the development of quantum dot solar cells and perovskite-based photovoltaics. These cutting-edge materials significantly enhance the efficiency of building-integrated solar panels, allowing office towers to generate more energy from less space. Transparent solar windows embedded with quantum dots can capture specific wavelengths of light, ensuring maximum energy conversion while maintaining natural lighting. This advancement transforms entire building facades into power-generating assets, making skyscrapers and office parks almost self-sufficient in electricity production.
Another emerging solution is artificial intelligence (AI)-assisted energy trading, where buildings autonomously buy and sell electricity based on real-time pricing and demand. Blockchain-powered smart contracts enable direct energy transactions between office buildings and local businesses, creating decentralized urban energy markets. This means a building with excess solar power during the day can sell electricity to nearby offices or data centers, while purchasing cheaper off-peak energy when needed.
To further enhance energy security, gravity-based energy storage systems (GBES) are being piloted in commercial high-rises. These systems use excess renewable power to lift heavy weights, storing energy in gravitational potential form. When energy is needed, the weights descend, driving generators and producing electricity. Unlike chemical batteries, gravity storage is non-degradable, maintenance-free, and 100% recyclable, making it a promising alternative for long-term energy storage.
The rise of wireless power distribution is also revolutionizing commercial office spaces. Resonant inductive coupling and laser-based energy transfer allow office devices, lighting systems, and even electric vehicles to charge wirelessly, eliminating cables and infrastructure bottlenecks. In smart office environments, this means desks, conference tables, and shared workspaces can be equipped with built-in wireless power zones, reducing clutter and improving workplace efficiency.
For enhanced cooling efficiency, liquid-air energy storage (LAES) is gaining traction. This system converts excess electricity into liquid air, which is stored at cryogenic temperatures. When needed, the liquid air expands, driving turbines to produce electricity. Since LAES systems double as cooling solutions, they provide both power storage and temperature regulation, reducing overall HVAC loads in commercial buildings.
As sustainable urbanization accelerates, commercial office buildings are also experimenting with living walls and bio-photovoltaic (BPV) energy systems. These vertical gardens, integrated with algae-based bioreactors, can absorb CO₂ while generating small amounts of electricity through microbial fuel cell technology. Not only do they provide clean air and noise reduction, but they also contribute to the building’s energy supply in an eco-friendly manner.
Finally, AI-driven demand-side management (DSM) platforms are enabling office buildings to respond dynamically to grid fluctuations. By predicting power demand surges, buildings can adjust HVAC settings, dim lighting, and shift computing loads in real-time, stabilizing the local energy grid while reducing costs. This level of automation ensures that commercial buildings are not just energy consumers, but also intelligent energy contributors that help prevent blackouts and peak demand crises.
Looking forward, the future of small-scale power plants for office buildings will be defined by hyper-efficient, AI-managed, and grid-interactive systems. By integrating advanced renewables, wireless power, intelligent storage, and decentralized energy trading, commercial buildings will become autonomous power centers, driving sustainability, reducing costs, and reshaping the future of corporate energy management.
Small Scale Power Plant for Industrial Parks
Small-scale power plants for industrial parks are transforming the way manufacturing hubs, logistics centers, and high-energy-demand facilities manage their electricity needs. Unlike commercial buildings, industrial parks require high-capacity, stable, and continuous power to support heavy machinery, automated production lines, data centers, and energy-intensive processes. As industries move towards energy independence, carbon neutrality, and smart automation, integrated microgrids, on-site renewable generation, and advanced storage systems are becoming essential components of modern industrial power solutions.
One of the most significant developments is the adoption of hybrid microgrids that combine solar, wind, biomass, and waste-to-energy solutions with battery storage and hydrogen fuel cells. These distributed energy systems allow industrial parks to generate and manage their own electricity, reducing dependence on external grids and minimizing the risk of blackouts, supply disruptions, and price volatility. With real-time AI-driven energy management systems, industrial plants can automatically switch between energy sources, prioritize low-cost power, and optimize consumption patterns for maximum efficiency and cost savings.
