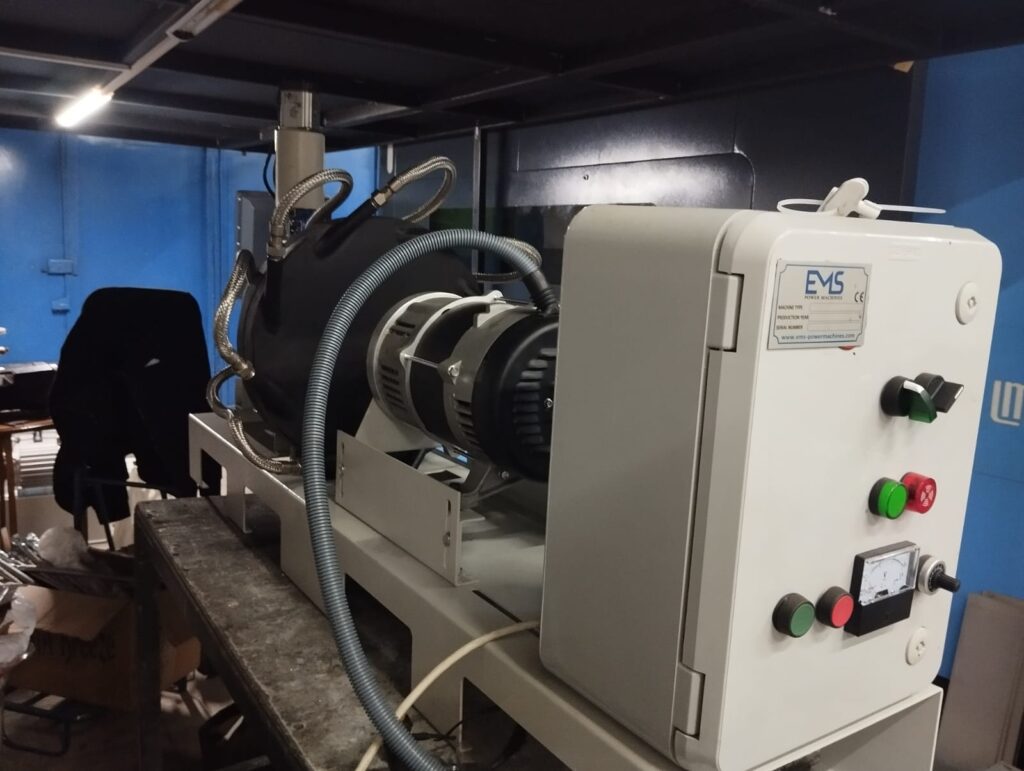
Steam Turbine: A steam turbine is a mechanical device that converts the thermal energy of steam into mechanical energy, typically rotational motion. It does this by directing high-pressure, high-temperature steam onto a series of blades or buckets mounted on a rotor. As the steam expands and loses pressure, it causes the rotor to spin, which can then be used to drive generators, pumps, compressors, or other machinery.
Key Points About Steam Turbines:
- Working Principle: Steam expands through turbine blades, transferring its energy to the rotor.
- Energy Conversion: Thermal energy (from steam) → Mechanical energy (rotational motion).
- Applications: Power generation in thermal power plants, propulsion in ships, mechanical drives in industries.
- Types: Impulse turbines, reaction turbines, and combinations of both.
In summary, steam turbines are essential components in many power generation and industrial processes, efficiently converting steam energy into useful mechanical work.
A steam turbine is a device that transforms the energy stored in high-pressure steam into mechanical energy by using a series of blades mounted on a rotating shaft. When steam is produced, typically in a boiler, it possesses a high temperature and pressure. This steam is directed onto the turbine blades, where its thermal energy is converted into kinetic energy. As the steam expands and flows over the blades, it imparts force to them, causing the rotor to turn. This rotational motion is then harnessed to perform useful work, such as driving an electrical generator to produce electricity or powering mechanical equipment in industrial settings.
The basic operation of a steam turbine relies on the principles of thermodynamics and fluid dynamics. Steam at high pressure and temperature enters the turbine, where it expands through nozzles or fixed blades, increasing its velocity while decreasing pressure. The high-velocity steam then strikes the moving blades attached to the rotor, transferring momentum and causing the rotor to rotate. Depending on the design, turbines can use either impulse action, reaction action, or a combination of both to extract energy from the steam. Impulse turbines convert the pressure energy of steam into velocity in nozzles before it hits the blades, while reaction turbines utilize both pressure drop and velocity change across the moving blades themselves.
Steam turbines are widely used in power generation due to their efficiency and ability to handle large volumes of steam at high temperatures. They form the heart of most thermal power plants, including coal, nuclear, and natural gas plants, where steam generated in boilers drives turbines connected to electric generators. Beyond electricity production, steam turbines also serve industrial processes, ship propulsion, and mechanical drives for compressors and pumps. Their design can vary significantly based on application, steam conditions, and required output power, but their fundamental role remains the conversion of steam’s thermal energy into mechanical rotation.
Overall, the steam turbine is a critical technology that underpins modern electricity generation and many industrial operations, offering a reliable and efficient way to convert heat energy into mechanical work.
Steam turbines operate under the fundamental laws of thermodynamics, specifically the Rankine cycle, which describes how water is converted to steam, expanded through the turbine to produce work, and then condensed back to water to repeat the cycle. The efficiency of a steam turbine depends largely on the pressure and temperature of the steam entering the turbine, as well as the quality of the steam—meaning how much moisture it contains. Dry steam with high pressure and temperature yields the best performance, while wet steam can cause erosion and damage to turbine blades, reducing efficiency and lifespan.
The design of a steam turbine includes several stages of blades, arranged in rows, each stage extracting a portion of the steam’s energy. This multi-stage design allows the turbine to efficiently extract energy over a range of steam pressures as the steam expands progressively through the turbine. The blades themselves are precisely engineered to optimize the conversion of steam energy into mechanical rotation, often shaped aerodynamically and manufactured from high-strength materials to withstand high temperatures, pressures, and mechanical stresses.
Steam turbines can be classified based on their operating pressure and their exhaust pressure. For example, condensing turbines exhaust steam at very low pressures into a condenser, maximizing energy extraction by using the pressure difference between the high-pressure steam inlet and the low-pressure exhaust. This type is common in electricity generation where maximizing efficiency is crucial. On the other hand, back-pressure turbines exhaust steam at higher pressures suitable for direct industrial use, such as heating or process steam, making them valuable in combined heat and power (CHP) systems.
Because steam turbines operate at very high rotational speeds, they are often coupled with reduction gears or designed to run generators at high speeds to generate electricity efficiently. Maintenance of steam turbines involves careful attention to blade condition, lubrication, and balancing to avoid vibrations and mechanical failures. Advances in metallurgy, blade design, and steam path sealing have continually improved turbine efficiency and reliability over the decades.
In summary, steam turbines are a cornerstone of modern power generation and industrial energy conversion, leveraging high-pressure steam to produce reliable, large-scale mechanical power. Their ability to efficiently convert thermal energy into rotational energy with minimal moving parts makes them durable, cost-effective, and essential for meeting global energy demands.
The Science of Steam: Understanding Turbines
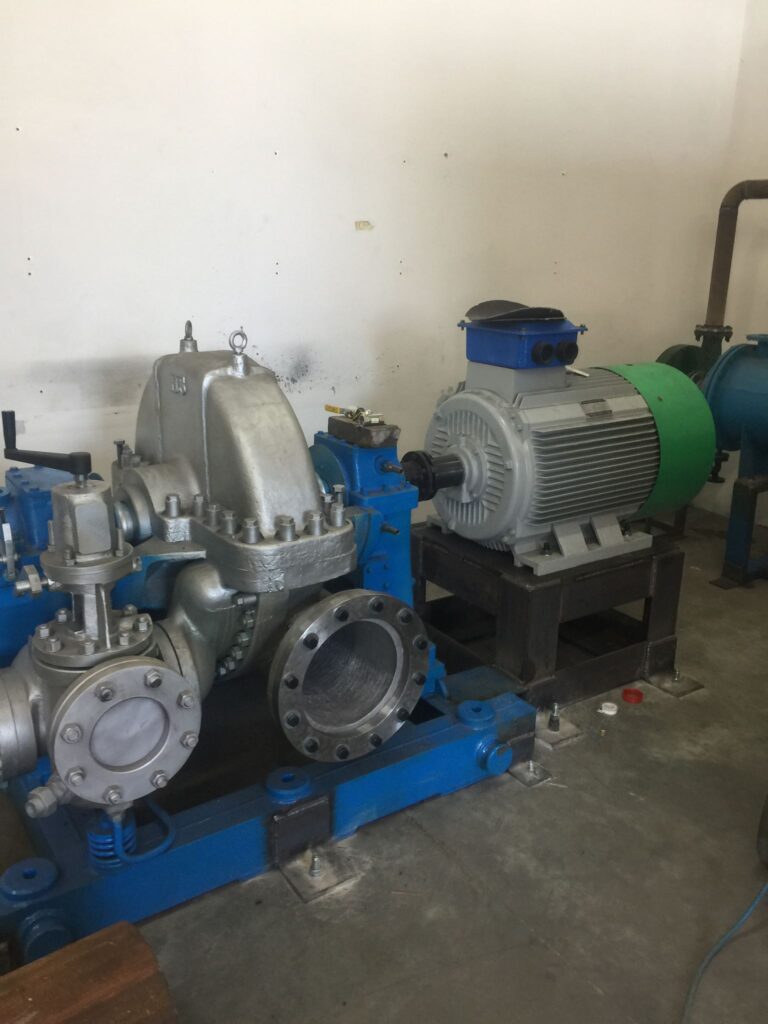
The science behind steam turbines lies at the intersection of thermodynamics, fluid mechanics, and mechanical engineering, where the goal is to efficiently convert the thermal energy of steam into useful mechanical work. At its core, a steam turbine uses the energy contained in steam—created by heating water under pressure—to generate rotational motion, which can then drive electrical generators or other machinery.
Steam itself is water in a gaseous state, produced by boiling water at high temperatures and pressures. This steam carries significant internal energy, stored as both heat and pressure. When this steam expands through the turbine, it undergoes a drop in pressure and temperature, converting its internal energy into kinetic energy—the energy of motion. The science behind this energy conversion process is governed primarily by the principles of the Rankine cycle, which is the thermodynamic cycle describing how heat energy is added and extracted in steam power plants.
Within the turbine, steam flows over rows of precisely engineered blades attached to a rotating shaft. The blades are designed to capture the kinetic energy of the steam as it moves at high speed. There are two main scientific principles at play here: impulse and reaction. In an impulse turbine, steam is accelerated through nozzles to produce a high-velocity jet that strikes the turbine blades, transferring energy through impact forces. In a reaction turbine, the steam pressure drops continuously over the moving blades themselves, generating a reactive force that turns the blades. Many modern turbines combine these two effects to maximize efficiency.
From a mechanical perspective, the materials and design of turbine components must withstand extreme conditions. Turbine blades operate in environments of very high temperature and pressure, often exceeding 500 degrees Celsius and hundreds of atmospheres of pressure. Materials science plays a crucial role in developing alloys and coatings that resist corrosion, thermal fatigue, and mechanical wear, ensuring long turbine life and safe operation.
Fluid mechanics explains how steam flows through the turbine, including how its velocity, pressure, and direction change as it moves through nozzles and blades. The blades’ shape and angle are designed to optimize the transfer of momentum from the moving steam to the rotor, minimizing energy losses due to turbulence or friction. The science of steam turbines also includes thermodynamic efficiency, which is a measure of how much of the steam’s energy is converted into mechanical energy versus how much is lost as heat or friction.
In essence, understanding steam turbines scientifically involves grasping how heat energy in steam is converted step-by-step into mechanical energy through carefully controlled fluid flow and mechanical design. This knowledge drives improvements in turbine efficiency, durability, and performance, enabling modern power plants to produce vast amounts of electricity reliably and sustainably.
The continuous improvement of steam turbine technology is deeply rooted in advancements in scientific understanding and engineering innovation. As power demands grow and environmental concerns increase, engineers and scientists strive to push the limits of turbine efficiency and durability. One key area of focus is increasing the steam temperature and pressure entering the turbine, as higher thermal energy directly translates to more mechanical power output. However, this also presents material challenges, requiring the development of superalloys and advanced cooling techniques to prevent blade deformation and failure under extreme conditions.
Another scientific challenge lies in minimizing energy losses within the turbine. These losses can occur due to friction between the steam and turbine components, aerodynamic drag on the blades, and steam leakage through gaps in the turbine casing. Engineers employ precise manufacturing methods and aerodynamic designs, such as curved and twisted blades, to optimize steam flow and reduce turbulence. Computational fluid dynamics (CFD) simulations are now extensively used to model and refine steam pathways, allowing designers to predict performance and identify inefficiencies before physical prototyping.
The science of steam turbines also extends to their integration into complex power plant systems. The Rankine cycle itself involves stages beyond the turbine, such as boilers, condensers, and feedwater pumps, all designed to maximize overall thermal efficiency. Innovations like reheating, where steam is expanded partially, reheated, and then expanded again in a second turbine, help extract more energy while controlling blade temperature. Regenerative feedwater heating, where steam is used to preheat the boiler’s feedwater, reduces fuel consumption and emissions, illustrating how thermodynamic science informs practical design choices.
From a broader perspective, steam turbines play a vital role in the transition to sustainable energy systems. While traditionally powered by fossil fuels, steam turbines are also integral to nuclear power plants and can be adapted to use steam generated by renewable biomass or concentrated solar power systems. This versatility underscores the importance of a deep scientific understanding, as adapting turbines to new steam sources involves tackling unique challenges in steam chemistry, corrosion, and temperature control.
In sum, the science behind steam turbines is a dynamic field that blends thermodynamics, fluid mechanics, materials science, and mechanical engineering. This blend drives ongoing improvements in efficiency, reliability, and environmental performance. Through scientific insight, steam turbines continue to be one of the most effective and widely used technologies for converting thermal energy into mechanical and electrical power, shaping the way the world generates and uses energy.
As steam turbine technology evolves, research continues to focus on pushing efficiency closer to the theoretical limits dictated by thermodynamics, while also addressing real-world constraints like material strength, maintenance costs, and environmental impact. One significant area of development is in combined cycle power plants, where steam turbines are paired with gas turbines. In these systems, the waste heat from a gas turbine is used to produce steam that then drives a steam turbine, effectively capturing more energy from the fuel and boosting overall plant efficiency beyond what either turbine could achieve alone. This synergy between different turbine technologies exemplifies how scientific principles and engineering creativity merge to optimize energy use.
The interaction between steam conditions and turbine components also influences operational strategies. For example, part-load operation or frequent cycling—turning the turbine on and off—can introduce thermal stresses and vibrations that shorten component life. Modern control systems use advanced sensors and algorithms to monitor steam quality, temperature, pressure, and vibration in real-time, allowing operators to adjust conditions dynamically and prevent damage. This integration of digital technology with traditional steam turbine science highlights the evolving nature of the field, combining classical physics with modern data analytics for safer and more efficient operation.
Another frontier in steam turbine science involves environmental considerations. The drive to reduce greenhouse gas emissions has led to improvements in fuel efficiency and the development of cleaner steam generation methods. Additionally, new materials and coatings are being researched to withstand corrosive steam environments, particularly when using alternative fuels or recycling steam in innovative ways. These advances ensure that steam turbines remain relevant in a future where sustainability and carbon footprint are paramount concerns.
Furthermore, ongoing scientific investigation explores how microstructural changes in turbine materials occur over time due to exposure to high temperatures and stresses, a phenomenon known as creep. Understanding creep and related fatigue mechanisms enables engineers to design maintenance schedules and component replacements that maximize turbine lifespan without risking catastrophic failures. The interplay between microscopic material science and large-scale mechanical design exemplifies the multi-scale nature of steam turbine science.
In essence, steam turbines represent a sophisticated blend of natural science and human ingenuity. The fundamental principles of thermodynamics, fluid dynamics, and materials science continue to be refined and applied in innovative ways, ensuring that steam turbines remain a backbone of power generation. This enduring technology exemplifies how deep scientific understanding, combined with practical engineering solutions, can meet complex global energy challenges both now and in the future.
Steam Power in Today’s Power Plants
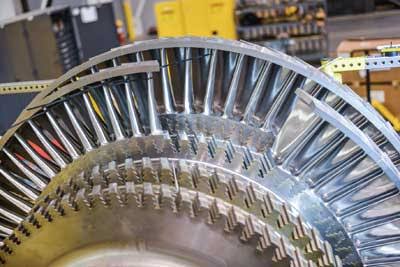
Steam power remains a cornerstone of modern electricity generation, underpinning a vast majority of the world’s power plants. Despite the rise of renewable energy sources like wind and solar, steam turbines continue to dominate because of their ability to efficiently convert thermal energy into large-scale mechanical and electrical power. Most coal-fired, nuclear, and many natural gas power plants rely heavily on steam turbines to generate electricity. In these plants, water is heated in boilers to create high-pressure steam, which then flows through steam turbines, spinning their rotors to drive electrical generators.
The versatility of steam turbines allows power plants to operate at different scales and under various fuel sources. Coal plants use steam generated by burning coal, nuclear plants produce steam through nuclear fission heat, and combined cycle plants integrate gas turbines with steam turbines by utilizing the waste heat from gas turbines to produce steam for additional power generation. This adaptability makes steam power a reliable and widely used technology for meeting the world’s baseload electricity demand—power that is needed continuously and consistently.
Modern power plants emphasize efficiency and emissions reduction, and steam turbine technology plays a critical role in these goals. By operating at higher steam pressures and temperatures, power plants can extract more energy from the fuel they consume, reducing fuel use per unit of electricity produced and cutting greenhouse gas emissions. Advanced materials and cooling technologies allow turbines to withstand these harsher conditions without sacrificing reliability. Moreover, innovations like reheating and regeneration in the steam cycle help to capture more energy and reduce waste heat.
Steam turbines are also integral to cogeneration or combined heat and power (CHP) systems, where the steam’s energy is used not only for electricity but also for industrial processes, district heating, or desalination. This multipurpose use of steam increases overall energy utilization efficiency and reduces waste, contributing to more sustainable and cost-effective energy solutions.
Despite their widespread use, steam turbines face challenges as the energy landscape shifts. The increasing penetration of intermittent renewables requires power plants to become more flexible, able to ramp output up and down quickly without damaging turbine components. Scientists and engineers are working on materials and control strategies that allow steam turbines to operate efficiently under variable loads, ensuring they remain relevant in a more dynamic grid.
In summary, steam power in today’s power plants combines mature, proven technology with ongoing innovation to meet the demands of modern energy systems. Through continual improvements in turbine design, materials, and operational strategies, steam turbines remain a vital component of the global energy infrastructure, balancing reliability, efficiency, and environmental responsibility in electricity generation.
Steam power’s role in today’s energy landscape is evolving alongside technological advancements and shifting demands for cleaner, more flexible energy sources. While steam turbines have traditionally been optimized for steady, continuous operation in large baseload power plants, the increasing integration of renewable energy sources like wind and solar has introduced variability into the grid. This has prompted a rethinking of how steam turbines are operated, pushing toward more flexible and responsive designs that can adjust output quickly without compromising efficiency or durability. This shift requires improvements not only in turbine materials and blade design but also in control systems that can manage rapid changes in steam flow and temperature.
The efficiency of steam power plants continues to improve through higher steam pressures and temperatures, enabled by breakthroughs in metallurgy and cooling technologies that allow turbine components to withstand extreme conditions. Supercritical and ultra-supercritical steam plants operate at pressures and temperatures well beyond traditional limits, extracting more energy from each unit of fuel and significantly reducing emissions per megawatt-hour generated. These advancements contribute to reducing the carbon footprint of fossil fuel-based power generation, which remains a critical step in transitioning to a lower-carbon energy future.
Moreover, combined cycle power plants represent a significant evolution in steam power’s role. By capturing the high-temperature exhaust gases from gas turbines to generate steam for steam turbines, combined cycle plants achieve thermal efficiencies that far surpass those of single-cycle plants. This efficient use of fuel reduces operational costs and greenhouse gas emissions, making combined cycle plants a preferred choice for natural gas power generation worldwide. Additionally, this configuration offers operational flexibility, allowing plants to ramp power output up or down more quickly than traditional steam-only plants.
Cogeneration or combined heat and power (CHP) plants further enhance the value of steam power by utilizing steam for both electricity generation and process heating. These plants are particularly common in industrial settings, where waste heat recovery and steam utilization improve overall energy efficiency and lower operational costs. By capturing and repurposing steam energy that might otherwise be lost, CHP systems help reduce fuel consumption and emissions while supporting industrial productivity.
Despite its many advantages, steam power does face challenges related to environmental concerns, particularly the emissions associated with burning fossil fuels. To address this, carbon capture and storage (CCS) technologies are increasingly being explored for integration with steam power plants. CCS involves capturing carbon dioxide emissions before they enter the atmosphere and storing them underground or using them in industrial processes. Although still developing, this approach has the potential to significantly reduce the environmental impact of steam-based power generation while maintaining its reliability and scale.
In the broader context of global energy transition, steam power’s adaptability is one of its greatest strengths. Whether through advanced materials that push operational limits, integration with renewable energy sources, or hybrid systems combining different power generation technologies, steam turbines remain central to meeting the world’s electricity needs. Their proven reliability, scalability, and efficiency ensure they will continue to play a vital role even as the energy sector evolves toward cleaner and more distributed power generation models. Steam power’s blend of mature science and ongoing innovation embodies the dynamic nature of modern energy systems, balancing tradition with transformation.
Looking ahead, the future of steam power in electricity generation is likely to be shaped by several converging trends in technology, policy, and environmental priorities. One important direction is the increasing emphasis on decarbonization. While steam turbines have traditionally relied on fossil fuels like coal and natural gas to generate the steam that drives them, there is growing interest in producing steam through low-carbon or carbon-neutral methods. For instance, advanced nuclear reactors can generate high-temperature steam without greenhouse gas emissions, offering a clean source of thermal energy for turbines. Similarly, emerging technologies like concentrated solar power (CSP) use mirrors to focus sunlight to heat fluids and generate steam, coupling renewable heat directly with steam turbines.
Hydrogen is another promising fuel for steam power plants. When hydrogen is combusted, it produces water vapor instead of carbon dioxide, and that water vapor can be used to drive steam turbines. Although the infrastructure for hydrogen production and distribution is still developing, steam turbines can be adapted or designed to work with hydrogen combustion or even pure steam cycles generated from hydrogen-powered heat sources, potentially enabling zero-carbon steam power generation in the future.
In addition to cleaner fuel sources, digitalization is transforming steam turbine operation and maintenance. Sensors embedded throughout turbines now collect vast amounts of data on temperature, pressure, vibration, and wear in real time. Advanced analytics and machine learning algorithms analyze this data to predict when maintenance will be needed, optimize operating conditions, and detect anomalies before they lead to failures. This predictive maintenance approach not only reduces downtime and repair costs but also extends turbine life, enhances safety, and improves overall plant efficiency.
On the materials front, research continues into developing new alloys and coatings capable of withstanding even higher temperatures and more aggressive steam chemistries. These advances allow turbines to operate closer to theoretical thermodynamic limits, further increasing efficiency and reducing emissions per unit of electricity generated. Additionally, additive manufacturing (3D printing) techniques are beginning to be used to produce complex turbine components with precision and potentially lower production costs, opening new possibilities in turbine design and customization.
Moreover, the evolving energy grid places new demands on steam turbines in terms of flexibility and responsiveness. As renewable energy penetration increases, steam turbines will need to ramp up and down more frequently and quickly to balance supply and demand. This requires improvements in turbine control systems, better thermal management to reduce stresses from temperature cycling, and design adaptations to accommodate variable load operation without sacrificing reliability.
Finally, the integration of steam turbines within hybrid energy systems is gaining attention. For example, pairing steam turbines with energy storage technologies or renewable hydrogen production facilities could help balance intermittent renewable generation and provide reliable, dispatchable power. Such hybrid configurations could be key in achieving net-zero emissions goals while maintaining grid stability.
In conclusion, while steam turbines are a mature and well-established technology, their role in today’s and tomorrow’s energy systems is far from static. Driven by innovation in fuels, materials, digital technologies, and system integration, steam power continues to adapt and evolve. This ongoing transformation ensures that steam turbines will remain a foundational element of global electricity generation, bridging the gap between traditional power generation methods and the sustainable energy future.
How Steam Turbines Work: A Beginner’s Guide
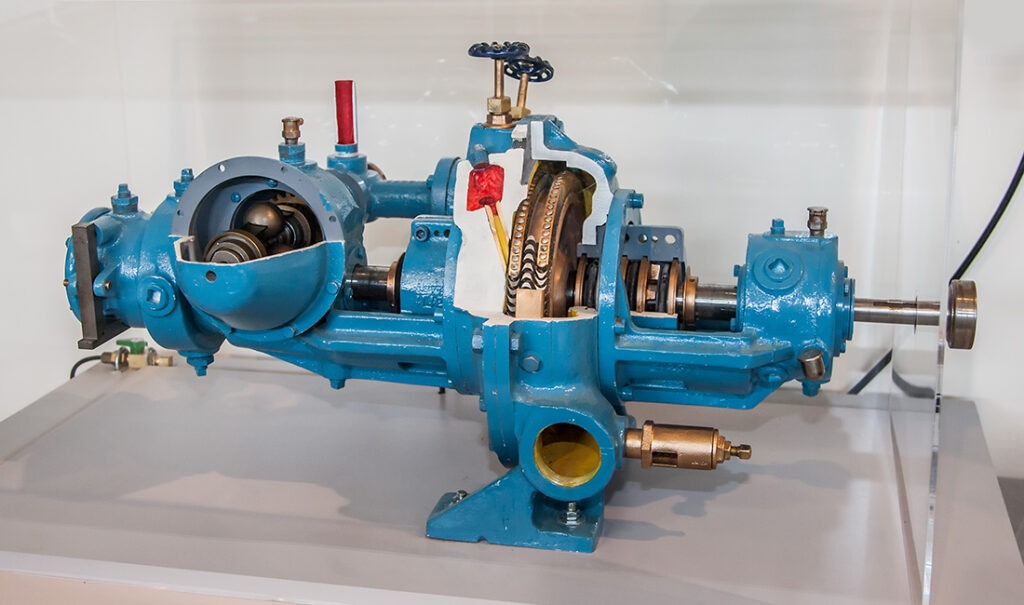
Steam turbines work by converting the energy stored in pressurized steam into mechanical energy that can drive generators or other machinery. The process begins in a boiler, where water is heated until it becomes high-pressure, high-temperature steam. This steam is then directed into the turbine, where it flows over a series of carefully shaped blades attached to a rotating shaft called the rotor. As the steam passes through the turbine blades, it expands and loses pressure, transferring its energy to the blades and causing the rotor to spin.
At the heart of the steam turbine’s operation is the conversion of thermal energy into kinetic energy, and then into mechanical energy. When steam is generated, it contains both heat (thermal energy) and pressure. As it enters the turbine, it is forced through nozzles that accelerate the steam, increasing its velocity and turning much of its pressure energy into kinetic energy. This high-speed steam then hits the turbine blades, which are arranged to capture the steam’s momentum effectively. The impact or reaction forces exerted by the steam cause the blades and attached rotor to turn, creating rotational mechanical energy.
There are two main types of turbines based on how they use steam energy: impulse and reaction turbines. In an impulse turbine, steam is expanded in nozzles before hitting the blades, so the blades mainly receive kinetic energy from the high-speed steam jet. In contrast, reaction turbines extract energy from both the steam’s pressure drop and velocity change as it flows continuously over moving blades, which are shaped like airfoils to create a reactive force that spins the rotor. Many modern turbines combine these two principles to maximize efficiency.
The turbine shaft is connected to a generator or mechanical equipment, so as the rotor spins, it produces electrical power or drives machinery. Steam exits the turbine at a much lower pressure and temperature, often entering a condenser where it is cooled and converted back into water to be reused in the boiler, completing the cycle.
Steam turbines are designed with multiple stages of blades arranged to gradually extract energy from the steam as it expands and slows down. This multi-stage design allows for efficient energy conversion over a wide range of steam pressures. The blades themselves are precisely engineered to withstand high temperatures, pressures, and stresses, often made from advanced alloys and cooled internally to prevent damage.
In summary, steam turbines work by harnessing the energy of expanding steam to spin blades attached to a rotor, converting thermal energy into mechanical energy that can be used for power generation or industrial applications. This elegant process is a cornerstone of modern power plants and industrial systems, providing reliable and efficient energy conversion.
As steam passes through the turbine stages, it gradually loses pressure and temperature, releasing its stored energy in a controlled manner. Each stage consists of a set of stationary blades, called nozzles or stators, and a set of rotating blades attached to the rotor. The stationary blades direct the flow of steam onto the rotating blades at the optimal angle, ensuring maximum transfer of energy. The rotating blades then convert the steam’s kinetic energy into mechanical energy, causing the rotor to spin. This continuous flow and expansion of steam through multiple stages allow the turbine to efficiently extract as much energy as possible.
The design of turbine blades is crucial for performance. They must be aerodynamically shaped to minimize losses due to turbulence and drag, and they are typically made from high-strength alloys that can withstand intense heat and pressure. In some advanced turbines, internal cooling passages within the blades help dissipate heat, prolonging the blade’s life and maintaining efficiency. Precision manufacturing and balancing of the rotor and blades ensure smooth operation at very high rotational speeds, often thousands of revolutions per minute, which is necessary for efficient electricity generation.
To maximize efficiency, steam turbines operate under specific conditions tailored to their intended application. For example, turbines in power plants often run with superheated steam—steam heated beyond its boiling point without moisture—to prevent blade erosion caused by water droplets. After expanding through the turbine, the steam is condensed back into water in a condenser to maintain a low pressure at the turbine exhaust, improving the pressure difference across the turbine and thus its efficiency.
Steam turbines are commonly used in conjunction with other systems to form thermodynamic cycles, like the Rankine cycle, which includes the boiler, turbine, condenser, and feedwater pump. This closed-loop system allows continuous generation of steam, expansion to produce power, condensation, and recycling of water. In combined cycle plants, steam turbines work alongside gas turbines to utilize waste heat effectively, further improving fuel efficiency and reducing emissions.
The simplicity and reliability of steam turbines, combined with their ability to handle large amounts of energy, make them indispensable in many industries beyond electricity generation, including marine propulsion, mechanical drives for compressors and pumps, and industrial processes requiring high-power output.
Understanding how steam turbines work provides insight into one of the most important technologies for converting heat into useful energy. Through precise engineering and sophisticated thermodynamic principles, steam turbines efficiently transform the force of expanding steam into rotational power, driving much of the world’s electricity and industrial activity with remarkable reliability and efficiency.
Steam turbines also rely heavily on the management of steam quality throughout the process to maintain performance and longevity. Moisture in steam can cause serious damage to turbine blades, as water droplets striking blades at high velocity lead to erosion and corrosion. To mitigate this, steam is often superheated to reduce moisture content before entering the turbine, ensuring that it remains dry and efficient during expansion. Additionally, some turbines include moisture separators or reheaters that remove or reduce moisture partway through the turbine stages, protecting downstream blades and maintaining optimal efficiency.
The efficiency of a steam turbine is influenced by how well it can convert the energy in the steam into mechanical energy with minimal losses. These losses arise from friction, turbulence, heat dissipation, and leakage of steam past seals and clearances. Engineers carefully design seals and blade clearances to minimize steam leakage, while advanced aerodynamic designs reduce turbulence and friction losses. Material advancements help minimize thermal losses by improving insulation and blade heat resistance. Collectively, these design considerations help achieve efficiencies that can exceed 40% in modern steam turbines, with combined cycle plants reaching overall efficiencies above 60%.
Operational control of steam turbines is another critical aspect. To ensure safe and efficient operation, turbines are equipped with control systems that regulate steam flow, temperature, and pressure. These systems can adjust valves and nozzles to respond to changing load demands or grid requirements. Additionally, protective mechanisms detect abnormal conditions like overspeed, vibration, or excessive temperature, automatically shutting down the turbine if necessary to prevent damage.
Maintenance is vital to the long-term performance of steam turbines. Regular inspections look for blade erosion, corrosion, wear in bearings, and alignment issues. Turbine blades may be repaired or replaced, and lubrication systems are closely monitored to avoid friction-related damage. Modern predictive maintenance techniques use sensor data and machine learning models to forecast potential failures before they occur, reducing downtime and repair costs.
Steam turbines’ combination of reliability, scalability, and efficiency has made them a fundamental technology in power generation for over a century. Their ability to convert heat energy from various fuel sources into mechanical power reliably and at scale continues to underpin electricity supply worldwide. As the energy landscape evolves toward cleaner sources and smarter grids, steam turbines are adapting with innovations in materials, controls, and integration with renewable technologies, ensuring they remain a key player in the global energy system for decades to come.
Key Components of a Steam Turbine
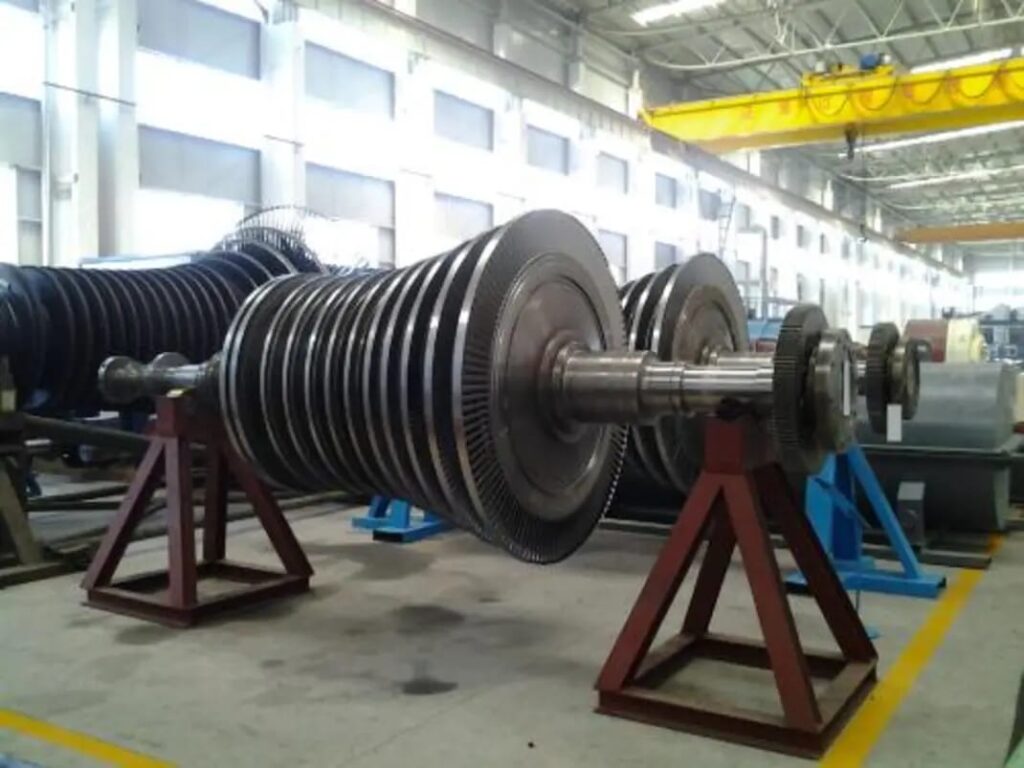
A steam turbine consists of several key components that work together to convert steam’s thermal energy into mechanical energy efficiently. The most essential parts include the rotor, blades, casing, nozzles, bearings, shaft, and the condenser system.
The rotor is the central rotating shaft of the turbine, to which the blades are attached. As steam passes over the blades, it causes the rotor to spin, transferring mechanical energy through the shaft to a generator or other machinery. The rotor must be precisely balanced and engineered to withstand high rotational speeds and stresses.
Blades are fixed onto the rotor in multiple stages and are designed to capture the energy of the steam. There are stationary blades, or nozzles, that direct the steam flow onto the moving blades at the right angle, and moving blades that convert steam momentum into rotational energy. Blades are typically made from high-strength alloys and sometimes feature internal cooling passages to handle extreme temperatures.
The casing encloses the turbine and directs steam flow through the blade stages. It maintains pressure containment and protects internal components. The casing also provides mounting points for bearings and seals that help maintain the turbine’s structural integrity and prevent steam leakage.
Nozzles are a type of stationary blade that accelerate and direct the steam flow onto the moving blades. By converting steam pressure into velocity, nozzles play a critical role in energy transfer efficiency. Some turbines use fixed nozzles, while others may have adjustable nozzles to control steam flow and turbine speed.
Bearings support the rotating shaft and maintain alignment during operation. They reduce friction between moving parts and absorb mechanical loads, ensuring smooth rotation at high speeds. Bearings must be durable and often use advanced lubrication systems to prevent wear.
The shaft connects the rotor to external equipment such as an electrical generator. It must transmit torque reliably without excessive vibration or bending, making its design critical to turbine performance and longevity.
