
Exhaust Back Pressure: A 50 kW power generator is a critical piece of equipment used in various industries, residential settings, and in emergency power supply situations. As a medium-sized generator, it provides sufficient power for small to medium commercial facilities or large residential properties. The role of generators has evolved significantly in recent years, particularly as power reliability becomes a growing concern for both consumers and businesses. The demand for reliable, efficient, and versatile generators has surged with the rising costs of energy and frequent occurrences of power outages due to natural disasters or grid failures.
This section explores the technical aspects, advantages, common applications, maintenance needs, and environmental considerations associated with 50 kW generators. We’ll also delve into the advancements in technology that have made modern generators more efficient and environmentally friendly, as well as the key factors to consider when choosing a generator of this size.
Technical Specifications of a 50 kW Generator
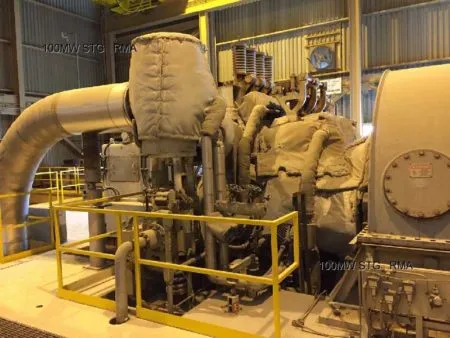
At its core, a 50 kW generator is designed to convert mechanical energy into electrical energy. Typically powered by diesel, natural gas, or propane, these generators are capable of producing 50 kilowatts of continuous electrical power, sufficient for small industrial applications, backup power for businesses, or off-grid locations.
Some of the key technical components include:
- Engine: The prime mover of the generator. For a 50 kW unit, it typically features a robust engine, often fueled by diesel, natural gas, or propane.
- Alternator: Converts mechanical energy from the engine into electrical energy.
- Fuel System: Ensures a continuous flow of fuel to the engine. The choice of fuel impacts the generator’s efficiency and emissions.
- Cooling System: Ensures the engine does not overheat during operation.
- Control Panel: Allows for monitoring and managing the generator’s operation, often equipped with automatic start-stop features and fault indicators.
- Exhaust System: Directs harmful emissions away from the generator and provides necessary filtration to meet environmental regulations.
The size of the engine, the quality of the alternator, and the control features will vary based on manufacturer and model, but for a 50 kW generator, reliability is key.
Fuel Options and Efficiency
One of the major decisions when choosing a generator is the type of fuel it will use. Fuel type significantly impacts operational efficiency, maintenance requirements, and environmental impact. Let’s explore some of the most common fuel options available for 50 kW generators:
Diesel-Powered Generators
Diesel is the most common fuel choice for 50 kW generators. Diesel engines are known for their durability and fuel efficiency. They offer a higher energy density than natural gas, which means diesel generators are often more efficient in terms of fuel consumption. Diesel-powered generators also require less frequent maintenance, making them popular in industrial and commercial settings where continuous operation is required.
However, diesel generators do produce more emissions compared to their gas counterparts, which makes them less environmentally friendly. Many modern diesel generators, though, are equipped with advanced emission control systems, such as diesel particulate filters (DPF) and selective catalytic reduction (SCR) systems, to meet stringent environmental regulations.
Natural Gas-Powered Generators
Natural gas is another popular fuel choice for 50 kW generators. Natural gas generators are cleaner than diesel in terms of emissions, producing fewer particulates and carbon dioxide. These generators are commonly used in residential and urban areas where environmental concerns and noise restrictions are a factor.
Natural gas generators are often connected to the local gas grid, providing a continuous and reliable fuel supply, which makes them ideal for backup power situations. However, natural gas generators typically have a lower energy density than diesel, which means they may not be as efficient in some applications.
Propane-Powered Generators
Propane is a versatile and clean-burning fuel option for 50 kW generators. It is especially useful in off-grid locations where access to diesel or natural gas is limited. Propane generators are similar in operation to natural gas units but are slightly more efficient due to the higher energy content of propane.
While propane generators offer environmental benefits and reliable performance, they require more frequent fuel deliveries and careful management of storage tanks to ensure a consistent fuel supply.
Applications of 50 kW Generators
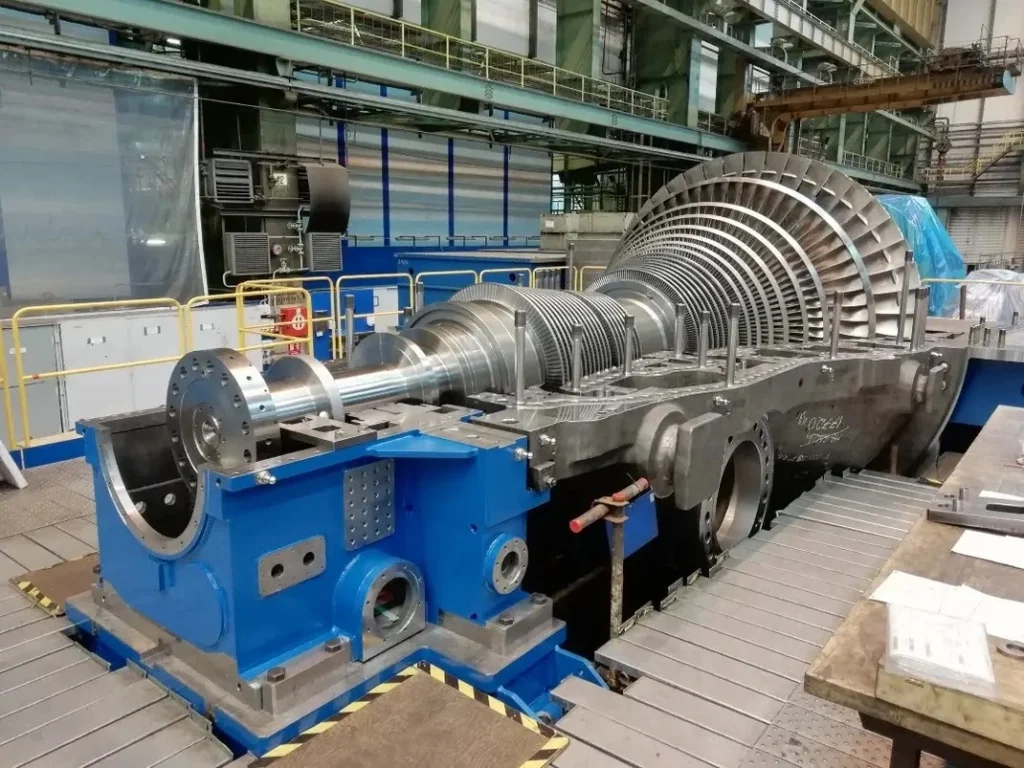
A 50 kW generator is versatile and can be used in a variety of settings. Some of the most common applications include:
Industrial Use
In small to medium-sized industrial operations, 50 kW generators can provide backup power for critical systems, such as computers, lighting, and security systems. These generators are also used in remote locations, such as construction sites, where access to the electrical grid may be limited or nonexistent.
Commercial Use
Businesses often rely on generators to maintain operations during power outages. A 50 kW generator can power essential equipment, lighting, HVAC systems, and communication networks. For small businesses, this can mean the difference between staying open during a power outage and losing significant revenue.