For industrial zones with high thermal energy needs, Combined Heat and Power (CHP) and Trigeneration (CCHP) systems are playing a crucial role. These systems simultaneously generate electricity, heat, and cooling from a single energy source, such as natural gas, biogas, or waste heat recovery. By capturing and reusing heat that would otherwise be wasted, industrial parks can increase overall energy efficiency to over 80%, significantly lowering fuel costs and carbon emissions.
Another game-changer in industrial energy is the expansion of green hydrogen production and storage. Hydrogen is emerging as a clean fuel for industrial processes, particularly in sectors like steel manufacturing, chemical production, and heavy transport. By utilizing on-site electrolysis powered by renewables, industrial parks can produce green hydrogen, store it for later use, and deploy it through fuel cells or hydrogen-powered machinery, eliminating reliance on fossil fuels.
Large-scale energy storage systems (ESS) are also evolving to meet the needs of industrial parks. Technologies like solid-state batteries, vanadium redox flow batteries (VRFBs), and compressed air energy storage (CAES) enable factories to store excess solar or wind energy and use it during peak demand hours. This not only lowers electricity costs but also provides a buffer against grid fluctuations and outages.
Beyond electricity generation, industrial parks are adopting waste-to-energy (WTE) systems to convert organic and industrial waste into usable power. Through anaerobic digestion, gasification, and plasma arc technology, facilities can turn factory waste, agricultural byproducts, and municipal refuse into biogas or synthetic fuels, reducing waste disposal costs while generating on-site renewable energy.
For energy-intensive operations, AI-driven energy management platforms are playing a vital role in optimizing power consumption. These systems use machine learning and real-time IoT sensor networks to predict demand spikes, automate energy distribution, and reduce unnecessary loads. For example, an AI-powered system can schedule high-energy machinery to operate during low-cost electricity periods, or dynamically adjust lighting, cooling, and compressed air usage based on production levels.
Industrial parks are also integrating high-voltage direct current (HVDC) microgrids, which offer lower transmission losses, improved power quality, and enhanced grid stability compared to traditional alternating current (AC) grids. HVDC technology is especially beneficial for multi-factory industrial zones, allowing facilities to share and balance energy loads more efficiently while enabling direct integration of renewable energy sources.
Another breakthrough is the development of gravity-based energy storage and kinetic energy recovery systems. Some industrial parks are now using massive crane-operated weight systems, which store energy by lifting heavy blocks and release it when needed by lowering them. Similarly, regenerative braking systems installed in automated conveyor belts, heavy-duty cranes, and factory robotics can capture kinetic energy and feed it back into the microgrid.
As industries move toward carbon neutrality, some industrial parks are adopting direct air carbon capture (DAC) technology, which removes CO₂ from the atmosphere and converts it into synthetic fuels or building materials. Paired with renewable energy generation and hydrogen storage, DAC can help offset industrial emissions while providing low-carbon energy alternatives for operations.
Looking forward, small-scale power plants for industrial parks will continue to evolve with AI-driven automation, hydrogen-based solutions, decentralized energy trading, and breakthrough storage technologies. By transitioning towards self-sufficient, resilient, and highly efficient energy ecosystems, industrial zones will reduce operational costs, enhance energy security, and contribute to a cleaner, more sustainable manufacturing future.
As industrial parks continue their transition towards self-sustaining energy ecosystems, the next wave of innovations is focused on enhancing resilience, reducing energy waste, and integrating cutting-edge smart technologies. Industrial zones are not only looking for ways to decarbonize operations but also to boost efficiency and lower costs through real-time energy optimization, advanced automation, and smart grid integration.
One of the key advancements shaping the future of small-scale power plants for industrial parks is the adoption of Artificial Intelligence (AI)-driven energy orchestration. AI-powered platforms use big data analytics, predictive modeling, and machine learning to optimize every aspect of an industrial park’s energy use. These systems continuously analyze electricity demand, grid conditions, production schedules, and weather forecasts to dynamically adjust power generation and consumption. This ensures that factories operate at peak efficiency, avoiding unnecessary energy expenses and maximizing renewable energy utilization.