Finally, the condenser is a key part of the steam cycle connected to the turbine exhaust. It cools and condenses the spent steam back into water, creating a low-pressure environment that improves the steam expansion in the turbine and allows the water to be recycled in the boiler. The condenser often uses cooling water from a river, lake, or cooling tower to remove heat.
Together, these components form the complex system of a steam turbine, working in unison to efficiently convert steam energy into mechanical power that drives much of the world’s electricity generation and industrial processes.
Beyond the primary components, there are several auxiliary systems that support the efficient and safe operation of a steam turbine. These include lubrication systems that supply oil to bearings and moving parts to reduce friction and wear, cooling systems that manage the heat generated during operation to prevent overheating, and sealing systems that minimize steam leakage around the shaft and between casing sections. Effective sealing is vital because even small leaks can reduce efficiency and increase operational costs.
Control systems are also crucial, monitoring parameters such as steam pressure, temperature, rotor speed, and vibration. These systems adjust valves and nozzles to regulate steam flow and turbine speed according to demand. In case of abnormal conditions like overspeed or excessive vibration, control systems initiate safety protocols to shut down the turbine and prevent damage. Modern turbines often employ digital monitoring and diagnostic tools, enabling predictive maintenance and real-time performance optimization.
The materials used in turbine components must withstand extreme conditions, including high temperatures, pressures, and corrosive steam environments. Advanced alloys with excellent strength and thermal resistance are standard, sometimes enhanced with protective coatings. Research into new materials and manufacturing techniques, such as additive manufacturing, is ongoing to improve component durability, reduce weight, and enable more complex blade geometries for better aerodynamic performance.
Maintenance and inspection of turbine components are critical to ensuring reliability and longevity. Turbine blades, for instance, may be inspected for signs of erosion, cracking, or deformation, which can occur due to high-speed steam flow and thermal cycling. Regular alignment checks, bearing condition monitoring, and lubrication system inspections help avoid mechanical failures. Planned maintenance schedules combined with condition-based monitoring reduce unexpected downtime and extend turbine service life.
Overall, the key components and supporting systems of a steam turbine work together to transform high-pressure steam into rotational energy safely and efficiently. The interplay between mechanical design, materials science, thermodynamics, and control technology ensures that steam turbines remain a highly effective solution for large-scale power generation and industrial applications around the world.
In addition to the core mechanical and control components, steam turbines are often integrated into larger systems that enhance their performance and efficiency. For example, many turbines incorporate reheaters that take partially expanded steam from an intermediate stage, reheat it in the boiler, and send it back into the turbine for further expansion. This process increases the average temperature at which heat is added, improving overall thermal efficiency and reducing moisture content in the later stages, which protects the blades.
Regenerative feedwater heating is another common feature, where steam extracted from various turbine stages is used to preheat the water entering the boiler. By raising the temperature of the feedwater before it reaches the boiler, less fuel is needed to convert it into steam, boosting the plant’s overall efficiency. This method recycles energy within the cycle, making the steam turbine system more economical and environmentally friendly.
Steam turbines also vary in design depending on their application. For instance, condensing turbines operate with a condenser at the exhaust to maximize pressure drop and efficiency, typical for power generation. Back-pressure turbines exhaust steam at higher pressures for use in industrial processes or heating applications. Extraction turbines allow steam to be drawn off at intermediate pressures for heating or process use while still producing mechanical power, making them ideal for combined heat and power (CHP) plants.
The scale of steam turbines can range from small units used in industrial facilities to massive machines that generate hundreds of megawatts in utility power plants. Larger turbines generally have more stages and operate at higher pressures and temperatures to maximize efficiency. They also require more sophisticated control and monitoring systems to manage the complex interplay of steam flow, temperature, and mechanical stresses.
Environmental considerations are increasingly influencing steam turbine design and operation. Efforts to reduce emissions have led to the adoption of cleaner fuels, improved combustion technologies in boilers, and integration with carbon capture systems. Additionally, the development of advanced materials and coatings helps turbines withstand more aggressive steam chemistries, including those associated with biomass or waste-derived fuels.
In summary, steam turbines are not just isolated machines but integral parts of sophisticated energy conversion systems that combine mechanical engineering, thermodynamics, materials science, and environmental technology. Their continuous evolution reflects the need for efficient, reliable, and cleaner energy solutions to meet global demands now and into the future.
The Role of Steam in Oil Refineries
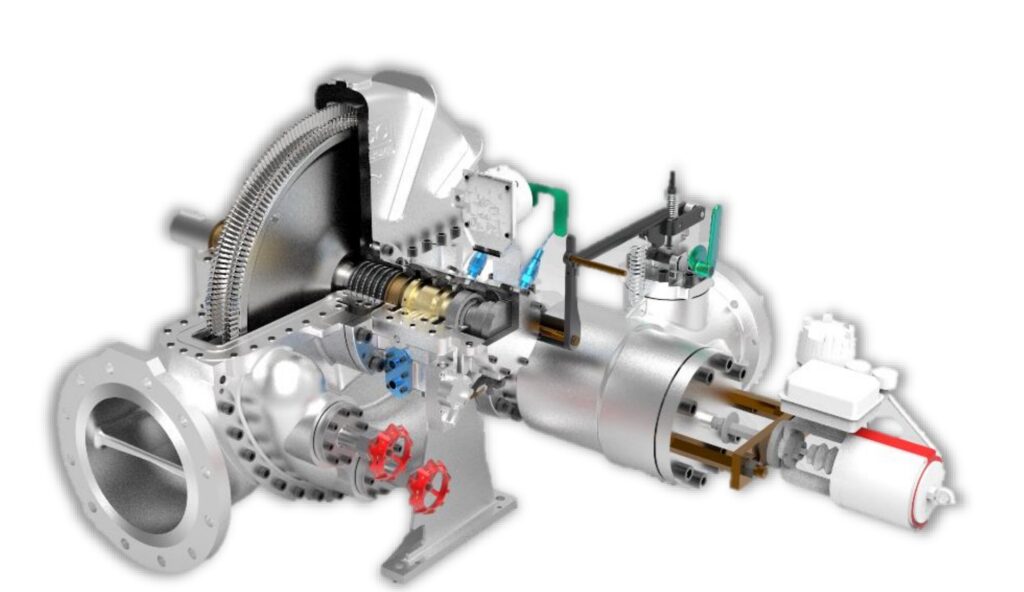
Steam plays a vital and multifaceted role in oil refineries, serving as a key utility that supports numerous processes essential for refining crude oil into valuable products like gasoline, diesel, jet fuel, and petrochemicals. Its importance lies not only in providing heat and energy but also in enabling precise control of chemical reactions, equipment operation, and safety measures throughout the refinery.
One of the primary uses of steam in refineries is heating. Many refining processes, such as distillation, catalytic cracking, and hydroprocessing, require precise temperature control to separate hydrocarbons or facilitate chemical transformations. Steam is used in heat exchangers, reboilers, and furnaces to transfer heat efficiently, maintaining optimal conditions for these complex processes. Because steam can carry large amounts of thermal energy at controlled temperatures and pressures, it offers a reliable and adjustable heat source.
Steam is also crucial for driving mechanical equipment. Steam turbines power compressors, pumps, and generators within the refinery, providing a dependable source of mechanical energy. These turbines convert thermal energy from steam into rotational energy, which in turn drives various machines essential for moving fluids, compressing gases, or generating electricity on-site, reducing the refinery’s dependence on external power sources.
In addition to heating and mechanical power, steam serves a key role in stripping and cleaning operations. For example, steam stripping is used to remove light hydrocarbons, impurities, or unwanted components from liquid streams. Injecting steam into vessels or pipelines helps separate volatile compounds from heavier fractions, improving product purity and process efficiency. Steam also aids in cleaning equipment by removing fouling or deposits through thermal and physical action.
Steam’s presence is critical for maintaining safety and operational stability. In some processes, steam is used to maintain pressure, prevent clogging or freezing in pipelines, and ensure the smooth flow of viscous fluids. It also acts as an inert medium in certain reactions, reducing the risk of unwanted side reactions or explosions. Moreover, steam can be used to control emissions by aiding in the combustion of volatile organic compounds in flare systems or thermal oxidizers.
Refineries often operate their own steam generation plants, typically fueled by refinery gas or other byproducts, to ensure a steady and cost-effective steam supply. The steam produced is carefully distributed across the facility through an extensive network of insulated pipes, with pressure levels tailored to different process requirements. High-pressure steam may be used for power generation and heavy heating, while lower-pressure steam supports process heating, stripping, or cleaning.
Efficient steam management is essential to refinery economics and environmental performance. Recovering condensate (the water formed when steam cools) and returning it to the boiler conserves water and energy, reducing fuel consumption and emissions. Advanced control systems monitor steam usage and optimize distribution, ensuring minimal waste and maximum process effectiveness.
In essence, steam acts as the lifeblood of oil refineries, enabling critical heat transfer, power generation, process control, and safety functions. Its versatility, reliability, and efficiency make it indispensable in transforming crude oil into the wide array of products society depends on, all while supporting the refinery’s operational integrity and sustainability goals.
Beyond its direct applications in heating and power, steam in oil refineries also plays a strategic role in enhancing process flexibility and responsiveness. Refinery operations often need to adjust rapidly to changing feedstock qualities, product demands, or regulatory requirements, and steam systems provide a controllable, on-demand source of thermal and mechanical energy to support these shifts. For example, during start-up, shutdown, or turnaround maintenance activities, steam is used extensively to safely warm up equipment, prevent condensation, and maintain pressure balance, reducing thermal stress and minimizing downtime.
Steam also facilitates catalytic reactions essential to refining processes. In hydrocracking and hydrotreating units, steam helps maintain reactor temperatures and improves the contact between hydrogen and hydrocarbons, enabling cleaner and more efficient chemical conversions. By controlling moisture levels and temperatures, steam helps optimize catalyst performance and lifespan, which are critical for refinery economics.
Furthermore, steam injection is used in enhanced oil recovery methods and in specific refining processes such as delayed coking, where steam prevents coke buildup and aids in the handling of heavy residues. In thermal cracking processes, steam helps reduce the partial pressure of hydrocarbons, favoring the breakdown of large molecules into more valuable lighter fractions.
The integration of steam systems with other utilities in the refinery, such as compressed air, cooling water, and electrical power, underscores its centrality to operational coordination. Control systems monitor steam quality, pressure, and flow rates to ensure each process receives the right steam conditions, avoiding disruptions or inefficiencies. Advanced monitoring and automation allow refineries to optimize steam consumption dynamically, balancing energy costs with process needs and environmental targets.
Environmental considerations are increasingly shaping steam usage in refineries. Efforts to reduce greenhouse gas emissions have driven improvements in steam generation efficiency, such as using combined heat and power (CHP) systems that simultaneously produce electricity and steam from the same fuel source. Recovery of waste heat from process streams to generate steam also enhances overall energy efficiency and lowers the refinery’s carbon footprint.
In summary, steam is deeply embedded in the fabric of oil refinery operations, far beyond a simple heating medium or power source. Its roles span thermal management, mechanical drive, chemical process enhancement, safety assurance, and environmental performance. This versatility makes steam an indispensable utility in refining, enabling the complex transformations required to turn crude oil into the diverse fuels and chemicals that power modern life.
In addition to its core functions, steam also plays a critical role in supporting the reliability and longevity of refinery equipment. Many refinery processes operate under extreme temperatures and pressures, which can cause significant wear and corrosion over time. Steam is used in cleaning and purging operations to prevent the buildup of deposits, scale, and other contaminants that could impair equipment performance or lead to failures. For example, steam blowouts are routine maintenance procedures where high-pressure steam is released to clear pipelines, vessels, and heat exchangers, removing coke deposits, residues, and other fouling materials. This helps maintain efficient heat transfer and fluid flow, reducing downtime and costly repairs.
Steam is also essential for instrument calibration and control system functioning. In control valves and pressure regulators, steam is used to actuate mechanisms, enabling precise control of flow rates and pressures within various process units. This accuracy is vital for maintaining optimal operating conditions, ensuring safety, and meeting product specifications.
Another key area where steam’s role is expanding is in the integration of refineries with renewable energy and sustainability initiatives. Some modern refineries are exploring the use of renewable sources such as biomass or biogas to generate steam, reducing reliance on fossil fuels and cutting emissions. Additionally, advanced carbon capture and utilization technologies often rely on steam to regenerate solvents or drive chemical reactions involved in capturing CO₂ from flue gases, thereby helping refineries meet increasingly stringent environmental regulations.
The steam system’s design and operation are complex and highly engineered to balance the diverse demands of refinery processes. Engineers must carefully consider factors such as steam pressure levels, temperature control, condensate return, water treatment, and system insulation to optimize performance and minimize energy losses. Proper maintenance of steam traps, valves, and pipelines is crucial to prevent leaks, which can waste energy and pose safety hazards.
In essence, steam is not only a versatile energy carrier within oil refineries but also a vital enabler of operational efficiency, equipment protection, process flexibility, and environmental stewardship. Its integration into refinery systems exemplifies the sophisticated interplay between thermodynamics, mechanical engineering, and chemical processing that defines modern refining technology. As refineries evolve to meet future energy and environmental challenges, the role of steam will continue to adapt, maintaining its position as a cornerstone of refinery operations worldwide.
Steam Systems in the Food & Beverage Industry
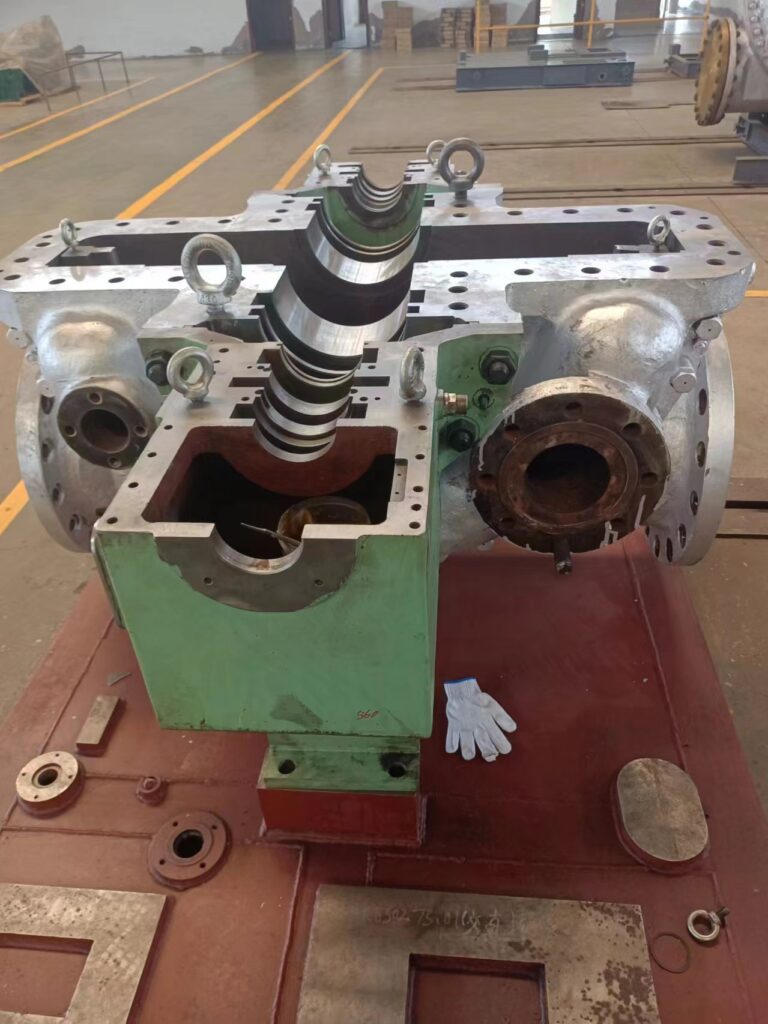
Steam systems are fundamental to the food and beverage industry, providing essential functions such as heating, sterilization, cooking, cleaning, and drying. The versatility, cleanliness, and controllability of steam make it an ideal utility in processes that demand strict hygiene, precise temperature control, and energy efficiency.
One of the primary uses of steam in food processing is sterilization and pasteurization. Steam’s high temperature effectively kills bacteria, molds, and other microorganisms on equipment surfaces, packaging, and food products themselves. This ensures product safety and extends shelf life. For instance, steam sterilization is widely used in dairy processing, canned foods, and bottled beverages to meet stringent health standards.
Steam is also employed in cooking and processing food products. It provides uniform heat transfer, which is critical for consistent product quality, whether in baking, blanching vegetables, cooking meats, or producing ready-to-eat meals. Steam’s ability to penetrate and heat quickly without direct contact helps preserve texture, flavor, and nutritional value.
In addition to cooking and sterilization, steam is used for cleaning and sanitation. Clean-in-place (CIP) systems utilize steam to disinfect pipelines, tanks, and processing equipment without disassembly. This reduces downtime and contamination risks, essential in maintaining continuous and safe production.
Drying is another important application where steam-generated heat removes moisture from food products, powders, or packaging materials. Controlled steam heating ensures efficient drying while preventing damage from overheating.
Steam also drives mechanical equipment within food plants. Steam turbines and engines can power mixers, conveyors, and pumps, providing reliable and clean mechanical energy that supports production processes.
The food and beverage industry often requires steam at different pressure levels. High-pressure steam may be used for sterilization or power generation, while lower-pressure steam serves heating, cooking, and cleaning purposes. Steam quality is critical, as contaminants or excessive moisture can affect product purity and process efficiency. Therefore, food-grade steam generation involves stringent water treatment, filtration, and monitoring.
Energy efficiency and sustainability are growing priorities. Many food processors integrate waste heat recovery systems to capture and reuse steam energy, reducing fuel consumption and emissions. Automation and control systems optimize steam usage by regulating pressure, flow, and temperature in real time, improving productivity and lowering costs.
In summary, steam systems are a cornerstone of the food and beverage industry, enabling safe, efficient, and high-quality production. Their ability to deliver clean, controllable heat and power supports diverse processing needs while meeting strict hygiene and environmental standards essential for consumer safety and product excellence.
Steam’s role in the food and beverage industry extends beyond basic heating and sterilization; it is deeply integrated into the entire production lifecycle, helping to ensure not only product quality but also operational reliability and regulatory compliance. Many processes rely on precisely controlled steam to maintain the consistency and safety of food products, which is critical given the strict standards imposed by food safety authorities worldwide. For example, in brewing and beverage production, steam is used to control fermentation temperatures, clean tanks, and sterilize bottles, ensuring that the final product meets taste and safety expectations.
The design of steam systems in food processing plants must prioritize hygiene and contamination prevention. This means that steam pipelines, valves, and equipment are constructed from stainless steel or other corrosion-resistant materials that are easy to clean and do not harbor bacteria. Steam traps and condensate removal systems are carefully maintained to prevent water accumulation, which could compromise steam quality and lead to product defects or microbial growth.
Furthermore, steam systems contribute significantly to energy management within food facilities. Since steam generation is energy-intensive, optimizing boiler performance, minimizing steam leaks, and recovering condensate are critical practices to reduce fuel use and operating costs. Many plants implement sophisticated monitoring and control technologies that allow operators to adjust steam parameters dynamically based on real-time production needs, thus avoiding energy waste and ensuring process stability.
In addition to supporting production processes, steam plays an important role in maintenance and safety protocols. It is used for sterilizing tools and workspaces, ensuring that equipment meets stringent sanitation standards before and after production runs. In cleaning operations, steam’s high temperature and pressure enable effective removal of residues and biofilms without the need for harsh chemicals, which aligns with consumer demand for cleaner, greener production methods.
Looking ahead, innovations in steam technology continue to enhance its role in the food and beverage industry. Advances such as low-pressure steam systems, more efficient boilers, and integration with renewable energy sources help reduce environmental impact while maintaining the high levels of control and hygiene that the industry requires. As consumer expectations evolve and regulations tighten, steam systems will remain indispensable, adapting through innovation to support safer, more efficient, and more sustainable food production worldwide.
Beyond production and sanitation, steam also plays a pivotal role in packaging processes within the food and beverage industry. Steam is used to sterilize packaging materials such as bottles, cans, and cartons before they are filled, ensuring that no contaminants compromise the product’s shelf life or safety. This is especially important in aseptic packaging, where sterile conditions are crucial to prevent spoilage without refrigeration. The rapid, uniform heating properties of steam make it ideal for such applications, allowing for quick turnaround times and high throughput on packaging lines.
In addition, steam is instrumental in climate control and humidity regulation within processing and storage areas. Maintaining the right humidity levels helps preserve product quality, prevents microbial growth, and reduces static buildup that can interfere with automated machinery. Steam humidification systems provide a controllable and clean way to manage indoor environments, supporting both food safety and operational efficiency.
The integration of steam systems with other utilities, such as compressed air, refrigeration, and water treatment, creates a comprehensive infrastructure that supports the complex needs of modern food manufacturing. For instance, the condensate collected from steam systems is often treated and reused as boiler feedwater, enhancing water efficiency and reducing waste. Advanced steam management software helps operators monitor system performance, predict maintenance needs, and optimize energy use, aligning operational goals with sustainability targets.
Moreover, steam’s versatility allows it to adapt to diverse food industry sectors, from bakery and confectionery to meat processing and dairy production. Each application demands specific steam qualities and delivery methods, tailored to the unique thermal and hygienic requirements of the products involved. This adaptability reinforces steam’s position as a fundamental utility, capable of meeting the evolving challenges of food production worldwide.
In summary, steam systems in the food and beverage industry are not merely support utilities but integral components of a sophisticated production ecosystem. They enable high standards of quality, safety, and efficiency while offering flexibility and sustainability, making steam indispensable in delivering the products that nourish and delight consumers globally.
Steam Engines in Space Research Concepts
Steam engines might sound like relics of the industrial age, but concepts involving steam power have intriguing applications and potential in space research and exploration. Although modern spacecraft primarily rely on chemical rockets, electric propulsion, and nuclear power, steam-based technologies are being explored for specific tasks where their unique properties could offer advantages, especially in resource utilization, environmental control, and propulsion on other planets or moons.
One key area of interest is in-situ resource utilization (ISRU), which aims to use materials found on extraterrestrial bodies to support space missions. On planets like Mars or the Moon, water ice deposits have been confirmed or are suspected, and extracting and using this water is crucial for sustaining human presence. Steam engines or turbines could be part of systems that convert locally sourced water into steam, providing mechanical power for drilling, excavation, or generating electricity without relying entirely on supplies from Earth. The relative simplicity and robustness of steam engines make them attractive candidates for such off-world applications, where repair opportunities are limited.
Another potential use for steam engines in space involves environmental control and life support systems (ECLSS). Steam can serve as a heat transfer medium to regulate temperatures inside habitats, greenhouses, or equipment enclosures. Its high heat capacity and phase-change properties allow efficient management of heat loads, critical for maintaining safe living and working conditions in harsh space environments.
Steam propulsion concepts have also been proposed for small-scale, low-thrust applications such as hopping rovers or landers on low-gravity bodies like asteroids, comets, or moons. By heating water or other volatiles and releasing steam jets, these vehicles could achieve short bursts of movement or adjust position with simpler, less expensive propulsion systems than traditional rocket engines. Such steam-powered “hoppers” could explore rugged terrain inaccessible to wheeled vehicles, increasing the scientific return of missions.
Thermal management in space probes and satellites is another domain where steam cycle principles might be adapted. The ability of steam to absorb, transfer, and reject heat efficiently can be harnessed in closed-loop heat engines or heat pumps that regulate onboard temperatures, ensuring the optimal operation of sensitive instruments.
Although still largely conceptual and experimental, the integration of steam engines into space research underscores the continuing relevance of classic thermodynamic principles in cutting-edge technology. Developing reliable, efficient, and versatile steam systems adapted for extraterrestrial environments could open new pathways for sustainable exploration, resource utilization, and habitat development beyond Earth.
In essence, steam engines in space research represent a fascinating fusion of old-world engineering and futuristic exploration, highlighting how even time-tested technologies can find new life in humanity’s quest to reach and thrive in the cosmos.
Building on these ideas, steam engines in space research also present promising opportunities for energy efficiency and system resilience. On long-duration missions, minimizing reliance on complex and failure-prone components is essential. Steam engines, with their relatively straightforward design and ability to use common materials like water, could offer a more robust alternative or complement to purely electric or chemical systems. The potential to regenerate and recycle steam repeatedly within closed environments aligns well with the sustainability requirements of off-world habitats.
In addition, the ability to produce steam from locally available resources reduces the mass and volume of supplies that must be launched from Earth, a major cost and logistical constraint in space missions. This makes steam-based power generation and propulsion attractive for establishing semi-permanent bases on the Moon or Mars, where extracting water ice and converting it into steam can power machinery, generate electricity, or facilitate mobility with reduced dependence on Earth resupply.
Research into microgravity effects on steam generation and turbine operation is ongoing, as fluid dynamics behave differently without gravity’s influence. Understanding these differences is crucial to designing efficient steam systems for space applications. Innovative solutions like capillary-driven steam flow and novel heat exchangers are being explored to ensure reliable operation in microgravity or low-gravity environments.
Moreover, hybrid systems combining steam engines with other technologies such as solar thermal collectors could enhance energy harvesting on planets with abundant sunlight. Solar energy can be used to heat water, generating steam to drive engines or turbines for power production or mechanical work. This hybrid approach leverages renewable energy sources and reduces fossil fuel dependence, critical for sustainable space exploration.
Ultimately, integrating steam engines into space exploration concepts reflects a broader trend of revisiting and adapting established technologies to meet new challenges. As humanity pushes further into space, combining the simplicity and reliability of steam power with advanced materials, automation, and resource utilization strategies may provide practical, cost-effective solutions that enhance mission success and longevity.
Thus, steam engines, far from being obsolete, hold untapped potential in the future of space research, serving as a bridge between traditional engineering principles and innovative extraterrestrial applications. Their continued development and testing could prove vital in expanding humanity’s presence beyond Earth, enabling more resilient, efficient, and sustainable exploration of the cosmos.
Expanding further, the development of steam engine technologies tailored for space environments also encourages interdisciplinary collaboration between aerospace engineers, mechanical engineers, and material scientists. Creating steam systems capable of withstanding the extreme temperatures, radiation, and vacuum conditions encountered in space requires innovations in materials that resist corrosion, fatigue, and thermal cycling. Advanced alloys, ceramics, and coatings are being investigated to ensure that steam turbines or pistons can operate reliably over long mission durations without frequent maintenance.
The modular nature of steam systems can also facilitate scalability and adaptability in space missions. Smaller steam engines could power robotic explorers or scientific instruments, while larger systems might support habitat infrastructure or industrial-scale operations such as mining and processing extraterrestrial materials. This flexibility allows mission planners to tailor steam-powered solutions to specific mission goals, environmental conditions, and available resources.
There is also potential synergy between steam systems and emerging technologies like additive manufacturing (3D printing) in space. Components for steam engines could be produced or repaired on-site using locally sourced materials, reducing dependency on Earth-based supply chains and enhancing mission autonomy. This approach aligns with the growing emphasis on in-situ manufacturing to support long-term space habitation and exploration.
From an educational and historical perspective, revisiting steam engines in space research provides an excellent opportunity to bridge classical engineering education with cutting-edge space science. It highlights the enduring value of thermodynamic principles and mechanical design while inspiring new generations of engineers to innovate by adapting proven technologies to novel challenges.
In conclusion, steam engines in space research are more than just an intriguing concept; they represent a practical avenue for developing sustainable, reliable, and efficient systems that can help humanity explore and inhabit new worlds. By leveraging steam power’s simplicity, adaptability, and synergy with local resource utilization, future space missions could achieve greater self-sufficiency, reduce costs, and open new frontiers in exploration, making steam engines an unlikely but promising hero of space technology’s next chapter.
Steam vs. Gas Turbines: A Technical Comparison
Steam turbines and gas turbines are two fundamental types of turbines widely used for power generation and mechanical drive applications, but they operate on different principles and have distinct characteristics that make each better suited for specific uses. Understanding their technical differences involves examining their working fluids, thermodynamic cycles, design, efficiency, operational aspects, and typical applications.
Steam turbines use steam — generated by boiling water in a boiler — as the working fluid. The high-pressure, high-temperature steam expands through a series of blades mounted on a rotating shaft, converting thermal energy into mechanical energy. Steam turbines generally operate on the Rankine cycle, which involves boiling water, expanding steam through the turbine, condensing the steam back to water, and then pumping the water back into the boiler. These turbines can be designed for high or low-pressure steam and are well suited for continuous, steady-state power generation. They are often used in large-scale power plants fueled by coal, nuclear, biomass, or waste heat recovery.
Gas turbines, on the other hand, use hot combustion gases—produced by burning fuel such as natural gas or liquid fuels—as the working fluid. Air is compressed, mixed with fuel, and ignited in a combustion chamber, producing high-temperature, high-pressure gases that expand through turbine blades to produce mechanical energy. Gas turbines operate on the Brayton cycle, which involves compressing air, combusting fuel with compressed air, expanding the hot gases through the turbine, and exhausting the gases. Gas turbines are known for quick start-up times, compact design, and high power-to-weight ratios, making them ideal for applications such as aircraft engines, peaking power plants, and mechanical drives.
In terms of efficiency, modern combined-cycle power plants leverage both turbine types by using the exhaust heat from gas turbines to generate steam for steam turbines, achieving efficiencies exceeding 60%. Individually, gas turbines typically have higher efficiency at smaller scales and variable loads, while steam turbines excel in large-scale, base-load applications with steady power output.
Steam turbines generally operate at lower rotational speeds than gas turbines, often requiring reduction gears for certain mechanical applications, while gas turbines rotate at very high speeds (tens of thousands of RPM) and typically connect directly to generators or compressors. The materials and cooling technologies differ as well; gas turbines must withstand extremely high combustion temperatures, necessitating advanced alloys and cooling methods, whereas steam turbines operate at lower temperatures but must handle high pressures and potential steam moisture.
Maintenance and operational considerations vary: steam turbines require careful water chemistry control to prevent corrosion and erosion from wet steam, whereas gas turbines need meticulous fuel quality and combustion management to avoid blade damage. Gas turbines also produce higher NOx emissions, requiring additional emission control technologies.
In summary, steam turbines are favored for large, continuous, and high-capacity power generation with fuels that enable steam production, while gas turbines provide flexible, fast-start, and compact power solutions. Their complementary characteristics are often combined in modern power plants to optimize performance, fuel efficiency, and environmental impact, showcasing how each technology’s strengths can be harnessed in tandem for advanced energy systems.
Delving deeper into their operational differences, steam turbines rely heavily on an external heat source to generate steam, making them dependent on boilers that burn fossil fuels, nuclear reactions, or utilize waste heat from other processes. This reliance means steam turbines are typically part of larger, more complex systems requiring significant infrastructure and time to start up or shut down. Their slow response to load changes makes them less suitable for applications where rapid power adjustments are needed but ideal for steady, continuous operation where efficiency and reliability over long periods are prioritized.
Gas turbines, in contrast, offer exceptional flexibility and rapid start-up capabilities, often reaching full power within minutes. This makes them highly valuable for peak load demands, emergency power supplies, or as part of combined-cycle plants where their exhaust heat is captured to drive steam turbines. Gas turbines’ compact size and lighter weight also allow their use in mobile or remote applications, such as powering aircraft, ships, or remote industrial facilities.
From a thermodynamic standpoint, the efficiency of steam turbines can be limited by the condensation phase in the Rankine cycle, where steam transitions back to liquid. The presence of moisture droplets can erode turbine blades and reduce efficiency, so careful management of steam quality is essential. Advances like supercritical and ultra-supercritical steam cycles have pushed steam turbine efficiencies higher by operating at extreme pressures and temperatures, but these require robust materials and complex designs.
Gas turbines operate with continuously flowing combustion gases, and their efficiency is influenced by the pressure ratio of the compressor and the turbine inlet temperature. Modern gas turbines utilize sophisticated cooling technologies and advanced materials such as single-crystal superalloys to withstand temperatures well above the melting points of their components. This technological evolution has enabled higher operating temperatures, boosting thermal efficiency.
In terms of environmental impact, both turbine types face challenges. Steam turbines, depending on their fuel source, can be associated with significant carbon emissions, especially when powered by coal or oil. Gas turbines burning natural gas emit less CO₂ and pollutants, but controlling nitrogen oxides (NOx) remains a critical concern. Innovations like dry low-NOx combustors and catalytic converters are commonly used to reduce emissions.
Operational costs also differ. Steam turbines typically have lower fuel costs per unit of electricity generated when operating at full load due to their high efficiency and ability to utilize cheaper fuels. However, they involve higher capital and maintenance costs because of their size and complexity. Gas turbines usually have higher fuel costs but lower capital expenditure and maintenance requirements, particularly in smaller installations.
Overall, choosing between steam and gas turbines depends on factors such as scale, fuel availability, required flexibility, environmental regulations, and economic considerations. The integration of both turbine types in combined-cycle plants exemplifies how their complementary attributes can be leveraged to maximize efficiency and minimize emissions, forming the backbone of modern power generation technology.
Further exploring their integration, combined-cycle power plants represent one of the most significant advancements in turbine technology by marrying steam and gas turbines to capitalize on their respective strengths. In these plants, a gas turbine generates electricity by combusting fuel and driving a generator, while its hot exhaust gases—still containing substantial thermal energy—are routed to a heat recovery steam generator (HRSG). The HRSG uses this heat to produce steam, which then drives a steam turbine to generate additional electricity. This cascade of energy use significantly boosts overall plant efficiency, often surpassing 60%, compared to about 35–40% for standalone steam or gas turbine plants.
This synergy not only enhances fuel utilization but also reduces greenhouse gas emissions per unit of electricity produced, aligning with increasingly strict environmental regulations and sustainability goals. Combined-cycle plants can also ramp power output up or down more rapidly than traditional steam-only plants, providing grid operators with valuable flexibility to balance variable renewable energy sources like wind and solar.
In terms of technology development, ongoing research aims to push the boundaries of both turbine types. For steam turbines, innovations include exploring ultra-supercritical steam conditions and novel blade materials to withstand even higher temperatures and pressures, which translate to better efficiency and power density. For gas turbines, advancements focus on raising turbine inlet temperatures, improving cooling techniques, and developing alternative fuels such as hydrogen to reduce carbon emissions.
Hydrogen, in particular, is gaining attention as a clean fuel that can be combusted in gas turbines with water vapor as the primary emission. This shift requires adapting turbine designs to handle different combustion characteristics, flame temperatures, and material stresses. On the steam side, hydrogen can also be used in boilers to generate steam without CO₂ emissions, further decarbonizing power generation.
Maintenance practices differ substantially as well. Steam turbines, with their large rotating masses and operating conditions, require regular inspections for blade integrity, shaft alignment, and sealing systems, along with rigorous water chemistry management to prevent corrosion and deposits. Gas turbines demand close monitoring of combustion chambers, turbine blades, and cooling systems, often employing sophisticated diagnostic tools to detect wear or damage before failures occur. Both turbine types benefit from predictive maintenance enabled by digital sensors and AI analytics, which improve reliability and reduce downtime.
In industrial contexts, steam turbines are often favored for their ability to handle large, steady loads and integrate with existing boiler infrastructure, while gas turbines are preferred for applications needing quick start-stop cycles or mobile power generation. Power plants, marine propulsion, and combined heat and power (CHP) systems also reflect this diversity, with turbines selected based on operational priorities, fuel availability, and economic factors.
In conclusion, while steam and gas turbines have distinct characteristics shaped by their thermodynamic cycles, working fluids, and mechanical designs, their ongoing evolution and strategic integration continue to drive efficient, flexible, and cleaner power generation worldwide. Understanding their technical differences and complementary roles is essential for engineers, operators, and policymakers aiming to optimize energy systems in an era of dynamic environmental and economic challenges.