Residential Use
Larger residential properties or small clusters of homes can also benefit from a 50 kW generator. These generators can provide whole-house backup power, ensuring that essential systems, such as heating, cooling, refrigeration, and medical equipment, continue to function during an outage. Additionally, they are often used in off-grid homes where the public utility is not available.
Agricultural Use
Farmers often rely on 50 kW generators to power irrigation systems, barns, greenhouses, and other agricultural equipment. In rural areas where power outages are more common, having a reliable generator ensures that crops and livestock are protected and that essential farming operations continue uninterrupted.
Maintenance and Longevity
Regular maintenance is essential for the longevity and reliability of any generator, especially those intended for continuous or backup use. The primary maintenance tasks for a 50 kW generator include:
- Oil and Filter Changes: Regular oil changes are necessary to keep the engine running smoothly. Oil filter changes should also be performed at the same time to prevent debris from circulating through the engine.
- Fuel System Maintenance: Diesel and propane generators require fuel system inspections to ensure that there are no blockages or leaks. Natural gas generators, while typically cleaner, still need periodic inspections.
- Battery Maintenance: The battery system that powers the generator’s control panel and automatic start features must be checked regularly for charge and replaced as needed.
- Cooling System Checks: Ensuring the generator’s cooling system is functioning correctly prevents overheating. Coolant levels should be checked, and the radiator should be inspected for clogs or leaks.
- Exhaust System Inspections: The exhaust system should be regularly inspected for leaks or blockages that could impact performance or safety.
Properly maintained, a 50 kW generator can last between 15,000 and 30,000 hours of operation, depending on the manufacturer, usage patterns, and the quality of maintenance.
Environmental and Regulatory Considerations
Generators, especially those powered by diesel, are subject to environmental regulations aimed at reducing harmful emissions. In the United States, for example, the Environmental Protection Agency (EPA) sets standards for emissions from diesel generators, requiring modern units to include technologies that reduce nitrogen oxides (NOx) and particulate matter (PM). These regulations are important for both environmental protection and public health.
In addition to federal regulations, some local and state governments impose stricter controls on emissions, particularly in urban areas where air quality is a significant concern. Many modern 50 kW generators are designed to meet these stringent standards, offering advanced emissions controls to ensure compliance.
Cost Considerations
The cost of a 50 kW generator can vary significantly depending on the fuel type, brand, and additional features such as automatic transfer switches, remote monitoring, and advanced emissions controls. Diesel generators are typically more expensive upfront due to their robust construction and higher fuel efficiency, but they may offer lower operational costs over time. Natural gas and propane generators tend to have lower upfront costs but may require more frequent fuel deliveries or higher maintenance costs.
For businesses and homeowners, the cost of a generator should be weighed against the potential cost of lost productivity or property damage during a power outage. Investing in a high-quality 50 kW generator can provide peace of mind, knowing that essential systems will remain operational when the grid fails.
Conclusion
A 50 kW power generator is a reliable, efficient solution for medium-sized power needs in industrial, commercial, and residential applications. When choosing a generator, factors such as fuel type, efficiency, emissions, and maintenance requirements should all be carefully considered. With proper maintenance and regular inspections, a 50 kW generator can provide years of reliable service, ensuring that critical operations remain uninterrupted during power outages.
Unique Ways to Generate Electricity
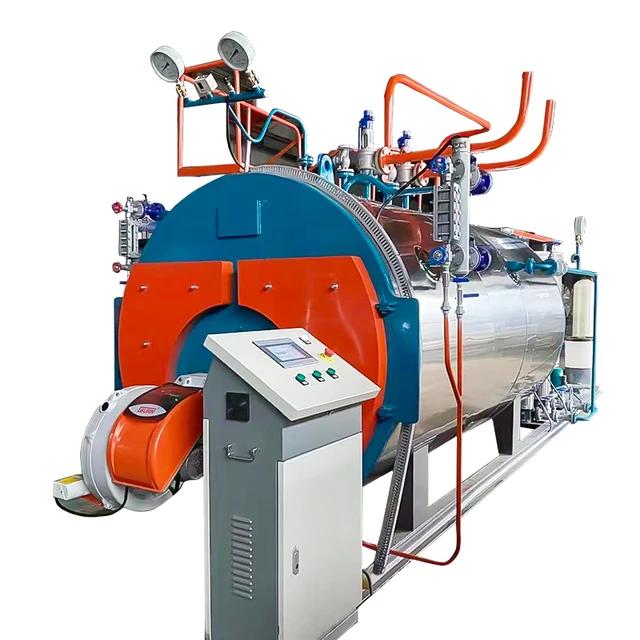
In an age of advancing technology and increasing environmental concerns, the search for sustainable, renewable, and innovative ways to generate electricity has gained global prominence. Traditional methods such as coal, oil, and natural gas have been the backbone of power generation for decades, but they come with significant environmental impacts. As the world grapples with climate change and the depletion of finite resources, the push toward more unique and sustainable methods of generating electricity has become critical.
This section delves into some of the most unconventional and innovative approaches to electricity generation, exploring technologies and concepts that have the potential to transform how we power our homes, businesses, and cities. These methods range from harnessing the natural forces of the earth to tapping into human energy and waste. They showcase the ingenuity and creativity driving the quest for a cleaner, greener future.
1. Piezoelectric Energy
Piezoelectricity is the ability of certain materials, such as quartz and ceramics, to generate an electric charge when subjected to mechanical stress. This technology is often used in small-scale applications like lighters or medical devices, but researchers are exploring its potential for large-scale electricity generation.
How Piezoelectric Energy Works
When pressure is applied to a piezoelectric material, the molecular structure of the material is deformed, causing a separation of electrical charges. This separation creates an electric potential, which can be captured and stored as electrical energy. This process can be repeated as long as the material is continuously subjected to stress.
Applications in Power Generation
Innovative uses of piezoelectric energy include embedding piezoelectric materials in flooring systems to capture the kinetic energy of people walking over them. For example, piezoelectric tiles could be installed in high-traffic areas such as shopping malls, airports, or train stations, generating small amounts of electricity from the foot traffic.
Another emerging concept is using piezoelectric materials in roadways to capture the mechanical energy of vehicles passing over them. While still in experimental stages, this approach could provide a supplementary energy source for street lighting, traffic signals, or electric vehicle charging stations.
2. Solar Updraft Tower
The solar updraft tower is a unique renewable energy concept that utilizes the sun’s heat to generate electricity. This technology is particularly effective in arid, sunny regions with high solar radiation levels.
How Solar Updraft Towers Work
A solar updraft tower consists of a large greenhouse-like structure at the base, which heats the air inside using the sun’s energy. As the hot air rises, it is funneled through a tall central tower. At the top of the tower, the rising air drives wind turbines, which generate electricity. The key advantage of this technology is that it can operate continuously, as the tower’s height allows for the capture of rising air even during the night, thanks to the retained heat in the ground.
Benefits and Challenges
One of the main benefits of the solar updraft tower is that it requires no external fuel source and has low operational costs once constructed. It also produces no emissions, making it an environmentally friendly option. However, the initial construction cost is high, and the large amount of land required makes it unsuitable for densely populated areas.
3. Kinetic Energy Harvesting from Footsteps
Kinetic energy harvesting is a novel concept where energy is captured from motion and converted into electrical power. One of the most promising applications of this technology involves harvesting energy from human footsteps.