Blockchain-enabled energy trading networks are also gaining traction in industrial parks. These systems allow different facilities within an industrial zone to buy, sell, and exchange electricity based on real-time supply and demand. A solar-powered warehouse that generates excess electricity during the day, for instance, can sell surplus power to a data center operating at night. By leveraging smart contracts and decentralized transactions, this approach reduces reliance on central utilities, enhances energy security, and creates a flexible, cost-efficient power-sharing ecosystem.
For energy storage, industrial parks are increasingly turning to liquid metal batteries and sodium-ion battery technology, which offer longer lifespans, lower costs, and greater resilience compared to traditional lithium-ion systems. These advanced storage solutions enable round-the-clock operation of renewable energy sources, ensuring that factories maintain an uninterrupted power supply even when solar or wind generation fluctuates.
Another critical innovation is high-efficiency hydrogen integration. Industrial parks are now deploying hydrogen microgrids, where excess renewable energy is converted into green hydrogen through electrolysis and stored for later use. This hydrogen can then be used to fuel industrial machinery, power hydrogen turbines, or supply fuel cells for backup energy. Some industrial parks are even exploring ammonia-based energy storage, which allows hydrogen to be safely transported and used on demand without the challenges of traditional hydrogen storage.
Superconducting power cables are also being introduced to reduce energy losses in industrial parks. Unlike conventional power transmission lines, superconductors can transport large amounts of electricity with nearly zero resistance, significantly improving energy efficiency and reducing heat losses. These cables enable industrial facilities to transfer power over long distances without voltage drops, making them ideal for large-scale manufacturing zones.
For heavy industries, waste heat recovery (WHR) and thermoelectric generators (TEGs) are being integrated to capture excess heat from industrial processes and convert it into electricity. Instead of allowing heat from furnaces, boilers, and turbines to dissipate, these technologies recycle thermal energy, improving overall energy efficiency while reducing cooling costs.
To further improve sustainability, industrial parks are also investing in microbial fuel cells (MFCs) and bioenergy solutions. These systems use biological processes to generate electricity from organic waste and wastewater, providing a dual benefit of energy production and waste treatment. Large-scale manufacturing parks with significant wastewater output can turn organic pollutants into power, creating a closed-loop energy cycle that minimizes environmental impact.
The next phase in industrial park electrification will also see the rise of wireless power transmission. Technologies such as resonant inductive coupling and microwave energy beaming are being developed to allow factories to transmit power without physical cables, reducing infrastructure complexity and enhancing operational flexibility. This innovation will be particularly useful for automated factories and robotic production lines, where equipment can receive power wirelessly, eliminating the need for fixed electrical connections.
Looking ahead, the integration of next-generation energy storage, AI-powered grid management, decentralized power trading, and advanced waste-to-energy solutions will make small-scale power plants in industrial parks more autonomous, resilient, and cost-effective. As energy technology continues to evolve, industrial zones will shift from traditional grid-dependent models to fully intelligent, self-regulating power ecosystems, ensuring a sustainable, high-efficiency future for global manufacturing and logistics.
The future of small-scale power plants for industrial parks is rapidly advancing toward fully autonomous, AI-driven, and highly efficient energy ecosystems. These next-generation industrial energy solutions will not only focus on reducing operational costs and improving resilience but also on maximizing sustainability, decentralization, and real-time energy optimization.
One of the most cutting-edge innovations transforming industrial parks is the rise of AI-powered energy autonomy. These smart energy management systems, integrated with real-time digital twins, allow industrial zones to simulate, predict, and optimize power flows with near-perfect accuracy. By continuously analyzing grid conditions, weather patterns, machine operations, and energy storage levels, AI ensures that industrial parks operate at maximum efficiency with minimal waste.
In addition to AI, the deployment of next-generation solid-state batteries and gravity-based energy storage is eliminating traditional energy bottlenecks. Unlike lithium-ion batteries, solid-state batteries offer higher energy density, faster charging times, and enhanced safety, making them an ideal solution for heavy industrial applications. Similarly, gravity-based energy storage (GBES) enables industrial parks to store and release large amounts of energy without relying on chemical degradation, making it a long-term, cost-effective energy solution.