Steam in Combined Heat and Power (CHP) Plants
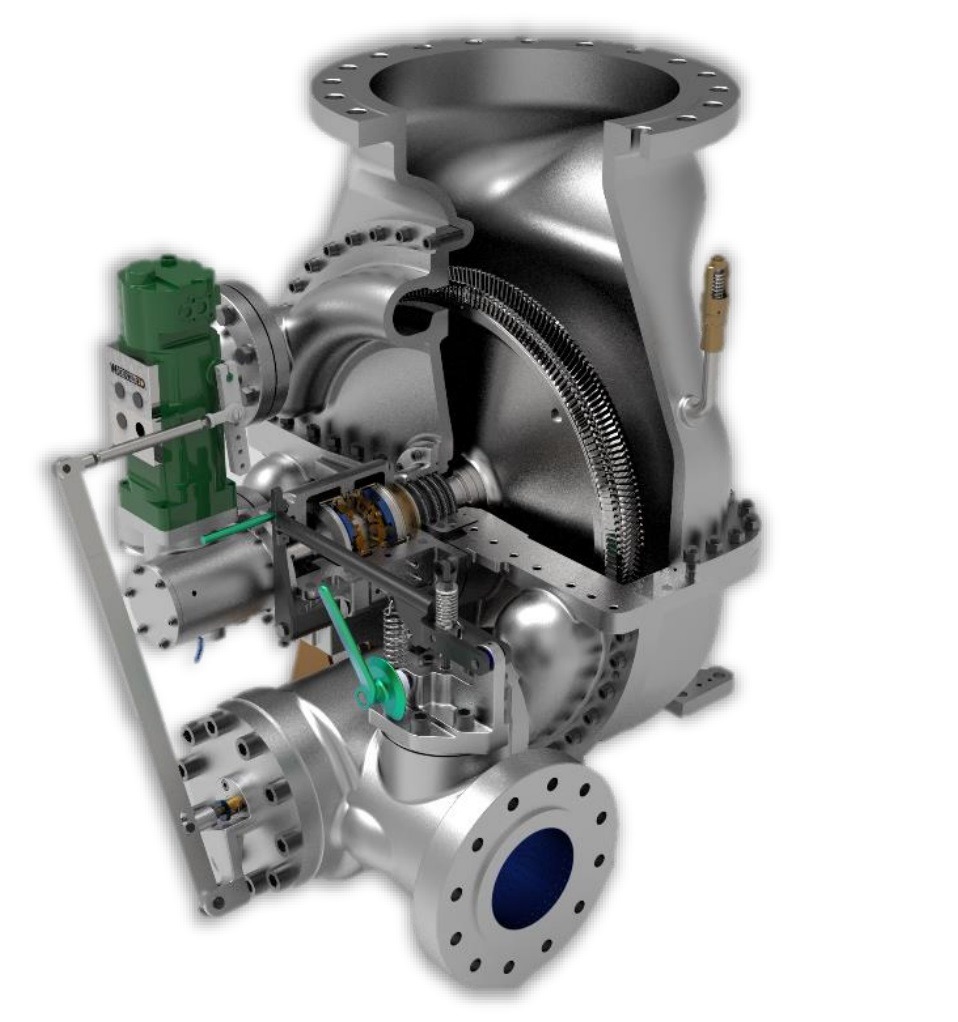
Steam plays a crucial role in Combined Heat and Power (CHP) plants, also known as cogeneration systems, which simultaneously generate electricity and useful thermal energy from a single fuel source. In these plants, steam is often the key working fluid that enables the efficient capture and utilization of energy that would otherwise be wasted in conventional power generation.
In a typical CHP setup, fuel—such as natural gas, biomass, coal, or waste—is combusted to produce high-pressure steam in a boiler or heat recovery steam generator (HRSG). This steam drives a steam turbine connected to an electrical generator, producing electricity. Instead of condensing and discarding the steam’s residual heat, CHP systems capture this thermal energy to provide heating or process steam for nearby industrial facilities, district heating networks, or other thermal applications.
The integration of steam turbines within CHP plants dramatically improves overall energy efficiency, often reaching total efficiencies of 70–90%, compared to around 35–50% for conventional power plants that discard waste heat. This efficiency gain translates to lower fuel consumption, reduced greenhouse gas emissions, and cost savings.
Steam conditions in CHP plants are tailored to match the thermal needs of the end-user. For example, high-pressure steam might be used to generate electricity, while lower-pressure steam extracted from intermediate turbine stages or directly from boilers is supplied for heating, sterilization, drying, or other industrial processes. This pressure cascade allows flexible and optimized use of steam energy.
CHP steam turbines are designed to operate reliably over wide load ranges, accommodating fluctuations in electricity and heat demand. Additionally, CHP plants may incorporate back-pressure steam turbines, where steam exhaust pressure remains high enough to be used directly for heating, eliminating the need for condensers and maximizing thermal output.
The use of steam in CHP extends beyond electricity and heating; it also supports absorption cooling systems, where steam drives chillers to provide air conditioning, further enhancing plant versatility and energy utilization. Moreover, steam in CHP systems can be generated from renewable sources, such as biomass or geothermal heat, enabling sustainable and low-carbon energy solutions.
Overall, steam’s adaptability, high energy density, and ability to deliver both mechanical and thermal energy make it indispensable in CHP plants. By effectively harnessing steam, these systems achieve superior energy performance, contributing significantly to energy conservation, environmental protection, and economic benefits in diverse industrial and urban applications.
In addition to improving efficiency and reducing emissions, steam’s role in CHP plants enhances energy security and reliability by enabling onsite power and heat generation. This decentralization reduces dependence on centralized grids, mitigates transmission losses, and provides resilience against grid disruptions, which is especially valuable for critical facilities like hospitals, universities, and manufacturing plants. The ability to produce steam for both power and thermal applications means that CHP plants can tailor energy output to meet fluctuating demands, maintaining stable operations and reducing waste.
The design of steam systems in CHP applications often incorporates advanced control strategies to optimize the balance between electricity generation and heat supply. Operators can adjust steam pressure and flow rates to prioritize either power output or thermal delivery depending on real-time requirements. This flexibility supports dynamic load management and integration with other energy sources, including renewables and energy storage, helping to stabilize overall energy systems.
Maintenance and operational considerations in steam-based CHP systems emphasize the importance of water quality, steam purity, and system integrity. Proper treatment of boiler feedwater and condensate recovery is essential to prevent scaling, corrosion, and turbine blade erosion, all of which can degrade performance and increase downtime. Automated monitoring and diagnostics are increasingly employed to detect early signs of issues and ensure smooth, efficient operation over long service periods.
Emerging trends in steam utilization within CHP plants include the integration of advanced materials and technologies to enable higher steam temperatures and pressures, thereby pushing efficiency boundaries further. Innovations such as supercritical and ultra-supercritical steam cycles, along with combined-cycle CHP configurations that use both gas and steam turbines, enable even greater energy recovery and lower emissions. These advancements also make CHP plants more adaptable to a variety of fuels, including hydrogen and biofuels, supporting the transition to cleaner energy systems.
Furthermore, steam’s versatility in CHP plants supports a broad range of industrial processes beyond simple heating, such as sterilization in food production, drying in paper manufacturing, chemical processing, and even enhanced oil recovery. By supplying process steam tailored to specific temperature and pressure requirements, CHP plants help industries reduce energy costs and improve sustainability.
In urban settings, district heating systems powered by steam-based CHP plants provide efficient, centralized heat distribution to residential and commercial buildings. This approach not only reduces carbon footprints compared to individual boilers but also leverages waste heat from electricity generation that would otherwise be lost, contributing to cleaner, smarter cities.
Ultimately, steam in combined heat and power plants embodies an efficient, flexible, and sustainable approach to energy utilization. Its capacity to deliver both electricity and useful heat from a single fuel source maximizes resource use, lowers emissions, and enhances energy resilience, making steam-driven CHP a vital technology in the ongoing efforts toward energy efficiency and climate goals worldwide.
Building further on steam’s role in combined heat and power plants, the future outlook for steam-based CHP systems is closely tied to advancements in digitalization and smart energy management. The incorporation of sensors, IoT devices, and AI-driven analytics enables real-time monitoring of steam quality, turbine performance, and thermal load demands. This data-driven approach allows operators to optimize steam production, reduce fuel consumption, and predict maintenance needs before failures occur, thus improving reliability and lowering operational costs.
Moreover, integrating steam-based CHP plants with renewable energy sources and energy storage systems is an emerging trend that enhances grid flexibility and supports decarbonization efforts. For example, during periods of high renewable generation, CHP plants can adjust steam output to complement variable energy supply, storing thermal energy in insulated tanks or thermal batteries for later use. This hybrid operation increases overall system efficiency and stability, enabling a smoother transition to low-carbon energy systems.
The adaptation of CHP plants to use alternative and cleaner fuels, such as green hydrogen, biomethane, and synthetic fuels, further extends the relevance of steam technology in a decarbonizing energy landscape. These fuels can be combusted in boilers or gas turbines to generate steam without the carbon emissions associated with fossil fuels, supporting climate targets while maintaining the benefits of cogeneration.
On the engineering front, ongoing research is focused on developing compact, modular steam turbine designs suitable for smaller-scale CHP applications, such as commercial buildings, hospitals, and remote communities. These scalable solutions can bring the efficiency and reliability of steam cogeneration to a wider range of users, including those who currently rely on less efficient, separate heat and power sources.
In addition, advances in materials science are enabling steam turbines to operate safely at higher temperatures and pressures, pushing the limits of thermodynamic efficiency. This progress reduces fuel consumption and emissions even further, while improving plant longevity and reducing maintenance intervals.
Lastly, policy frameworks and financial incentives promoting energy efficiency and emissions reduction are accelerating the adoption of steam-based CHP systems worldwide. Governments and utilities recognize the value of cogeneration in achieving sustainability goals, energy security, and economic competitiveness, often supporting CHP projects through grants, tax credits, or favorable regulatory treatment.
In essence, steam in combined heat and power plants remains a cornerstone of efficient energy utilization, evolving continuously through technological innovation and integration with emerging energy paradigms. Its enduring adaptability and performance advantages position steam cogeneration as a key contributor to a cleaner, more resilient, and economically viable energy future.
Recovering Waste Heat with Steam Systems
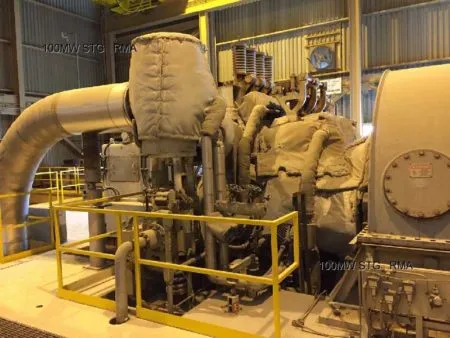
Recovering waste heat with steam systems is a highly effective strategy for improving energy efficiency and reducing operational costs in industrial processes and power generation. Waste heat—thermal energy lost to the environment from exhaust gases, cooling systems, or process equipment—represents a significant untapped energy resource. Steam systems capitalize on this by capturing the waste heat and converting it into useful steam that can drive turbines, provide process heat, or generate electricity.
In typical waste heat recovery steam systems, exhaust gases from furnaces, boilers, gas turbines, or other high-temperature sources pass through a heat recovery steam generator (HRSG). The HRSG absorbs thermal energy from these gases to convert feedwater into steam at desired pressure and temperature levels. This steam can then be utilized in multiple ways: to power steam turbines for electricity generation, to supply heat to industrial processes, or to provide district heating.
The efficiency benefits of waste heat recovery with steam systems are substantial. By converting what would otherwise be lost energy into productive output, facilities can reduce fuel consumption, lower greenhouse gas emissions, and enhance overall energy utilization. This approach often shortens the payback period for investments in steam system upgrades and contributes to sustainability goals.
Waste heat recovery steam systems are widely used in combined-cycle power plants, where gas turbine exhaust heat generates steam for additional power production. Beyond power generation, industries such as cement, steel, chemical manufacturing, and refineries use these systems to reclaim heat from processes like kiln exhaust, flue gases, or furnaces.
Design considerations for effective waste heat recovery steam systems include the temperature and flow rate of the waste gas, the steam pressure and temperature required, and integration with existing process equipment. HRSGs can be customized with multiple pressure levels and reheaters to optimize steam quality and energy extraction.
Challenges in waste heat recovery include managing fouling and corrosion within heat exchangers due to contaminants in exhaust gases, as well as maintaining steam purity to protect turbines and piping. Regular maintenance, advanced materials, and appropriate water treatment are critical to ensuring long-term system reliability.
Innovations in waste heat recovery include compact, modular HRSG designs that can be retrofitted to existing equipment, as well as advanced control systems that dynamically adjust steam generation to match fluctuating process demands. Integration with energy storage and smart grids further enhances the flexibility and economic viability of these steam-based recovery systems.
In summary, recovering waste heat with steam systems transforms a major source of energy loss into valuable power and heat, making it a cornerstone technology for industries aiming to boost efficiency, cut emissions, and improve sustainability. By harnessing waste heat, steam systems not only conserve fuel but also contribute to the economic and environmental performance of modern industrial and energy infrastructure.
Expanding on waste heat recovery with steam systems, the economic and environmental advantages make these technologies increasingly attractive in a variety of sectors. By capturing and repurposing energy that would otherwise dissipate into the atmosphere, companies can achieve significant cost savings on fuel and reduce their carbon footprint, aligning with global efforts to combat climate change and meet stringent emissions regulations.
One critical factor in maximizing the effectiveness of steam-based waste heat recovery is the careful integration of the system with existing plant operations. Engineers must evaluate the temperature profiles of exhaust gases, the demand for steam or electricity within the facility, and potential impacts on equipment performance. For example, low-grade waste heat at relatively low temperatures may be better suited for direct heating or low-pressure steam applications rather than electricity generation, while high-temperature waste heat offers greater potential for driving steam turbines efficiently.
Advanced heat exchanger designs are essential in optimizing heat transfer from waste gases to water or steam. Technologies such as finned tubes, enhanced surface geometries, and corrosion-resistant alloys improve thermal efficiency and durability, helping to maintain performance over time despite exposure to potentially corrosive flue gases or particulates.
Another important aspect is the management of transient operating conditions, as industrial processes often experience fluctuating loads and variable waste heat availability. Modern control systems equipped with sensors and automation can dynamically adjust steam generation rates, turbine operation, and steam distribution to match real-time demands. This flexibility ensures that the recovered heat is utilized effectively without causing operational bottlenecks or energy wastage.
In addition to traditional fossil-fuel-based industries, waste heat recovery using steam systems is gaining traction in renewable energy contexts. For example, biomass power plants often incorporate waste heat recovery steam generators to maximize energy output, while geothermal plants use steam extracted from underground reservoirs for both electricity generation and district heating.
Emerging trends also include coupling waste heat recovery steam systems with carbon capture technologies. By integrating steam generation and utilization with processes that capture CO₂ emissions from flue gases, facilities can move towards near-zero emissions while maintaining high energy efficiency.
Furthermore, the modular nature of modern HRSGs and steam turbines facilitates retrofitting existing plants to incorporate waste heat recovery without extensive downtime or capital expenditure. This adaptability supports incremental improvements in older facilities, helping industries meet evolving regulatory and sustainability targets cost-effectively.
Overall, waste heat recovery with steam systems exemplifies a pragmatic, proven approach to energy conservation that delivers measurable benefits across diverse industrial landscapes. Its continued evolution through materials innovation, digitalization, and integration with clean energy technologies positions it as a vital component of sustainable energy strategies worldwide.
Steam Energy and Carbon Footprint Reduction
Steam energy plays a significant role in reducing the carbon footprint across many industries and power generation sectors by enabling more efficient use of fuel and integration with cleaner energy technologies. The fundamental advantage of steam systems lies in their ability to convert thermal energy into mechanical work and process heat with high efficiency, especially when combined with technologies like combined heat and power (CHP) and waste heat recovery. By maximizing the utilization of the energy content in fuels, steam systems minimize wasted energy, which directly translates into lower fuel consumption and reduced greenhouse gas emissions.
In power plants, steam turbines powered by fossil fuels such as coal, natural gas, or oil have traditionally been major sources of carbon dioxide emissions. However, improvements in steam cycle efficiency—through supercritical and ultra-supercritical steam conditions—allow plants to extract more energy per unit of fuel burned, thereby lowering the amount of CO₂ released for each megawatt-hour of electricity produced. Additionally, combined-cycle plants that pair gas turbines with steam turbines use the waste heat from gas turbines to generate steam, significantly boosting overall plant efficiency and reducing carbon intensity.
Beyond conventional fuels, steam systems also enable the integration of renewable and low-carbon energy sources. Biomass-fired boilers produce steam from organic materials, offering a carbon-neutral or even carbon-negative energy source when sustainably managed. Geothermal plants use naturally occurring steam or hot water to generate electricity with minimal emissions. Furthermore, emerging technologies such as hydrogen combustion in boilers can generate steam without direct CO₂ emissions, positioning steam systems as key enablers of future low-carbon energy infrastructure.
Steam’s role in combined heat and power plants further enhances carbon footprint reduction by capturing and utilizing thermal energy that would otherwise be wasted. By providing both electricity and useful heat from a single fuel input, CHP systems drastically improve fuel utilization efficiency and reduce total emissions compared to separate heat and power generation. This efficiency gain is particularly valuable in industrial sectors where process steam is essential, such as chemical manufacturing, food processing, and paper production.
Moreover, steam-driven waste heat recovery systems capture residual heat from industrial processes or power generation exhaust streams, converting it into additional useful energy and lowering the overall carbon footprint. The implementation of advanced materials and control technologies allows steam systems to operate at higher temperatures and pressures, increasing thermodynamic efficiency and further reducing emissions.
Despite these advantages, challenges remain in decarbonizing steam systems fully. Water and fuel availability, infrastructure constraints, and the need for materials capable of withstanding extreme conditions at high efficiency levels require ongoing innovation. Additionally, transitioning from fossil fuels to alternative, low-carbon fuels like green hydrogen demands modifications to boilers, turbines, and associated systems.
Overall, steam energy contributes substantially to carbon footprint reduction by enabling efficient fuel use, facilitating integration with renewable and low-carbon fuels, and supporting cogeneration and waste heat recovery. As technology advances and sustainability pressures mount, steam systems are poised to remain essential components of cleaner, more efficient energy landscapes worldwide.
Building further on steam energy’s contribution to carbon footprint reduction, it is important to consider the broader system-level impacts and emerging technologies that enhance sustainability. The versatility of steam allows it to act as a bridge between traditional energy systems and the evolving low-carbon future. For instance, retrofitting existing steam plants with modern control systems, advanced materials, and emissions-reducing technologies can extend their operational life while significantly lowering carbon emissions. This approach offers a cost-effective pathway for industries and utilities to meet increasingly stringent environmental regulations without complete infrastructure overhaul.
In addition to efficiency improvements, the shift toward alternative fuels in steam systems is gaining momentum. Green hydrogen, produced via electrolysis using renewable electricity, can be combusted in boilers to produce steam without CO₂ emissions, representing a transformative opportunity for decarbonizing heat-intensive industries. However, integrating hydrogen presents technical challenges such as flame stability, NOx emissions control, and material compatibility, necessitating research and development efforts to adapt steam generation equipment accordingly.
Carbon capture, utilization, and storage (CCUS) technologies are also being coupled with steam-based power plants to further reduce carbon footprints. By capturing CO₂ emissions from flue gases, particularly in coal- or gas-fired steam plants, CCUS enables continued use of fossil fuels with minimal climate impact. The synergy between steam generation and CCUS can be enhanced through process optimization, such as using steam for solvent regeneration in carbon capture systems, improving overall energy efficiency.
Another promising avenue is the integration of steam energy systems with renewable energy sources in hybrid configurations. Solar thermal plants, for example, generate steam directly from concentrated sunlight, reducing reliance on fossil fuels. Excess renewable electricity can also be used to produce hydrogen or to power electric boilers, providing flexible steam generation that complements variable renewable generation and supports grid stability.
Water management remains a critical aspect of steam energy’s environmental performance. Efficient water use, recycling of condensate, and treatment to prevent scaling and corrosion are essential to maintaining system reliability and minimizing environmental impact. Innovations in water-saving technologies and alternative working fluids for steam turbines are being explored to address water scarcity concerns, especially in arid regions.
Furthermore, digitalization and smart monitoring enable precise control of steam systems, optimizing combustion, heat transfer, and turbine operation to minimize fuel use and emissions. Predictive maintenance powered by AI helps avoid unexpected downtime and maintain peak performance, thereby supporting sustainable operation.
Overall, steam energy’s role in carbon footprint reduction is multifaceted, involving technological innovation, fuel diversification, integration with renewable energy, and improved operational practices. As the global energy landscape continues to evolve toward sustainability, steam systems will remain vital for their ability to efficiently deliver both power and heat while adapting to cleaner fuels and carbon management strategies. Their continued advancement and integration are key to achieving ambitious climate targets and building resilient, low-carbon energy infrastructure worldwide.
Looking ahead, the future of steam energy in carbon footprint reduction will be shaped by the convergence of several technological, economic, and policy trends. As governments and industries worldwide accelerate decarbonization efforts, steam systems will increasingly serve as platforms for innovation that combine efficiency, flexibility, and sustainability. One significant trend is the rise of sector coupling, where steam generation is integrated with electrical grids, renewable energy sources, and energy storage solutions to create interconnected, multi-vector energy systems. In this context, steam turbines and boilers can operate in coordination with variable renewable generation—like solar and wind—adjusting steam production to balance grid demands and store excess energy thermally or chemically.
Hydrogen’s role as a clean fuel in steam systems will likely expand as production scales up and costs decline. This transition will not only reduce emissions but also enable seasonal energy storage, as hydrogen can be produced during periods of excess renewable electricity and then converted back to steam energy on demand. Additionally, advances in materials science, such as the development of superalloys and ceramic coatings, will allow steam turbines and boilers to withstand higher temperatures and pressures, pushing thermodynamic efficiency to new heights and lowering emissions further.
In industrial sectors, the electrification of certain processes and the shift to green hydrogen will complement steam systems rather than replace them entirely, as many processes still require high-quality steam for heating, sterilization, and chemical reactions. This coexistence underscores the importance of flexible steam generation technologies that can adapt to a variety of fuel sources and operational modes while maintaining efficiency and environmental performance.
Policy frameworks and financial incentives will continue to play a pivotal role in accelerating the adoption of low-carbon steam technologies. Carbon pricing, emissions standards, and subsidies for clean energy investments incentivize retrofits and new projects that reduce carbon intensity. Moreover, international cooperation and knowledge sharing foster the dissemination of best practices and technological advances across regions and industries.
Finally, public awareness and corporate sustainability commitments are driving demand for cleaner energy solutions, pushing companies to invest in steam systems that align with environmental goals. Transparency in emissions reporting and life cycle assessments highlight the benefits of efficient steam cogeneration and waste heat recovery, reinforcing their role in sustainable energy portfolios.
In summary, steam energy is poised to remain a cornerstone of global efforts to reduce carbon footprints, leveraging its adaptability, efficiency, and compatibility with emerging clean technologies. Through continuous innovation and integration within broader energy systems, steam-based solutions will help build a resilient, low-carbon future that balances economic growth with environmental stewardship.
Can Steam Engines Run on Biomass?
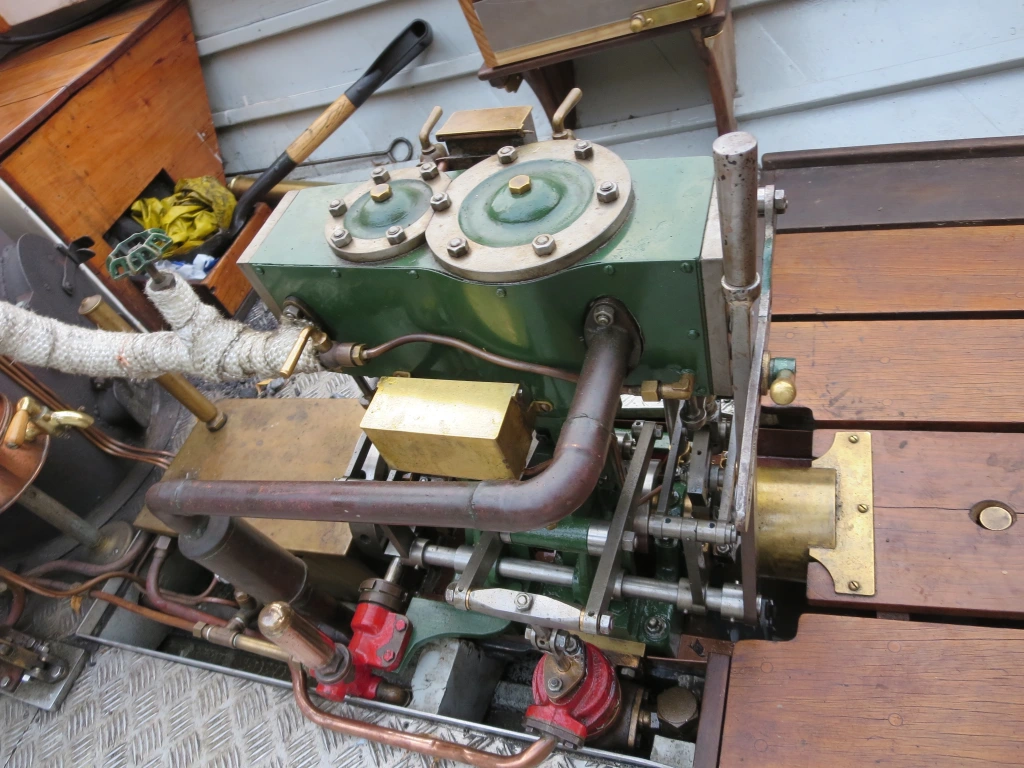
Yes, steam engines can absolutely run on biomass. In fact, using biomass as a fuel for steam generation is a well-established method for producing renewable energy. Biomass refers to organic materials—such as wood chips, agricultural residues, sawdust, sugarcane bagasse, and even some forms of waste—that can be combusted or gasified to produce heat. This heat then boils water to generate steam, which can drive steam engines or turbines for mechanical work or electricity production.
The process begins with the combustion of biomass in a boiler. The heat from this combustion converts water into high-pressure steam. This steam is then directed to a steam engine or more commonly, in modern setups, a steam turbine. The expanding steam drives the engine’s pistons or the turbine’s blades, converting thermal energy into mechanical energy, which can be used directly or turned into electricity via a generator.
Running steam engines on biomass offers a number of environmental benefits. Biomass is considered a renewable resource because the carbon dioxide released during combustion is offset by the CO₂ absorbed by the plants during their growth. This gives it a smaller net carbon footprint compared to fossil fuels, provided the biomass is sourced sustainably. Additionally, biomass fuels can often be obtained locally, reducing transportation emissions and supporting rural economies.
Technologically, older piston-type steam engines and boilers can be adapted to use solid biomass, although modern biomass power plants typically employ more efficient and scalable steam turbines. Some biomass systems are designed for direct combustion, while others use gasification to convert the biomass into a combustible gas, which is then burned to generate steam. This can offer cleaner combustion and better control over emissions.
However, there are challenges. Biomass has a lower energy density than fossil fuels, which means more fuel is needed to generate the same amount of energy. The handling and storage of biomass can be more complex due to moisture content, potential for decay, and variability in quality. Combustion of biomass can also produce more particulates and ash than natural gas, requiring effective emissions control systems and maintenance.
Despite these limitations, steam engines and turbines powered by biomass continue to be a viable and increasingly popular solution in the quest for sustainable energy, particularly in rural areas or developing regions where biomass is abundant and other fuels are less accessible. From small-scale systems providing local heat and power to large biomass power stations integrated into national grids, steam technology remains a flexible and valuable tool in the transition to cleaner energy sources.
The ability of steam engines to run on biomass not only supports renewable energy generation but also opens up opportunities for decentralized and community-scale power solutions. In regions with agricultural or forestry activities, the availability of biomass residues offers a cost-effective and sustainable fuel source that can be used to drive steam engines for both electricity and thermal energy production. This dual-purpose capability is especially advantageous in combined heat and power (CHP) configurations, where the waste heat from electricity generation is captured and used for heating buildings, greenhouses, or industrial processes, thereby increasing overall efficiency and reducing fuel requirements.
Biomass-powered steam systems also contribute to energy security by reducing dependence on imported fossil fuels. In countries striving to reduce their carbon emissions and promote self-sufficiency, local biomass resources offer a practical alternative. Moreover, by utilizing materials that would otherwise go to waste—such as sawmill scraps, rice husks, or corn stalks—these systems help manage agricultural waste and reduce methane emissions from organic decomposition, further improving their environmental footprint.
One of the notable advantages of steam engines, particularly in rural or off-grid contexts, is their simplicity and robustness. While not as efficient as modern steam turbines, piston-type steam engines can tolerate fuel variability, are easier to maintain with basic tools, and can operate under conditions that might challenge more sensitive technologies. This makes them particularly well-suited for small communities, farms, or industries that generate their own biomass waste and want to convert it into usable energy on-site.
As technology progresses, biomass combustion systems are becoming cleaner and more efficient. Advanced combustion techniques, such as fluidized bed boilers, enable more complete burning of biomass with lower emissions. These systems can handle a variety of biomass types and moisture levels, making them highly adaptable. Innovations in feedstock preparation, such as drying and pelletizing, improve the consistency and energy content of biomass fuels, enhancing combustion performance and reducing wear and tear on steam engines.
In terms of environmental impact, the carbon neutrality of biomass hinges on sustainable sourcing and land management practices. If biomass is harvested faster than it regenerates, or if land-use changes negate the carbon savings, the benefits can be diminished. Therefore, responsible supply chain practices and verification systems are essential to ensure the true sustainability of biomass fuel use in steam energy systems.
Economically, the viability of running steam engines on biomass depends on the availability and cost of biomass compared to other fuels, the scale of the operation, and potential incentives such as renewable energy credits or carbon offsets. In many cases, the use of waste biomass provides not only a free or low-cost fuel source but also solves a waste disposal problem, creating a win-win situation.
In conclusion, steam engines fueled by biomass represent a practical and sustainable method for generating energy in a variety of settings. Whether for industrial use, rural electrification, or as part of a broader renewable energy strategy, they demonstrate how traditional steam technology can be effectively adapted to meet modern energy and environmental challenges. With continued improvements in efficiency, emissions control, and fuel handling, biomass-powered steam systems are likely to play an increasingly important role in the global transition to clean and resilient energy systems.
As global interest in sustainable and decentralized energy continues to grow, the potential for biomass-powered steam systems is expanding beyond traditional applications. In developing regions, where access to reliable electricity is limited, small-scale steam engines fueled by locally available biomass can provide a transformative solution for lighting, irrigation, grain milling, and refrigeration. These systems not only foster economic development but also reduce the reliance on diesel generators, which are costly to operate and contribute significantly to greenhouse gas emissions and air pollution.
Moreover, steam systems offer thermal energy that is often overlooked but critically important. In many industrial and agricultural processes, such as drying crops, pasteurizing milk, or processing food, steam is a preferred and efficient medium for delivering controlled heat. Biomass-fueled steam boilers can fulfill this role with minimal infrastructure, especially in settings where biomass residues are a byproduct of the main activity, thereby creating an efficient closed-loop system.
The long-term value of biomass steam energy also lies in its compatibility with circular economy principles. Rather than viewing biomass residues as waste, this approach sees them as energy resources that can be continuously cycled through the system. When managed sustainably, biomass production and consumption form a regenerative loop, supporting soil health, reducing landfill use, and minimizing environmental degradation. Some systems even incorporate biochar production—a form of charcoal created during biomass combustion—which can be returned to the soil to enhance fertility and sequester carbon.
In industrialized nations, the interest in co-firing—where biomass is burned alongside coal in existing steam power plants—has grown as a transitional strategy for reducing carbon emissions without requiring the immediate shutdown of legacy infrastructure. While co-firing requires careful handling of fuel mixtures and modifications to boilers, it offers a practical and relatively low-cost path to cleaner energy in the short term. Over time, these facilities can transition to 100% biomass operation or be converted to support other renewable technologies.
Government policies and incentives continue to shape the trajectory of biomass steam technologies. Carbon taxes, renewable portfolio standards, and feed-in tariffs make biomass-based electricity generation more competitive, especially in markets where emissions reduction is a priority. As international agreements push for greater sustainability and energy equity, funding and research into small-scale and rural biomass steam systems are likely to increase, further driving innovation and adoption.
Education and training also play an important role in the successful deployment of these systems. Operators must be familiar with biomass combustion principles, boiler safety, maintenance routines, and emission control practices. Investments in workforce development ensure not only the reliability of the technology but also the creation of local jobs and technical expertise.
In future energy scenarios that emphasize resilience, diversity, and environmental responsibility, steam engines powered by biomass occupy a unique and valuable niche. They blend established thermodynamic principles with renewable fuel flexibility, allowing them to serve as both transitional and long-term components of sustainable energy systems. Their ability to scale—from powering a single farm to supporting community-wide microgrids—makes them versatile tools in the effort to decarbonize energy production and extend its benefits to all corners of the globe.
Water Use and Environmental Impact of Steam Systems
Steam systems, while vital to industrial processes and power generation, have significant interactions with the environment—most notably through their use of water and their broader ecological footprint. Water is the fundamental working fluid in steam systems, used to generate steam, transfer heat, and maintain efficient thermal cycles. As such, the quantity, quality, and management of water in these systems directly affect both operational performance and environmental sustainability.
The most obvious aspect of water use in steam systems is consumption for steam generation and cooling. In a typical power plant or industrial setting, large volumes of water are needed to feed boilers and absorb waste heat in condensers. Once water is converted into steam, it either performs mechanical work or is used for heating before being condensed back into liquid and cycled through the system again. This closed-loop setup reduces water loss, but in reality, some water is always lost to blowdown (to remove impurities), leaks, or evaporation in cooling towers. As a result, fresh water must be continually added to maintain system balance, especially in high-pressure and high-purity applications where the quality of water must be strictly controlled.
This dependency on water poses environmental challenges, particularly in regions facing water scarcity. Steam systems that withdraw water from rivers, lakes, or aquifers can strain local resources, disrupt aquatic ecosystems, and alter thermal balances in nearby bodies of water. Thermal pollution is a specific concern—when warmer water used in condensers is discharged back into natural waterways, it can lower oxygen levels and impact aquatic life. To mitigate these effects, modern facilities implement cooling towers, dry cooling systems, or closed-cycle cooling to minimize water withdrawal and reduce thermal discharge.
Chemical treatment of water in steam systems also carries environmental implications. To prevent scaling, corrosion, and microbial growth in boilers and condensers, a variety of chemical additives are used—such as phosphates, amines, or biocides. While necessary for maintaining equipment efficiency and longevity, these substances can be harmful if not properly managed and can contaminate soil or water if discharged without adequate treatment. Environmental regulations strictly govern the handling and disposal of boiler blowdown and cooling water to prevent pollution.
From a broader perspective, the environmental impact of steam systems extends beyond water use. The source of energy used to generate steam—whether fossil fuels, biomass, or electricity—determines the system’s carbon footprint. Fossil-fueled steam systems emit greenhouse gases and other pollutants unless paired with mitigation technologies like carbon capture or emissions controls. Biomass-fueled systems can reduce net emissions, but they still produce particulates and require careful sourcing to ensure sustainability.
Efforts to reduce the environmental impact of steam systems focus on improving efficiency, conserving water, and switching to cleaner energy sources. High-efficiency boilers and turbines extract more energy per unit of fuel, reducing both emissions and water use per megawatt of output. Advanced water treatment and recovery technologies, such as reverse osmosis, condensate polishing, and zero-liquid-discharge systems, help minimize freshwater intake and environmental contamination. Dry cooling systems, while more energy-intensive, eliminate the need for water-based cooling altogether—an important solution in arid environments.
In summary, steam systems are inherently water-intensive, and their environmental impact hinges on how water is sourced, used, and discharged, as well as how the steam itself is generated. Through thoughtful design, responsible operation, and continuous innovation, it is possible to significantly reduce their ecological footprint. As climate change and water scarcity grow in global importance, sustainable water use and environmental stewardship will remain central to the evolution of steam energy systems.
Improving the sustainability of steam systems involves not only technological upgrades but also strategic planning and system-level integration. One of the key opportunities lies in enhancing water efficiency across the entire steam cycle. This can be achieved by recovering and reusing condensate wherever possible, since it is already treated and pressurized, reducing the need for additional chemical treatment and energy input. Efficient condensate return systems can significantly cut down on both water usage and boiler fuel consumption, especially in large industrial facilities where steam is distributed across long distances.
Another important aspect of reducing water-related environmental impacts is leak detection and repair. Steam losses through leaking valves, pipes, or traps not only waste energy but also contribute to unnecessary water and chemical losses. Regular maintenance programs that include ultrasonic leak detection, steam trap testing, and thermal imaging can identify inefficiencies early and ensure that the steam system operates close to its designed performance. These operational improvements are relatively low-cost and can yield substantial environmental benefits over time.
At the design level, system optimization can lead to more sustainable water and energy use. For example, integrating steam systems with waste heat recovery units can extract additional thermal energy from flue gases or other high-temperature streams, reducing the steam load on boilers. This lowers fuel use and, consequently, the amount of water required for steam generation and cooling. In co-generation systems, the steam produced is used both for power generation and for process heating, dramatically increasing overall energy efficiency and reducing environmental impact per unit of output.
Material selection also plays a role in minimizing environmental risks. Corrosion-resistant materials in boiler and piping systems reduce the need for aggressive chemical treatments and extend the lifespan of components, reducing waste and environmental hazards. New advances in membrane filtration and ion exchange technologies allow for higher purity water recycling, making it feasible to reuse industrial wastewater or treat non-traditional water sources, such as saline or brackish water, for steam generation.
Policy and regulatory frameworks increasingly encourage or require facilities to manage water and emissions more responsibly. Compliance with environmental standards often necessitates investment in monitoring systems, reporting mechanisms, and performance audits. Many industries voluntarily adopt sustainability targets, and steam system upgrades are a practical pathway to meet those goals. Companies that proactively manage their steam and water use can also benefit reputationally, demonstrating their commitment to environmental stewardship to customers, investors, and regulators.