How It Works
Kinetic energy harvesting devices are installed in areas with high foot traffic, such as sidewalks, sporting venues, or public transportation hubs. When people walk over these devices, the kinetic energy from their movement is converted into electricity through a combination of pressure sensors, piezoelectric materials, or other mechanical components.
Potential Applications
This technology is already being tested in several public spaces around the world. For example, in some European cities, kinetic tiles have been installed in pedestrian walkways, generating small amounts of electricity that can be used for powering streetlights or advertising displays. In the future, this method could be scaled up to provide supplemental power for buildings, bus stops, or charging stations for electronic devices.
While this method currently produces small amounts of electricity, it has potential in combination with other renewable sources to contribute to the overall energy mix in urban environments.
4. Tidal Energy
Tidal energy harnesses the power of ocean tides to generate electricity. As the tides rise and fall, the kinetic energy from the movement of water can be captured and converted into electrical power through a variety of technologies, such as tidal barrages, tidal turbines, and tidal fences.
Tidal Barrages
A tidal barrage is a dam-like structure built across the entrance of an estuary. As the tide flows in and out, water is trapped in the barrage and released through turbines, generating electricity. Tidal barrages have been used in places like France, where the La Rance Tidal Power Station has been operating successfully for decades.
Tidal Turbines
Tidal turbines operate similarly to wind turbines, but they are placed underwater to capture the energy of moving water. These turbines are typically installed in areas with strong tidal currents, such as narrow channels or around coastal headlands. Because water is much denser than air, tidal turbines can generate more electricity with smaller blades compared to wind turbines.
Challenges of Tidal Energy
While tidal energy is highly predictable and reliable, the main challenges are the high cost of installation and the potential environmental impacts on marine ecosystems. However, advances in turbine design and environmental mitigation strategies are making tidal energy a more viable option in the renewable energy mix.
5. Algae Biofuel
Algae, a simple aquatic organism, has shown great promise as a renewable energy source. Algae can be cultivated and processed into biofuel, which can be used to generate electricity or as an alternative to fossil fuels in transportation.
How Algae Biofuel Works
Algae grow rapidly and can be cultivated in a variety of environments, including freshwater, saltwater, or even in waste treatment facilities. Once harvested, algae can be processed to extract oils, which are then refined into biofuel. This biofuel can be burned in power plants to generate electricity or used as a replacement for traditional diesel or gasoline.
Advantages of Algae as a Power Source
One of the main advantages of algae biofuel is its ability to absorb large amounts of carbon dioxide (CO2) during growth, helping to mitigate greenhouse gas emissions. Additionally, algae can be grown on non-arable land and does not compete with food crops for resources. Algae biofuel is also highly versatile, as it can be used for power generation, transportation, or even in chemical production.
Challenges and Future Potential
While algae biofuel has great potential, challenges remain in terms of cost and scalability. Large-scale production of algae biofuel is still expensive compared to fossil fuels, but ongoing research is focused on reducing costs and improving efficiency. As technological advancements continue, algae biofuel may become a key player in the renewable energy landscape.
6. Geothermal Energy from Abandoned Mines
Abandoned mines, often considered environmental hazards, are being re-imagined as sources of geothermal energy. Geothermal energy is heat derived from the Earth’s internal layers, and it can be used to generate electricity or for direct heating purposes.
How It Works
Abandoned mines are often filled with water, which absorbs heat from the surrounding rock. This water can be pumped to the surface and used in a heat exchange process to generate electricity or to provide heating and cooling for buildings. Some abandoned coal mines, for example, have been repurposed as geothermal energy sources, helping to transform old industrial sites into renewable energy hubs.
Benefits
Using abandoned mines for geothermal energy generation has several benefits, including the reuse of existing infrastructure, reduction of environmental hazards, and the potential for year-round energy production. Geothermal energy is highly reliable, with minimal environmental impact once systems are in place.
Challenges
The primary challenge of geothermal energy from mines is the upfront cost of developing the necessary infrastructure. Additionally, not all mines are suitable for geothermal energy production, and site-specific studies must be conducted to assess viability.
7. Waste-to-Energy Conversion
Waste-to-energy (WtE) is a method of generating electricity by burning or processing waste materials. This approach not only provides an alternative energy source but also helps reduce the volume of waste sent to landfills.
Types of Waste-to-Energy Technologies
- Incineration: The most common method of WtE, where waste is burned at high temperatures to generate steam, which powers turbines to generate electricity.
- Gasification: A process where waste materials are heated in a low-oxygen environment to produce syngas (a mixture of carbon monoxide and hydrogen), which can then be burned to generate electricity.
- Anaerobic Digestion: Organic waste is broken down by bacteria in the absence of oxygen, producing biogas that can be used to generate electricity.
Environmental Considerations
WtE technologies help reduce landfill waste and generate electricity, but they can also produce harmful emissions, particularly from incineration. However, modern WtE plants are equipped with advanced filtration systems to minimize pollutants.
Conclusion
The future of electricity generation is evolving rapidly, with numerous innovative and unique methods being developed to meet the world’s growing energy needs. From piezoelectric materials and tidal energy to algae biofuel and waste-to-energy conversion, these technologies offer new and exciting possibilities for creating cleaner, more sustainable energy sources. While each method has its challenges, ongoing research and technological advancements are helping to make these unconventional approaches more viable and scalable, potentially transforming the global energy landscape in the years to come.
10 Ways to Generate Electricity
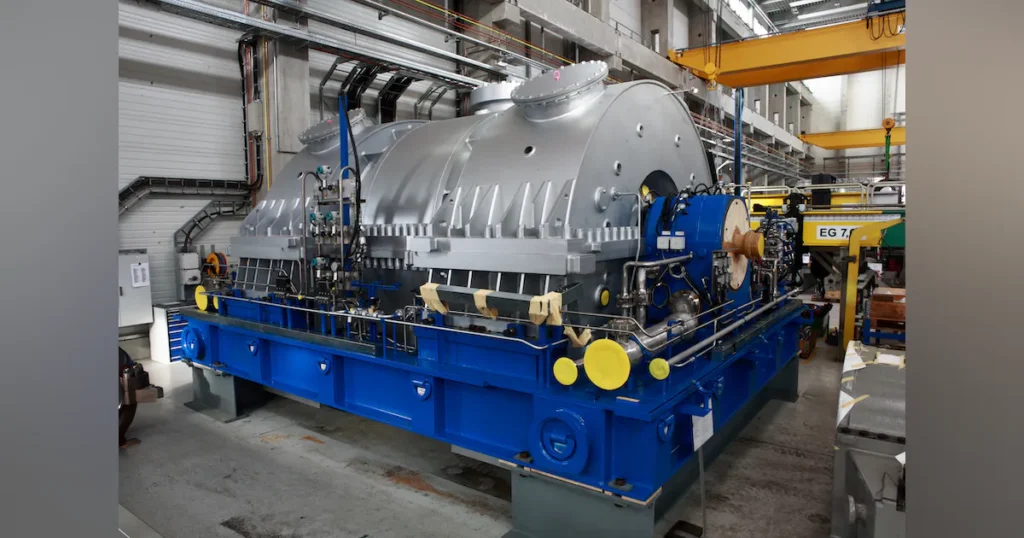
Electricity is essential for modern life, powering homes, industries, transportation, and communications systems. As energy demand rises, so does the need for a diverse range of electricity generation methods. Traditionally, electricity has been generated through the burning of fossil fuels, nuclear power, or large hydroelectric dams. However, technological advancements and environmental concerns have driven the development of various other methods to produce electricity. Some are conventional but evolving, while others are more innovative and represent the future of sustainable energy.