The rise of industrial-scale hydrogen ecosystems is another game-changer. Industrial parks are now integrating on-site hydrogen production, storage, and utilization to power high-temperature manufacturing, heavy machinery, and industrial vehicles. The development of high-efficiency hydrogen fuel cells, ammonia-based hydrogen storage, and hydrogen combustion turbines is making it possible for industrial parks to operate independently of fossil fuels, drastically reducing carbon emissions while maintaining high-power industrial processes.
To further enhance energy independence, industrial parks are adopting direct-current (DC) microgrids in place of traditional AC grids. DC-based energy networks are more efficient, require fewer conversion steps, and reduce transmission losses, making them particularly valuable for data centers, automated factories, and industrial robotics. These next-generation smart microgrids are designed to dynamically adjust power allocation, ensuring that energy flows only where it is needed, eliminating waste and optimizing efficiency.
Beyond traditional renewables, industrial parks are also harnessing geothermal and advanced bioenergy solutions. Deep geothermal wells allow for continuous, high-efficiency heat and power generation, while next-gen bioenergy plants convert agricultural, industrial, and municipal waste into synthetic fuels, hydrogen, and bioelectricity. These systems create circular energy economies, where waste is transformed into valuable power sources, reducing both operational costs and environmental impact.
Another emerging technology is the integration of wireless power transmission across industrial sites. Using resonant inductive coupling and laser-based energy transfer, factories can power machinery, automated transport systems, and mobile robotics without physical cables, increasing flexibility and reducing infrastructure maintenance. This is particularly useful in smart factories where robotic systems require constant movement and reconfiguration.
At the grid level, blockchain-based decentralized energy markets are allowing industrial parks to trade excess power, store energy as digital assets, and participate in real-time energy price arbitrage. This means that industrial zones can sell surplus solar, wind, or hydrogen-generated power to nearby facilities or store energy during off-peak hours to be used later at lower costs. This approach ensures stable energy pricing, greater financial control, and a more balanced grid load.
To further reduce industrial carbon footprints, direct air carbon capture (DAC) technologies are being integrated into power plants within industrial parks. These systems capture CO₂ emissions directly from industrial exhaust and ambient air, converting them into synthetic fuels, construction materials, or stored underground. When combined with hydrogen electrolysis and renewable power generation, DAC can offset emissions from even the most energy-intensive industries, helping industrial parks move toward net-zero operations.
Looking into the near future, industrial parks will become self-regulating, AI-managed, and energy-autonomous ecosystems, where renewables, hydrogen, AI-driven grids, and advanced storage solutions seamlessly interact to create a resilient, high-performance, and sustainable industrial power network. These advancements will not only reduce dependency on traditional power grids but will also enable industrial zones to become key contributors to national and regional energy stability, ensuring a smoother transition to a fully decarbonized economy.
Small Scale Power Plant for Remote Villages
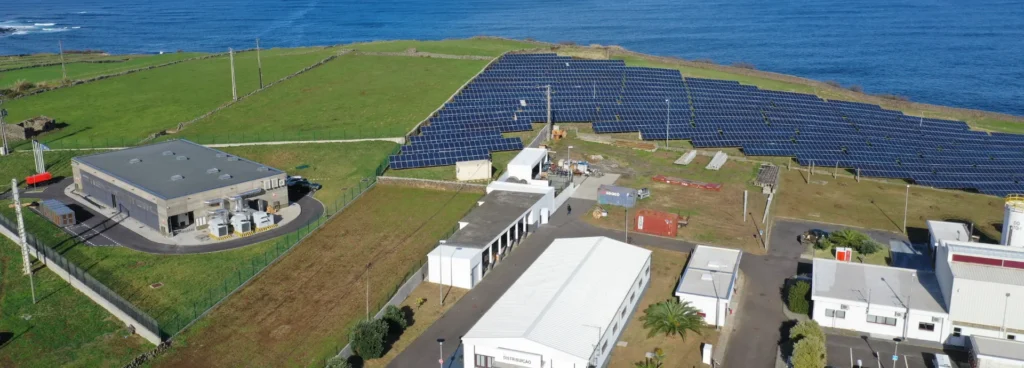
Small-scale power plants for remote villages are revolutionizing how off-grid and underserved communities gain access to reliable, sustainable, and affordable electricity. Unlike urban centers connected to national grids, many remote villages face severe energy poverty, relying on kerosene, diesel generators, or biomass for basic energy needs. However, advancements in distributed renewable energy, microgrids, and innovative storage solutions are transforming these villages into self-sufficient energy hubs, improving quality of life, economic development, and environmental sustainability.