Climate change introduces further complexity. As water availability becomes more variable and extreme weather events disrupt supply chains and infrastructure, steam systems will need to be more resilient. Facilities are beginning to incorporate climate risk assessments into their design and operations, ensuring that water-intensive systems can function reliably even under stress. Innovations such as hybrid cooling systems that switch between wet and dry cooling based on ambient conditions can offer the flexibility needed to adapt to future water constraints.
Ultimately, while steam systems have traditionally been seen as water-intensive and environmentally challenging, the technologies and practices available today offer clear pathways toward significant impact reduction. The key lies in integrating efficiency measures, responsible resource management, and innovative design principles into a cohesive strategy. With growing awareness of environmental issues and stronger incentives to act, steam system sustainability is not only achievable—it’s becoming an essential component of modern energy and industrial systems.
Looking forward, the evolution of steam systems in terms of water use and environmental impact will be closely linked to advances in digitalization and smart technologies. The incorporation of sensors, real-time monitoring, and data analytics allows operators to gain unprecedented insight into system performance, water consumption patterns, and emissions. These technologies enable predictive maintenance, where potential issues such as leaks, corrosion, or scaling can be identified before they cause significant damage or inefficiencies. As a result, plants can optimize water and energy use continuously, responding dynamically to changing operational conditions while minimizing waste and environmental harm.
Artificial intelligence and machine learning algorithms are being developed to analyze vast datasets from steam systems to find hidden inefficiencies and suggest improvements. For instance, optimizing boiler firing rates, adjusting steam pressure, or balancing condensate return can all be automated to maximize resource efficiency. These intelligent control systems not only reduce operational costs but also decrease water withdrawal and pollutant discharges, helping facilities meet stricter environmental regulations and corporate sustainability targets.
Another frontier is the integration of steam systems with renewable energy sources and emerging clean technologies. For example, solar thermal plants can generate steam directly using concentrated sunlight, significantly reducing reliance on fossil fuels and water consumption associated with combustion processes. In hybrid systems, surplus renewable electricity can power electric boilers or heat pumps that produce steam without combustion, further lowering emissions and water use related to cooling. This diversification of steam generation sources makes the overall system more resilient and environmentally friendly.
Water treatment innovations continue to expand the possibilities for using alternative water sources in steam systems. Technologies such as membrane bioreactors, advanced oxidation processes, and electrochemical treatments can purify wastewater or non-traditional water sources to the high quality needed for boiler feedwater. This reduces dependence on freshwater supplies and opens opportunities for steam systems to operate sustainably even in water-stressed regions.
Policy developments will increasingly encourage or mandate low-impact water use and emissions from steam systems. Water footprint accounting, life cycle assessments, and stricter discharge limits are becoming standard requirements in many industries. These regulations push facilities to adopt best practices in water conservation, treatment, and emissions control. Incentives such as tax breaks, grants, or carbon credits for implementing green steam technologies further stimulate innovation and investment.
Public and stakeholder awareness of water scarcity and pollution issues is also driving demand for transparency and sustainable operation. Companies that demonstrate responsible management of steam systems can gain competitive advantages, enhance brand value, and meet growing consumer expectations for environmental responsibility.
In essence, the future of steam systems lies in a holistic approach that combines technological innovation, operational excellence, resource stewardship, and policy alignment. By harnessing smart technologies, embracing renewable integration, optimizing water use, and adhering to stringent environmental standards, steam systems can continue to provide reliable, efficient energy while minimizing their environmental footprint. This balance will be critical to meeting global energy needs sustainably in an era of climate change and increasing resource constraints.
Comparing Steam with Diesel and Electric Power
Comparing steam power with diesel and electric power involves evaluating multiple factors including efficiency, environmental impact, fuel availability, operational flexibility, and cost, as each technology has unique strengths and challenges that make them suitable for different applications and contexts.
Steam power, traditionally fueled by coal, biomass, or other combustibles, operates by generating high-pressure steam to drive turbines or engines, converting thermal energy into mechanical or electrical energy. It is highly effective in large-scale, continuous power generation such as in thermal power plants or industrial processes requiring both heat and power (combined heat and power systems). Steam turbines can achieve high efficiencies, especially when integrated with modern combined cycle systems, and they excel in handling large energy loads and providing stable base-load power. Additionally, steam systems can use a wide variety of fuels, including renewable biomass, which can reduce reliance on fossil fuels and lower carbon emissions when managed sustainably.
Diesel power relies on internal combustion engines that burn diesel fuel to produce mechanical energy, commonly converted to electricity via generators. Diesel engines are highly flexible, with rapid start-up times and the ability to operate efficiently at variable loads. They are widely used in transportation, backup power systems, and remote or off-grid locations where grid electricity is unavailable or unreliable. Diesel engines tend to have high energy density fuels, enabling long-range mobility and compact power generation. However, diesel combustion produces significant greenhouse gases, nitrogen oxides, and particulate matter, contributing to air pollution and climate change. The cost and supply volatility of diesel fuel, along with maintenance requirements and noise, also impact their desirability.
Electric power, when discussed here, generally refers to power delivered via electrical grids or generated by electric motors powered by batteries or other energy sources. Electric power itself is an energy carrier rather than a generation method, but electric motors are highly efficient, quiet, and low-maintenance compared to combustion engines. The environmental impact of electric power depends on how the electricity is produced. When sourced from renewables such as solar, wind, or hydropower, it offers a clean, sustainable energy option. Conversely, electricity generated from coal or natural gas can carry significant environmental costs. The rise of battery technology and grid modernization enhances electric power’s viability in transportation, industrial applications, and residential use, enabling decarbonization and integration with smart grids.
In terms of efficiency, modern combined cycle steam plants can reach efficiencies upwards of 60%, surpassing traditional diesel generators, which typically operate around 30–40% efficiency. Electric motors often exceed 90% efficiency in converting electrical energy to mechanical energy. However, losses in electricity generation, transmission, and storage must be accounted for when comparing the overall system efficiency.
Environmental impacts vary widely. Steam power’s emissions depend on the fuel source—coal-fired steam plants emit high levels of CO₂ and pollutants unless equipped with mitigation technologies, while biomass steam plants offer lower net emissions but face challenges related to sustainable sourcing and particulate emissions. Diesel engines emit considerable air pollutants and greenhouse gases. Electric power’s environmental footprint depends on generation methods; renewable electricity greatly reduces emissions, while fossil fuel-based electricity can be as polluting as diesel or coal steam systems.
Operational flexibility is another differentiator. Diesel engines provide quick response and are suited for intermittent or backup power, while steam plants excel at steady, continuous operation but require longer start-up and ramp times. Electric power’s flexibility depends on generation sources and grid management; with increasing renewable penetration, grid stability and storage solutions become critical.
Fuel availability and infrastructure also shape the comparison. Diesel fuel is widely available globally but subject to market fluctuations and geopolitical risks. Steam power requires water and fuel inputs, which may be abundant in industrial or centralized settings but less so in arid or remote areas. Electric power infrastructure is expanding rapidly, but grid access and reliability remain challenges in many regions.
Cost factors include capital investment, fuel costs, maintenance, and lifespan. Steam plants typically involve high upfront costs and complex infrastructure but benefit from lower fuel costs when using biomass or waste fuels. Diesel generators have lower capital costs and are easier to deploy quickly but incur higher operational fuel and maintenance expenses. Electric power’s cost depends heavily on generation sources, with renewable electricity costs falling rapidly but requiring investment in storage and grid upgrades.
In summary, steam power, diesel engines, and electric power each have roles shaped by their technical characteristics and contextual factors. Steam systems are ideal for large-scale, continuous, or combined heat and power applications, especially where diverse fuel options are beneficial. Diesel engines suit flexible, mobile, or emergency power needs but carry higher emissions and fuel costs. Electric power, especially when sourced renewably, offers high efficiency and environmental benefits, with growing importance in a decarbonizing energy landscape. The choice among them depends on specific application requirements, resource availability, environmental priorities, and economic considerations.
When looking deeper into the practical implications of choosing between steam, diesel, and electric power, it becomes clear that the context of use heavily influences which technology is most appropriate. In industrial settings, steam power remains a backbone due to its ability to provide both electricity and process heat efficiently. Many industries, such as chemical manufacturing, pulp and paper, and refining, rely on steam systems because they can be integrated with boilers and turbines to produce cogeneration—simultaneous heat and power—thus maximizing fuel utilization and reducing waste. This dual output is something diesel generators and electric motors cannot directly match without separate systems.
Conversely, diesel power’s strength lies in its portability and reliability under variable conditions. Diesel generators are indispensable for remote locations, construction sites, military operations, and emergency backup power, where immediate power supply and ease of transport are critical. Their capability to ramp up quickly without warm-up periods and their relatively compact size make them unmatched in these scenarios. However, as environmental regulations tighten, the use of diesel generators is increasingly scrutinized, especially in urban areas where air quality concerns demand cleaner alternatives.
Electric power, on the other hand, represents the future trajectory of many energy systems due to its flexibility and the expanding availability of renewable generation. Electrification of transportation and industry is rapidly gaining momentum as battery technologies improve and renewable electricity becomes cheaper and more accessible. Electric motors offer nearly instantaneous torque, quiet operation, and minimal maintenance, making them ideal for a wide range of applications from passenger vehicles to industrial machinery. However, their dependency on grid stability and energy storage technologies introduces complexity and potential limitations, particularly in areas with underdeveloped infrastructure or high variability in renewable generation.
Cost considerations also reveal trade-offs. While steam plants require significant capital investment and are best suited for large, centralized facilities, their operational costs can be quite competitive when utilizing low-cost fuels such as biomass or waste heat. Diesel engines have lower upfront costs but often incur higher lifetime expenses due to fuel consumption and maintenance. Electric systems’ cost structure is shifting dramatically; while initial investments in batteries and grid upgrades are substantial, the declining cost of renewable electricity and the absence of fuel expenses make them economically attractive over time, especially when environmental costs are internalized.
Sustainability trends are further tilting the balance in favor of electric and biomass-fueled steam systems. The global push toward decarbonization, driven by climate change goals, is encouraging industries and utilities to move away from diesel and coal-based steam power toward cleaner alternatives. Biomass steam plants, when sourced sustainably, and renewable-powered electric systems contribute significantly to reducing carbon footprints. Diesel, with its fossil fuel dependency and pollutant emissions, faces increasing regulatory and social pressure, prompting the development of cleaner fuels and hybrid solutions.
In addition to technical and economic factors, safety and environmental compliance shape technology selection. Steam systems require stringent water treatment and pressure vessel maintenance to avoid hazards, while diesel engines present risks related to fuel storage and emissions. Electric systems generally offer safer, cleaner operation but depend on robust electrical infrastructure and cybersecurity measures to prevent outages or attacks.
In conclusion, while steam power continues to play a crucial role in specific industrial and large-scale applications due to its reliability, fuel flexibility, and cogeneration capabilities, diesel power remains vital for flexible, mobile, and emergency uses despite environmental drawbacks. Electric power is rapidly gaining ground as the preferred energy form for many sectors due to efficiency, environmental benefits, and technological advancements. The interplay of efficiency, emissions, cost, operational flexibility, and infrastructure considerations ultimately determines the optimal choice among these energy options for any given situation. As technologies evolve and environmental imperatives intensify, hybrid and integrated approaches combining the strengths of steam, diesel, and electric systems are likely to become increasingly common, leveraging their complementary advantages to build resilient, efficient, and sustainable energy solutions.
Looking ahead, the convergence of steam, diesel, and electric power technologies is shaping innovative hybrid energy systems designed to optimize performance, reduce emissions, and enhance reliability. For example, some modern power plants combine steam turbines with gas or diesel engines in combined cycle configurations, where the waste heat from combustion engines is recovered to generate steam, which then drives a turbine to produce additional electricity. This integration boosts overall efficiency far beyond what any single system can achieve alone and reduces fuel consumption and emissions. Similarly, hybrid microgrids in remote or off-grid locations may combine diesel generators with renewable energy sources and battery storage, using steam systems for thermal loads while electric power manages dynamic electrical demand. These hybrid setups balance fuel costs, environmental impact, and operational flexibility to suit specific local needs.
Electrification and digital control systems are also enabling more precise and adaptive operation of steam and diesel units, improving efficiency and reducing environmental impact. Smart control allows plants to optimize when and how each technology is used, responding to fuel price fluctuations, grid demands, or emission limits. For instance, during periods of low electricity demand or high renewable generation, steam plants may reduce output or shift operation to provide heat only, while diesel engines or batteries handle peak power needs. Conversely, when renewables are scarce, diesel or steam systems ramp up to maintain reliability. This orchestration requires sophisticated forecasting, sensor networks, and automation, increasingly driven by artificial intelligence.
From an environmental perspective, continued innovation focuses on minimizing pollutants and greenhouse gases from all three technologies. Advanced emission control systems—such as selective catalytic reduction for diesel engines or carbon capture and storage for steam plants—are becoming more common. Research into alternative fuels is also progressing rapidly: biofuels, synthetic fuels, and hydrogen can substitute traditional diesel or coal feedstocks, dramatically cutting carbon footprints. For electric power, ongoing expansion of clean generation capacity coupled with grid modernization and energy storage helps ensure sustainable, reliable supply.
Regulatory frameworks worldwide are tightening, imposing stricter emissions standards, water use restrictions, and efficiency requirements that influence technology adoption and development. Governments incentivize cleaner technologies through subsidies, tax credits, and research funding, accelerating the transition away from fossil-fuel-dependent systems. This evolving landscape pushes industries and utilities to invest in retrofits, new builds, and operational improvements that reduce environmental impact while maintaining economic viability.
Social and economic factors also play a role. Public demand for sustainability, corporate responsibility commitments, and investor pressures increasingly prioritize low-carbon, low-impact energy solutions. At the same time, energy equity considerations highlight the importance of flexible, reliable power access—particularly in underserved regions—where diesel generators still provide essential services but may gradually be replaced or supplemented by more sustainable steam and electric hybrid systems.
In essence, the future energy ecosystem will be characterized by a mosaic of complementary technologies rather than a single dominant solution. Steam, diesel, and electric power each contribute unique advantages, and their intelligent integration—leveraging strengths and mitigating weaknesses—offers the best path toward resilient, efficient, and environmentally responsible energy systems. As innovation, policy, and market dynamics evolve, these technologies will continue adapting, enabling a cleaner and more sustainable energy future tailored to diverse needs and contexts.
How Efficient Are Steam Engines Today?
Today’s steam engines, especially modern steam turbines used in power generation and industrial applications, have significantly improved efficiency compared to their early counterparts. While traditional reciprocating steam engines typically had efficiencies around 5-10%, modern steam turbines can reach thermal efficiencies of about 35% to 45% in conventional single-cycle plants. When integrated into combined cycle power plants—where waste heat from gas turbines is used to generate steam for additional power production—efficiencies can exceed 60%, making steam technology much more competitive.
These improvements come from advances in materials, design, and operation. High-pressure and supercritical steam conditions, improved blade aerodynamics, tighter manufacturing tolerances, and better thermal insulation all contribute to reducing energy losses. Additionally, sophisticated control systems optimize steam temperature and pressure, matching load demands while minimizing fuel consumption.
However, efficiency varies widely depending on the application. In smaller-scale or older installations, efficiencies may be lower due to less advanced technology or operational constraints. Industrial steam engines used for mechanical drive or heating often prioritize reliability and continuous operation over peak efficiency.
It’s also important to consider the overall system efficiency, which includes not just the steam engine itself but the entire steam cycle—fuel preparation, boiler performance, steam generation, turbine operation, condensate recovery, and auxiliary systems like feedwater pumps and cooling towers. Proper maintenance, water treatment, and operational practices are critical to maintaining high efficiency over time.
In summary, modern steam engines, particularly turbines, are far more efficient than their historical versions, with state-of-the-art plants reaching efficiencies that rival or exceed many other power generation methods. Continuous innovation and integration with combined cycle systems and cogeneration setups further enhance their performance and sustainability.
Beyond the impressive thermal efficiencies achieved in modern steam turbines, ongoing research and development continue to push the boundaries of what steam technology can deliver. Innovations in materials science, such as advanced alloys and ceramics capable of withstanding higher temperatures and pressures, allow steam cycles to operate under supercritical and ultra-supercritical conditions. These conditions enable steam to remain in a fluid state beyond the critical point, which reduces phase change losses and improves thermal efficiency significantly. Plants utilizing ultra-supercritical steam cycles can operate at efficiencies approaching 50%, which translates to lower fuel consumption and reduced greenhouse gas emissions per unit of electricity generated.
In addition to material advancements, improvements in turbine blade design and manufacturing techniques have resulted in more aerodynamic and precisely engineered blades that minimize turbulence and frictional losses. This enhances the conversion of steam’s thermal energy into mechanical energy, contributing to higher overall system efficiency. Coupled with computerized control systems that continuously monitor and adjust operating parameters in real time, steam turbines can maintain optimal performance even as load demands fluctuate.
Another crucial factor in achieving and sustaining high efficiency is the integration of the steam turbine within broader energy systems. Combined heat and power (CHP) plants utilize the heat generated by steam turbines not only for electricity but also for industrial processes or district heating, which can push overall fuel utilization efficiency to upwards of 80%. This approach maximizes the value derived from the fuel input and minimizes waste, making steam turbines highly competitive in applications where both power and heat are required.
Despite these advancements, challenges remain that can impact real-world efficiencies. Boiler performance is a key determinant—efficient combustion and heat transfer in the boiler are essential to producing high-quality steam. Any inefficiencies or losses here can cascade through the system, reducing turbine efficiency. Water purity and quality also play critical roles, as impurities can cause scaling and corrosion, degrading heat exchange surfaces and turbine components over time. This necessitates rigorous water treatment protocols and regular maintenance schedules to preserve efficiency.
Environmental regulations increasingly influence steam turbine operation by requiring the reduction of emissions such as nitrogen oxides, sulfur oxides, and particulate matter. Implementing emission control technologies can introduce additional energy penalties, slightly reducing net efficiency. However, these technologies are vital for compliance and environmental stewardship, and ongoing research aims to minimize their impact on overall performance.
In smaller-scale or older steam engines, efficiency remains limited by design constraints and operational factors. Reciprocating steam engines, once common in locomotives and early industrial machinery, typically operate at much lower efficiencies and are largely replaced by turbines in modern applications. However, niche uses and heritage applications still value these engines for their mechanical simplicity and robustness despite their lower efficiency.
Looking forward, the role of steam engines in the evolving energy landscape will likely focus on integration with renewable and hybrid systems, where their ability to provide stable, dispatchable power and process heat complements intermittent energy sources like wind and solar. Innovations such as advanced thermal energy storage can also work synergistically with steam cycles to smooth out supply variability and enhance overall system efficiency.
In essence, while the fundamental principles of steam engines have remained consistent for over a century, continuous improvements in technology, materials, and system integration have elevated their efficiency and utility to meet modern energy demands. With careful design, operation, and maintenance, steam turbines today stand as a highly efficient and versatile technology, capable of delivering reliable power and heat with reduced environmental impact.
In addition to technological advancements, the operational strategies employed in steam turbine plants significantly affect their efficiency. Operators increasingly rely on real-time data analytics and predictive maintenance to anticipate and prevent equipment degradation before it leads to performance losses. For example, sensors monitor vibration, temperature, and pressure throughout the steam cycle, enabling early detection of issues like blade erosion, bearing wear, or scaling in boilers. By addressing these proactively, plants can avoid unscheduled shutdowns and maintain peak efficiency over longer periods.
Another emerging trend is the use of flexible operation modes in steam turbines, especially in power grids with high penetration of renewables. Traditional steam plants were designed for steady, base-load operation, but modern grids require rapid ramping up and down to balance intermittent solar and wind power. Advances in turbine design, materials, and control systems now allow steam turbines to adapt more quickly without sacrificing efficiency or lifespan. This flexibility not only supports grid stability but also enhances the economic viability of steam-based generation in increasingly dynamic markets.
Environmental sustainability considerations also drive ongoing improvements. The integration of carbon capture and storage (CCS) technologies with steam power plants offers a pathway to drastically reduce CO₂ emissions, making steam turbines compatible with stringent climate targets. While CCS adds complexity and energy consumption, research aims to minimize these penalties through innovative capture methods and process integration. Additionally, the use of alternative fuels such as sustainably sourced biomass or hydrogen in steam boilers can lower carbon footprints and open new avenues for steam power in a low-carbon future.
Water management remains a critical component of steam system efficiency and environmental impact. Innovations in closed-loop cooling, dry cooling technologies, and water recycling reduce the large water withdrawals traditionally associated with steam power plants. This is particularly important in water-scarce regions where steam plants must balance energy production with local water resource sustainability. Implementing advanced water treatment and monitoring further protects turbine components and ensures consistent steam quality, sustaining high efficiency.
Furthermore, the push toward digital twins—virtual replicas of steam turbines and their associated systems—enables operators and engineers to simulate and optimize performance under various conditions without physical trials. These digital models can test new operational strategies, forecast maintenance needs, and identify efficiency improvements, accelerating innovation and reducing downtime.
While steam engines may sometimes be overshadowed by emerging technologies like advanced gas turbines and battery storage, their unique ability to generate large-scale, reliable power and heat keeps them integral to the global energy mix. Their adaptability to cleaner fuels, combined heat and power applications, and integration with smart grid technologies ensures they will continue to evolve and contribute meaningfully to energy systems.
Ultimately, the efficiency of steam engines today is not just a product of their mechanical design but a reflection of holistic system optimization, advanced materials, digital innovation, and sustainable operation practices. As these elements converge, steam power remains a vital, efficient, and adaptable technology poised to meet the complex demands of modern energy production and environmental responsibility.
Steam Power vs. Solar Energy
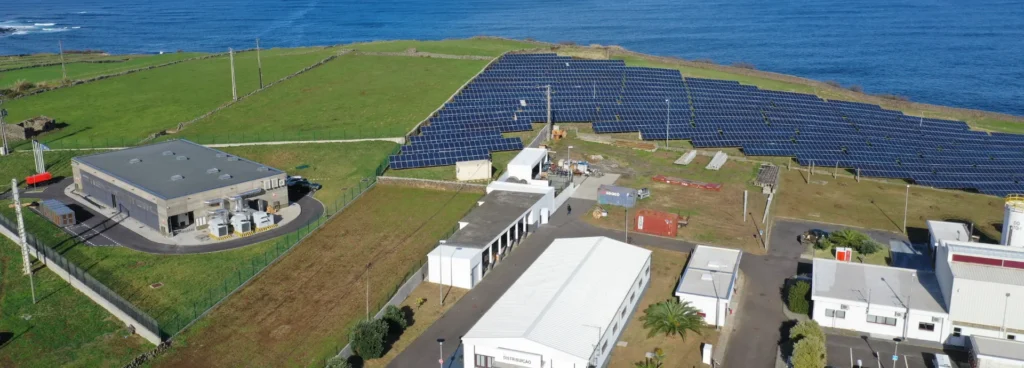
Steam power and solar energy represent two fundamentally different approaches to harnessing energy, each with its own strengths, limitations, and ideal applications, reflecting distinct technologies, environmental impacts, and operational characteristics.
Steam power, traditionally generated by burning fossil fuels or biomass to create high-pressure steam that drives turbines, has long been a backbone of industrial and utility-scale electricity generation. It excels at providing continuous, reliable power, especially in large-scale plants capable of delivering consistent base-load electricity. Modern steam power plants can achieve high efficiencies, particularly when configured as combined cycle or combined heat and power systems, and are valued for their ability to use a variety of fuels, including coal, natural gas, biomass, or waste materials. Their operation is well-understood, infrastructure is widely established, and they can integrate cogeneration—simultaneous production of electricity and heat—which enhances overall fuel utilization.
Solar energy, on the other hand, harnesses sunlight directly, primarily through photovoltaic (PV) panels that convert sunlight into electricity or concentrated solar power (CSP) systems that use mirrors to focus sunlight to produce heat, which then drives steam turbines. Solar energy is renewable, abundant, and produces no direct emissions during operation, making it a cornerstone of sustainable energy strategies. Its modular nature allows for deployment across scales, from small rooftop installations to large solar farms. However, solar energy is inherently intermittent, dependent on weather, time of day, and geographic location, which poses challenges for reliability and grid integration without adequate storage solutions.
From an efficiency perspective, steam power plants typically convert 30-45% of fuel energy into electricity in conventional setups, with combined cycle plants reaching over 60%. Solar PV panels currently convert around 15-22% of sunlight into electricity, though advanced technologies push this higher, while CSP systems can achieve thermal efficiencies of about 20-25%. The lower conversion efficiency of solar is offset by the free and infinite nature of sunlight, eliminating fuel costs and associated emissions.
Environmental impact sharply contrasts between the two. Steam power plants, especially those using fossil fuels, emit greenhouse gases, air pollutants, and generate thermal pollution and waste products. Biomass-fired steam plants offer a more sustainable alternative but still require careful management of feedstock. Solar energy generates no direct emissions, requires minimal water during operation, and has a significantly smaller environmental footprint, though manufacturing and disposal of panels raise concerns about material use and lifecycle impacts.
Operational flexibility and scalability also differ. Steam power plants are capital-intensive, designed for long operational lifetimes, and best suited for steady, continuous output, though newer designs enable greater flexibility. Solar installations can be rapidly deployed, scaled incrementally, and located closer to consumption points, reducing transmission losses. However, solar’s intermittency necessitates energy storage, grid upgrades, or backup generation, often supplied by steam or other dispatchable power sources.
Cost trends favor solar energy increasingly as technology matures and production scales, driving down capital and levelized costs of electricity (LCOE). Meanwhile, steam power infrastructure involves substantial upfront investment and ongoing fuel costs but benefits from mature supply chains and operational expertise.
In summary, steam power and solar energy serve complementary roles in the energy landscape. Steam power offers dependable, high-capacity generation with fuel flexibility and cogeneration benefits, essential for industrial processes and base-load electricity. Solar energy provides clean, renewable power with low operational costs and environmental impact, well-suited for distributed generation and decarbonization goals. The future energy system is likely to blend these technologies, leveraging steam power’s reliability and solar’s sustainability, supported by innovations in storage and grid management to achieve efficient, resilient, and low-carbon energy solutions.
Continuing from that, the integration of steam power and solar energy is already being explored in various innovative ways to harness the advantages of both technologies while mitigating their limitations. One promising approach is concentrated solar power (CSP) combined with thermal energy storage, where solar heat is used to generate steam that drives turbines similar to conventional steam plants. By storing excess heat in molten salts or other materials, CSP plants can produce electricity even when sunlight is not available, effectively bridging the gap between intermittent solar supply and the need for consistent power. This hybridization leverages steam turbines’ proven efficiency and flexibility, creating renewable baseload power without combustion emissions.
Additionally, some conventional steam power plants are incorporating solar thermal systems to preheat feedwater or supplement boiler heat input, reducing fossil fuel consumption and emissions. This incremental integration helps transition traditional power infrastructure towards cleaner energy without complete overhaul, making decarbonization more achievable and cost-effective in the near term.
However, challenges remain in fully realizing the synergy between steam and solar. CSP plants require significant land area, high initial investment, and are geographically limited to regions with abundant direct sunlight. Steam power plants, while flexible, still depend on fuel availability and may face regulatory pressures due to emissions and water use. Balancing these factors requires careful planning, policy support, and advances in technology such as more efficient thermal storage, hybrid fuels like hydrogen, and improved turbine designs optimized for variable input conditions.
From a grid perspective, combining solar generation with steam power enhances stability and reliability. Solar’s daytime peak generation can reduce steam plant operation during low-demand periods, conserving fuel and extending equipment life. Conversely, steam plants can ramp up during evening or cloudy periods when solar output drops, ensuring uninterrupted electricity supply. This dynamic coordination, enabled by smart grid technologies and demand-side management, is crucial as renewable penetration increases globally.
Economically, the decreasing costs of solar photovoltaics and battery storage challenge steam power’s competitiveness, especially in markets emphasizing rapid deployment and distributed generation. Yet, steam power’s scalability and ability to provide high-capacity, continuous power keep it indispensable for industrial processes, large urban centers, and regions where grid infrastructure is still developing. The future energy landscape will likely reflect a mosaic of technologies, where steam and solar coexist and complement each other within integrated, flexible energy systems designed for resilience, sustainability, and affordability.
In conclusion, steam power and solar energy, while fundamentally different in operation and characteristics, offer complementary strengths. Their combined use, whether through hybrid plants, cogeneration, or grid coordination, presents a powerful pathway to meet growing energy demands while reducing environmental impacts. Continued innovation, supportive policies, and strategic investments will determine how effectively these technologies integrate to build the clean, reliable, and efficient energy systems of tomorrow.
Looking further ahead, the evolving energy landscape suggests even deeper integration between steam power and solar energy, supported by advances in digital technologies, materials science, and system engineering. For instance, the development of advanced control systems powered by artificial intelligence and machine learning allows for real-time optimization of hybrid steam-solar plants, improving responsiveness to grid demands and maximizing overall efficiency. These smart systems can predict weather patterns, adjust turbine operation, and manage thermal storage dynamically, ensuring that energy production aligns closely with consumption patterns and market signals.
Material innovations are also poised to enhance the durability and performance of steam turbines operating with variable thermal inputs characteristic of solar-augmented systems. New high-temperature alloys, coatings, and cooling techniques extend component life and allow turbines to handle fluctuating steam qualities without efficiency losses or mechanical stress. This flexibility is crucial as solar energy introduces variability that traditional steam plants were not originally designed to accommodate.
Moreover, research into alternative working fluids beyond water and steam, such as supercritical CO₂ or organic Rankine cycles, promises to expand the applicability and efficiency of solar-thermal power generation. These fluids can operate at lower temperatures and pressures or with improved thermodynamic properties, enabling more compact, efficient, and potentially lower-cost turbine systems that integrate well with solar thermal inputs.
The push for decarbonization and energy security further drives the blending of steam power and solar energy with other renewable sources, storage technologies, and emerging fuels like green hydrogen. Green hydrogen, produced by electrolysis powered by excess solar electricity, can serve as a clean fuel for boilers or turbines, creating a closed-loop renewable system that leverages solar generation to produce and consume hydrogen on-site. This creates opportunities for seasonal energy storage and grid balancing, critical for handling renewable variability on a larger scale.
Economically, the continued decline in costs for solar panels, thermal storage, and digital infrastructure, combined with increasing carbon pricing and environmental regulations, strengthens the business case for hybrid steam-solar plants. Governments and industry stakeholders are increasingly recognizing the value of these integrated solutions in achieving climate goals, ensuring grid reliability, and supporting economic development, especially in sunny regions with established thermal power infrastructure.
Socially, these hybrid approaches contribute to energy access and resilience, providing stable, affordable power to communities while reducing pollution and carbon emissions. In developing regions, leveraging existing steam infrastructure with solar augmentation can accelerate electrification and industrialization with a lower environmental footprint. Community engagement and workforce development around these emerging technologies also create new opportunities for sustainable economic growth.
In summary, the future of energy lies in the smart, flexible integration of diverse technologies where steam power and solar energy play vital, interconnected roles. Through ongoing innovation, collaboration, and investment, these systems will evolve to meet the complex challenges of energy demand, climate change, and sustainable development, enabling a cleaner, more resilient, and equitable energy future for all.
Steam Distribution Networks in Large Facilities
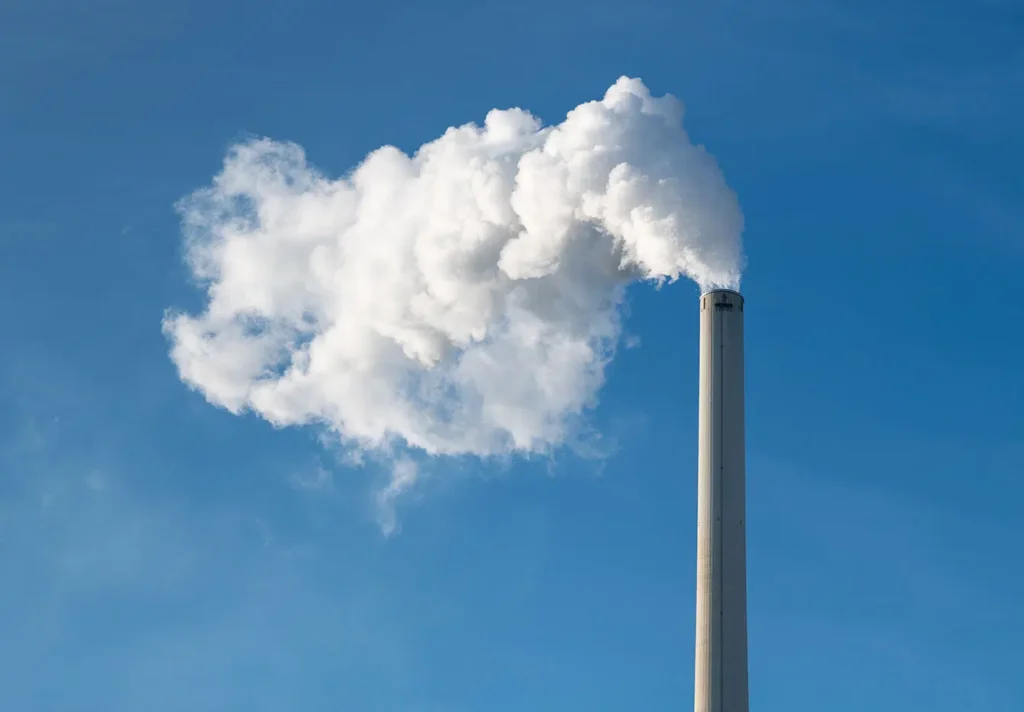
Steam distribution networks in large facilities are complex systems designed to deliver steam efficiently and safely from the central boiler or steam generator to various points of use across the plant or campus. These networks are critical for industries such as chemical processing, food and beverage manufacturing, pharmaceuticals, paper mills, and large institutional campuses, where steam serves multiple roles—from driving turbines and machinery to providing process heat, sterilization, and space heating.
A typical steam distribution network consists of insulated piping that transports steam at controlled pressures and temperatures through main headers and branch lines to end users. The system must be carefully designed to minimize heat losses, pressure drops, and water hammer risks while maintaining the quality and reliability of steam supply. High-quality insulation on pipes and fittings helps reduce thermal losses, improving overall system efficiency and reducing fuel consumption at the boiler.
Because steam expands and contracts with temperature changes and pressure fluctuations, expansion loops or joints are incorporated into the piping layout to absorb movement and prevent mechanical stresses that could cause leaks or failures. Proper drainage of condensate along the lines is essential to avoid water hammer—a dangerous surge caused by trapped condensate impacting high-velocity steam flow—which can damage pipes and equipment. This is typically managed through the strategic placement of steam traps and drip legs that automatically remove condensate without letting live steam escape.
Pressure regulation stations and control valves are distributed throughout the network to adjust steam pressure and flow rates based on demand, ensuring consistent steam quality and protecting downstream equipment from damage due to overpressure or fluctuations. Monitoring instrumentation such as pressure gauges, temperature sensors, and flow meters provide operators with real-time data to manage the network efficiently.
In large facilities, steam may be supplied at multiple pressure levels—high, medium, and low—to match the specific requirements of various processes, maximizing energy use. Pressure reduction stations safely drop steam from higher to lower pressures where needed, often recovering some energy in the process.
Regular maintenance is vital to keep the distribution network operating safely and efficiently. This includes inspecting insulation integrity, steam trap functionality, valve operation, and pipe condition to detect leaks or corrosion early. Leaks not only waste energy but can pose safety hazards and reduce steam quality.
Advanced steam distribution systems integrate with facility energy management systems, allowing for automated control, remote monitoring, and predictive maintenance. These smart networks optimize steam delivery, reduce energy waste, and support rapid response to changing process demands, enhancing overall facility productivity and sustainability.
In summary, steam distribution networks in large facilities are intricate, engineered systems essential for reliable and efficient steam delivery. Their design and operation require careful attention to thermodynamics, fluid mechanics, materials, and control technologies to support diverse industrial processes while minimizing energy losses and ensuring safety.
Steam distribution networks in large facilities are designed not only to transport steam but also to ensure the steam’s quality is preserved throughout the system. Maintaining dry, superheated steam where necessary is critical because the presence of moisture or condensate can reduce process efficiency, cause corrosion, and damage sensitive equipment. To achieve this, steam separators or dryers may be installed at strategic points to remove entrained water droplets, ensuring that only high-quality steam reaches the end-use equipment. Additionally, some systems employ steam reheaters to raise steam temperature after pressure drops, restoring thermal energy before it enters turbines or process units.
The layout and routing of steam pipelines must also consider the facility’s physical constraints and operational needs. Pipes are typically routed overhead or in dedicated trenches to minimize interference with other utilities and facilitate maintenance access. The network design takes into account potential future expansions, allowing for modular growth without major disruptions. Safety is a paramount concern, so the network incorporates pressure relief valves, emergency shutoff systems, and adherence to codes and standards governing steam systems, such as ASME guidelines.