This section outlines 10 diverse methods of generating electricity, covering both well-established techniques and emerging technologies, with a focus on efficiency, sustainability, and environmental impact.
1. Solar Power
Solar power is one of the most popular and widely used renewable energy sources. It harnesses the energy of the sun to generate electricity through photovoltaic (PV) cells or concentrated solar power (CSP) systems.
Photovoltaic Cells (PV)
Photovoltaic cells, often seen in solar panels, directly convert sunlight into electricity. When sunlight strikes the cell, it causes electrons in the semiconductor material (usually silicon) to move, creating an electric current. Solar PV technology is scalable and can be used in small rooftop installations as well as large solar farms.
Concentrated Solar Power (CSP)
CSP systems use mirrors or lenses to concentrate sunlight onto a small area, where it heats a fluid, often molten salt. This fluid is used to produce steam, which drives a turbine connected to a generator. CSP is often used in large-scale solar power plants.
Advantages and Challenges
Solar power is a clean, renewable source of electricity with no direct emissions. However, solar power generation is intermittent, depending on weather conditions and daylight hours. Battery storage solutions or hybrid systems with other energy sources are often necessary to ensure a consistent supply of electricity.
2. Wind Power
Wind power is another rapidly growing source of renewable energy, with wind turbines generating electricity from the kinetic energy of the wind.
How Wind Power Works
Wind turbines have large blades that rotate when the wind blows. The blades are connected to a rotor, which spins a generator to produce electricity. Wind power can be harnessed on land (onshore wind farms) or at sea (offshore wind farms). Offshore wind farms tend to be more efficient due to stronger, more consistent winds over oceans.
Benefits and Limitations
Wind power is a clean and renewable source of energy, producing no emissions during operation. It is relatively cost-effective once turbines are installed. However, wind power is also intermittent and dependent on local weather conditions. Turbine installations can have aesthetic and noise concerns, and offshore wind farms face higher maintenance costs due to harsh marine environments.
3. Hydroelectric Power
Hydroelectric power, one of the oldest and most established forms of renewable energy, generates electricity by harnessing the potential energy of flowing or falling water.
Types of Hydroelectric Power
- Conventional Dams: Water stored in a reservoir behind a dam is released through turbines, which generate electricity. The height and volume of the water determine the energy potential.
- Run-of-the-River Systems: These systems divert part of a river’s flow through a turbine without creating large reservoirs. While they produce less energy than large dams, they have a smaller environmental footprint.
- Pumped-Storage Hydroelectricity: Water is pumped to a higher elevation during periods of low electricity demand and released to generate power during peak demand times, acting as a form of energy storage.
Advantages and Environmental Impacts
Hydroelectric power is reliable and can generate large amounts of electricity with relatively low operational costs. However, large dams can disrupt ecosystems, displace communities, and alter water flows. Run-of-the-river systems and small-scale hydro projects are considered more environmentally friendly alternatives.
4. Biomass Energy
Biomass energy is generated by burning organic materials such as wood, agricultural residues, or dedicated energy crops. It can also be produced from biogas through the anaerobic digestion of organic matter.
How Biomass Power Works
Biomass can be burned directly to generate heat, which produces steam to drive turbines and generate electricity. Alternatively, biomass can be converted into biogas or liquid biofuels, which can be used in conventional power plants or engines.
Sustainability and Challenges
Biomass energy is considered renewable as long as the organic material is sustainably sourced. However, the burning of biomass does release carbon dioxide (CO2), though at lower levels than fossil fuels. The carbon released is theoretically offset by the CO2 absorbed during the growth of the biomass. Ensuring sustainable sourcing and managing emissions are key challenges in biomass energy generation.
5. Geothermal Energy
Geothermal energy taps into the heat stored within the Earth’s crust to generate electricity. It is a reliable and consistent source of power, as the Earth’s heat is available 24/7, unlike solar or wind power.
How Geothermal Power Plants Work
In geothermal power plants, wells are drilled deep into the Earth to access hot water or steam reservoirs. The heat from these reservoirs is used to produce steam, which drives turbines connected to electricity generators. There are three main types of geothermal plants: dry steam, flash steam, and binary cycle, each designed to utilize different temperature ranges of geothermal reservoirs.
Advantages and Limitations
Geothermal energy is a clean and sustainable power source with minimal emissions. However, it is geographically limited to areas with significant geothermal activity, such as near tectonic plate boundaries. High upfront costs for exploration and drilling also present challenges to widespread adoption.
6. Tidal Energy
Tidal energy harnesses the movement of ocean tides to generate electricity. As the tides rise and fall, their kinetic energy can be captured using different technologies.
Types of Tidal Power Systems
- Tidal Barrages: Dams are built across tidal estuaries. As water flows in and out with the tide, it is released through turbines to generate electricity.
- Tidal Stream Generators: These systems function similarly to underwater wind turbines, capturing the energy from moving water.
- Dynamic Tidal Power: A newer concept that involves constructing long, dam-like structures perpendicular to the coast to capture the energy of tidal currents.
Benefits and Drawbacks
Tidal energy is highly predictable and reliable, making it a consistent source of renewable energy. However, tidal power plants are expensive to build, and their environmental impact on marine ecosystems must be carefully managed.
7. Nuclear Power
Nuclear power generates electricity by using nuclear reactions to produce heat, which then creates steam to drive turbines connected to generators.
How Nuclear Power Works
In nuclear reactors, atoms of uranium or other fissile materials are split in a controlled chain reaction. The heat released from this process is used to generate steam, which powers turbines that generate electricity.
Pros and Cons
Nuclear power produces large amounts of electricity with no greenhouse gas emissions during operation. It is a reliable, base-load energy source that can operate continuously for extended periods. However, concerns about nuclear waste, high construction costs, and the potential for catastrophic accidents (such as Chernobyl or Fukushima) remain significant challenges.
8. Natural Gas Combined Cycle
Natural gas is one of the most widely used fossil fuels for electricity generation. Combined cycle power plants enhance the efficiency of natural gas by using two stages of energy conversion.
How Combined Cycle Power Plants Work
In a combined cycle plant, natural gas is burned to power a gas turbine. The waste heat from the gas turbine is then used to produce steam, which drives a steam turbine, generating additional electricity. This two-step process makes combined cycle plants more efficient than traditional single-cycle gas plants.
Environmental Considerations
Natural gas emits significantly less CO2 than coal or oil when burned, making it a cleaner option among fossil fuels. However, it is still a non-renewable resource and contributes to greenhouse gas emissions.
9. Waste-to-Energy
Waste-to-energy (WtE) is the process of generating electricity by burning or processing waste materials. This method reduces the volume of waste going to landfills while also providing an energy source.
How WtE Works
In incineration-based WtE plants, waste is burned to produce heat, which generates steam to drive electricity-producing turbines. Other methods include anaerobic digestion, which breaks down organic waste to produce biogas that can be burned for power.
Advantages and Environmental Impact
Waste-to-energy reduces landfill waste and provides a supplementary source of electricity. However, WtE plants must be carefully managed to reduce emissions, as burning waste can release pollutants such as dioxins and particulate matter.