One of the most effective solutions for remote villages is the development of solar-powered microgrids. These systems consist of solar panels, battery storage, and smart energy distribution networks, allowing entire communities to share electricity efficiently. Advances in perovskite solar cells and bifacial photovoltaic panels are increasing energy generation, even in low-sunlight regions, ensuring year-round power availability. With the integration of machine learning-based demand forecasting, microgrids can dynamically adjust power allocation, prioritizing essential services such as lighting, healthcare facilities, schools, and water pumps.
In addition to solar, wind and small-scale hydro power are being deployed to supplement energy generation in remote areas. Vertical-axis wind turbines (VAWTs) are gaining popularity due to their ability to generate power at low wind speeds, making them ideal for coastal, hilly, and desert regions. For villages located near rivers, run-of-river hydro systems provide a continuous and stable source of electricity without the need for large dams, preserving local ecosystems and water access.
Energy storage remains a crucial challenge, and emerging solutions such as sodium-ion batteries, zinc-air batteries, and iron-flow batteries are replacing costly and short-lived lead-acid alternatives. These new storage technologies are cheaper, non-toxic, and highly durable, allowing remote communities to store excess solar and wind energy for nighttime and cloudy days. In some areas, gravity-based energy storage—where excess energy lifts heavy weights, which later descend to generate electricity—provides an ultra-low-maintenance, long-term backup solution.
To reduce dependence on expensive diesel fuel, villages are increasingly adopting biomass gasification and waste-to-energy systems. These technologies convert organic waste, agricultural residues, and animal dung into biogas or syngas, which can be used for cooking, heating, and electricity production. By recycling waste into energy, villages not only reduce pollution but also create economic opportunities in biofuel production and distribution.
One of the most innovative developments is the use of community-driven blockchain energy trading platforms. In many remote villages, households with solar panels and battery storage can sell excess power to their neighbors via peer-to-peer (P2P) energy markets. This decentralized approach empowers communities to manage their own electricity, ensuring that every household has access to affordable power while eliminating the need for costly centralized infrastructure.
For water-scarce regions, solar desalination and water-powered energy systems are improving both energy access and clean water availability. Advanced solar stills, atmospheric water generators (AWGs), and desalination microgrids use excess renewable energy to extract drinking water from the air or purify saline water, ensuring that communities have both electricity and sustainable water sources.
In healthcare, small-scale power plants are saving lives by ensuring continuous electricity supply for rural hospitals and clinics. The integration of solar refrigeration, battery-powered medical devices, and AI-assisted telemedicine is bringing modern healthcare services to the most remote locations. With satellite-powered internet and low-energy diagnostic equipment, doctors can perform remote consultations, AI-based disease detection, and vaccine storage without relying on unstable power grids.
Education is also benefiting from electrification efforts, as schools in remote villages now have access to internet-powered learning, digital classrooms, and e-library systems. Solar-powered community learning hubs are emerging as centers for skills training, entrepreneurship development, and online education, helping rural populations participate in the digital economy.
Looking ahead, remote village electrification will continue to be shaped by solar-hydrogen hybrid systems, AI-powered microgrids, decentralized energy trading, and innovative storage technologies. These advancements will create self-reliant, resilient, and environmentally friendly communities, eliminating energy poverty while boosting rural development and economic opportunities.