Energy efficiency in steam distribution networks is a major focus area because even small losses can translate into significant fuel consumption and operating costs over time. Insulation materials and techniques continue to improve, with modern options like aerogel-based insulation offering higher thermal resistance with thinner layers, reducing both heat loss and pipe diameter requirements. Leak detection systems, sometimes utilizing ultrasonic or infrared technology, enable early identification of steam leaks that would otherwise go unnoticed, enabling prompt repairs and minimizing waste.
Condensate recovery systems are integral to maximizing efficiency within large steam distribution networks. After steam releases its energy at process points, the condensate is collected, often under vacuum conditions, and returned to the boiler feedwater system. Recycling condensate conserves water, reduces the need for chemical treatment, and improves boiler efficiency because the returning condensate is already hot, requiring less energy to convert back to steam. Proper condensate management involves pumps, flash tanks, and deaerators to remove dissolved gases that can cause corrosion.
In some facilities, district heating concepts are applied, where steam distribution networks extend beyond individual buildings or units, providing centralized heating or steam supply to multiple structures or campuses. This approach leverages economies of scale and centralized control, improving overall system efficiency and simplifying maintenance. However, it requires meticulous coordination and communication among facility operators to manage pressure drops, balancing loads, and scheduling maintenance without disrupting critical processes.
Technological advancements have enabled the digitization of steam distribution systems, transforming them from static infrastructure into intelligent, adaptive networks. Sensors embedded throughout the system feed data into centralized control platforms that utilize analytics and machine learning to optimize steam flow, predict failures, and schedule maintenance proactively. This reduces downtime, extends equipment lifespan, and improves safety by quickly identifying abnormal conditions like pressure spikes or steam quality degradation.
Environmental regulations and sustainability goals are also shaping the design and operation of steam distribution networks. Facilities are increasingly focused on reducing carbon footprints and water consumption, which drives efforts to minimize steam leaks, optimize pressure levels to avoid unnecessary throttling losses, and implement heat recovery from exhaust or vented steam. Renewable fuel sources such as biomass or biogas are being integrated into boiler systems, and some facilities explore electrification options or hybrid systems combining steam with solar thermal inputs, reflecting broader trends in decarbonization.
Ultimately, the complexity and importance of steam distribution networks in large facilities demand a multidisciplinary approach combining mechanical engineering, thermodynamics, control systems, and environmental science. Success depends on meticulous design, rigorous maintenance, continuous monitoring, and a commitment to operational excellence. When managed effectively, these networks not only support critical industrial processes reliably and efficiently but also contribute significantly to a facility’s energy conservation and sustainability objectives.
Beyond traditional steam distribution, emerging trends in large facilities emphasize integration with broader energy management and sustainability frameworks. One important development is the coupling of steam networks with renewable energy sources, such as solar thermal systems or biomass boilers, to reduce reliance on fossil fuels. Facilities increasingly implement hybrid systems where steam generation is partially or fully supplemented by renewable inputs, decreasing greenhouse gas emissions while maintaining the flexibility and reliability steam provides.
Another key innovation is the use of advanced control algorithms that dynamically adjust steam pressure, flow, and temperature based on real-time demand from various process units. By precisely matching steam supply to actual needs, facilities minimize energy waste caused by overproduction or excessive pressure drops. These controls can also orchestrate load shifting, temporarily storing thermal energy during low-demand periods and releasing it when demand peaks, smoothing out consumption profiles and reducing fuel consumption.
Water management remains critical in steam systems, and many facilities now deploy sophisticated water treatment and recycling technologies integrated with steam distribution. Effective removal of dissolved oxygen and other corrosive agents extends pipe and equipment life, while reuse of condensate reduces fresh water withdrawals. Facilities also track water use carefully to identify opportunities for efficiency gains and regulatory compliance, often integrating water metrics into overall sustainability reporting.
In terms of maintenance and reliability, predictive analytics powered by sensor data and machine learning are transforming steam distribution network management. Instead of relying solely on scheduled inspections, facilities use condition-based maintenance to anticipate failures before they occur. For example, vibration sensors on steam traps or ultrasonic monitoring of pipe integrity provide early warning of potential leaks or blockages, allowing targeted interventions that reduce downtime and repair costs.
The increasing complexity of steam distribution networks also encourages the use of digital twins—virtual replicas of the physical system that simulate performance under various scenarios. Operators and engineers can test modifications, optimize configurations, and train personnel in a risk-free environment. This digital approach accelerates innovation and improves operational safety and efficiency.
Safety enhancements are continually incorporated into steam distribution networks. Automated shutdown systems, pressure relief devices, and fail-safe valves are standard, but modern designs also include real-time monitoring for hazardous conditions like pressure spikes, steam leaks, or pipe ruptures. Coupled with remote alarm systems, these measures improve response times and protect personnel and equipment.
From an economic perspective, investments in steam distribution optimization often yield substantial returns through fuel savings, reduced maintenance, extended equipment lifespan, and regulatory compliance. Facilities that adopt comprehensive steam management programs typically see improvements in operational costs and environmental performance, supporting corporate sustainability goals and enhancing competitiveness.
In large industrial settings, steam distribution networks form a vital artery connecting energy production to process consumption. Their design, operation, and continual improvement represent a complex challenge requiring expertise across disciplines and a commitment to leveraging new technologies. As industries face increasing pressure to reduce emissions, improve efficiency, and enhance resilience, the role of optimized steam distribution networks becomes ever more central to achieving these objectives in a practical, reliable, and economically viable way.
Commercial Boiler Systems: How They Work
Commercial boiler systems are engineered setups designed to generate steam or hot water for heating, process needs, or power generation in commercial and industrial facilities. At their core, boilers convert energy—usually from burning fuels like natural gas, oil, coal, or biomass, or from electricity—into thermal energy by heating water contained within the system. This heated water or steam is then circulated throughout the building or plant to provide heating, power equipment, or support manufacturing processes.
The basic operation of a commercial boiler involves fuel combustion within a burner, producing hot gases that transfer heat to water inside the boiler’s pressure vessel through metal surfaces called heat exchangers. Depending on the design, boilers can be fire-tube or water-tube types. In fire-tube boilers, hot gases pass through tubes surrounded by water, while in water-tube boilers, water flows inside tubes heated externally by combustion gases. Water-tube boilers generally operate at higher pressures and temperatures, making them suitable for larger, high-capacity applications.
Once water is heated to produce steam or hot water, it is directed into a distribution system. For steam boilers, the steam pressure and temperature are controlled to meet specific requirements; steam can be saturated or superheated, depending on the application. Hot water boilers supply water at controlled temperatures, circulating it via pumps through radiators, heat exchangers, or process equipment.
Modern commercial boilers are equipped with safety devices such as pressure relief valves, low-water cutoffs, and flame detection systems to prevent hazardous operating conditions. Control systems manage fuel flow, combustion air, water level, and temperature to optimize efficiency and ensure safe operation. Many boilers also include economizers or condensing sections that recover residual heat from exhaust gases to preheat incoming water, enhancing fuel efficiency.
Fuel flexibility varies by boiler type and design; some systems can burn multiple fuel types or switch fuels to adapt to availability and cost. Additionally, boilers may be integrated with auxiliary equipment such as deaerators to remove dissolved gases from feedwater, reducing corrosion risk, and blowdown systems to control the concentration of impurities in boiler water.
In commercial buildings, boilers commonly provide space heating and hot water for sanitary use, often integrated with HVAC systems. In industrial settings, boilers support processes requiring steam, such as sterilization, chemical reactions, drying, or power generation via steam turbines.
Overall, commercial boiler systems are essential, reliable sources of heat and steam, combining robust engineering with safety and efficiency features tailored to a wide range of applications. Proper design, installation, operation, and maintenance are critical to ensuring their longevity, performance, and environmental compliance.
Commercial boiler systems rely heavily on precise control and monitoring to maintain optimal performance and safety. Automated control systems regulate combustion air and fuel ratios to achieve efficient burning, minimizing fuel consumption and emissions. These systems use sensors to continuously monitor parameters such as flame presence, combustion temperature, steam pressure, water level, and exhaust gas composition. Advanced control algorithms adjust the burner operation in real time to respond to varying load demands, ensuring steady steam or hot water output without wasteful overproduction or dangerous conditions like overheating or low water levels.
Fuel handling and supply are critical components of many commercial boiler installations. For boilers that burn solid fuels like coal or biomass, specialized feeding systems such as conveyors, feeders, or augers ensure a consistent fuel supply. Liquid and gaseous fuel systems include pumps, valves, and pressure regulators to maintain stable fuel delivery. Fuel storage and treatment may also be necessary, for example, fuel oil tanks with heating systems to maintain viscosity or gas filtration systems to remove impurities. Proper fuel management not only supports reliable boiler operation but also helps meet environmental regulations on emissions and fuel efficiency.
Water treatment and conditioning are essential to maintain boiler longevity and performance. Untreated feedwater can contain dissolved minerals, oxygen, and other impurities that cause scaling, corrosion, or foaming inside the boiler. Scaling reduces heat transfer efficiency and can lead to localized overheating, while corrosion damages metal surfaces and weakens structural integrity. Treatment typically involves chemical dosing to remove oxygen, soften water, and adjust pH, often combined with filtration and deaeration. Regular water testing and blowdown procedures help control contaminant levels, protecting boiler components and ensuring safe operation.
Maintenance of commercial boiler systems includes routine inspections, cleaning, and servicing to prevent failures and extend equipment life. Fire-tube boilers require periodic cleaning of combustion gaseside surfaces to remove soot and deposits, while water-tube boilers need inspections for tube integrity and potential leaks. Boiler controls and safety devices must be tested regularly to verify correct functioning. Preventive maintenance schedules often include checks of burners, fuel supply systems, pumps, valves, and instrumentation. Prompt identification and repair of leaks, corrosion, or mechanical wear reduce downtime and costly repairs.
In many commercial applications, boilers are integrated into larger building or process automation systems. This integration allows centralized monitoring and control of heating, ventilation, air conditioning, and process steam needs, improving overall energy management and occupant comfort. Energy management systems can optimize boiler operation by scheduling heating cycles based on occupancy or production schedules, and by coordinating with other energy sources like heat pumps or solar thermal systems. These smart systems contribute to energy savings, reduced emissions, and enhanced operational flexibility.
Environmental considerations play a growing role in the design and operation of commercial boilers. Regulatory limits on emissions such as nitrogen oxides (NOx), sulfur oxides (SOx), carbon monoxide (CO), and particulate matter require the use of cleaner fuels, improved combustion technology, or emissions control equipment like scrubbers and selective catalytic reduction systems. Condensing boilers, which recover latent heat from exhaust gases by condensing water vapor, achieve higher efficiencies and lower emissions, making them popular in commercial heating applications. Facility operators increasingly monitor emissions and fuel consumption closely, driven by sustainability goals and compliance requirements.
Overall, commercial boiler systems are complex, highly engineered installations that provide reliable and controllable heat and steam essential for a wide variety of commercial and industrial uses. Their effectiveness depends on careful design, proper fuel and water management, advanced control technologies, rigorous maintenance, and adherence to safety and environmental standards. As energy efficiency and environmental concerns grow in importance, commercial boiler systems continue to evolve with innovations that enhance performance, reduce emissions, and support integration into smarter, more sustainable facility energy systems.
Looking ahead, the future of commercial boiler systems is being shaped by technological advancements and shifting priorities toward sustainability and digitalization. One significant trend is the increasing adoption of low-emission combustion technologies. These include ultra-low NOx burners and staged combustion processes that reduce pollutant formation at the source. Coupled with real-time emissions monitoring and adaptive control systems, these technologies enable boilers to operate within stricter environmental regulations without sacrificing performance or efficiency.
Electrification is another emerging direction, where electric boilers are gaining traction in applications where emissions reduction and rapid responsiveness are key. Electric boilers eliminate on-site combustion, thus producing zero local emissions and reducing the complexity of fuel handling. Although traditionally more expensive to operate due to electricity costs, advances in renewable energy generation and grid decarbonization are making electric boilers an increasingly viable option for commercial facilities.
Integration with smart building and industrial Internet of Things (IoT) systems is transforming boiler management by providing comprehensive data collection, remote diagnostics, and predictive maintenance capabilities. Sensors embedded throughout the system monitor temperature, pressure, vibration, and fuel flow, feeding data into cloud-based analytics platforms. These platforms use machine learning algorithms to identify patterns indicative of emerging issues, enabling maintenance to be scheduled proactively before breakdowns occur, thereby reducing downtime and maintenance costs.
Hybrid systems combining boilers with other heat sources, such as heat pumps, solar thermal collectors, or waste heat recovery units, are also gaining momentum. These systems optimize energy use by switching between or combining heat sources depending on cost, availability, and environmental impact. For example, during periods of low heating demand, a facility might rely on heat pumps, reserving boiler use for peak loads or backup. Such flexibility enhances overall energy efficiency and lowers greenhouse gas emissions.
Water management technologies continue to evolve, with advanced water treatment systems minimizing chemical use and improving condensate recovery rates. Membrane filtration, reverse osmosis, and ultraviolet sterilization are increasingly integrated into boiler feedwater preparation, reducing fouling and corrosion while extending boiler life. Real-time water quality monitoring helps operators maintain optimal conditions and comply with regulatory standards.
Modular boiler designs offer greater scalability and ease of installation, allowing facilities to add capacity incrementally as demand grows. These smaller, factory-built units can be deployed quickly with less on-site construction, reducing capital costs and enabling more flexible energy strategies.
Economic pressures and regulatory incentives encourage facility managers to pursue energy audits and implement comprehensive steam and heating system optimization programs. These programs analyze system performance holistically, identifying inefficiencies in boiler operation, distribution piping, insulation, and end-use equipment. Corrective actions can yield substantial fuel savings, reduce maintenance expenses, and lower emissions.
Finally, workforce training and development are critical to supporting the sophisticated operation and maintenance of modern commercial boiler systems. As systems incorporate advanced controls, digital tools, and environmental technologies, technicians and engineers need ongoing education to ensure safe, efficient, and compliant operation.
In summary, commercial boiler systems are evolving rapidly, driven by environmental imperatives, technological innovation, and the digitization of energy management. Facilities that embrace these trends stand to benefit from improved efficiency, reduced emissions, greater operational flexibility, and lower lifecycle costs, positioning themselves well for a sustainable energy future.
Steam in Textile and Chemical Industries
Steam plays a vital and multifaceted role in both the textile and chemical industries, serving as an essential energy carrier and process medium that supports a wide range of manufacturing operations. In the textile industry, steam is integral to various stages such as dyeing, drying, finishing, and pressing of fabrics. Its consistent heat and high-temperature capacity enable precise control of process conditions, ensuring color fixation, moisture removal, and fabric texture development with high quality and efficiency. Steam-based heating is preferred because it delivers uniform temperature, rapid response, and easy control, which are critical for maintaining product standards and meeting tight production schedules.
During the dyeing process, steam is used to heat dye baths to the required temperatures, facilitating chemical reactions that bind dyes to fibers. This heat also aids in the penetration of dyes into textile fibers, enhancing color fastness and uniformity. In finishing operations, steam is used in calenders and presses to smooth, glaze, or emboss fabrics, improving their appearance and tactile properties. The drying phase often relies on steam-heated cylinders or tunnels to evaporate moisture without damaging delicate fibers. Moreover, steam can be used to generate humidification in textile mills, maintaining optimal humidity levels that prevent fabric shrinkage or static buildup.
In the chemical industry, steam’s versatility extends to providing process heat, driving reactors, distillation columns, and heat exchangers, and facilitating various unit operations that require controlled thermal energy. Steam is often a primary source of thermal energy for endothermic reactions, where precise temperature control influences reaction rates and product yields. It also powers turbines for mechanical drives or electricity generation within chemical plants, integrating energy supply with process operations.
Steam heating in chemical processes supports tasks such as solvent recovery, evaporation, crystallization, and sterilization. In distillation, steam may be injected directly (steam stripping) or used indirectly to supply heat to reboilers, enabling separation of chemical mixtures based on boiling points. Many chemical reactions require superheated steam to maintain necessary thermal conditions or to drive catalytic processes. Additionally, steam is used to maintain temperature in storage tanks or pipelines to prevent solidification or viscosity changes of certain chemicals.
Beyond heating, steam in both industries is involved in cleaning and sterilization of equipment, ensuring product quality and compliance with hygiene standards, especially in specialty chemical or textile applications involving medical textiles or high-purity chemicals.
The efficient management of steam systems is critical in these industries because steam production and distribution often represent significant energy costs. Optimizing boiler operation, minimizing steam losses, recovering condensate, and maintaining steam quality directly impact production costs and environmental footprint. Both industries benefit from integrated steam management practices that balance process needs with sustainability goals.
In summary, steam is a cornerstone utility in the textile and chemical industries, underpinning critical heating and processing functions with its reliable, controllable, and efficient thermal energy delivery. Its proper use enhances product quality, operational efficiency, and environmental performance, making it indispensable in these manufacturing sectors.
Steam’s role in the textile and chemical industries extends beyond just heat supply; it also serves as a driving force for various mechanical operations. In textile mills, steam turbines or engines may be used to power machinery directly, providing a compact and reliable source of mechanical energy. This use of steam-driven equipment reduces dependence on electrical systems in certain contexts, enhancing operational flexibility. Similarly, in chemical plants, steam turbines can generate electricity or drive compressors and pumps, integrating energy generation with process requirements and improving overall plant efficiency.
The quality of steam is particularly important in both industries to prevent equipment damage and ensure product consistency. Wet steam containing moisture droplets can cause corrosion, erosion, or uneven heating, which negatively affects delicate textile fibers or sensitive chemical reactions. Therefore, steam systems in these industries often include separators, dryers, and traps to maintain dry, high-quality steam. Regular monitoring and maintenance of steam traps, condensate return lines, and insulation are standard practices to minimize energy losses and maintain system reliability.
Condensate recovery is a key component in steam system efficiency within textile and chemical manufacturing. After steam transfers its energy in heating or processing, the condensate—still containing substantial thermal energy—is collected and returned to the boiler feedwater system. This recycling reduces fresh water consumption, lowers the energy required to produce steam, and reduces chemical treatment needs. Facilities often employ closed-loop condensate systems equipped with pumps and heat exchangers to maximize recovery and minimize waste.
The integration of steam with other utilities is common in these industries. For example, compressed air systems, cooling water circuits, and process water treatments are coordinated with steam generation and distribution to optimize overall plant energy use. Heat recovery from flue gases or waste streams further enhances energy efficiency by preheating feedwater or generating low-pressure steam for secondary processes.
Automation and control technologies have become increasingly important in managing steam in textile and chemical plants. Advanced control systems adjust boiler output, steam pressure, and temperature in response to real-time process demands, avoiding energy waste and stabilizing production quality. Data analytics and digital monitoring provide insights into system performance, enabling predictive maintenance and reducing downtime.
Environmental regulations also influence steam use in these industries, driving efforts to reduce emissions, improve fuel efficiency, and minimize water consumption. Many facilities are adopting cleaner fuels such as natural gas or biomass, installing emissions control devices, and implementing water conservation measures. These initiatives help meet regulatory requirements and align with broader sustainability goals, improving both environmental and economic outcomes.
In summary, steam is deeply embedded in the operational fabric of the textile and chemical industries, not only as a source of thermal energy but also as a key component in mechanical power, process control, and energy management. Its efficient and reliable use supports product quality, cost-effectiveness, and environmental stewardship, making steam systems a strategic asset in these sectors’ ongoing efforts to innovate and compete globally.
Looking forward, the continued evolution of steam technology in the textile and chemical industries is tightly linked to advancements in energy efficiency, digital integration, and sustainability. Both industries are under increasing pressure to reduce operational costs and environmental impact, and optimizing steam systems offers a significant opportunity to address these challenges. Emerging technologies such as AI-driven energy management platforms can analyze vast amounts of operational data to identify inefficiencies, predict maintenance needs, and recommend process adjustments that conserve fuel and reduce emissions.
Hybrid energy solutions combining steam with renewable sources are becoming more common. For instance, solar thermal collectors can preheat boiler feedwater or generate low-pressure steam, decreasing fossil fuel consumption. Biomass boilers and waste-to-energy systems provide alternative fuels that reduce carbon footprints while utilizing by-products or residues from the manufacturing process itself, promoting circular economy principles within the industries.
Advanced materials and coatings are being developed to enhance the durability and heat transfer efficiency of steam system components, reducing maintenance intervals and energy losses. Innovations in insulation materials help minimize heat dissipation from pipes and vessels, preserving steam quality throughout the distribution network. Modular steam system components allow easier upgrades and scalability, enabling facilities to adapt quickly to changing production demands or regulatory requirements.
Water conservation technologies continue to improve, focusing on minimizing consumption and enhancing condensate recovery rates. New treatment methods reduce chemical usage and wastewater generation, supporting stricter environmental regulations and corporate sustainability targets. The use of real-time water quality sensors and automated treatment dosing ensures optimal feedwater conditions, protecting boiler health and process integrity.
Workforce development remains a crucial factor in harnessing these technological advances. Training programs increasingly emphasize digital literacy, system integration skills, and sustainability awareness to equip technicians and engineers with the capabilities required for modern steam system operation and management.
Overall, steam’s enduring relevance in the textile and chemical industries is reinforced by its adaptability to emerging energy trends and environmental imperatives. By integrating cutting-edge technologies and sustainable practices, these industries can continue to leverage steam as a reliable, efficient, and versatile resource that supports both high-quality production and responsible resource stewardship well into the future.
Steam Applications in Pharmaceutical Processing
Steam is an indispensable utility in pharmaceutical processing, playing a crucial role in ensuring product quality, safety, and regulatory compliance. In pharmaceutical manufacturing, steam is primarily used for sterilization, heating, drying, and as a source of process energy. Its ability to deliver consistent, controllable, and high-temperature heat makes it ideal for critical operations where precision and hygiene are paramount.
One of the most vital applications of steam in pharmaceuticals is sterilization. Steam sterilizers, or autoclaves, use saturated steam under pressure to eliminate microorganisms from equipment, instruments, and products, ensuring that medicines are free from contamination. This sterilization method is favored because steam penetrates effectively, kills a broad spectrum of pathogens rapidly, and leaves no harmful residues. Steam sterilization is applied in sterilizing glassware, surgical instruments, packaging materials, and even the final product containers.
Steam is also employed in heating process vessels, reactors, and transfer lines to maintain precise temperature control during chemical reactions, blending, and formulation. Many pharmaceutical compounds require specific temperature ranges for proper synthesis or to maintain stability, and steam jackets or coils provide uniform heating that is easy to regulate. The rapid response of steam heating helps maintain consistent process conditions, reducing variability in product quality.
Drying of pharmaceutical ingredients and products is another area where steam plays a key role. Steam-heated dryers and evaporators remove moisture efficiently, which is critical in ensuring the stability and shelf life of medications. Maintaining controlled drying parameters with steam prevents degradation of sensitive compounds and ensures uniform product characteristics.
Steam is also used in clean-in-place (CIP) systems for cleaning and sanitizing production equipment without disassembly, minimizing downtime and contamination risks. The high temperature of steam aids in dissolving residues and killing microbes within pipes, tanks, and valves, supporting stringent hygiene standards.
Quality and purity of steam are paramount in pharmaceutical applications. Typically, clean steam or pharmaceutical-grade steam is generated using specially designed boilers and treatment systems to avoid contaminants like minerals, chemicals, or oils that could compromise product safety. This steam must meet strict standards for pressure, temperature, and purity as specified by regulatory bodies such as the FDA or EMA.
Energy efficiency and environmental impact are also important considerations. Pharmaceutical facilities often incorporate heat recovery systems, condensate return, and advanced boiler controls to optimize steam generation and minimize fuel consumption. Waste steam or condensate is carefully managed to prevent environmental harm and conserve resources.
In summary, steam’s versatility, reliability, and hygienic properties make it a cornerstone of pharmaceutical processing. Its applications in sterilization, heating, drying, and cleaning underpin the production of safe, effective medicines while meeting the industry’s rigorous quality and regulatory demands. Proper steam system design, operation, and maintenance are critical to supporting pharmaceutical manufacturing’s high standards and continuous innovation.
Steam’s importance in pharmaceutical processing extends beyond basic applications to advanced manufacturing techniques, where it supports critical steps like aseptic processing, formulation, and lyophilization. In aseptic processing, where products are manufactured in sterile environments without microbial contamination, steam is used to sterilize air filters, isolators, and cleanrooms. The clean steam ensures that all surfaces and equipment exposed to the product remain free from contaminants, protecting product integrity and patient safety.
Pharmaceutical formulations often require precise temperature control during mixing and chemical synthesis. Steam provides consistent heat to reaction vessels and jacketed tanks, allowing for tight control of reaction kinetics and ensuring reproducibility. This precision is vital when working with sensitive active pharmaceutical ingredients (APIs) or complex biologics, where slight temperature variations can affect efficacy or stability.
Lyophilization, or freeze-drying, is another process supported indirectly by steam. Steam-generated heat is used in the condenser and vacuum systems to facilitate moisture removal from freeze-dried products, which are often sterile injectables or biologics. The reliable energy supply steam provides ensures process efficiency and product quality.
Pharmaceutical plants also rely on steam for humidification in cleanrooms, maintaining controlled humidity levels critical for product stability and employee comfort. Proper humidity control prevents static electricity buildup, which could damage delicate electronic equipment or compromise sensitive powders and formulations.
The pharmaceutical industry demands steam systems designed to minimize contamination risk. This involves specialized piping, typically stainless steel, with sanitary welds and surfaces that prevent microbial growth. Steam traps and condensate removal must be carefully managed to avoid water hammer and ensure only dry steam contacts process equipment. Automated control systems maintain steam pressure and temperature within strict tolerances, allowing operators to monitor and adjust parameters in real time to meet process requirements.
Because pharmaceutical products have a global reach and strict regulatory oversight, documentation and validation of steam system performance are essential. Facilities maintain detailed records of steam quality tests, maintenance activities, and calibration of instrumentation to demonstrate compliance with Good Manufacturing Practices (GMP) and regulatory inspections.
Energy efficiency initiatives in pharmaceutical steam systems often include the use of condensing economizers, variable speed drives on pumps and fans, and integration with plant-wide energy management systems. These efforts reduce operational costs and environmental impact while maintaining the uncompromising quality standards the industry demands.
In essence, steam is a backbone utility in pharmaceutical manufacturing, enabling processes that demand sterility, precision, and reliability. Its careful management—from generation to distribution and recovery—ensures that pharmaceuticals are produced safely, efficiently, and consistently, supporting public health on a global scale.
Beyond its fundamental roles, steam in pharmaceutical processing is increasingly integrated with modern digital technologies to enhance operational control and compliance. Advanced monitoring systems use sensors and IoT devices to track steam quality parameters like pressure, temperature, humidity, and purity continuously. Real-time data allows for immediate detection of anomalies such as contamination, pressure drops, or condensate buildup, enabling rapid corrective actions that prevent production interruptions or compromised batches. These smart systems also support predictive maintenance by analyzing trends over time, reducing unplanned downtime and maintenance costs.
Sustainability concerns are driving pharmaceutical manufacturers to optimize steam generation and usage further. Many facilities are adopting cogeneration systems that simultaneously produce steam and electricity from the same fuel source, improving overall energy efficiency. Waste heat recovery units capture residual heat from exhaust gases or condensate to preheat boiler feedwater or supply low-pressure steam for secondary processes. By maximizing resource use and minimizing emissions, these innovations help companies meet stringent environmental regulations and corporate social responsibility goals.
Pharmaceutical steam systems also face unique challenges related to scalability and flexibility. As drug development increasingly moves toward personalized medicine and smaller production batches, steam systems must adapt to more variable load demands without sacrificing stability or quality. Modular steam generation units and flexible control systems allow facilities to scale output quickly, ensuring that small-scale or rapid-turnaround production runs maintain the same rigorous standards as large, continuous processes.
Training and workforce development remain essential to managing these increasingly sophisticated steam systems. Operators and engineers require specialized knowledge of both traditional steam engineering principles and modern digital control technologies. Ongoing education ensures that personnel can maintain system integrity, troubleshoot complex issues, and implement continuous improvements that enhance safety, efficiency, and compliance.
In summary, steam’s role in pharmaceutical processing is dynamic and evolving, blending time-tested thermal energy applications with cutting-edge technologies and sustainability initiatives. This synergy supports the industry’s critical mission of delivering safe, effective medicines while adapting to emerging manufacturing trends and regulatory landscapes. Properly managed steam systems remain foundational to pharmaceutical quality and innovation, reinforcing their place at the heart of modern healthcare production.
Steam Engines in Paper and Pulp Manufacturing
Steam engines have historically been central to the paper and pulp manufacturing industry, providing reliable mechanical power and thermal energy necessary for the complex processes involved in transforming raw wood and recycled fibers into finished paper products. Even as modern facilities increasingly rely on electric motors and steam turbines, steam engines and steam-driven systems continue to play important roles, especially in plants prioritizing energy efficiency and integration of steam as a multipurpose resource.
In paper and pulp manufacturing, steam is used extensively for heating, drying, and powering equipment. The process begins with the preparation of raw materials, where wood chips or recycled paper are treated chemically or mechanically to separate cellulose fibers. Steam-heated digesters facilitate the chemical pulping process by providing uniform high temperatures and pressure conditions necessary to break down lignin and free fibers without damaging them. This step is critical for producing pulp of consistent quality, which directly influences paper strength and texture.
Steam engines or turbines often drive large mechanical components such as grinders, refiners, and pumps. Historically, large stationary steam engines converted the thermal energy of steam into rotational mechanical power to operate these machines before widespread electrification. Today, steam turbines remain common in integrated pulp and paper mills, where steam produced in recovery boilers—using black liquor, a byproduct of pulping—supplies both electricity generation and mechanical drives, improving overall plant energy efficiency.
Drying is another energy-intensive stage in paper manufacturing, requiring steam to heat drying cylinders that remove moisture from the wet paper web as it moves through the production line. The consistent, controllable heat from steam ensures rapid evaporation without damaging the paper surface, enabling high-speed production of sheets or rolls with uniform moisture content. This steam-heated drying process significantly impacts final product quality, including strength, finish, and printability.
Beyond powering machinery and drying, steam is used for cleaning and sterilization within the plant, maintaining hygienic conditions and preventing buildup of residues that could affect paper quality or equipment longevity. Steam also supports environmental controls, such as treating effluents or operating scrubbers that reduce emissions from chemical processes.
Efficient management of steam in pulp and paper plants is essential due to the sector’s high energy consumption and environmental footprint. Integrated steam systems recover heat from waste streams, reuse condensate, and optimize boiler operation to minimize fuel use and emissions. Many mills operate combined heat and power (CHP) systems, capturing steam energy for both mechanical power and electricity generation while supplying process heat, achieving substantial energy savings and reducing greenhouse gas emissions.
In summary, steam engines and steam-powered systems remain fundamental to paper and pulp manufacturing by providing vital mechanical and thermal energy across multiple stages. Their integration with modern energy recovery and control technologies enables mills to produce high-quality products efficiently and sustainably, maintaining steam’s central role in this traditional yet evolving industry.
Steam’s role in paper and pulp manufacturing extends deeply into the energy and process infrastructure of mills. Recovery boilers, a hallmark of modern pulp mills, burn spent pulping liquors to generate high-pressure steam, which then powers steam turbines connected to electrical generators and mechanical equipment. This not only recycles chemical byproducts but also produces much of the mill’s energy needs, making the process more self-sufficient and reducing reliance on external fuels. The high-pressure steam from recovery boilers drives turbines that supply electricity for the entire plant and mechanical energy for critical operations, demonstrating an elegant synergy between chemical recovery and energy generation.
In addition to energy generation, steam supports refining processes where pulp fibers are mechanically treated to improve bonding and paper strength. Steam-heated refiners soften fibers and improve their flexibility, enhancing the final paper’s physical properties. This stage requires precise temperature control, which steam systems reliably provide. Furthermore, steam is used in paper coating and calendaring processes, where heated rollers improve surface smoothness and printability. Maintaining consistent steam supply and temperature is critical for producing high-quality coated papers and specialty products.
Steam system design in paper and pulp plants also incorporates extensive condensate recovery networks. Condensate, still rich in thermal energy, is collected from dryers, steam traps, and process equipment, then returned to boilers. This reduces water consumption and the energy needed to produce fresh steam, lowering operational costs and environmental impact. Proper maintenance of steam traps and insulation is vital to minimize energy losses and ensure efficient operation.
The pulp and paper industry’s environmental regulations push for cleaner operations, influencing steam system management. Advanced emission controls on boilers, combined with optimized combustion processes, reduce pollutants such as sulfur oxides, nitrogen oxides, and particulate matter. Water treatment systems integrated with steam generation help manage effluents, ensuring compliance with discharge limits while conserving water through recycling.
Automation and control systems enhance the reliability and efficiency of steam applications in pulp and paper manufacturing. Real-time monitoring of steam pressure, temperature, flow, and quality allows operators to optimize production, prevent equipment damage, and maintain product consistency. Predictive analytics help schedule maintenance before failures occur, reducing downtime and improving plant availability.
In emerging trends, some mills are exploring hybrid energy systems that incorporate renewable sources alongside traditional steam generation to further reduce carbon footprints. Biomass boilers fueled by wood residues and black liquor recovery remain cornerstones of sustainable energy practices, while solar thermal and waste heat recovery technologies are beginning to complement steam systems.
Overall, steam engines and steam-powered equipment remain deeply integrated into paper and pulp manufacturing processes, balancing traditional methods with modern efficiency and sustainability demands. Steam’s versatility as a heat source and mechanical power driver continues to support the industry’s goals of producing high-quality paper products while minimizing energy consumption and environmental impact.
Looking ahead, the future of steam applications in paper and pulp manufacturing is closely tied to advancements in energy integration, digitalization, and sustainability. As mills face growing pressure to reduce greenhouse gas emissions and energy costs, optimizing steam systems becomes a strategic priority. Enhanced process integration allows mills to capture more waste heat from various stages—such as drying, chemical recovery, and power generation—and recycle it into useful steam, reducing fuel consumption and improving overall energy efficiency.
Digital technologies, including IoT sensors and AI-driven analytics, enable more precise control and predictive maintenance of steam systems. By continuously monitoring parameters like steam quality, pressure fluctuations, and equipment wear, these systems can anticipate failures before they cause costly downtime and optimize boiler load to match production demand dynamically. This not only improves reliability but also reduces unnecessary fuel use and emissions.
Sustainability initiatives are driving increased adoption of biomass and bioenergy in steam generation, leveraging the industry’s abundant wood residues and black liquor to produce cleaner, renewable energy onsite. Some mills are exploring integration with district heating networks or co-locating with other industries to share steam and electricity resources, maximizing energy use across multiple facilities and reducing environmental footprints.
Innovation in materials and equipment design also supports steam system improvements. Advances in boiler and turbine technology boost thermal efficiency and operational flexibility, while better insulation and pipework design reduce heat losses. Modular steam generation units and smart steam traps improve adaptability and ease of maintenance, allowing mills to scale operations efficiently and respond quickly to market demands.
Water conservation remains a critical concern, with new treatment technologies and closed-loop condensate systems reducing water consumption and minimizing wastewater discharge. This aligns with stricter environmental regulations and growing community expectations around industrial water stewardship.
Workforce development continues to be essential as steam systems become more complex and digitally connected. Training programs focused on both traditional steam engineering and modern digital tools prepare operators and engineers to manage these evolving systems effectively, ensuring safe, efficient, and sustainable plant operation.
In essence, steam will remain a cornerstone of paper and pulp manufacturing, but its role will continue to evolve through integration with advanced technologies and sustainability practices. This evolution will help the industry meet future challenges, maintain product quality, and operate with greater environmental responsibility, securing steam’s place as a vital resource in one of the world’s most energy-intensive industries.
Troubleshooting Steam Turbine Failures and Issues
Troubleshooting steam turbine failures and issues requires a methodical understanding of both the mechanical and thermal systems that comprise the turbine and its supporting infrastructure. Steam turbines operate under extreme pressure, temperature, and rotational speeds, so even minor deviations in operating conditions can lead to significant performance loss, mechanical wear, or outright failure. Identifying and correcting these problems promptly is essential for minimizing downtime, avoiding costly repairs, and maintaining safe, efficient operation.
One of the most common issues encountered in steam turbines is vibration, which can result from imbalance in the rotor, misalignment of couplings, worn bearings, or steam flow instability. Vibration can cause fatigue in components over time, leading to cracks or complete structural failure. Troubleshooting begins with vibration analysis using sensors and software to detect patterns that indicate specific causes. For example, a dominant frequency corresponding to rotor speed suggests imbalance, while harmonics may point to misalignment or looseness. Once identified, the issue can be corrected through balancing, alignment procedures, or replacing faulty components.
Another critical area of concern is blade erosion, often caused by wet steam, contaminants, or foreign particles entering the turbine. Erosion reduces blade efficiency and can lead to fractures. Inspecting steam quality and installing proper filters or moisture separators can mitigate this issue. In cases where erosion has already occurred, damaged blades must be replaced or refurbished, and upstream conditions should be improved to prevent recurrence.