10. Hydrogen Fuel Cells
Hydrogen fuel cells generate electricity by combining hydrogen and oxygen in an electrochemical reaction that produces electricity, water, and heat.
How Fuel Cells Work
In a hydrogen fuel cell, hydrogen molecules are split into protons and electrons. The electrons travel through an external circuit, creating electricity, while the protons move through an electrolyte to combine with oxygen, forming water.
Applications and Future Potential
Hydrogen fuel cells are used in various applications, including electric vehicles, backup power systems, and portable electronics. The only byproduct is water, making fuel cells a clean energy source. However, the production of hydrogen is currently energy-intensive and often reliant on fossil fuels, though research into green hydrogen (produced via renewable energy) holds promise for the future.
Conclusion
The generation of electricity is becoming increasingly diverse, with both traditional and innovative methods contributing to the global energy mix. Each method outlined here has its own strengths and challenges, but together they reflect the transition toward more sustainable, efficient, and environmentally friendly electricity generation. As technology continues to advance, the role of renewable and innovative energy sources will only grow, ensuring a more resilient and cleaner energy future for the world.
Nuclear Steam Converter

The concept of a nuclear steam converter is central to the operation of nuclear power plants, where nuclear reactions generate immense heat that is ultimately converted into electricity. The term “nuclear steam converter” typically refers to the process by which heat from a nuclear reactor is used to create steam, which in turn drives turbines connected to electricity generators. This complex system represents the heart of nuclear power generation, efficiently transforming the energy released from nuclear fission into usable electrical power.
Nuclear energy is one of the most powerful and efficient means of generating electricity, offering the advantage of high energy density with minimal greenhouse gas emissions. However, the technology is also surrounded by challenges, including the need for safe handling of radioactive materials and management of nuclear waste. In this section, we will delve deeply into the workings of nuclear steam converters, the processes involved, and their critical role in modern nuclear power plants.
1. The Role of the Nuclear Steam Converter
The primary role of a nuclear steam converter in a power plant is to efficiently convert the heat produced by nuclear fission into steam, which drives turbines to generate electricity. The process is analogous to that of conventional thermal power plants that burn fossil fuels to create steam, but with a nuclear reactor as the heat source instead of coal, natural gas, or oil.
Nuclear power plants use different types of reactors, but they all follow a similar basic process involving a nuclear steam converter. The reactor generates heat from nuclear fission reactions, typically using uranium or plutonium as fuel. This heat is then transferred to a fluid, usually water, which is heated to create steam. The steam is directed towards turbines, which spin generators to produce electricity.
2. How Nuclear Fission Generates Heat
At the core of a nuclear power plant is the nuclear reactor, where fission—the splitting of atomic nuclei—takes place. The most commonly used fuel in nuclear reactors is uranium-235 (U-235). When a neutron strikes the nucleus of a U-235 atom, it causes the nucleus to split into smaller atoms, releasing a significant amount of energy in the form of heat. This process also releases more neutrons, which can then trigger further fission reactions in a self-sustaining chain reaction.
Control of the Fission Process
The nuclear fission process needs to be carefully controlled to avoid a runaway reaction, which could lead to excessive heat generation or even a meltdown. Control rods made of materials that absorb neutrons, such as cadmium, boron, or hafnium, are inserted into the reactor core to regulate the rate of fission. By adjusting the position of these control rods, operators can control the rate of the nuclear reaction and the amount of heat produced.
3. The Heat Transfer Mechanism
In a nuclear power plant, the heat generated by fission must be efficiently transferred from the reactor core to the steam generator. This is done using a coolant, which is typically either water or a gas such as carbon dioxide or helium. In most nuclear power plants, water is used as both the coolant and the working fluid in the steam generation process.
Types of Reactors and Coolants
- Pressurized Water Reactors (PWRs): In a PWR, the water used as a coolant is kept under high pressure, preventing it from boiling even at high temperatures. The hot, pressurized water is circulated through a heat exchanger, where it transfers its heat to a secondary loop of water, which then boils to create steam.
- Boiling Water Reactors (BWRs): In a BWR, the water is allowed to boil inside the reactor vessel itself. The steam generated in the reactor is sent directly to the turbine to produce electricity, eliminating the need for a secondary loop.
Both PWRs and BWRs are widely used in nuclear power plants around the world, with PWRs being the most common.
4. Steam Generation and Turbine Operation
Once the heat from the nuclear reactor has been transferred to water, it turns into steam. This high-pressure steam is then channeled to turbines, which are connected to generators. As the steam passes over the blades of the turbine, it causes the turbine to spin. The rotational energy of the turbine is converted into electrical energy by the generator, which operates on the principle of electromagnetic induction.
Efficiency of Steam Conversion
The efficiency of converting nuclear heat into electricity depends largely on how well the steam turbines can harness the energy from the steam. Nuclear plants typically operate with a thermal efficiency of around 33%, meaning that about one-third of the heat generated by fission is converted into electricity. The remainder of the heat is dissipated through cooling systems, which is why nuclear power plants are often located near large bodies of water to facilitate cooling.
5. Condensation and Heat Exchangers
After the steam has passed through the turbines, it must be condensed back into water to be reused in the cycle. This is accomplished in the condenser, a large heat exchanger that transfers the remaining heat in the steam to a cooling fluid, typically water from a nearby river, lake, or ocean.
Cooling Systems
There are two primary types of cooling systems used in nuclear power plants:
- Once-Through Cooling: In this system, water is drawn from a natural source, passed through the condenser, and then returned to the source at a higher temperature. While effective, once-through cooling can have environmental impacts, such as thermal pollution, which can harm aquatic life.
- Closed-Loop Cooling: In a closed-loop system, water is circulated within the plant, and the excess heat is dissipated via cooling towers or other heat exchange mechanisms. Closed-loop systems reduce environmental impacts but require more infrastructure and energy to operate.
6. The Importance of Safety Systems
Safety is a paramount concern in nuclear power plants, particularly in the nuclear steam conversion process. Several layers of safety systems are in place to prevent overheating and to ensure that radioactive materials are contained.
Primary Containment Systems
The reactor core is housed within a thick containment structure, designed to prevent the release of radioactive materials in the event of an accident. In PWRs, the primary coolant loop is also contained within a steel pressure vessel to withstand high temperatures and pressures.
Emergency Cooling Systems
In case of a reactor malfunction or loss of coolant, emergency cooling systems are activated to prevent the reactor core from overheating. These systems can inject water directly into the reactor core or circulate coolant to dissipate excess heat.
7. Environmental Impact and Waste Management
Nuclear power plants, despite their clean energy production, do generate radioactive waste, which needs to be carefully managed. The waste produced from the fission process includes spent nuclear fuel, which remains radioactive for thousands of years and must be stored safely.
Short-Term and Long-Term Waste Storage
Spent fuel is initially stored in large water-filled pools, which provide shielding and cooling for the highly radioactive material. After a period of years, the spent fuel can be transferred to dry cask storage, where it is stored in sealed containers made of steel and concrete.
Nuclear Waste Disposal Challenges
One of the most significant challenges facing the nuclear industry is the long-term disposal of high-level radioactive waste. Geological repositories, where waste can be stored deep underground in stable rock formations, are being considered as the best option for permanent disposal. However, political and technical challenges have delayed the development of such facilities.