EMS Power Machines
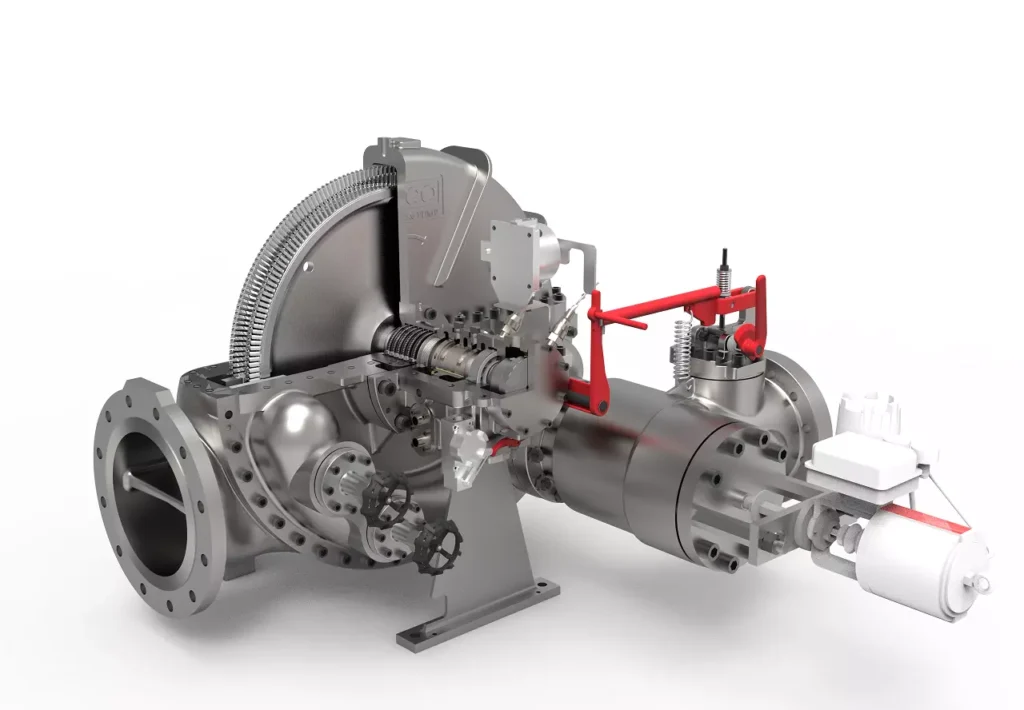
We design, manufacture and assembly Power Machines such as – diesel generators, electric motors, vibration motors, pumps, steam engines and steam turbines
EMS Power Machines is a global power engineering company, one of the five world leaders in the industry in terms of installed equipment. The companies included in the company have been operating in the energy market for more than 60 years.
EMS Power Machines manufactures steam turbines, gas turbines, hydroelectric turbines, generators, and other power equipment for thermal, nuclear, and hydroelectric power plants, as well as for various industries, transport, and marine energy.
EMS Power Machines is a major player in the global power industry, and its equipment is used in power plants all over the world. The company has a strong track record of innovation, and it is constantly developing new and improved technologies.
Here are some examples of Power Machines’ products and services:
- Steam turbines for thermal and nuclear power plants
- Gas turbines for combined cycle power plants and industrial applications
- Hydroelectric turbines for hydroelectric power plants
- Generators for all types of power plants
- Boilers for thermal power plants
- Condensers for thermal power plants
- Reheaters for thermal power plants
- Air preheaters for thermal power plants
- Feedwater pumps for thermal power plants
- Control systems for power plants
- Maintenance and repair services for power plants
EMS Power Machines is committed to providing its customers with high-quality products and services. The company has a strong reputation for reliability and innovation. Power Machines is a leading provider of power equipment and services, and it plays a vital role in the global power industry.
EMS Power Machines, which began in 1961 as a small factory of electric motors, has become a leading global supplier of electronic products for different segments. The search for excellence has resulted in the diversification of the business, adding to the electric motors products which provide from power generation to more efficient means of use.