Thermal expansion and differential heating can also lead to rotor distortion or casing misalignment, particularly during startup or shutdown if temperature ramping is too rapid. Symptoms may include difficulty achieving rated speed, abnormal thrust bearing wear, or contact between rotating and stationary parts. Monitoring temperature gradients and implementing controlled warming and cooling sequences can prevent thermal stress-related problems. Corrective action may involve resetting clearances or adjusting operational procedures.
Oil system failures are another frequent cause of steam turbine issues. Contaminated or degraded lubrication oil can lead to bearing damage, increased friction, and overheating. This may present as rising bearing temperatures or high vibration levels. Routine oil analysis helps detect early signs of contamination, and maintaining proper filtration, reservoir cleanliness, and oil quality extends bearing life and turbine reliability. In some cases, replacing damaged bearings and flushing the lubrication system may be necessary.
Seal and gland leakage can reduce turbine efficiency and pose safety risks. Excessive steam leakage from seals may indicate worn labyrinth seals, improper assembly, or casing distortion. Gland steam pressure should be carefully controlled, and routine inspections performed to ensure sealing surfaces are intact. In vacuum sections, air ingress due to packing wear or pipe leaks can disrupt condenser performance and reduce overall turbine output. Leak detection techniques such as pressure decay or helium tests can locate the sources, which are then repaired or resealed.
Control system issues can manifest as instability in turbine speed, poor load response, or failure to follow setpoints. These may be due to faulty sensors, stuck valves, or malfunctioning actuators. Troubleshooting typically involves checking signal integrity, verifying calibration of transducers, and testing actuator movement. Modern digital control systems offer diagnostic tools that assist in isolating faults and restoring control precision.
Steam path deposits, often due to carryover of boiler water impurities, can accumulate on blades and diaphragms, restricting flow and altering pressure profiles. These deposits reduce efficiency and may cause mechanical imbalance. Regular chemical analysis of steam and water, combined with proper boiler blowdown and treatment, prevents fouling. If deposits are found, offline chemical cleaning or manual removal may be required.
In conclusion, successful troubleshooting of steam turbine failures hinges on systematic condition monitoring, precise diagnostics, and well-documented maintenance practices. By recognizing symptoms such as vibration, noise, temperature fluctuations, or performance drops and tracing them back to root causes, operators can implement corrective actions that restore reliable, efficient operation. Ongoing analysis, predictive maintenance tools, and skilled personnel remain key to preventing future problems and extending the turbine’s operational life.
Steam turbine troubleshooting also involves understanding how external factors like operational loads, process changes, and startup/shutdown sequences impact turbine health over time. Frequent or rapid cycling, for example, introduces repeated thermal stresses that can weaken materials and lead to cracking in rotors, casings, or internal components. These thermal fatigue issues often begin subtly, presenting as minor changes in alignment or unexpected vibration, but they can develop into serious structural damage if ignored. To counter this, operators must closely follow OEM-recommended warm-up and cool-down procedures, ensuring that all parts expand and contract gradually to minimize internal stresses.
Another nuanced challenge arises in the form of partial discharge and electrical insulation failures in generator systems connected to steam turbines. Although not part of the turbine itself, generator issues can feed back into the mechanical system, causing load instability or sudden trips. Careful coordination between turbine and generator condition monitoring is vital. Infrared thermography, insulation resistance testing, and partial discharge detectors help assess generator health and prevent cascading failures that begin electrically but end mechanically.
Hydraulic control issues, particularly in older turbines with servo-hydraulic actuators, can also be problematic. These systems respond to governor and control signals to modulate steam valves and other critical elements. If hydraulic fluid is contaminated, pressure regulators fail, or servo valves stick, the result can be erratic control, overspeed events, or sluggish response to load changes. These symptoms can mimic mechanical problems, making it essential to inspect hydraulic filters, clean or replace valves, and verify actuator motion as part of a comprehensive diagnostic routine.
In high-pressure turbines, stress corrosion cracking is another failure mode that demands attention. This form of damage, typically affecting rotor and blade materials exposed to high stress and corrosive steam impurities, can develop unnoticed until a failure occurs. Nondestructive testing methods like ultrasonic inspection, magnetic particle testing, and dye penetrant techniques are used to detect early-stage cracking during scheduled outages. Where detected, components may require grinding, localized repair, or full replacement depending on the severity.
Steam bypass system problems can also influence turbine health indirectly. These systems are used during startup or load rejection to divert steam safely and maintain pressure control. If bypass valves leak, fail to open or close properly, or are out of calibration, the main turbine may experience pressure surges or thermal shocks. Routine stroke tests and actuator checks help ensure bypass systems operate as intended and protect the turbine from abrupt load transitions.
Operators should also consider ambient and environmental conditions. In coastal or humid environments, the presence of chlorides in the air or feedwater can accelerate corrosion, especially in condensers and low-pressure turbine stages. Adequate sealing, corrosion-resistant materials, and water chemistry control are key defenses. Likewise, seasonal variations in cooling water temperature affect condenser vacuum and, consequently, back-pressure on the turbine. Monitoring condenser performance and maintaining vacuum integrity are essential to preserving thermal efficiency.
Documentation and trend analysis play a crucial role in effective troubleshooting. Modern control systems typically archive a wealth of operating data, allowing engineers to identify patterns and subtle performance shifts long before alarms trigger. By reviewing historical data on vibration, load fluctuations, steam temperatures, and turbine speed trends, operators can pinpoint deviations from baseline conditions and intervene proactively. The use of digital twins or performance simulation models further enhances this capability, offering a virtual reference against which actual turbine behavior can be compared.
Ultimately, effective troubleshooting of steam turbines is a balance of technical skill, experience, instrumentation, and preventive culture. It demands not only the ability to react to symptoms but also the foresight to predict and prevent failures through consistent monitoring, proper maintenance, and attention to every detail—from steam purity to oil chemistry to control logic. As turbines remain critical assets in power generation, industrial processing, and cogeneration applications, maintaining their integrity is both an operational and strategic priority.
To further ensure reliable operation and efficient troubleshooting of steam turbines, collaboration among operators, maintenance engineers, and original equipment manufacturers (OEMs) is essential. OEMs often provide detailed guidelines on acceptable vibration thresholds, clearance tolerances, steam purity specifications, and overhaul intervals, which should be strictly followed and integrated into the facility’s maintenance strategy. Deviations from these standards can lead to a cascade of failures—such as axial displacement exceeding bearing limits or pressure drops indicating nozzle clogging—that are avoidable with vigilant adherence to best practices.
Turbine overhauls, though infrequent, are vital opportunities to assess internal wear, check alignment, measure clearances, and inspect all rotating and stationary parts for pitting, erosion, or fatigue. During these overhauls, components like rotor blades, diaphragms, bearings, seals, and casings undergo thorough inspection and, if necessary, refurbishment or replacement. Detailed records of all measurements and findings should be maintained to inform future condition assessments and trend analysis. Over time, this data becomes an invaluable resource for predicting wear rates and scheduling future interventions more efficiently.
In some cases, performance degradation may not stem from a single component failure but from a combination of small inefficiencies across multiple systems—such as a slightly fouled condenser, marginally degraded steam seals, and a partially blocked feedwater heater—all contributing to a noticeable drop in turbine output. This cumulative effect underscores the importance of viewing the steam turbine as part of a larger thermodynamic system rather than in isolation. An integrated performance assessment, including heat rate analysis, enthalpy drop calculations, and back-pressure evaluations, can help locate where energy is being lost and guide targeted improvements.
Steam chemistry also plays a critical, though often underappreciated, role in turbine longevity. Impurities such as silica, sodium, and chlorides—if not properly managed in boiler feedwater—can lead to deposition, corrosion, or stress-assisted cracking in turbine internals. Strict water chemistry control through continuous monitoring of conductivity, pH, dissolved oxygen, and chemical dosing ensures that steam remains pure and non-aggressive to turbine materials. Condensate polishing systems and demineralization units must be maintained diligently to support this effort.
The human factor cannot be overstated. Operator training and awareness are as crucial as the hardware itself. Understanding normal turbine sounds, temperature behavior during load changes, and the implications of seemingly minor alarm conditions can make the difference between a quick fix and a major shutdown. Hands-on experience, complemented by simulator training and periodic refresher courses, equips operators with the skills to recognize early warnings and respond effectively under pressure.
Looking forward, predictive maintenance technologies continue to reshape turbine troubleshooting. By integrating machine learning with sensor data, facilities can now forecast probable failure points with increasing accuracy. This shift from reactive to proactive maintenance reduces unplanned downtime and helps allocate resources more effectively. However, these tools must be fed with clean, consistent data and interpreted by knowledgeable personnel who understand both the physics of the turbine and the limitations of the analytics.
In sum, troubleshooting steam turbine issues is not simply a technical process—it is a disciplined practice of observation, analysis, collaboration, and continuous learning. Whether managing a small industrial turbine or a large utility-grade machine, success depends on the seamless integration of precision monitoring, rigorous maintenance, effective documentation, and a deeply embedded culture of reliability. By embracing both traditional engineering principles and modern diagnostic innovations, turbine operators can extend equipment life, reduce costs, and ensure stable power generation or industrial output for years to come.
Steam Turbine Control Systems: How They Work

Steam turbine control systems are integral to the safe, efficient, and reliable operation of steam turbines, ensuring they perform as intended under varying load conditions, startup and shutdown sequences, and emergency scenarios. These systems manage the flow of steam into the turbine, regulate the turbine’s speed, monitor critical parameters, and protect the turbine from damage due to operational anomalies. At their core, they comprise a blend of mechanical, hydraulic, and increasingly digital or electronic components that work in concert to provide real-time control over the turbine’s behavior.
At the heart of any steam turbine control system is the governor, which maintains the turbine’s speed within tightly controlled limits. In mechanical-hydraulic systems, this is traditionally accomplished using a flyball governor that adjusts steam valve positions in response to changes in rotational speed. Modern control systems, however, typically use digital electronic governors, or electro-hydraulic governors, which provide faster, more precise control. These systems rely on speed sensors and programmable logic controllers (PLCs) or distributed control systems (DCS) to interpret feedback signals and command actuators accordingly.
A key function of the control system is managing steam admission via control valves—typically stop valves and control (or governing) valves. The stop valves serve as the first line of safety, quickly closing to isolate the turbine from the steam source in case of an emergency trip. Control valves modulate the amount of steam entering the turbine, thereby regulating speed and load. In multi-stage turbines, these valves can be sequenced to introduce steam to different stages depending on power demand, a process known as sequential or nozzle governing.
Load control is another critical aspect of turbine operation, particularly for turbines connected to electrical grids. In grid applications, the control system ensures that the turbine supplies consistent power output and maintains synchronization with the grid frequency. This involves load-sharing logic in cogeneration or parallel setups and automatic generation control (AGC) in larger plants. The system continuously adjusts valve positions to match turbine output to demand without overshooting or undershooting the desired load.
Temperature and pressure regulation are also vital. The control system monitors main steam temperature and pressure, turbine casing temperatures, and exhaust conditions to ensure the turbine operates within its design limits. Sensors feed this data into the control logic, which can adjust bypass systems, start auxiliary heaters, or modulate valves to protect components from thermal stress or overpressure conditions. In high-efficiency plants, reheating stages may be coordinated through the same system to balance performance and safety.
Another major function is turbine startup and shutdown sequencing. Steam turbines require careful ramp-up and cool-down to prevent thermal shock and mechanical distortion. The control system automates this process by gradually opening valves, adjusting steam flow, and monitoring metal temperatures to ensure that startup occurs in a controlled, step-by-step fashion. Similarly, during shutdown, the system ensures steam is gradually withdrawn and turbine speed reduced in a way that avoids mechanical stress or vacuum collapse in the condenser.
Modern steam turbine control systems are increasingly integrated with condition monitoring and diagnostics platforms. This integration allows for continuous tracking of vibration, bearing temperature, oil pressure, and other health indicators. Alarms and interlocks can automatically trip the turbine if unsafe conditions are detected—such as overspeed, high thrust bearing temperature, or lube oil failure. This automated protection system is crucial for preserving both equipment and personnel safety.
Human-machine interfaces (HMIs) provide operators with real-time visualizations of turbine conditions and control parameters. These interfaces allow for manual overrides, parameter tuning, and performance analysis. Operators can observe trends, review alarm histories, and simulate control actions to improve turbine response and identify potential inefficiencies.
Ultimately, steam turbine control systems are the brain of turbine operation. They ensure the turbine starts, runs, and stops in a predictable, optimized manner; respond intelligently to system demands and grid fluctuations; and provide layered protection against failure. As digital control technologies evolve, these systems are becoming more autonomous, data-driven, and capable of predictive maintenance, making them an increasingly vital part of modern energy infrastructure.
Steam turbine control systems also incorporate sophisticated safety mechanisms designed to automatically shut down the turbine under hazardous conditions, such as overspeed, low lube oil pressure, or high exhaust temperature. Overspeed protection is particularly critical, as excessive rotational speed can cause catastrophic mechanical failure. To prevent this, most turbines are equipped with both primary electronic overspeed detection and an independent mechanical overspeed trip device. These systems continuously monitor turbine speed, and if a threshold—usually around 110% of rated speed—is breached, they trigger immediate closure of the steam stop valves, halting steam admission and bringing the turbine to a safe stop.
Another core element of control is load shedding and load rejection handling. In the event of a sudden loss of electrical load—such as when a generator disconnects from the grid—the turbine must rapidly reduce steam input to prevent overspeed. The control system must respond within fractions of a second to this kind of transient event. Modern systems employ fast-acting servo valves and finely tuned control loops to instantly throttle steam, stabilize speed, and protect both the turbine and the electrical network from disturbances.
The integration of feedforward and feedback control strategies is another refinement seen in advanced systems. Feedback control uses real-time sensor inputs to correct deviations from target values, while feedforward control anticipates changes based on known disturbances or planned operations, such as expected load increases. This combination enhances system responsiveness and minimizes instability, especially in complex applications like cogeneration plants where steam extraction and heat supply must be tightly coordinated with electrical generation.
Redundancy is often built into turbine control architectures to ensure high reliability. Critical sensors like speed pickups, pressure transducers, and temperature elements are often installed in duplicate or triplicate configurations. Control processors may operate in parallel, with voting logic determining valid signals and suppressing anomalies. This redundancy ensures the turbine remains under control even if one or more components fail. Additionally, the system software often features watchdog timers and heartbeat signals to monitor the health of the controllers themselves, enabling fast switchover to backup systems if a fault is detected.
As part of broader plant automation systems, turbine controls are frequently integrated into centralized Distributed Control Systems or SCADA platforms, enabling remote supervision, diagnostics, and coordination with other plant equipment like boilers, generators, and condensers. This integration allows for real-time optimization based on overall plant efficiency, not just turbine output. For example, if a turbine is operating under partial load, the system may adjust steam conditions or extraction levels to maintain optimal thermal balance and fuel efficiency across the entire facility.
In industrial environments where steam is used for both mechanical drive and process heating, control systems also manage steam extraction points and backpressure regulation. The system must delicately balance power generation with steam requirements for heating, drying, or other processes, often under dynamic conditions. This calls for complex logic that modulates extraction valves based on downstream pressure sensors and process demand forecasts, maintaining both stable turbine operation and uninterrupted service to the process loads.
Cybersecurity has emerged as a growing concern in turbine control systems, particularly as they become more connected to plant-wide networks and external interfaces. Unauthorized access to control logic or supervisory systems poses real risks to operational safety and continuity. As a result, turbine control architectures increasingly include secure communication protocols, firewalls, user authentication, and audit trails to protect against intrusion or sabotage.
Training and simulation also play a key role in leveraging the full potential of steam turbine control systems. Digital twin models allow operators to simulate turbine behavior under various scenarios, from routine load changes to emergency shutdowns. These simulations improve operator response times, refine startup and shutdown strategies, and allow engineers to test control system updates or tuning adjustments in a virtual environment before applying them in the field.
In the end, the complexity and precision of modern steam turbine control systems reflect the essential role turbines play in energy and process industries. These systems do more than regulate speed and load—they act as intelligent guardians of turbine performance, integrating protection, optimization, and communication functions to ensure that these machines deliver maximum reliability, efficiency, and longevity in a wide range of demanding applications.
As control systems continue to evolve, their role in predictive maintenance and lifecycle management of steam turbines becomes even more pronounced. Embedded sensors and analytics platforms now allow for the early detection of performance degradation, component fatigue, and mechanical imbalances long before they manifest as noticeable issues. These systems collect vast amounts of data—vibration levels, bearing temperatures, steam valve positions, rotor speeds, and pressure profiles—and use algorithms to identify deviations from baseline conditions. By trending this data over time, operators can forecast when maintenance should be performed, schedule it during planned outages, and avoid costly unplanned downtime.
Advanced analytics also help in refining control strategies. Turbine performance curves and control loop behaviors can be adjusted based on real-world data to improve efficiency at part loads, enhance response times during load transitions, and reduce wear on moving components. Some systems are now equipped with machine learning models that continuously optimize control parameters based on turbine age, environmental conditions, and operational history. These models can identify patterns of inefficiency or instability and recommend control logic adjustments or hardware checks. Though these systems require careful tuning and validation, they represent a step toward more autonomous, adaptive turbine operation.
Environmental compliance is another domain increasingly influenced by turbine control systems. In plants subject to emissions regulations, the turbine’s steam flow must be coordinated with the combustion process and emissions control equipment. The turbine control system communicates with boiler controls, feedwater regulators, and flue gas treatment systems to ensure that steam production stays within environmental limits. For example, when operating at low loads, the control system might reduce steam flow rates or divert excess steam to maintain the proper combustion-air ratio and minimize emissions.
In CHP (Combined Heat and Power) and district energy systems, turbine control extends beyond the turbine itself into the broader thermal and electrical distribution network. Here, load-following capability is critical. The control system must adapt quickly to changes in thermal demand, such as fluctuations in heating or cooling loads in urban buildings. This calls for highly responsive extraction control and tight integration with building management systems or district heating operators. These dynamic interactions are governed by coordinated control logic that can prioritize thermal output or electrical generation based on real-time demand and economic factors.
One of the most subtle yet critical functions of the control system is maintaining mechanical stress within acceptable limits during transient conditions. Rapid load changes, emergency shutdowns, or steam source disruptions can impose significant torsional and thermal stresses on turbine components. The control system mitigates this by carefully controlling the rate of steam admission and extraction, monitoring thermal gradients, and sequencing auxiliary systems like gland sealing steam, condenser vacuum, and lube oil pumps. The goal is to maintain smooth transitions that protect the turbine’s structural integrity and reduce long-term fatigue.
Control system upgrades are also a frequent aspect of turbine lifecycle management. As older analog systems become obsolete, they are replaced with digital platforms that offer better diagnostics, remote access, and future compatibility. These upgrades are complex, requiring detailed mapping of existing wiring, careful signal calibration, and rigorous testing to ensure no function is lost or misinterpreted. When done correctly, they provide a leap in reliability and functionality, making it easier to interface with modern plant controls and external monitoring services.
Furthermore, as decarbonization efforts expand, steam turbines operating in renewable or hybrid plants are experiencing new modes of control. In solar-thermal or biomass plants, steam conditions may fluctuate more than in traditional fossil-fueled plants. The turbine control system must accommodate these variations, managing partial loads, variable steam quality, and frequent starts and stops. Controls must be flexible and robust enough to handle these challenges without compromising turbine safety or efficiency.
Ultimately, steam turbine control systems are the culmination of mechanical engineering, thermodynamics, electronics, and software working in seamless harmony. They not only ensure safe operation but also elevate the turbine’s performance by making it smarter, more adaptive, and more resilient. Whether in traditional power stations, industrial co-generation setups, or renewable energy hybrids, the sophistication of control systems defines how well steam turbines meet the challenges of modern energy systems.
The Role of Steam Turbines in Combined Heat and Power (CHP) Systems
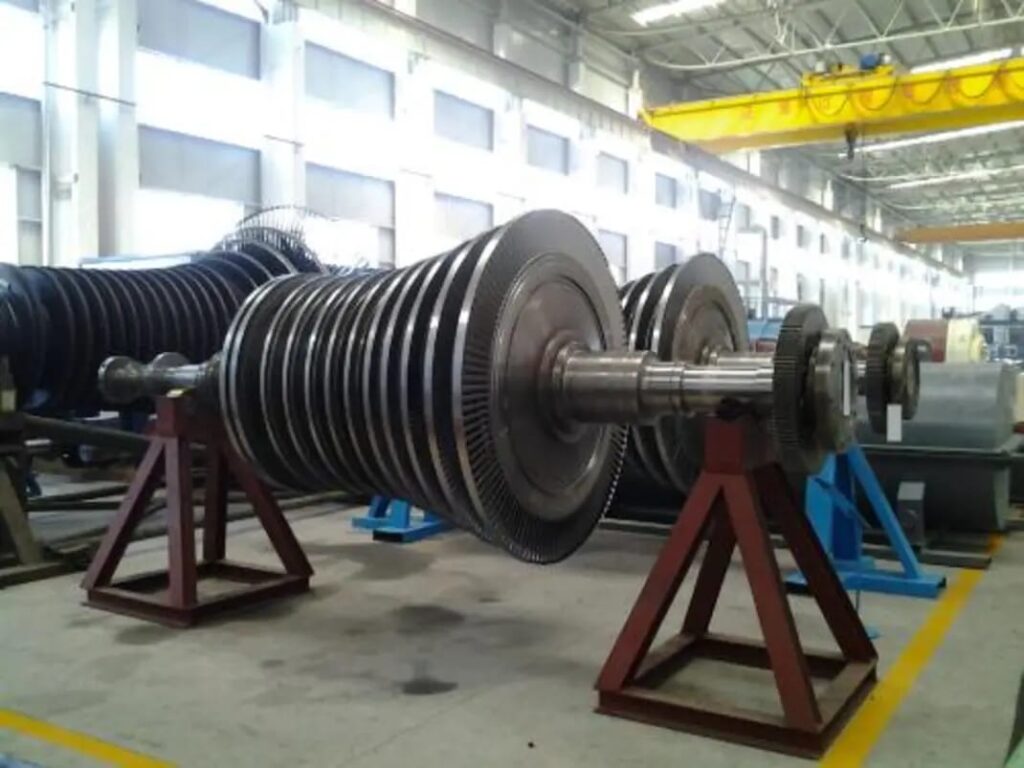
Steam turbines play a pivotal role in Combined Heat and Power (CHP) systems by enabling the simultaneous generation of electricity and useful thermal energy from a single fuel source, which significantly improves overall energy efficiency. Unlike conventional power plants that waste large amounts of energy as heat during electricity generation, CHP systems harness this thermal byproduct—often through the use of a steam turbine—to meet industrial or district heating demands, resulting in fuel-use efficiencies that can exceed 80%.
In a typical CHP system, high-pressure steam is produced in a boiler or heat recovery steam generator (HRSG) and then expanded through a steam turbine to generate electricity. As the steam exits the turbine at a lower pressure and temperature, instead of being condensed and wasted, it is directed to supply heat for various purposes such as space heating, water heating, industrial processes, or absorption chillers for cooling. This extraction of heat post-expansion is what differentiates CHP from a conventional condensing turbine cycle.
Steam turbines used in CHP configurations are often designed for back-pressure or extraction-back-pressure operation. In a back-pressure turbine, all the steam exits the turbine at a usable pressure level and goes directly to the thermal process. In extraction systems, a portion of the steam is tapped from intermediate stages at specified pressures to serve heating needs, while the remainder may continue to generate additional power or be condensed. The flexibility of this design allows for optimization of electrical and thermal outputs based on demand, making the system highly adaptable to seasonal and operational variations.
One of the key advantages of steam turbines in CHP systems is their ability to deliver stable and continuous power and heat, making them ideal for applications with steady energy loads such as hospitals, universities, food processing plants, chemical industries, and district heating networks. Their robustness and long operational life also contribute to lower lifecycle costs and higher reliability compared to internal combustion engines or gas turbines in certain CHP scenarios.
Moreover, steam turbine-based CHP systems can be integrated with a wide range of fuels including natural gas, coal, biomass, municipal waste, and even geothermal heat, providing fuel flexibility and supporting decarbonization initiatives. Biomass-fired CHP plants, for instance, combine renewable fuel with the proven efficiency of steam turbines to deliver both power and process steam with a low carbon footprint.
Control systems within these CHP installations are tasked with managing the turbine’s performance while responding to variable steam and heat loads. They balance the extraction rates and turbine output to ensure neither electricity generation nor thermal delivery is compromised. When electricity demand is low but heat demand remains high, the turbine may operate more as a thermal engine, prioritizing heat delivery. Conversely, during high electrical demand, more steam can be routed through to generate power, depending on the process requirements.
Environmental benefits of steam turbine CHP systems are substantial. By capturing and using waste heat that would otherwise be discarded, these systems reduce overall fuel consumption and emissions, particularly CO₂, NOₓ, and SO₂. This not only lowers the environmental footprint of industrial and commercial facilities but can also help them meet stringent regulatory standards and qualify for incentives tied to energy efficiency and sustainability.
In conclusion, steam turbines are at the core of many effective CHP systems, enabling the efficient use of fuel to meet both power and thermal needs. Their flexibility, reliability, and compatibility with various fuel types make them a central technology in efforts to increase energy efficiency, reduce emissions, and enhance energy resilience across a wide range of applications.
The continued development and application of steam turbines in CHP systems reflects their enduring value in both industrial and municipal energy strategies. As the demand for energy efficiency and sustainability grows, especially in urban areas and energy-intensive industries, steam turbine-based CHP solutions offer a compelling way to meet these goals without fundamentally overhauling existing infrastructure. Their ability to operate continuously at high load factors makes them especially suitable for base-load energy provision, ensuring that critical facilities like hospitals, data centers, and manufacturing plants remain powered and heated around the clock. This reliability is particularly vital in regions where electrical grid stability is uncertain or where energy costs are high, as CHP systems can operate independently or in parallel with the grid, providing backup power and reducing peak demand charges.
Furthermore, the scalability of steam turbines allows CHP systems to be tailored to different project sizes—from small-scale installations that serve a single building or industrial process to large centralized plants that provide heat and electricity to entire districts. In district energy systems, steam turbines form the backbone of cogeneration plants that distribute steam through underground pipe networks to buildings for space heating, domestic hot water, or even industrial use. The ability to generate electricity and distribute heat in a coordinated fashion results in optimized fuel use and a smaller environmental footprint for urban centers. As cities increasingly pursue decarbonization goals, retrofitting or expanding district heating networks powered by biomass or waste-fueled CHP plants becomes an attractive pathway, with steam turbines continuing to serve as the primary mechanical engine driving both energy outputs.
Another growing area of application is in industrial parks or eco-industrial clusters, where a central CHP plant powered by a steam turbine serves multiple facilities with both electrical and thermal energy. This shared approach to energy production promotes resource efficiency, lowers costs through economies of scale, and facilitates the integration of renewable and waste-derived fuels. For instance, in pulp and paper mills, sugar refineries, or chemical plants where steam is already required for processes, installing a back-pressure steam turbine allows excess thermal energy to generate electricity, improving the site’s overall energy balance without requiring additional fuel. These integrated solutions not only enhance profitability but also contribute to corporate sustainability objectives and carbon reduction commitments.
As energy markets evolve with increasing penetration of intermittent renewables such as wind and solar, steam turbine CHP systems also have the potential to complement these technologies by providing dispatchable thermal and electrical energy. While solar and wind may fluctuate with weather and time of day, a CHP system can run steadily, helping stabilize the grid and ensuring thermal demand is met regardless of renewable output. In hybrid configurations, surplus renewable energy could even be used to generate steam (via electric boilers or resistive heating) and stored as thermal energy for later use, effectively turning the steam turbine into part of a larger energy storage and conversion platform. This flexibility supports both grid reliability and deep decarbonization.
One of the important technical trends is the integration of steam turbines with digital monitoring and optimization tools in CHP systems. Real-time performance data, predictive maintenance algorithms, and load forecasting tools are now being used to enhance turbine efficiency and reliability. Operators can monitor steam pressures, temperatures, vibration patterns, and load distributions to fine-tune turbine performance and ensure optimal coordination between electrical and thermal outputs. These smart systems improve uptime, reduce operating costs, and extend equipment life, all while ensuring regulatory compliance and environmental safety.
Looking ahead, policy incentives, carbon pricing mechanisms, and the ongoing shift toward decentralized energy generation are expected to further drive the adoption of CHP systems with steam turbines at their core. Governments and industries alike are recognizing the dual benefits of increased energy efficiency and reduced emissions that CHP offers. As more regions introduce carbon penalties for wasteful generation and reward efficient energy use, steam turbine CHP systems stand out as a proven, adaptable, and increasingly essential technology for modern, resilient energy infrastructures. Whether deployed in industrial retrofits, new district heating projects, or renewable-fueled hybrid systems, steam turbines will remain a central player in the transition toward a more efficient and sustainable energy future.
The advancement of steam turbine technology in CHP systems is also being shaped by innovations in materials, design, and integration techniques. Turbine manufacturers are continually developing components that can withstand higher temperatures and pressures, allowing for more efficient steam cycles and reduced fuel consumption. Materials such as advanced alloys and coatings are enabling longer operational life and better performance under demanding thermal conditions. This means that steam turbines in CHP settings can now achieve efficiencies and output levels that were previously limited to large utility-scale power plants, making them viable for a broader range of applications.
Parallel to this, modular CHP solutions featuring steam turbines are gaining traction, especially in settings where installation space or capital investment is constrained. These modular systems can be prefabricated, transported to the site, and installed with minimal disruption to existing operations. This approach reduces installation time and costs, while also allowing for easier scalability. If demand for heat or electricity grows over time, additional modules can be added or upgraded without overhauling the entire system. This flexibility is valuable in industries undergoing gradual expansion or urban centers where infrastructure development must occur in phases.
Another area where steam turbines are seeing expanded use in CHP is in waste-to-energy plants. Here, municipal solid waste, industrial waste, or biomass is combusted to produce steam, which then powers a steam turbine to generate electricity while providing district heating or process steam. These facilities not only offer a method of waste reduction and energy recovery but also contribute to circular economy principles by turning local waste streams into useful energy. With growing emphasis on sustainable waste management, steam turbine CHP units are becoming cornerstones of environmentally responsible infrastructure planning.
In developing economies, steam turbine-based CHP systems also hold promise for improving energy access and industrial productivity. Many regions lack robust electrical grids but have localized sources of biomass or agricultural waste that can be used to fuel boilers. Deploying CHP systems in these settings can provide reliable, decentralized electricity and heat for processing food, textiles, or chemicals—industries that often struggle with unreliable grid power. The ability of steam turbines to operate on a variety of fuels, including low-grade or unrefined biomass, makes them particularly valuable for energy resilience in rural or remote areas.
From a financial standpoint, the economics of steam turbine CHP systems are often favorable when properly sized and integrated. The high efficiency of cogeneration results in lower fuel costs per unit of energy produced, while revenues or savings from electricity generation and thermal energy offset initial capital investments. Many regions also offer incentives, tax credits, or grants for CHP installations, particularly when they contribute to emissions reductions or grid stability. Additionally, companies that invest in CHP with steam turbines often see improvements in energy security and independence, protecting them from volatile energy markets and supply disruptions.
As the world moves toward more distributed and decarbonized energy systems, steam turbines in CHP applications provide a bridge between traditional centralized power generation and emerging decentralized, sustainable models. They embody a proven technology with modern flexibility—capable of integrating with renewable resources, improving industrial energy efficiency, and supporting resilient, low-emission energy networks. Their adaptability, durability, and efficiency ensure they will remain a critical part of the energy landscape for decades to come, not only as mechanical workhorses but as key contributors to a smarter, cleaner, and more efficient energy future.
Steam turbines in Combined Heat and Power (CHP) systems also contribute significantly to grid stability and energy resilience, particularly as electrical grids worldwide face increasing complexity due to the integration of renewable energy sources like wind and solar. Unlike intermittent renewables, steam turbine CHP units can provide consistent, controllable power output, offering what is often referred to as “dispatchable” generation. This means operators can adjust their output quickly in response to fluctuations in demand or supply elsewhere on the grid, helping to balance load and maintain frequency and voltage stability. In many ways, CHP steam turbines act as reliable anchors within the evolving energy ecosystem, supporting the integration of variable renewables by smoothing out peaks and valleys in power supply.
Furthermore, the ability to simultaneously produce heat and power in CHP systems dramatically improves fuel utilization, lowering overall greenhouse gas emissions per unit of useful energy compared to separate heat and power production. This efficiency gain is particularly critical in sectors with significant thermal demand, such as manufacturing, food processing, and district heating. For example, a paper mill with a steam turbine CHP plant can generate electricity to power its operations while simultaneously using extracted steam for drying paper, thus maximizing the energy derived from the fuel source. This dual-use approach drastically reduces fuel consumption and carbon emissions, supporting industrial decarbonization goals and compliance with increasingly stringent environmental regulations.
In addition to industrial applications, CHP steam turbines are increasingly being adopted in institutional settings like hospitals, universities, and large commercial buildings, where reliable heating and power are essential. These facilities benefit from CHP’s inherent efficiency and reliability, reducing operational costs and enhancing energy security. For hospitals, where power outages can have critical consequences, the local generation capacity offered by steam turbine CHP units ensures continuous operation of life-saving equipment, heating systems, and sterilization processes. Universities and campuses often use district heating powered by CHP plants to maintain comfortable environments across multiple buildings while minimizing their carbon footprint.
The integration of modern digital control and monitoring technologies within steam turbine CHP systems further enhances their operational performance and flexibility. Advanced sensors and control algorithms enable real-time optimization of steam extraction, turbine speed, and load distribution to match fluctuating thermal and electrical demands. Predictive maintenance tools analyze equipment condition and performance trends, allowing operators to schedule maintenance proactively, reducing downtime and extending equipment life. This digital transformation also facilitates remote monitoring and diagnostics, enabling expert support and troubleshooting without the need for on-site visits, which can be particularly valuable in geographically dispersed or difficult-to-access installations.
Looking ahead, as the push for sustainability intensifies, steam turbine CHP systems are expected to evolve in tandem with developments in alternative fuels and hybrid energy systems. The growing availability of green hydrogen, biogas, and synthetic fuels offers new pathways to reduce the carbon footprint of steam generation. Steam turbines designed or retrofitted to operate with these cleaner fuels will play a crucial role in maintaining high efficiency while supporting a transition away from fossil fuels. Hybrid systems combining steam turbines with battery storage, solar thermal, or waste heat recovery technologies are also gaining traction, offering even greater flexibility and efficiency.
In sum, steam turbines remain a cornerstone technology in the energy landscape, particularly within Combined Heat and Power systems where their ability to convert steam into both electricity and useful heat drives efficiency and sustainability. Their adaptability to diverse fuels, integration with digital controls, and compatibility with emerging renewable energy paradigms position them well for continued relevance in a decarbonizing world. As industries and communities strive for cleaner, more resilient energy solutions, steam turbine CHP systems stand out as proven, versatile tools capable of meeting complex and evolving energy needs efficiently and reliably.
As steam turbine technology advances, ongoing research focuses on improving efficiency, reducing emissions, and expanding fuel flexibility to meet future energy challenges. One area of intense development is the enhancement of turbine blade design and materials to tolerate higher steam temperatures and pressures. Higher steam parameters translate directly into better thermodynamic efficiency, allowing CHP systems to extract more energy from the same amount of fuel. Novel alloys, thermal barrier coatings, and advanced manufacturing techniques such as additive manufacturing enable the creation of blades that maintain strength and resist corrosion and creep under increasingly harsh operating conditions. These improvements contribute not only to performance gains but also to longer component lifespans and reduced maintenance intervals, which are critical for CHP plants operating continuously or on variable load profiles.
Simultaneously, there is a growing emphasis on improving the integration of steam turbines with other components of CHP plants and wider energy systems. For instance, coupling steam turbines with organic Rankine cycle (ORC) units allows the recovery of lower-grade heat that traditional steam cycles cannot efficiently utilize. This hybridization expands the operational envelope of CHP systems, enabling even greater overall fuel utilization and providing additional revenue streams through electricity sales or heat delivery. Furthermore, developments in steam turbine control systems—leveraging artificial intelligence and machine learning—facilitate adaptive optimization that continuously fine-tunes operational parameters based on real-time data, ensuring maximum efficiency and reliability even under fluctuating demands or changing fuel qualities.
Environmental considerations remain paramount, driving innovations to minimize water consumption and thermal pollution associated with steam turbine CHP plants. Closed-loop cooling systems, dry cooling technologies, and advanced condensers reduce the environmental footprint and make steam turbines suitable for deployment in water-scarce regions. Additionally, integrating carbon capture technologies with CHP plants is an area of active research, aiming to combine high-efficiency power and heat generation with significant reductions in greenhouse gas emissions. While these systems are complex and capital-intensive, progress in solvent development, membrane technologies, and process integration holds promise for making carbon capture economically viable in CHP applications.