8. Future Developments in Nuclear Steam Conversion
As the world looks to reduce its reliance on fossil fuels and combat climate change, nuclear energy is expected to play an increasingly important role in the energy mix. Several advanced nuclear technologies are under development, aimed at improving the efficiency and safety of nuclear power plants.
Small Modular Reactors (SMRs)
SMRs are a new class of nuclear reactors that are smaller, more efficient, and safer than traditional reactors. They are designed to be built in factories and transported to the site, reducing construction times and costs. SMRs also have advanced safety features, such as passive cooling systems, which can operate without human intervention or external power.
Generation IV Reactors
Generation IV reactors represent the next leap in nuclear technology, promising even greater efficiency, safety, and sustainability. These reactors will use advanced coolants, such as molten salt or liquid metal, and can operate at higher temperatures, allowing for more efficient steam conversion and greater electricity output. They are also designed to minimize nuclear waste and enhance fuel efficiency, with some designs capable of using spent nuclear fuel from traditional reactors.
Conclusion
The nuclear steam converter is a critical component of nuclear power plants, enabling the conversion of nuclear heat into electricity through the generation of steam. While the basic principles of nuclear steam conversion have remained consistent since the early days of nuclear energy, advancements in reactor design and safety systems continue to push the boundaries of what is possible. With ongoing research and development, the efficiency and sustainability of nuclear power are set to improve, making it an even more viable option in the global transition toward clean energy.
Steam Condenser Heat Exchanger

In thermal power plants, whether they run on coal, natural gas, nuclear energy, or biomass, one critical component that ensures efficient operation is the steam condenser heat exchanger. This device is responsible for condensing steam back into water after it has passed through the turbines, thus completing the cycle of converting heat into electricity. It plays a vital role in improving efficiency, conserving water, and ensuring the system can operate continuously without excessive loss of resources.
A steam condenser heat exchanger is essential not only for its role in maintaining system efficiency but also for its environmental impact. By condensing steam and allowing it to be reused, condensers help reduce the amount of water needed for cooling, which can lessen the environmental footprint of power plants.
This section explores how steam condensers work, their designs, the role they play in power generation, and the technological advancements that are improving their efficiency and sustainability.
1. The Purpose of a Steam Condenser Heat Exchanger
The primary function of a steam condenser heat exchanger in a power plant is to convert the steam that exits the turbines back into water. This water, now at a much lower temperature, is reused in the boiler or reactor for the next cycle of steam generation. The conversion from steam to liquid is achieved by removing the latent heat from the steam, which is transferred to a cooling medium, usually water from a river, lake, or cooling tower system.
The condenser lowers the pressure at the exhaust side of the turbine, which helps increase the efficiency of the turbine by maximizing the pressure difference between the high-pressure inlet steam and the low-pressure outlet steam. This pressure difference drives the turbines more effectively, generating more electricity.
2. The Condensation Process
To understand how a steam condenser works, it’s essential to grasp the basic principles of condensation. When steam is cooled below its saturation temperature, it releases its latent heat and transforms into a liquid state—water. The condenser captures this heat through heat exchanger tubes, and the resulting water, known as condensate, is collected and returned to the boiler or steam generator for reuse.
The Latent Heat of Vaporization
Latent heat is the heat required to convert a substance from one phase to another without changing its temperature. In the case of steam, the heat energy used to convert water into steam is stored as latent heat. When this heat is removed, steam reverts to water. The condenser’s job is to efficiently extract this latent heat and transfer it to the cooling medium, which carries it away, often to a large body of water or a cooling tower.
Thermodynamic Efficiency
One of the most important roles of the condenser is improving the thermodynamic efficiency of the power plant. By creating a vacuum at the turbine exhaust, the steam is drawn out more quickly, allowing for more energy to be extracted from the steam before it condenses. This process increases the work output of the turbine and, consequently, the overall efficiency of electricity generation.
3. Types of Steam Condenser Heat Exchangers
There are several types of condensers used in power plants, each designed for different applications and operational needs. The most common types are the surface condenser and the jet condenser.
Surface Condenser
The surface condenser is the most commonly used condenser in large power plants. It operates on the principle of indirect heat exchange, meaning the steam does not mix with the cooling water. Instead, steam passes over a series of tubes filled with cold water, and as it loses heat, it condenses into water.
- Design: Surface condensers consist of a large cylindrical shell filled with horizontal tubes. Cooling water flows through the tubes, while steam enters the shell and condenses on the outside of the tubes. The condensed water, or condensate, collects at the bottom of the condenser, from where it is pumped back to the boiler.
- Advantages: Surface condensers are highly efficient and prevent contamination of the condensate with the cooling water, which is crucial in systems that rely on high-purity water, such as nuclear power plants.
- Disadvantages: Surface condensers are large and require significant amounts of cooling water, which is why they are often located near bodies of water or use cooling towers.
Jet Condenser
Jet condensers, also known as contact condensers, operate on the principle of direct contact between steam and cooling water. In this design, steam is directly mixed with cooling water, condensing it immediately.
- Design: In a jet condenser, steam enters a chamber where it comes into contact with a spray or jet of cold water. The water absorbs the latent heat, causing the steam to condense. The resulting mixture of water and condensate is then pumped out.
- Advantages: Jet condensers are simpler and more compact than surface condensers. They are also less expensive to install and maintain.
- Disadvantages: Because steam and water mix directly, the cooling water must be clean and free of contaminants. Additionally, jet condensers cannot maintain as high a vacuum as surface condensers, leading to lower overall efficiency.
4. Cooling Mediums and Heat Transfer
In a steam condenser heat exchanger, the efficiency of heat removal is dependent on the cooling medium. Most power plants use water as the cooling medium, as it has excellent heat transfer properties and is readily available. However, the choice of cooling medium and heat transfer design can have significant implications for the environment and plant operation.
Water Cooling
Water is the most common cooling medium used in steam condensers, as it has a high heat capacity and is typically available in large quantities near power plants. There are two primary methods of water cooling:
- Once-Through Cooling: In this system, water is drawn from a natural source (such as a river or ocean), passed through the condenser to absorb heat, and then discharged back into the environment. While simple and efficient, once-through cooling can cause thermal pollution, as the warmer water is returned to the natural source, which can disrupt local ecosystems.
- Closed-Loop Cooling: In a closed-loop system, cooling water circulates within the plant and is reused. The heated water is cooled in a cooling tower or heat exchanger before being sent back to the condenser. This method minimizes water usage and environmental impact but requires more infrastructure and energy for cooling.
Air Cooling
In areas where water is scarce, air cooling can be used as an alternative. In air-cooled condensers, fans are used to blow air over the condenser tubes, transferring heat from the steam to the air. While air-cooled systems use less water, they are generally less efficient than water-cooled systems due to air’s lower heat capacity.
5. Environmental Considerations
Steam condensers, particularly those using water as a cooling medium, can have a significant environmental impact if not properly managed. The primary concerns include thermal pollution and water consumption.
Thermal Pollution
Thermal pollution occurs when heated water is discharged into natural bodies of water, raising the temperature of the ecosystem. This can lead to harmful effects on aquatic life, such as reduced oxygen levels and disruption of breeding cycles. Many modern power plants use cooling towers to dissipate excess heat into the atmosphere instead of releasing it into water sources, helping to mitigate these impacts.