Another promising frontier is the adaptation of steam turbines for emerging energy vectors like green hydrogen. Hydrogen combustion can produce high-temperature steam with minimal emissions, and steam turbines capable of operating efficiently on hydrogen-rich steam mixtures could become integral to future low-carbon energy systems. The design challenges here include managing combustion dynamics, material compatibility, and maintaining performance across variable fuel compositions, but successful implementation would position steam turbines at the heart of a hydrogen economy, complementing their traditional roles.
In the context of urban energy systems, steam turbine CHP plants are increasingly integrated into smart grids and energy communities, where coordinated management of distributed energy resources optimizes local energy generation, consumption, and storage. This integration enables dynamic responses to grid signals, price fluctuations, and renewable generation variability, turning steam turbine CHP units into flexible assets that support grid resilience and decarbonization. Advanced energy management systems allow operators to prioritize thermal or electrical output depending on market conditions or demand profiles, maximizing economic and environmental benefits.
Educationally and operationally, the future of steam turbine CHP technology will depend heavily on workforce development and knowledge sharing. Training programs that combine traditional mechanical and thermodynamic expertise with digital skills and sustainability principles are critical to prepare engineers and technicians for the evolving landscape. Collaborative research initiatives, industry partnerships, and international standards development will further accelerate the adoption of best practices and technological innovations.
Ultimately, steam turbines in CHP systems continue to embody a mature yet dynamic technology platform. They represent a bridge between conventional energy paradigms and emerging sustainable solutions, balancing efficiency, reliability, and environmental performance. Their ongoing evolution—driven by material science, digitalization, environmental imperatives, and fuel diversification—ensures that steam turbine CHP systems will remain vital contributors to global energy systems, supporting the transition toward a cleaner, smarter, and more resilient energy future.
Steam Turbine Performance Optimization
Optimizing the performance of steam turbines is essential for maximizing efficiency, reliability, and cost-effectiveness in power generation and industrial applications. Steam turbine performance optimization involves a combination of design improvements, operational strategies, maintenance practices, and control technologies aimed at extracting the maximum useful energy from steam while minimizing losses and wear.
One of the fundamental factors in performance optimization is maintaining optimal steam conditions—pressure, temperature, and quality—at the turbine inlet. High-quality steam with minimal moisture content reduces blade erosion and corrosion, which can degrade turbine efficiency over time. Modern steam turbines are designed to operate with superheated steam at high pressure and temperature to achieve better thermodynamic efficiency, but these conditions must be carefully controlled to avoid material stress and premature failure.
Efficient aerodynamic design of turbine blades and stages is another critical area. Advances in computational fluid dynamics (CFD) allow engineers to model steam flow more accurately, identifying areas where flow separation, turbulence, or shock losses occur. Optimized blade profiles, improved sealing technologies, and the use of variable geometry nozzles help maintain ideal steam expansion and minimize energy losses. Regular inspection and refurbishment of blades to address erosion, deposits, or surface damage also ensure sustained aerodynamic performance.
Thermal and mechanical balancing of the turbine components is vital to reduce vibrations and mechanical stresses that can compromise reliability and efficiency. Precise alignment of shafts, bearings, and seals reduces friction and wear, which directly affect output power and operational longevity. Condition monitoring systems employing vibration analysis, temperature sensors, and oil quality measurements help detect early signs of imbalance or degradation, enabling proactive maintenance.
Control system optimization plays a significant role in performance enhancement. Modern steam turbines utilize advanced control algorithms to adjust steam flow, pressure, and extraction points dynamically in response to changing load demands or process requirements. This flexibility ensures that the turbine operates near its optimal efficiency point regardless of fluctuations in demand, fuel quality, or ambient conditions. Load-following capabilities also improve the integration of steam turbines into combined heat and power (CHP) plants or grids with variable renewable energy sources.
Minimizing steam leakage through improved sealing technology—such as labyrinth seals, brush seals, and carbon rings—reduces losses and helps maintain designed steam flow rates and pressures. Additionally, maintaining proper condenser vacuum levels and cooling water temperatures is crucial since backpressure affects turbine efficiency. Optimizing condenser performance ensures effective condensation of exhaust steam, enabling the turbine to extract maximum work.
Operational practices, including startup and shutdown procedures, significantly impact turbine performance and lifespan. Gradual ramp-up and cooldown cycles prevent thermal shock to turbine components, reducing the risk of cracking or distortion. Training operators on best practices and implementing automated control sequences help standardize these processes.
Regular maintenance and overhaul schedules based on predictive analytics extend turbine life and maintain high efficiency. Condition-based maintenance uses real-time data and historical trends to identify when components need servicing or replacement, avoiding unnecessary downtime and costly failures.
Finally, retrofitting existing turbines with modern components or control systems can improve performance without requiring full replacement. Upgrades might include more efficient blades, advanced coatings, improved seals, and state-of-the-art digital control platforms, all contributing to higher efficiency, better reliability, and reduced emissions.
In summary, optimizing steam turbine performance is a multidisciplinary effort encompassing design, operation, monitoring, and maintenance. By focusing on maintaining ideal steam conditions, refining aerodynamic and mechanical components, implementing intelligent control systems, and adhering to rigorous maintenance protocols, operators can significantly enhance turbine efficiency, extend equipment life, and reduce operational costs—ultimately delivering more sustainable and economical energy production.
Improving steam turbine performance further involves continuous monitoring and data analysis to detect subtle inefficiencies and emerging faults. The use of condition monitoring systems equipped with sensors measuring temperature, pressure, vibration, and acoustic emissions allows operators to gain detailed insights into turbine health and performance in real time. These systems often incorporate machine learning algorithms that analyze patterns in the data to predict issues before they escalate, enabling proactive maintenance and avoiding unexpected downtime. This predictive maintenance approach not only improves reliability but also optimizes maintenance schedules, reducing costs and extending the turbine’s operational life.
Another important factor in performance optimization is the effective management of steam parameters through the entire turbine cycle. This includes careful control of steam extraction for process heat or reheating, which must be balanced against the need to maximize electrical output. Reheat cycles, where steam is expanded partially, reheated, and then expanded again, improve efficiency by reducing moisture content in the later stages of the turbine and increasing average steam temperature throughout expansion. Optimizing the reheating process and timing steam extractions can significantly enhance overall cycle efficiency, but requires precise control and coordination between the turbine and steam supply systems.
Fuel quality and combustion control upstream of the turbine also impact performance. In CHP plants, ensuring consistent fuel quality and stable boiler operation is crucial to delivering steam at optimal conditions. Variations in fuel composition or combustion efficiency can lead to fluctuations in steam pressure and temperature, reducing turbine efficiency and increasing wear. Advanced boiler controls, combustion monitoring, and fuel treatment systems help maintain steady steam generation, contributing to stable turbine operation.
Environmental conditions such as ambient temperature and humidity can influence turbine performance, especially in open-loop cooling systems or facilities exposed to variable climates. Cooling water temperature affects condenser vacuum and thus the turbine’s backpressure. Maintaining consistent cooling conditions through improved heat exchangers, cooling towers, or alternative cooling technologies helps sustain high efficiency. Some plants use supplementary cooling methods or hybrid cooling systems to mitigate performance losses during hot or dry periods.
In terms of operational strategy, load management plays a key role. Steam turbines perform best when operated near their design load. Running turbines continuously at partial loads can reduce efficiency and increase mechanical stress. Therefore, careful planning of load distribution, integration with other energy sources, and demand-side management can help maintain turbine operation within optimal ranges. In systems with multiple turbines or parallel generation units, load sharing and coordination ensure each unit operates efficiently, reducing fuel consumption and emissions.
Retrofits and upgrades also contribute significantly to performance optimization. Older steam turbines can benefit from modernization programs that replace outdated components with newer, more efficient parts. Blade redesigns, improved sealing systems, enhanced bearings, and upgraded control hardware and software can collectively boost efficiency and reliability. Such modernization can be more cost-effective than complete turbine replacement, offering a favorable return on investment through fuel savings and reduced maintenance costs.
Lastly, training and skill development for plant operators and maintenance personnel are vital. Well-trained staff can identify operational anomalies early, execute proper startup and shutdown procedures, and maintain equipment according to best practices. Continuous education on evolving technologies, control systems, and diagnostic tools empowers teams to optimize turbine performance consistently.
In essence, steam turbine performance optimization is a dynamic, ongoing process that combines technology, operational expertise, and data-driven decision-making. By focusing on maintaining ideal steam conditions, leveraging advanced monitoring and control, managing fuel and environmental factors, and investing in upgrades and training, operators can achieve higher efficiency, lower costs, and longer equipment life—ultimately enhancing the sustainability and profitability of steam turbine applications across industries.
Advancements in digitalization and Industry 4.0 technologies are playing an increasingly pivotal role in steam turbine performance optimization. The integration of Internet of Things (IoT) devices, cloud computing, and big data analytics allows for unprecedented levels of data collection, processing, and actionable insights. Sensors embedded throughout the turbine system continuously gather vast amounts of operational data—covering parameters such as rotor speed, steam temperature and pressure, vibration levels, and lubricant condition. This data is transmitted in real time to centralized platforms where sophisticated algorithms analyze it to detect patterns, anomalies, or deviations from optimal performance benchmarks. Operators and maintenance teams can receive alerts or detailed reports, enabling faster decision-making and timely interventions that minimize performance degradation or avoid catastrophic failures.
The application of machine learning and artificial intelligence (AI) models further enhances predictive maintenance and optimization capabilities. These models learn from historical turbine operation and maintenance records to predict future component behavior, failure probabilities, and efficiency trends. By simulating various operational scenarios, AI-driven systems can recommend optimal operating parameters, schedule maintenance activities proactively, and even assist in diagnosing complex problems that might be difficult for human operators to detect early. Over time, as these systems accumulate more data, their predictive accuracy improves, leading to progressively better turbine performance and reliability.
In parallel, digital twin technology is gaining traction in steam turbine optimization. A digital twin is a virtual replica of the physical turbine, reflecting its real-time status and operational environment. This digital model can simulate turbine responses under different operating conditions, helping engineers test control strategies, evaluate the impact of potential modifications, and optimize performance without risking damage to the actual equipment. Digital twins enable “what-if” analyses that support strategic decision-making in design upgrades, operational changes, and maintenance planning. They also facilitate remote troubleshooting by providing detailed diagnostics and visualizations that experts can access from anywhere, accelerating problem resolution.
Furthermore, the integration of steam turbines into smart grid architectures offers new avenues for performance optimization. Smart grids utilize real-time communication between generation assets, energy storage, and consumers to balance supply and demand dynamically. Steam turbine CHP plants connected to smart grids can adjust their output to match grid needs, participate in demand response programs, and optimize fuel usage based on electricity market prices or renewable generation forecasts. This dynamic operation requires advanced control systems capable of rapid response and fine-tuned steam management, ensuring turbines operate efficiently while contributing to grid stability and resilience.
Energy storage technologies, such as thermal energy storage or hybridization with batteries, are also enhancing the flexibility and efficiency of steam turbine systems. Thermal storage allows excess steam or heat to be stored during low-demand periods and used later when demand spikes, smoothing turbine operation and reducing the need for frequent startups and shutdowns, which can wear components and reduce efficiency. Battery storage complements this by storing excess electricity generated during low-load conditions for later use, further stabilizing output and improving overall system efficiency.
Sustainability considerations continue to influence turbine performance strategies. Operators increasingly monitor emissions and fuel consumption alongside traditional performance metrics, striving to minimize the environmental footprint. Advanced combustion controls, fuel switching capabilities, and integration with renewable or low-carbon fuels are part of this trend, requiring adaptive turbine operation to maintain efficiency across varying fuel types. Additionally, waste heat recovery and utilization for industrial processes or district heating improve overall system efficiency and reduce fuel consumption, making steam turbines central to circular economy approaches in energy management.
Finally, regulatory frameworks and market incentives shape how steam turbines are optimized. Policies promoting energy efficiency, emissions reductions, and renewable integration encourage operators to adopt best practices and invest in modernization technologies. Incentives such as carbon credits, efficiency standards, and financial support for CHP projects make optimization efforts economically attractive. Compliance with safety and environmental regulations also ensures that performance improvements do not come at the expense of reliability or operational safety.
In conclusion, steam turbine performance optimization is evolving rapidly, driven by technological innovation, digital transformation, environmental imperatives, and market dynamics. The fusion of advanced materials, smart control systems, predictive analytics, and integrated energy management positions steam turbines as flexible, efficient, and sustainable assets in modern energy systems. This ongoing evolution not only enhances the operational and economic viability of steam turbines but also reinforces their critical role in the transition toward cleaner, more resilient, and smarter energy infrastructures worldwide.
Common Steam Turbine Configurations Explained
Steam turbines come in several common configurations, each designed to meet specific operational needs and application requirements. Understanding these configurations helps in selecting the right turbine type for a given power generation or industrial process. The main types include impulse turbines, reaction turbines, condensing turbines, back-pressure turbines, extraction turbines, and combination turbines.
Impulse turbines operate on the principle that high-velocity steam jets are directed onto curved blades mounted on a rotor. The kinetic energy of the steam jet causes the rotor to spin, converting thermal energy into mechanical work. The steam pressure drops entirely in the nozzles before hitting the blades, so the blades experience only velocity change, not pressure drop. These turbines are often used in high-pressure applications and are suitable for handling varying steam conditions.
Reaction turbines, on the other hand, rely on a combination of pressure and velocity changes within the turbine blades. Both fixed and moving blades form nozzles, and the steam expands as it passes through the blades, generating a reactive force that spins the rotor. Because the pressure drop happens across both stationary and rotating blades, reaction turbines require a continuous steam flow and are commonly used in low- to medium-pressure stages of multi-stage turbines. They are favored for their smooth operation and high efficiency in partial load conditions.
Condensing turbines are designed to exhaust steam into a condenser where it is converted back to water under a vacuum. This configuration maximizes energy extraction from steam by creating a low-pressure sink at the exhaust, enabling the turbine to extract more work from the steam expansion. Condensing turbines are commonly used in electricity generation plants where maximizing electrical output is critical. They operate with high inlet steam pressures and temperatures, producing significant power in large-scale applications.
Back-pressure turbines differ in that they exhaust steam at a higher pressure rather than into a condenser. The exhaust steam is typically used for industrial heating or process applications. This type of turbine is common in Combined Heat and Power (CHP) plants, where the thermal energy in the exhaust steam is valuable for heating or manufacturing processes. Back-pressure turbines are less focused on maximizing electrical output and more on providing usable steam at a controlled pressure for downstream applications.
Extraction turbines incorporate one or more steam extraction points along the turbine stages. Steam can be withdrawn partially at these points for process heating or feedwater heating while the remainder continues expanding to generate power. This flexibility makes extraction turbines ideal for industrial plants that require both electricity and steam at different pressures and temperatures. Proper design and control of extraction points optimize the balance between electrical generation and thermal energy delivery.
Combination turbines blend features of impulse and reaction turbines or combine condensing and back-pressure sections within a single unit. For example, a turbine may start with a high-pressure impulse section followed by a low-pressure reaction section. Or, a turbine may have a back-pressure stage for process steam extraction combined with a condensing stage for maximum electrical output. These hybrid designs provide versatility and efficiency, meeting complex operational demands in modern power plants and industrial facilities.
In summary, steam turbine configurations vary to match specific steam conditions, load requirements, and end-use objectives. Impulse turbines excel in high-pressure environments, reaction turbines provide smooth partial-load operation, condensing turbines maximize power output, back-pressure turbines support process steam needs, extraction turbines offer combined heat and power capabilities, and combination turbines deliver tailored performance for complex applications. Selecting the right configuration is crucial for optimizing efficiency, reliability, and overall system integration.
Beyond the basic configurations, steam turbines often incorporate multiple stages to gradually extract energy from steam as it expands and loses pressure and temperature throughout the turbine. Multi-stage turbines combine high-pressure, intermediate-pressure, and low-pressure sections, each optimized for specific steam conditions to maximize overall efficiency. High-pressure stages typically use impulse or reaction designs to handle the initial high-energy steam, while low-pressure stages are designed to accommodate larger steam volumes at lower pressures. The staged approach allows better control over steam expansion and reduces mechanical stresses on turbine components by spreading the work across multiple sections.
In many modern power plants and industrial settings, turbines are equipped with reheaters that take partially expanded steam from the high-pressure section, reheat it in the boiler, and return it to an intermediate or low-pressure stage. This reheating cycle increases the average steam temperature during expansion, improving thermal efficiency and reducing moisture content in the later stages of the turbine, which protects blades from erosion and corrosion. The design and control of reheating and multi-stage expansion require precise coordination to maintain optimal temperature and pressure profiles throughout the turbine, ensuring longevity and performance.
Another important aspect in turbine configurations is the inclusion of steam extraction points for feedwater heating in regenerative cycles. Extraction of steam at various intermediate stages is used to preheat the boiler feedwater before it enters the steam generator, improving cycle efficiency by reducing the fuel needed to produce steam. Regenerative feedwater heating cycles are a common feature in large thermal power plants and significantly enhance overall plant efficiency. The design of extraction turbines must balance the amount of steam extracted for feedwater heating against the steam available for power generation, requiring careful thermodynamic analysis.
Seal arrangements and shaft configurations also vary among steam turbine designs to accommodate different operational requirements. Some turbines use single or double casing designs, where steam flows through one or two separate casings arranged in series or parallel to optimize flow paths and pressure management. Tandem compound turbines connect multiple turbine sections on a single shaft, transmitting mechanical power efficiently to the generator or driven equipment. Cross-compound arrangements use separate shafts for different turbine sections, often with reduction gearing, offering flexibility in matching turbine speeds to generator requirements or allowing partial operation of sections for variable load scenarios.
The choice of materials and cooling methods in turbine configurations plays a crucial role in performance and durability. High-temperature sections of the turbine utilize advanced alloys and thermal barrier coatings to withstand steam temperatures that can exceed 600°C (1112°F). Blade cooling techniques, such as internal air cooling or steam cooling passages, are employed in some advanced turbines to manage thermal stresses and prevent material degradation. These engineering solutions enable turbines to operate at higher steam parameters, boosting efficiency but requiring complex design and manufacturing processes.
Maintenance accessibility and modularity are also considered in turbine configurations. Modular designs facilitate easier inspection, repair, and component replacement, reducing downtime during overhauls. Turbines designed with removable blade rows or split casings enable targeted maintenance without disassembling the entire unit, which is particularly beneficial in large-scale power plants with tight operational schedules. Advances in monitoring technology further support maintenance planning by providing real-time insights into component conditions.
Emerging trends in steam turbine configurations include the integration of hybrid cycles and combined technologies. For example, some plants incorporate steam turbines alongside gas turbines in combined cycle configurations, where exhaust gases from the gas turbine generate steam for the steam turbine, substantially increasing overall plant efficiency. Innovations also explore the use of organic Rankine cycles in conjunction with steam turbines to recover lower-grade heat. These hybrid configurations reflect the drive toward maximizing energy extraction from fuel and waste heat sources.
Customization of steam turbine configurations to specific industrial processes is common. For instance, pulp and paper mills, chemical plants, and refineries often require steam at various pressures and temperatures for their processes, influencing turbine design to accommodate multiple extraction points and tailored exhaust pressures. Such bespoke configurations ensure that steam turbines provide not only mechanical power but also valuable thermal energy in the form of process steam, optimizing resource utilization and cost-efficiency.
In conclusion, steam turbine configurations encompass a wide range of designs and features to meet diverse operational needs, from simple single-stage units to complex multi-stage, reheated, regenerative, and hybrid systems. Understanding these configurations and their implications for efficiency, flexibility, maintenance, and integration is essential for engineers, operators, and decision-makers seeking to harness steam turbine technology effectively. By selecting and tailoring the right configuration, facilities can achieve optimal performance, reliability, and economic benefits across power generation and industrial applications.
Looking ahead, steam turbine configurations continue to evolve in response to changing energy landscapes, technological advances, and environmental regulations. One key trend is the increasing emphasis on flexibility and adaptability to accommodate variable renewable energy sources like wind and solar. As grids integrate higher shares of intermittent renewables, steam turbines are expected to operate more frequently under partial load and cycling conditions, rather than steady, baseload operation. This shift demands configurations that can handle frequent startups, shutdowns, and load changes without compromising efficiency or reliability. Innovations such as advanced control systems, flexible blade designs, and robust materials are being integrated into turbine configurations to meet these dynamic operating profiles.
Additionally, the drive toward decarbonization is influencing steam turbine configurations through the adoption of low-carbon fuels and integration with carbon capture technologies. Many new turbine designs consider compatibility with hydrogen-enriched fuels or synthetic fuels produced from renewable sources. This requires modifications in materials, sealing systems, and combustion processes upstream of the turbine to accommodate different chemical properties and combustion characteristics while maintaining optimal steam conditions. Integration with carbon capture, utilization, and storage (CCUS) systems also affects turbine configurations by altering steam cycle parameters and adding components like steam regenerators or heat exchangers, necessitating re-optimization of the turbine layout.
The expansion of small and modular steam turbines represents another evolving configuration trend. Smaller-scale turbines designed for distributed generation, industrial facilities, or remote locations emphasize simplicity, compactness, and rapid deployment. These units often feature simplified single-stage or few-stage designs, modular construction for easy transportation and installation, and enhanced automation for minimal operator intervention. Although their individual capacity is lower than large utility turbines, collectively these small turbines contribute to resilient, decentralized energy systems that complement large centralized plants.
Emerging digital technologies such as augmented reality (AR) and virtual reality (VR) are also impacting how turbine configurations are designed, assembled, and maintained. AR and VR tools enable engineers and technicians to visualize complex turbine assemblies in 3D during design or maintenance activities, improving accuracy and reducing errors. Digital collaboration platforms facilitate remote expert support for troubleshooting or retrofitting turbines, making specialized knowledge accessible globally. This virtual interaction enhances the ability to optimize turbine configurations in real time and adapt them to specific site conditions or operational demands.
Sustainability is further driving the exploration of eco-friendly turbine components and manufacturing methods. Efforts to reduce the carbon footprint of turbine production include the use of recycled materials, additive manufacturing (3D printing) for complex parts, and eco-efficient coatings that extend component life while minimizing environmental impact. These manufacturing innovations allow for lighter, stronger turbine parts that support higher efficiency and reduce maintenance frequency, feeding back into improved turbine configurations that are both high-performance and sustainable.
Lastly, international standards and collaboration among turbine manufacturers, research institutions, and industry bodies continue to shape the evolution of steam turbine configurations. Harmonizing design codes, testing protocols, and performance benchmarks ensures that turbines meet safety, reliability, and environmental criteria across global markets. Collaborative research fosters innovation in blade aerodynamics, sealing technology, and thermal management, which feeds into future turbine configurations optimized for emerging challenges and opportunities.
In summary, steam turbine configurations are becoming more versatile, intelligent, and environmentally conscious. The convergence of digital transformation, flexible operation, sustainable practices, and global collaboration is driving the next generation of steam turbines. These advances promise to enhance the role of steam turbines in a low-carbon, decentralized, and digitally connected energy future, maintaining their relevance and value in a rapidly changing world.
The Evolution of Steam Turbine Technology
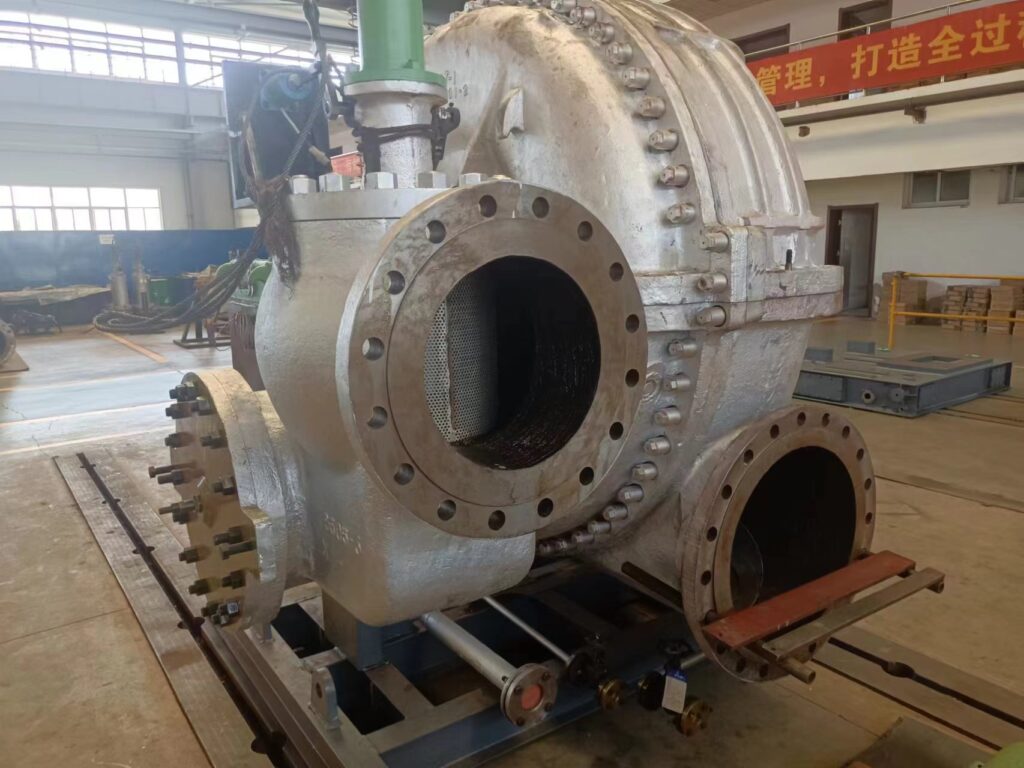
The evolution of steam turbine technology is a remarkable journey that reflects centuries of scientific discovery, engineering innovation, and industrial advancement. From the earliest experiments with steam power to the sophisticated turbines operating in today’s power plants, this evolution illustrates humanity’s quest to harness thermal energy efficiently and reliably.
The roots of steam turbine technology trace back to the late 17th and early 18th centuries, when inventors like Thomas Savery and Thomas Newcomen developed early steam engines that converted steam pressure into mechanical motion. These early engines were large, inefficient, and primarily used for pumping water from mines. However, they laid the foundation for understanding steam’s potential as a power source. The breakthrough came in the late 19th century when Sir Charles Parsons invented the modern steam turbine in 1884. Parsons’ design introduced a rotor with multiple blades that could efficiently convert high-velocity steam jets into rotational energy, revolutionizing power generation with unprecedented speed and smoothness compared to reciprocating steam engines.
Following Parsons’ innovation, steam turbine technology rapidly advanced throughout the 20th century, driven by growing industrial demand for electricity and mechanical power. Improvements in materials science allowed turbines to withstand higher steam pressures and temperatures, leading to significant gains in efficiency. The development of multi-stage turbines, combining impulse and reaction stages, optimized energy extraction across a wide range of steam conditions. Reheat cycles and regenerative feedwater heating further enhanced thermal efficiency, allowing power plants to produce more electricity from the same amount of fuel.
World wars and the subsequent industrial boom accelerated turbine development, particularly for naval propulsion and large-scale electricity generation. Steam turbines became integral to naval ships, providing reliable and powerful propulsion systems. In power plants, turbine designs adapted to various fuels and scales, from small industrial units to massive utility-scale generators producing hundreds of megawatts. Advances in precision manufacturing and balancing techniques improved turbine reliability and reduced maintenance needs.
The late 20th and early 21st centuries saw the integration of digital control systems and monitoring technologies into steam turbine operations. Automated control improved turbine responsiveness and safety, while sensors and diagnostic tools enabled predictive maintenance and optimized performance. Concurrently, environmental concerns and fuel diversification influenced turbine designs to accommodate cleaner fuels, lower emissions, and integration with renewable energy sources.
Today, steam turbine technology continues to evolve with a focus on sustainability, flexibility, and digitalization. Modern turbines are designed to operate efficiently under variable loads and in combined heat and power (CHP) configurations, maximizing energy utilization and reducing waste. Research into advanced materials, blade cooling techniques, and additive manufacturing aims to push the boundaries of steam temperature and pressure limits, further improving efficiency and durability. Digital twins and AI-driven analytics provide real-time insights, enhancing operational decision-making and maintenance strategies.
Emerging trends also include the development of small modular turbines for decentralized energy production, hybrid cycles combining steam with gas or organic Rankine cycles, and adaptations for low-carbon fuels such as hydrogen blends. These innovations reflect a broader energy transition toward cleaner, more resilient power systems.
In summary, the evolution of steam turbine technology is a story of continuous refinement and adaptation. From humble beginnings as simple steam engines to today’s high-tech turbines, this technology has been central to industrialization and energy generation worldwide. Its ongoing evolution ensures that steam turbines remain a vital component of modern energy infrastructure, capable of meeting future challenges in efficiency, environmental responsibility, and operational flexibility.
As steam turbine technology progressed, the focus increasingly shifted toward improving thermal efficiency, reliability, and adaptability to diverse energy needs. Early turbines operated at relatively low steam pressures and temperatures, limiting their efficiency and power output. However, advancements in metallurgy and materials engineering enabled turbines to withstand much higher steam conditions. The introduction of alloys capable of tolerating elevated temperatures without significant creep or corrosion allowed engineers to push steam temperatures beyond 600°C and pressures well above 200 bar. This leap in operational parameters significantly enhanced the thermodynamic efficiency of power cycles, reducing fuel consumption and emissions per unit of electricity generated.
The development of multi-stage turbines also played a crucial role in the evolution of steam turbine technology. Instead of relying on a single expansion stage, engineers designed turbines with numerous sets of blades arranged in high-, intermediate-, and low-pressure sections. This approach enabled a more gradual and efficient conversion of steam’s energy, minimizing losses and mechanical stress on components. Each stage extracted energy from the steam as it expanded and cooled, and the design was tailored to optimize blade geometry, steam flow rates, and pressure drops at every stage. The use of computational fluid dynamics (CFD) and advanced modeling tools in recent decades has refined blade profiles and flow paths, further boosting efficiency and reducing vibration and fatigue issues.
In parallel, the incorporation of reheat cycles became a hallmark of modern steam turbine design. By routing partially expanded steam back to the boiler for reheating before continuing expansion in lower-pressure turbine stages, power plants could maintain higher average steam temperatures during expansion, thus improving efficiency and reducing moisture content in the later stages. Moisture can erode turbine blades and reduce mechanical reliability, so reheating not only improves energy extraction but also extends turbine life. This innovation required precise coordination between boiler operation, steam piping, and turbine controls to maintain optimal temperature and pressure conditions throughout the cycle.
Regenerative feedwater heating, achieved through the use of steam extraction points at various turbine stages, further advanced the evolution of steam turbines. By extracting steam at intermediate pressures to preheat the feedwater entering the boiler, power plants could reduce the energy needed to convert water into steam, improving overall cycle efficiency. This technique has become standard in large-scale thermal power plants and involves intricate balancing to maximize the benefits without compromising turbine output.
Another significant evolutionary milestone was the integration of advanced control and monitoring systems. Early turbines relied on manual control and limited instrumentation, but modern units are equipped with sophisticated digital control systems that continuously adjust turbine parameters in real time to optimize performance and safety. Sensors monitor vibrations, temperatures, pressures, and rotational speeds, enabling predictive maintenance that minimizes unplanned outages and extends turbine life. The use of condition-based monitoring and artificial intelligence algorithms allows operators to detect early signs of wear, imbalance, or other faults, ensuring timely interventions before failures occur.
Materials science continued to drive innovation, especially with the introduction of thermal barrier coatings and internal cooling techniques for turbine blades. These technologies protect critical components from extreme thermal and mechanical stresses, allowing turbines to operate at ever-higher steam temperatures. Advanced manufacturing methods such as precision casting, forging, and additive manufacturing have enabled the production of complex blade geometries with improved aerodynamic performance and structural integrity. These developments support higher efficiencies and longer maintenance intervals.
Environmental regulations and the global push for decarbonization have also influenced steam turbine evolution. Turbine designs now often incorporate features to handle alternative fuels such as biomass, synthetic fuels, or hydrogen blends, which have different combustion and steam characteristics compared to traditional fossil fuels. Moreover, steam turbines are increasingly integrated into combined heat and power (CHP) systems and combined cycle plants, maximizing the utilization of thermal energy and reducing greenhouse gas emissions. The flexibility of modern turbines to ramp quickly and operate efficiently under varying loads supports the integration of intermittent renewable energy sources, contributing to more resilient and sustainable power grids.
Looking to the future, steam turbine technology is poised to benefit from ongoing research in materials science, digitalization, and hybrid energy systems. Efforts to develop ultra-supercritical steam cycles with steam temperatures exceeding 700°C aim to push efficiency boundaries further. Integration with digital twins—virtual replicas of turbines that simulate performance and predict maintenance needs in real time—promises to optimize operations and reduce lifecycle costs. Hybrid configurations that combine steam turbines with emerging energy technologies, such as fuel cells or concentrated solar power, may open new pathways for low-carbon power generation.
In summary, the evolution of steam turbine technology has been characterized by continuous improvements in materials, design, control systems, and operational flexibility. These advancements have transformed steam turbines from rudimentary engines into highly efficient, reliable, and adaptable machines central to modern power generation and industrial processes. The ongoing innovation ensures that steam turbines will continue to play a vital role in the global energy landscape, supporting the transition toward cleaner, smarter, and more sustainable energy systems.
As steam turbine technology advances, greater emphasis is being placed on sustainability and environmental impact reduction. One of the major challenges has been addressing the carbon emissions associated with conventional fossil-fueled steam power plants. To this end, the evolution of steam turbines is closely linked with efforts to integrate carbon capture and storage (CCS) technologies. Modern turbine designs are increasingly optimized to work with CCS systems, which capture CO₂ from flue gases before they are released into the atmosphere. These integrated systems often require turbines to operate efficiently with altered steam conditions or in conjunction with additional heat exchangers and absorbers, driving innovations in turbine thermodynamics and material resilience.
In addition, the rise of renewable energy sources has prompted the adaptation of steam turbines for hybrid and flexible operations. Steam turbines are now frequently paired with gas turbines in combined cycle power plants, where the high-temperature exhaust from gas turbines generates steam that drives a steam turbine, significantly boosting overall plant efficiency. These combined cycles can reach thermal efficiencies above 60%, far surpassing traditional steam-only plants. The flexibility to switch between base-load and peak-load operation also allows steam turbines to complement variable renewable generation such as wind and solar, maintaining grid stability and reliability.
Another frontier in steam turbine evolution involves the use of alternative fuels like biomass and hydrogen. Biomass-fired boilers produce steam that can drive conventional steam turbines, enabling renewable and carbon-neutral power generation when sustainably sourced. Hydrogen, with its high energy content and clean combustion, is gaining attention as a future fuel, especially when produced via electrolysis using renewable electricity. However, burning hydrogen or hydrogen blends can create different steam conditions and combustion dynamics, requiring turbines with specially designed components and seals to handle changes in steam chemistry and reduce risks of material degradation.
Digital transformation is also revolutionizing steam turbine design, operation, and maintenance. Advanced sensors, Internet of Things (IoT) devices, and big data analytics enable operators to monitor turbines in real time, predict failures before they occur, and optimize performance dynamically. Digital twins create virtual models of turbines that simulate wear, thermal stresses, and performance under varying operating conditions, allowing engineers to fine-tune operations for maximum efficiency and longevity. This digital layer enhances the reliability and cost-effectiveness of turbines, making them more responsive to the evolving demands of modern power systems.
Furthermore, additive manufacturing techniques are beginning to impact turbine component production. 3D printing allows for the creation of complex blade geometries and internal cooling channels that were previously impossible or too costly to manufacture using traditional methods. These innovations improve aerodynamic efficiency, reduce weight, and enhance cooling effectiveness, enabling turbines to safely operate at higher temperatures and pressures, thereby pushing performance limits.
The modularization and downsizing of steam turbines for distributed generation and industrial applications also mark a significant shift. Smaller, modular turbines can be rapidly deployed in decentralized energy systems, providing reliable power and heat close to the point of use. This reduces transmission losses and supports microgrids and industrial symbiosis where waste heat and steam are recycled across multiple processes, enhancing overall energy efficiency and sustainability.
Looking forward, research into new materials such as ceramics and ultra-high-temperature alloys promises to further increase turbine operating temperatures and pressures, potentially revolutionizing efficiency. In parallel, innovations in blade coatings, sealing technologies, and lubrication systems aim to reduce wear, corrosion, and maintenance needs, ensuring turbines remain cost-effective and durable over longer service lives.
In conclusion, the evolution of steam turbine technology continues unabated, driven by the need for cleaner energy, higher efficiency, operational flexibility, and digital integration. As global energy systems shift toward decarbonization and smarter grids, steam turbines remain indispensable, adapting through innovation to meet the challenges and opportunities of the future energy landscape. Their legacy of transformation and resilience positions them as key enablers of sustainable and reliable power generation for decades to come.