Water Usage
Power plants with steam condensers require vast amounts of water to cool the steam efficiently. In areas where water is scarce, this can put pressure on local water supplies, leading to conflicts over water use. Closed-loop cooling systems, which recycle water, help reduce the overall demand for freshwater but still require some water to make up for losses due to evaporation.
6. Maintenance and Efficiency of Steam Condenser Heat Exchangers
Proper maintenance of steam condenser heat exchangers is critical for ensuring efficient operation and extending the lifespan of the equipment. Poorly maintained condensers can suffer from reduced heat transfer efficiency, leading to lower overall power plant efficiency and increased operational costs.
Fouling and Scaling
Over time, condensers can suffer from fouling, where impurities in the cooling water (such as algae, silt, or minerals) accumulate on the surface of the heat exchanger tubes. This buildup acts as an insulating layer, reducing the rate of heat transfer and decreasing condenser efficiency. Regular cleaning of the condenser tubes is essential to prevent fouling and maintain optimal performance.
Corrosion
Corrosion is another common issue in steam condensers, particularly in systems that use saltwater for cooling. Saltwater is highly corrosive and can damage the condenser tubes over time, leading to leaks and reduced efficiency. Corrosion-resistant materials, such as stainless steel or titanium, are often used in condenser construction to combat this issue.
7. Technological Advances in Steam Condensers
Advancements in technology are continually improving the design and operation of steam condensers. Innovations include new materials for heat exchanger tubes that resist fouling and corrosion, improved cooling tower designs, and more efficient air-cooling systems.
High-Performance Materials
Modern condensers increasingly use advanced materials such as titanium or polymer coatings to reduce fouling and corrosion. These materials are not only more durable but also improve heat transfer efficiency, allowing for smaller, more compact condenser designs.
Hybrid Cooling Systems
In areas with limited water resources, hybrid cooling systems are being developed that combine water and air cooling. These systems use water during periods of peak demand, when maximum efficiency is required, and switch to air cooling during off-peak times to conserve water.
Conclusion
The steam condenser heat exchanger is a crucial component of thermal power plants, ensuring that the steam cycle operates efficiently and that water resources are conserved. By removing latent heat from steam and condensing it back into water, condensers enable the continuous operation of power plants, while also improving overall efficiency and reducing environmental impact. With ongoing technological advancements, the future of steam condenser design looks to improve efficiency, sustainability, and adaptability, making them an even more integral part of power generation systems.
Steam Turbine Control Valves

Steam turbines are integral components of power plants, where they convert thermal energy from steam into mechanical energy, which then drives electrical generators. Within this complex system, steam turbine control valves play a crucial role. They regulate the flow, pressure, and temperature of the steam entering the turbine, thus controlling the speed and power output of the turbine. These valves are vital for ensuring the safety, efficiency, and reliability of the power generation process.
Steam turbine control valves must withstand extreme conditions, such as high temperatures and pressures, and operate with precision to maintain turbine stability. The importance of these valves extends beyond simple regulation—they are essential for optimizing performance, preventing damage to the turbine, and ensuring smooth transitions during startup, load changes, and shutdowns.
This section explores the different types of steam turbine control valves, their functions, design considerations, and their role in enhancing efficiency and safety in power generation.
1. The Role of Steam Turbine Control Valves
Steam turbine control valves are responsible for regulating the flow of steam into the turbine. By controlling the amount of steam entering the turbine, these valves determine the amount of power generated. Efficient control of steam flow is critical to maximizing the efficiency of the turbine and the overall power plant, ensuring smooth operation and preventing damage to the turbine caused by excess pressure or flow.
The valves are also instrumental during startup and shutdown procedures, allowing operators to gradually increase or decrease steam flow to avoid sudden changes in pressure or temperature, which could damage the turbine.
2. Types of Steam Turbine Control Valves
Steam turbines typically use several types of control valves, each serving a specific function in managing steam flow and pressure. The primary types include governor valves, stop valves, reheat valves, and bypass valves.
Governor Valves
Governor valves are the primary control valves that regulate the amount of steam entering the high-pressure stage of the turbine. These valves control the turbine’s speed by adjusting the steam flow rate. They are often used in tandem with other control systems to ensure precise turbine operation under varying loads.
- Function: Governor valves are designed to modulate the steam flow during normal turbine operation, adjusting the load and speed of the turbine to meet power generation demands.
- Operation: These valves operate continuously, responding to signals from the turbine’s governor system, which monitors the turbine’s speed and adjusts the valve position accordingly. By modulating the steam flow, the governor system ensures that the turbine operates at the desired speed and power output.
Stop Valves
Stop valves, also known as emergency stop valves or main steam stop valves, are safety devices that shut off the steam supply to the turbine in the event of an emergency. They act as a protective mechanism, preventing damage to the turbine by cutting off steam flow if a dangerous condition is detected, such as overspeed or overpressure.
- Function: The primary role of stop valves is to rapidly isolate the turbine from the steam supply in emergency situations, such as a turbine trip, to protect the equipment and ensure safety.
- Operation: These valves remain fully open during normal operation and close only when an emergency signal is received from the turbine’s control system. Stop valves are designed to close quickly and reliably to prevent steam from continuing to flow to the turbine after a trip event.
Reheat Valves
In steam turbines with reheat cycles, reheat stop valves and reheat intercept valves are used to control the flow of steam between the high-pressure and low-pressure stages of the turbine. After passing through the high-pressure turbine, steam is sent back to the boiler to be reheated before it is introduced into the low-pressure turbine.
- Function: Reheat valves control the steam entering the reheater section, ensuring that steam temperature and pressure are optimized for the low-pressure turbine stage.
- Operation: These valves modulate the reheated steam flow and help to maintain the desired turbine load during reheating cycles. Reheat intercept valves also provide a quick shutdown capability for the reheated steam in emergency situations.
Bypass Valves
Bypass valves are used to divert steam around the turbine when the turbine is not ready to accept steam, such as during startup or shutdown. They allow the boiler to continue operating while the turbine is being brought up to operating speed or while it is cooling down.
- Function: Bypass valves direct steam away from the turbine and into the condenser or other heat exchangers to maintain pressure balance within the system during transient conditions.
- Operation: These valves are critical during the startup phase when the turbine is warming up and cannot yet accept high-pressure steam. Bypass valves ensure that steam pressure remains controlled and that the system operates smoothly without causing damage to the turbine or boiler.
3. Design Considerations for Steam Turbine Control Valves
Designing steam turbine control valves requires consideration of multiple factors, including the high temperatures and pressures they will encounter, the need for precise control, and the potential for wear and tear over time. Valve designers must take into account the specific requirements of the power plant, the type of turbine, and the operational conditions under which the valves will operate.
High-Pressure and High-Temperature Operation
Steam turbines operate under extreme conditions, with steam temperatures often exceeding 500°C (932°F) and pressures reaching over 3,500 psi (pounds per square inch). Steam turbine control valves must be constructed from materials that can withstand these harsh conditions without deforming, corroding, or losing efficiency.
- Materials: The most commonly used materials for control valves are high-strength alloys such as stainless steel, Inconel, and titanium. These materials offer excellent resistance to heat and pressure while also being durable enough to withstand long-term use in power plants.
- Thermal Expansion: Valves must be designed to accommodate thermal expansion, as temperature changes can cause components to expand or contract, potentially affecting valve performance. Proper design and material selection are critical to ensuring that valves operate reliably even under fluctuating temperature conditions.
Flow Control Precision
Precision is essential in steam turbine control valves, especially for governor valves, which must adjust the steam flow rate accurately in response to changes in turbine speed or load. The valve design must allow for fine adjustments in the steam flow while minimizing pressure drops or turbulence that could affect turbine efficiency.
- Actuators and Control Systems: Modern control valves are equipped with advanced actuators and control systems that enable precise positioning of the valve in response to signals from the turbine control system. These actuators are typically pneumatic, hydraulic, or electric, each offering different advantages in terms of response time, accuracy, and reliability.
- Cavitation and Erosion: Steam control valves are susceptible to cavitation and erosion, particularly at high flow rates or when dealing with wet steam. Cavitation occurs when the pressure inside the valve drops below the vapor pressure of the steam, causing vapor bubbles to form and collapse, which can damage the valve surfaces. Erosion occurs when steam particles wear away the valve material over time. Valve design must minimize these effects through proper selection of materials and flow paths.
4. The Role of Steam Turbine Control Valves in Efficiency and Load Control
Steam turbine control valves are integral to improving the efficiency of power generation. They manage the flow of steam to ensure that the turbine operates at its most efficient point, depending on the load demand. By regulating steam flow, control valves help optimize fuel use, reduce waste, and improve overall plant efficiency.
Load Matching and Frequency Regulation
Steam turbines often operate in systems where electricity demand fluctuates throughout the day. Control valves allow operators to match the steam flow to the load demand, ensuring that the turbine produces just enough power to meet grid requirements without wasting fuel or causing excess wear on the equipment.
- Automatic Load Control: Modern turbines are equipped with automated control systems that continuously adjust the valve position based on real-time data from the grid and turbine sensors. This ensures that the turbine operates efficiently across a range of loads while maintaining grid frequency stability.
- Frequency Regulation: Control valves also play a critical role in maintaining the frequency of the electrical grid. Small adjustments to the steam flow can be made rapidly to correct any deviations from the target frequency, ensuring stable power delivery.
Startups and Shutdowns
During turbine startups and shutdowns, steam control valves help protect the turbine from thermal stresses and pressure fluctuations. Gradually opening or closing the valves allows steam to be introduced or removed from the turbine in a controlled manner, reducing the risk of damage to the turbine blades or other critical components.
- Slow Warming and Cooling: During startup, the turbine must be gradually brought up to operating temperature to avoid thermal shock, which could crack or warp the turbine components. Control valves modulate steam flow during this process, ensuring that the temperature increases at a controlled rate. Similarly, during shutdown, steam flow is gradually reduced to allow the turbine to cool down slowly.
5. Maintenance and Reliability of Steam Turbine Control Valves
Proper maintenance of steam turbine control valves is crucial for ensuring the long-term reliability and efficiency of the power plant. These valves experience significant wear due to the harsh conditions they operate under, and any failure can result in costly downtime or damage to the turbine.
Preventive Maintenance
Preventive maintenance programs are essential for keeping steam control valves in good working order. Regular inspections can identify signs of wear, such as erosion, corrosion, or leakage, allowing for repairs or replacements before a major failure occurs.
Actuator Maintenance: The actuators that control the valve movement also require regular maintenance to ensure they are responding accurately to control signals. Pneumatic and hydraulic
Valve Testing: Valves should be tested periodically to ensure they are functioning correctly. This includes testing the responsiveness of the actuators, checking for leaks, and verifying that the valves can fully open and close.
EMS Power Machines
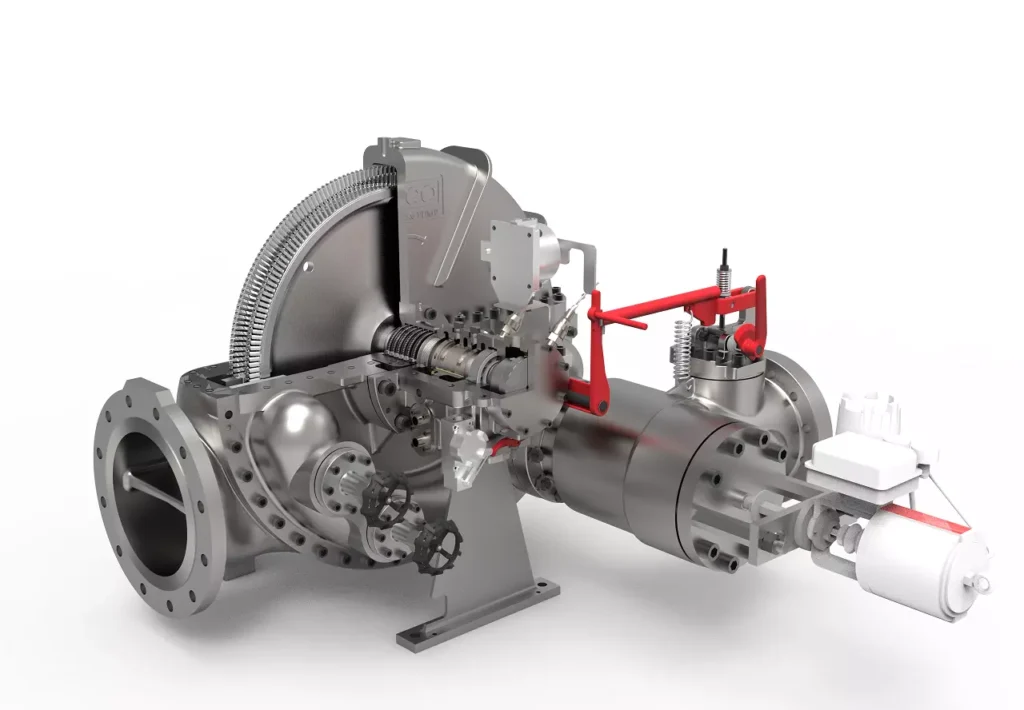
We design, manufacture and assembly Power Machines such as – diesel generators, electric motors, vibration motors, pumps, steam engines and steam turbines
EMS Power Machines is a global power engineering company, one of the five world leaders in the industry in terms of installed equipment. The companies included in the company have been operating in the energy market for more than 60 years.
EMS Power Machines manufactures steam turbines, gas turbines, hydroelectric turbines, generators, and other power equipment for thermal, nuclear, and hydroelectric power plants, as well as for various industries, transport, and marine energy.
EMS Power Machines is a major player in the global power industry, and its equipment is used in power plants all over the world. The company has a strong track record of innovation, and it is constantly developing new and improved technologies.
Here are some examples of Power Machines’ products and services:
- Steam turbines for thermal and nuclear power plants
- Gas turbines for combined cycle power plants and industrial applications
- Hydroelectric turbines for hydroelectric power plants
- Generators for all types of power plants
- Boilers for thermal power plants
- Condensers for thermal power plants
- Reheaters for thermal power plants
- Air preheaters for thermal power plants
- Feedwater pumps for thermal power plants
- Control systems for power plants
- Maintenance and repair services for power plants
EMS Power Machines is committed to providing its customers with high-quality products and services. The company has a strong reputation for reliability and innovation. Power Machines is a leading provider of power equipment and services, and it plays a vital role in the global power industry.
EMS Power Machines, which began in 1961 as a small factory of electric motors, has become a leading global supplier of electronic products for different segments. The search for excellence has resulted in the diversification of the business, adding to the electric motors products which provide from power generation to more efficient means of use.