Types of Steam Turbines: Impulse vs. Reaction
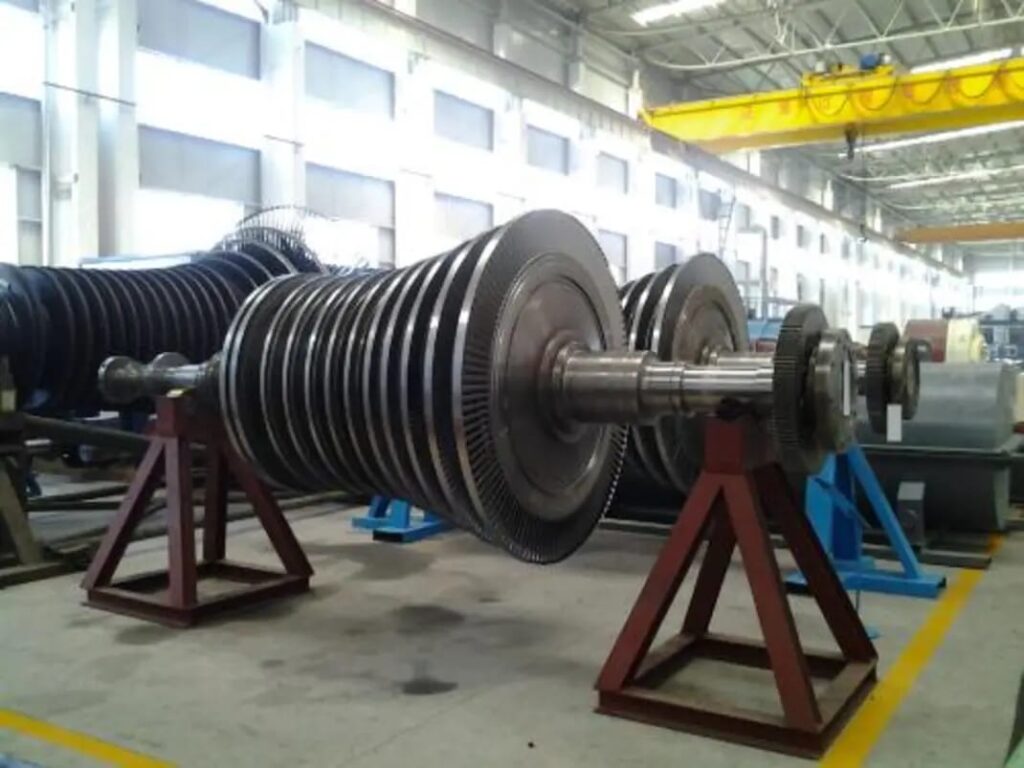
Steam turbines mainly fall into two fundamental types based on how they convert steam energy into mechanical work: impulse turbines and reaction turbines. Both types rely on high-pressure steam expanding through blades attached to a rotating shaft, but they differ significantly in their design principles and how the steam forces act on the blades.
Impulse turbines operate on the principle that high-velocity steam jets are directed at the turbine blades, which are shaped like buckets mounted on the rotor. In this design, the steam expands entirely in stationary nozzles before striking the blades, converting the steam’s pressure energy into kinetic energy. The moving blades then change the direction of the steam flow, and the impulse of the steam jet causes the rotor to turn. Since the steam pressure drops only in the fixed nozzles and remains constant while flowing over the moving blades, the blades mainly experience a change in velocity, which generates the torque needed to spin the turbine shaft. Impulse turbines typically feature robust blades that can handle the impact forces from the steam jets. Because the pressure drop happens outside the moving blades, the design simplifies blade construction and reduces stress caused by pressure gradients. They are well suited for high-speed operation and are commonly used in high-pressure stages of multi-stage turbines.
In contrast, reaction turbines derive their rotational force from both the impulse and reactive forces generated as steam expands continuously through both fixed and moving blades. In this design, the steam pressure drops gradually as it passes over the curved fixed guide vanes and then further expands across the moving blades attached to the rotor. The moving blades act as nozzles themselves, accelerating the steam and creating a reactive force that pushes the blades forward, similar to the recoil of a rocket engine. This continuous expansion means the blades experience pressure differences on their surfaces, resulting in a combined effect of pressure and velocity changes generating torque. Reaction turbines typically have blades with aerodynamic profiles designed to harness both forces efficiently. They tend to operate at lower speeds than impulse turbines and are common in the low-pressure stages of steam turbines where steam volume is higher but pressure is lower.
Both types of turbines are often combined in multi-stage steam turbines to maximize energy extraction. High-pressure stages typically use impulse turbines to handle the high-pressure, low-volume steam, while intermediate and low-pressure stages employ reaction turbines to extract energy from lower-pressure, higher-volume steam. This hybrid approach leverages the strengths of each type to optimize efficiency, reliability, and mechanical stress distribution.
Additionally, impulse turbines are favored for their simplicity and ability to handle high temperatures and pressures, making them suitable for initial expansion stages in power plants. Reaction turbines, with their smooth pressure gradients and aerodynamic blade design, excel in extracting energy from steam at later stages with larger volumes and lower pressure, contributing to higher overall turbine efficiency.
In summary, impulse turbines convert steam energy primarily through the impact of high-velocity jets on stationary blades, while reaction turbines generate rotation through continuous steam expansion and reactive forces on moving blades. Understanding these fundamental differences helps engineers design efficient steam turbines tailored to specific pressure and flow conditions, ultimately optimizing power generation and mechanical performance.
Both impulse and reaction turbines have their unique advantages and specific applications, which often leads to their combination within a single steam turbine unit to achieve optimal performance. Impulse turbines, due to their design where steam expansion occurs mostly in fixed nozzles, are mechanically simpler and easier to manufacture with fewer aerodynamic complexities. This makes them highly suitable for handling the initial high-pressure steam coming directly from the boiler. Their blades, often bucket-shaped, are designed to withstand the intense forces of steam jets, allowing the turbine to operate at very high rotational speeds with reduced risk of blade deformation or failure. The relatively small blade size and lower flow rates in impulse turbines mean they can be constructed more compactly for the high-pressure stages.
On the other hand, reaction turbines are characterized by a more gradual expansion of steam through both stationary and moving blades, which creates a continuous pressure drop and generates reactive forces that contribute to shaft rotation. This results in smoother operation and reduced vibration compared to impulse turbines. The blades in reaction turbines are more complex, often aerofoil-shaped to maximize the conversion of steam energy to mechanical energy through both pressure and velocity changes. Reaction turbines typically handle the lower-pressure, higher-volume steam stages, where the steam has expanded and cooled somewhat, requiring larger blades to accommodate the increased steam flow. Their design allows for improved efficiency in extracting energy from steam at these conditions, especially in the intermediate and low-pressure stages of the turbine.
The integration of both types in multi-stage turbines is common practice in modern power plants, where the steam first passes through impulse stages to extract energy at high pressure and then through reaction stages to capture the remaining energy at lower pressures. This staged approach optimizes the overall thermodynamic efficiency by carefully matching blade design and steam conditions throughout the turbine’s length. It also helps in managing mechanical stresses, as the transition from impulse to reaction stages allows for gradual changes in steam velocity and pressure, reducing blade fatigue and extending turbine lifespan.
Beyond their mechanical and aerodynamic differences, impulse and reaction turbines also differ in their operational and maintenance characteristics. Impulse turbines, due to their simpler blade design, can be easier to inspect and repair, with blades often designed to be replaceable individually. Reaction turbines require more precise manufacturing and balancing due to their complex blade shapes and the presence of significant pressure differences across moving blades, which can increase maintenance complexity and cost. However, their ability to handle larger steam volumes efficiently often outweighs these challenges in large-scale power generation applications.
In summary, the choice between impulse and reaction turbines—or more commonly, their combination within a single machine—depends on the specific steam conditions, desired efficiency, operational flexibility, and mechanical considerations. Engineers design steam turbines by leveraging the high-speed, high-pressure advantages of impulse stages along with the high-volume, low-pressure efficiency of reaction stages to build reliable, powerful, and efficient systems that form the backbone of modern thermal power generation worldwide.
Beyond the classic impulse and reaction designs, modern steam turbines have also evolved into various specialized configurations tailored to specific industrial applications and operational requirements. For example, back-pressure turbines are designed to exhaust steam at pressures higher than atmospheric, allowing the steam to be used for heating or process purposes downstream, making them ideal for combined heat and power (CHP) plants and industrial facilities where both electricity and thermal energy are needed. In contrast, condensing turbines expand steam down to very low pressures—often below atmospheric pressure—allowing maximum energy extraction and efficiency for power generation plants connected to condensers that convert exhaust steam back into water.
Another variation is the extraction turbine, which can bleed off steam at one or more intermediate stages to supply process steam or feedwater heaters without stopping the turbine. This flexibility improves overall plant efficiency by recycling steam energy where it is most needed. Additionally, controlled extraction and admission of steam allow these turbines to better match variable load demands, which is crucial for grid stability and industrial processes with fluctuating energy needs.
The design of blades and rotors in modern turbines has also become highly sophisticated. Advanced aerodynamic profiling reduces turbulence and flow separation, minimizing energy losses and mechanical vibrations. Rotors are dynamically balanced with high precision to avoid resonance and fatigue, essential for turbines that operate at thousands of revolutions per minute. Cooling techniques, such as internal air cooling or steam cooling of blades, allow turbines to withstand extreme temperatures in ultra-supercritical power plants where steam conditions exceed traditional limits.
Materials science has played a pivotal role in advancing turbine technology, with the introduction of high-strength alloys and ceramic coatings that resist corrosion, oxidation, and creep deformation at elevated temperatures. These materials extend turbine service life and enable operation under harsher steam conditions, further boosting efficiency and output. Combined with improved sealing technologies that minimize steam leakage and advanced lubrication systems, modern turbines achieve greater reliability and reduced maintenance intervals.
Control systems have similarly advanced from purely mechanical governors to complex electronic and digital controls that monitor a wide range of parameters in real time. These systems enable precise regulation of steam flow, turbine speed, and load balancing, ensuring safe and efficient operation even during rapid changes in demand. Integration with plant-wide automation and diagnostic platforms allows predictive maintenance and reduces downtime, optimizing the turbine’s lifecycle cost and availability.
Steam turbines continue to evolve in response to emerging energy trends, such as integration with renewable sources, hydrogen fuel adaptation, and carbon capture compatibility. Hybrid power plants that combine steam turbines with gas turbines, fuel cells, or energy storage systems leverage the strengths of multiple technologies to create flexible, low-carbon energy solutions. As the global energy landscape shifts towards sustainability and decarbonization, steam turbines remain a critical technology, adapting through innovation to meet the challenges of tomorrow’s power generation and industrial needs.
In essence, while impulse and reaction turbines form the foundational concepts, the broader family of steam turbine designs and technologies has diversified and matured to deliver unparalleled efficiency, flexibility, and durability. This continuous evolution ensures steam turbines will remain vital in global energy systems, supporting everything from large-scale electricity generation to industrial processes and emerging clean energy applications for many decades ahead.
The Thermodynamics Behind Steam Turbines
Steam turbines operate based on fundamental thermodynamic principles that govern the conversion of thermal energy from steam into mechanical work. At the core of this process is the Rankine cycle, a thermodynamic cycle widely used in power plants to generate electricity efficiently. Understanding the thermodynamics behind steam turbines involves exploring how steam’s pressure, temperature, and enthalpy change as it expands through the turbine stages, converting heat into useful mechanical energy.
The Rankine cycle starts with water being pumped to high pressure, which requires relatively little work compared to the energy extracted later. This high-pressure water then enters a boiler where heat is added, transforming it into high-temperature, high-pressure steam. The steam carries a significant amount of thermal energy in the form of enthalpy, which is the sum of internal energy and flow work. This high-energy steam is directed into the steam turbine, where it expands through a series of blades or stages, causing the turbine rotor to spin and generate mechanical power.
During the expansion in the turbine, the steam’s pressure and temperature decrease as it does work on the blades, converting its enthalpy into mechanical energy. This process ideally occurs isentropically, meaning it is reversible and without entropy generation, maximizing the conversion efficiency. However, in real turbines, friction, turbulence, and other irreversible processes cause entropy to increase, slightly reducing efficiency. The difference between the inlet and outlet steam enthalpy represents the net work output of the turbine.
As steam expands, it also undergoes changes in phase and quality. In early stages of expansion, steam is typically superheated, existing entirely as vapor above its boiling point at the given pressure. As it expands further and pressure drops, the steam temperature falls, and moisture begins to form, especially in low-pressure stages. Excess moisture is undesirable because water droplets can erode turbine blades and reduce efficiency. To mitigate this, many turbines use reheating cycles, where partially expanded steam is sent back to the boiler for reheating before further expansion, maintaining higher steam quality throughout the turbine.
The thermodynamic efficiency of the turbine is influenced by several key factors: inlet steam pressure and temperature, exhaust pressure, the degree of superheat, reheating, and regenerative feedwater heating. Higher inlet pressures and temperatures increase the available energy for conversion and improve efficiency, but require materials and designs that withstand more severe conditions. Lower exhaust pressures, often achieved by condensing the steam in a vacuum condenser, enable more extensive expansion and energy extraction but come with increased complexity and cost.
Regenerative feedwater heating, where steam is extracted at intermediate turbine stages to preheat the feedwater entering the boiler, recovers some of the thermal energy that would otherwise be wasted, improving the overall cycle efficiency. This process reduces the heat input needed in the boiler, lowering fuel consumption and emissions.
The first law of thermodynamics, which is the conservation of energy, governs the energy balance in the turbine. The difference in steam enthalpy between inlet and outlet equals the mechanical work output minus losses due to inefficiencies. The second law of thermodynamics, concerning entropy, explains why some energy is always lost as unusable heat due to irreversible processes like friction and turbulence inside the turbine and the steam flow passages.
Advanced steam turbines incorporate design features and operating practices to approach ideal thermodynamic performance as closely as possible. These include precise blade geometry to minimize aerodynamic losses, steam path sealing to reduce leakage, high-quality steam generation with superheating and reheating, and sophisticated control systems to optimize load and operating conditions.
In summary, the thermodynamics behind steam turbines revolve around converting the enthalpy of high-pressure, high-temperature steam into mechanical work through an expansion process ideally following the Rankine cycle. Efficiency depends on maximizing the energy extracted during expansion while minimizing losses due to irreversible phenomena and moisture formation. Understanding these thermodynamic principles is essential for designing, operating, and optimizing steam turbines in power generation and industrial applications.
The thermodynamic behavior of steam within a turbine is closely tied to its pressure, temperature, and phase changes throughout the expansion process. When steam enters the turbine at high pressure and temperature, it contains a large amount of enthalpy—the energy available for conversion into mechanical work. As the steam expands through the turbine blades, this enthalpy decreases, releasing energy that turns the rotor. Ideally, this expansion happens isentropically, meaning the process is reversible and no entropy is generated, which corresponds to the maximum theoretical efficiency. In practice, however, real turbines face losses caused by friction between steam and blades, turbulence in the flow, blade surface roughness, and leakage around seals, all contributing to an increase in entropy and reduced efficiency.
During expansion, the steam’s pressure and temperature drop, and its quality—the ratio of vapor to liquid phase—can change significantly. If the steam becomes too wet (contains too much moisture), water droplets can impact turbine blades at high velocity, causing erosion, corrosion, and fatigue that reduce turbine life and performance. To avoid this, many steam turbines incorporate reheat cycles, where steam partially expanded in the turbine is routed back to the boiler to be reheated before continuing through the subsequent turbine stages. This reheating raises the steam temperature again, improving its quality and allowing more efficient energy extraction while protecting turbine components.
The efficiency of steam turbines is also influenced by the exhaust pressure at the turbine outlet. Lowering the exhaust pressure, typically by using a condenser that creates a vacuum, enables steam to expand further and release more energy. This maximizes work output but requires additional equipment and operational complexity. On the other hand, some turbines exhaust steam at higher pressures intentionally, especially in industrial applications where the steam is used for heating or other processes downstream, sacrificing some power output for useful thermal energy.
A key strategy to improve overall cycle efficiency is regenerative feedwater heating. Steam is extracted at intermediate turbine stages to preheat the water entering the boiler, which reduces the amount of fuel needed to convert water into steam. This process recovers thermal energy that would otherwise be lost and smooths temperature gradients in the system, enhancing thermal efficiency and reducing wear on components.
Thermodynamically, the turbine converts the thermal energy of steam into mechanical energy in accordance with the first law of thermodynamics—energy conservation. The decrease in steam enthalpy from inlet to outlet translates directly into mechanical work, minus losses. The second law, dealing with entropy and irreversibility, explains why perfect conversion is impossible, as some energy is inevitably dissipated as heat due to turbulence, friction, and non-ideal fluid behavior.
In modern turbines, engineering efforts focus on minimizing these losses by optimizing blade geometry to reduce aerodynamic drag and flow separation, using advanced materials that tolerate higher temperatures and stresses, and implementing tight clearances and seals to limit steam leakage. Computer modeling and digital twins simulate thermodynamic performance, allowing designers to predict and enhance turbine efficiency under various operating conditions.
In conclusion, steam turbines function as practical embodiments of thermodynamic principles, converting steam’s enthalpy into mechanical work through controlled expansion. The interplay of pressure, temperature, entropy, and phase changes governs the turbine’s performance, while engineering advancements continually push these machines closer to ideal thermodynamic efficiency, making them vital in power generation and industrial energy systems worldwide.
Beyond the fundamental thermodynamics, the operational efficiency and longevity of steam turbines depend heavily on managing the complex interactions between steam flow, temperature gradients, mechanical stresses, and material properties. As steam expands and cools, thermal stresses develop within the turbine blades and casing due to uneven heating and cooling rates, which can lead to fatigue and cracking if not properly controlled. Engineers use detailed thermal and structural analyses to design blades and components that withstand these stresses, incorporating features such as flexible blade roots, stress-relief notches, and advanced alloys with high creep resistance. These measures ensure that turbines can operate continuously at high load and temperature without premature failure.
Moreover, the quality of steam entering the turbine is critical to maintaining efficiency and reducing maintenance. Impurities and dissolved solids in the steam can deposit on blades and cause corrosion or fouling, disrupting aerodynamic profiles and heat transfer. Therefore, steam is carefully treated and purified before entering the turbine system, often passing through water treatment plants and steam separators. Continuous monitoring of steam chemistry and turbine condition is also essential to detect early signs of degradation or contamination.
In the control of steam turbines, modern instrumentation and automation play a vital role. Sensors monitor parameters such as steam pressure, temperature, vibration, rotor speed, and blade clearance in real time. These inputs feed into sophisticated control systems that adjust steam flow, valve positions, and turbine speed to optimize performance, maintain safe operating limits, and respond quickly to changes in power demand or steam conditions. Such automation improves not only efficiency but also the safety and reliability of the turbine during startup, shutdown, and transient operations.
Thermodynamic optimization also extends to integrating the steam turbine within the larger power plant or industrial process. The turbine’s performance influences and is influenced by the boiler, condenser, feedwater heaters, and auxiliary systems. Advanced cycle designs, such as supercritical and ultra-supercritical Rankine cycles, operate at even higher pressures and temperatures to push thermodynamic limits, demanding turbines that can handle extreme conditions. In combined cycle power plants, steam turbines work alongside gas turbines, using waste heat from gas turbine exhaust to generate steam, thereby improving overall plant efficiency and reducing fuel consumption.
Finally, ongoing research focuses on improving thermodynamic efficiency through innovations like improved blade aerodynamics using computational fluid dynamics, advanced coatings to withstand higher steam temperatures, and hybrid cycles incorporating alternative working fluids or integrating renewable energy sources. The goal remains to maximize energy conversion from heat to mechanical work while minimizing fuel use, emissions, and operational costs.
In summary, the thermodynamics behind steam turbines form the backbone of their design and operation, but their real-world efficiency and reliability are achieved through meticulous engineering of materials, steam quality control, automated management, and integration within complex energy systems. This combination ensures steam turbines remain indispensable components in the global energy landscape.
How Steam Turbines Convert Heat to Electricity
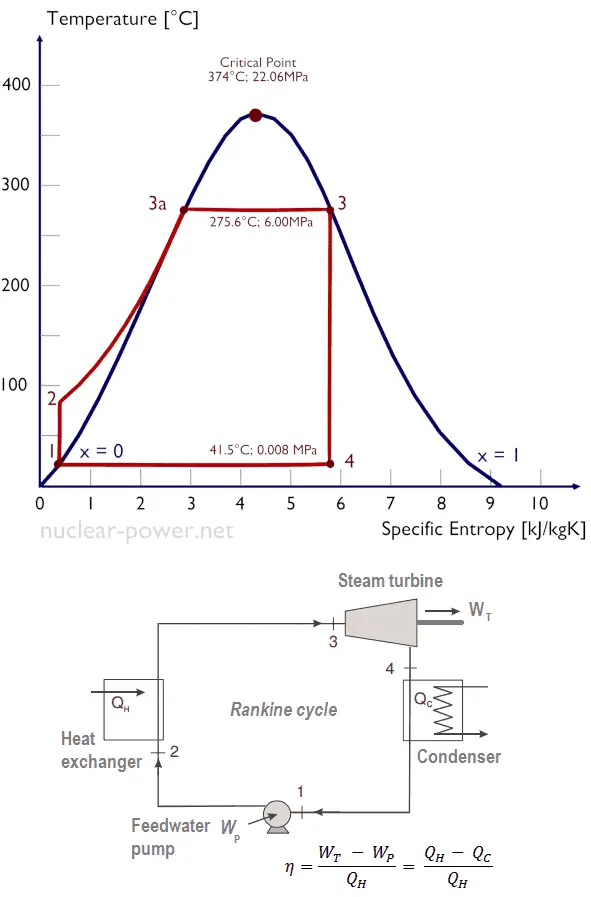
Steam turbines convert heat to electricity by harnessing the energy stored in high-pressure, high-temperature steam to drive a generator that produces electrical power. The process begins in a boiler, where fuel—such as coal, natural gas, biomass, or nuclear energy—is burned or used to heat water, converting it into steam. This steam carries significant thermal energy in the form of pressure and temperature.
The high-energy steam is then directed into the steam turbine, which consists of a rotor fitted with multiple stages of blades. As the steam flows over these blades, it expands and loses pressure and temperature, transferring its thermal energy to the turbine blades by exerting force on them. This force causes the turbine rotor to spin rapidly, converting the steam’s thermal energy into mechanical rotational energy.
Attached directly to the turbine shaft is an electrical generator, typically a large synchronous generator. As the turbine shaft rotates, it turns the rotor inside the generator, which consists of electromagnets spinning within coils of wire. This relative motion induces an electric current through electromagnetic induction, thereby converting mechanical energy into electrical energy.
The steam, having lost much of its energy in the turbine, exits at a lower pressure and temperature, often entering a condenser where it is cooled and condensed back into water. This condensate is then pumped back into the boiler to repeat the cycle, making the process continuous and efficient.
The overall efficiency of converting heat into electricity depends on several factors: the temperature and pressure of the steam entering the turbine, the design and condition of the turbine blades, how effectively the steam expands and transfers energy, and the efficiency of the generator. Advances such as superheating the steam, reheating between turbine stages, and regenerative feedwater heating help maximize the energy extracted from the steam, increasing the electrical output relative to the heat input.
In essence, steam turbines act as the crucial link between thermal energy generated by fuel combustion or nuclear reactions and the electrical power that fuels homes, industries, and infrastructure worldwide. Their ability to efficiently convert heat into rotational motion and then into electricity makes them foundational in modern power generation.
The conversion of heat to electricity in steam turbines is a highly integrated and continuous process where each component of the system plays a vital role in maximizing efficiency and reliability. After steam leaves the turbine, the low-pressure exhaust steam is often routed to a condenser where it is cooled by circulating water, typically from a river, lake, or cooling tower. This condensation process creates a vacuum that lowers the exhaust pressure, allowing the steam to expand more fully in the turbine and extract more energy, thus improving the overall efficiency of the cycle. The condensed water, or condensate, is then collected and pumped back into the boiler as feedwater, completing the closed-loop cycle and ensuring minimal water loss.
The turbine and generator are mechanically coupled so that the rotational speed of the turbine matches the generator’s requirements, often at thousands of revolutions per minute. Maintaining this speed precisely is crucial for synchronizing with the electrical grid frequency, which is usually 50 or 60 hertz depending on the region. Sophisticated control systems monitor the turbine speed, steam flow, and electrical output to regulate fuel input and steam conditions, allowing the plant to respond smoothly to changes in electricity demand and maintain grid stability.
The thermodynamic cycle that steam turbines operate on—the Rankine cycle—benefits greatly from enhancements such as reheating and regeneration. Reheating involves extracting partially expanded steam from the turbine, sending it back to the boiler for reheating, and then reintroducing it to later turbine stages. This raises the steam temperature, reduces moisture content at the final stages, and increases the total work output. Regeneration uses steam extracted at various points to preheat the feedwater before it enters the boiler, reducing the energy needed to convert water into steam and thus conserving fuel.
Modern steam turbines use advanced materials like nickel-based superalloys and ceramics capable of withstanding extremely high temperatures and pressures, allowing power plants to operate at supercritical or ultra-supercritical conditions. These conditions push the limits of thermodynamic efficiency, sometimes exceeding 45%, significantly reducing fuel consumption and emissions per unit of electricity generated compared to older technologies.
The electrical generator coupled to the turbine converts mechanical energy into electrical energy through electromagnetic induction. It consists of a rotor with a magnetic field rotating inside a stator containing coils of wire. As the rotor spins, it induces an alternating current in the stator windings, which is then transmitted to transformers and power lines for distribution. Generator efficiency is also critical, and modern designs achieve efficiencies above 98%, ensuring minimal energy loss during conversion.
Throughout the entire process, numerous auxiliary systems support efficient operation, including lubrication systems for bearings, sealing systems to prevent steam leakage, vibration monitoring to detect mechanical issues early, and cooling systems to manage heat generated in both the turbine and generator. Integrated monitoring and diagnostics software use real-time data to optimize performance and predict maintenance needs, reducing downtime and extending the life of expensive equipment.
Ultimately, the seamless conversion of thermal energy from steam into electricity by steam turbines is a cornerstone of modern power generation. Their ability to efficiently harness heat from a wide range of energy sources—fossil fuels, nuclear reactors, biomass, or even concentrated solar power—makes steam turbines versatile and indispensable in meeting the world’s growing energy demands while advancing toward cleaner and more sustainable energy systems.
In addition to their central role in electricity generation, steam turbines have evolved to accommodate a variety of energy sources and configurations, further enhancing their versatility and efficiency. For instance, in nuclear power plants, steam turbines are driven by steam generated through the heat produced by nuclear fission, rather than combustion. This steam, typically at lower temperatures than fossil-fuel boilers, still follows the same thermodynamic principles but requires turbines designed to handle the specific conditions and purity requirements of nuclear steam. The clean, continuous heat source from nuclear reactors allows for steady power output with low greenhouse gas emissions, making steam turbines critical in low-carbon energy production.
Moreover, advancements in combined cycle power plants integrate gas turbines and steam turbines to achieve higher overall efficiencies. In such plants, a gas turbine burns natural gas to generate electricity and produces high-temperature exhaust gases. Instead of releasing these gases into the atmosphere, they are directed to a heat recovery steam generator (HRSG) that produces steam for a steam turbine. This combined approach can push total plant efficiency beyond 60%, significantly outperforming traditional steam-only cycles. Steam turbines in these configurations are specially designed to handle the steam conditions produced by HRSGs, often involving rapid changes in load and temperature, necessitating robust materials and flexible controls.
In renewable energy sectors, steam turbines also find applications. Concentrated solar power (CSP) plants use mirrors or lenses to focus sunlight onto a receiver, generating high-temperature steam that drives turbines similarly to fossil-fuel plants but without combustion emissions. Here, the steam turbine must be adaptable to fluctuations in solar input and sometimes incorporate thermal energy storage systems that provide steam during periods without sunlight, ensuring steady power generation.
Another dimension of steam turbine use is in industrial cogeneration or combined heat and power (CHP) systems. These systems simultaneously produce electricity and useful thermal energy, often for heating or manufacturing processes. Steam turbines in CHP plants extract energy from steam to generate electricity but also supply lower-pressure steam or hot water for process heating, improving overall fuel utilization efficiency. By capturing and using waste heat rather than discarding it, CHP systems can achieve total energy efficiencies of 70-90%, far surpassing typical standalone power generation plants.
The ongoing development of materials science and engineering, including coatings resistant to high-temperature corrosion and fatigue, advanced blade cooling techniques, and additive manufacturing, continues to push steam turbine performance. Computational fluid dynamics (CFD) and finite element analysis (FEA) allow precise modeling of steam flow and mechanical stresses, optimizing blade shapes and turbine components for maximum efficiency and reliability.
Ultimately, steam turbines represent a mature yet continually advancing technology that transforms heat energy into mechanical and then electrical energy through well-understood thermodynamic processes. Their adaptability to various fuels and applications, combined with improvements in efficiency, durability, and control, ensures they remain a fundamental component in global energy systems—supporting the transition to cleaner, more efficient, and more sustainable power generation for decades to come.
The Role of Steam Turbines in Power Plants
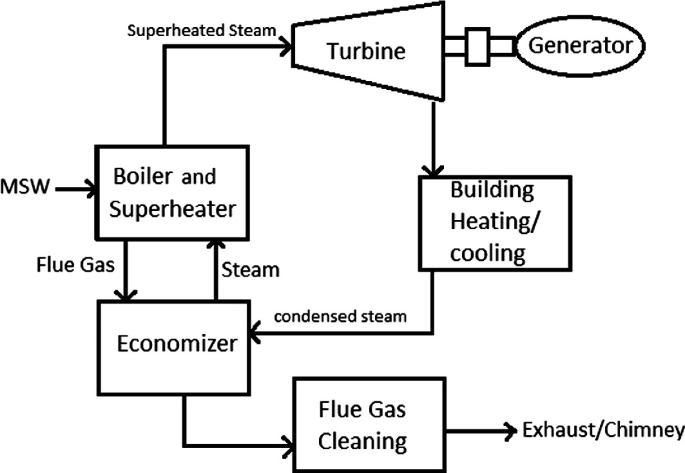
Steam turbines play a central role in power plants by serving as the primary machinery that converts thermal energy from steam into mechanical energy, which is then transformed into electrical energy. In a typical thermal power plant—whether fueled by coal, natural gas, biomass, or nuclear reactions—the process begins with the generation of high-pressure, high-temperature steam in a boiler or steam generator. This steam is then directed into the steam turbine, where it expands through multiple stages of blades fixed on a rotating shaft. As the steam passes over these blades, it imparts force that causes the turbine rotor to spin at high speeds, typically thousands of revolutions per minute. This mechanical energy is coupled directly to an electrical generator, which converts the rotational motion into electricity through electromagnetic induction.
The efficiency and capacity of a power plant largely depend on the design and operation of its steam turbine. Large-scale turbines are engineered to extract the maximum possible energy from steam, operating under high pressures and temperatures to improve thermodynamic efficiency. Modern steam turbines are built to withstand these extreme conditions, using advanced alloys and precision engineering to maintain reliability over prolonged operation. The steam’s expansion within the turbine is carefully staged to balance the pressure drop and control moisture content, preventing damage to turbine blades and maintaining efficiency.
Beyond electricity generation, steam turbines in power plants are integrated into a complex system of components that include boilers, condensers, feedwater heaters, and control systems. After passing through the turbine, steam typically enters a condenser where it is cooled and condensed back into water. This condensate is then recycled back into the boiler, ensuring a continuous loop that conserves water and improves plant efficiency. Additionally, feedwater heaters use steam extracted from intermediate turbine stages to preheat the water before it re-enters the boiler, reducing the fuel required to generate steam and improving overall cycle efficiency.
Steam turbines also enable flexible operation of power plants, allowing them to adjust power output in response to changing electricity demands. Turbine control systems regulate steam flow and rotational speed to maintain grid stability and meet variable load requirements. This flexibility is crucial for balancing supply and demand in modern electrical grids, especially with increasing integration of intermittent renewable energy sources like solar and wind.
In combined cycle power plants, steam turbines work alongside gas turbines to boost efficiency. The waste heat from gas turbine exhaust is used to produce steam in a heat recovery steam generator, which then drives a steam turbine. This hybrid approach significantly increases the overall efficiency of the plant compared to single-cycle operations, making steam turbines indispensable even in plants primarily fueled by natural gas.
In summary, steam turbines in power plants act as the vital link between thermal energy production and electricity generation. Their ability to efficiently convert steam’s heat into mechanical power, their integration within complex thermodynamic cycles, and their adaptability to different fuel sources and operational conditions underscore their essential role in supplying reliable, large-scale electrical power across the globe.
Steam turbines are fundamental to power plants because they enable the large-scale conversion of heat energy into usable electrical energy with high efficiency and reliability. In essence, they act as the mechanical heart of the plant, taking the thermal energy produced by burning fuels or nuclear reactions and transforming it into the rotational energy needed to generate electricity. This conversion process is critical because heat itself cannot be directly used to power electrical grids; it must first be converted into mechanical motion and then into electrical current.
The design of steam turbines in power plants is highly specialized to maximize the extraction of energy from steam. High-pressure steam enters the turbine and flows through a series of stages, each consisting of stationary and moving blades. The stationary blades direct the steam flow efficiently onto the moving blades, which convert the steam’s kinetic and pressure energy into shaft rotation. By expanding the steam in multiple stages rather than all at once, the turbine ensures smoother operation, reduces mechanical stress, and limits moisture buildup that can erode blade surfaces.
After the steam has passed through the turbine, it exits at a much lower pressure and temperature. To maximize efficiency, this exhaust steam is sent to a condenser, where it is cooled and condensed back into liquid water. The condenser typically uses cooling water from a river, cooling tower, or other source to absorb heat from the steam. By maintaining the exhaust at low pressure (often creating a vacuum), the turbine can expand the steam further, extracting more energy and increasing the overall efficiency of the power cycle. This step is crucial in the Rankine cycle, the thermodynamic cycle that governs most steam power plants.
The condensed water, or condensate, is then pumped back into the boiler feedwater system, often passing through feedwater heaters that use extracted steam from intermediate turbine stages to preheat the water. This regeneration process reduces the fuel needed to convert water into steam by recovering some of the thermal energy that would otherwise be wasted, improving the plant’s fuel economy and reducing emissions.
Modern steam turbines are designed to operate at extremely high pressures and temperatures, especially in supercritical and ultra-supercritical power plants. These advanced designs push the thermodynamic limits of efficiency, allowing plants to produce more electricity from the same amount of fuel. However, operating at such extreme conditions demands advanced materials capable of withstanding high thermal stresses and corrosion, along with precise manufacturing and quality control to ensure reliability and longevity.
Power plants also rely on sophisticated control systems to manage steam turbine operation. These systems regulate steam flow, turbine speed, and electrical output, ensuring smooth responses to changes in electricity demand and maintaining grid stability. During startup, shutdown, or load changes, the turbine control system carefully adjusts parameters to avoid mechanical shocks or thermal stresses that could damage the turbine.
In some plants, especially combined heat and power (CHP) facilities, steam turbines provide not only electricity but also useful heat for industrial processes or district heating. Here, the exhaust steam from the turbine is captured for heating rather than condensed, improving overall energy utilization and reducing waste.
The integration of steam turbines into power plants represents a balance of thermodynamics, mechanical engineering, materials science, and control technology. Their ability to convert thermal energy into electricity efficiently and reliably makes them indispensable in meeting global electricity demands, providing flexible power generation that can accommodate various fuel sources, from fossil fuels and nuclear energy to renewables like biomass and solar thermal. This versatility ensures that steam turbines will continue to be at the core of power generation infrastructure for the foreseeable future.
Steam turbines also play a critical role in shaping the operational flexibility and environmental performance of modern power plants. As energy grids become increasingly complex with the integration of renewable sources like wind and solar—both of which are intermittent by nature—steam turbines help balance supply and demand by ramping power production up or down relatively quickly. Although traditionally viewed as suited for steady baseload operation, advances in turbine design and control technology have enhanced their ability to handle variable loads without compromising efficiency or causing excessive wear. This flexibility supports grid stability, allowing power plants to complement renewables and ensure continuous electricity availability.
From an environmental perspective, steam turbines contribute to reducing emissions by enabling more efficient fuel utilization. Higher thermal efficiencies mean less fuel is burned for the same amount of electricity generated, directly lowering carbon dioxide and pollutant emissions. Moreover, when integrated into combined cycle plants, steam turbines help capture and utilize waste heat from gas turbines, substantially improving overall plant efficiency and further cutting emissions. In CHP applications, using steam turbines to generate both electricity and heat maximizes the energy extracted from fuel sources, minimizing waste and reducing the environmental footprint of industrial and district energy systems.
Another key aspect is the role of steam turbines in emerging clean energy technologies. Concentrated solar power (CSP) plants use steam turbines driven by solar-heated steam, providing renewable electricity with the added advantage of thermal storage, which helps supply power even when the sun isn’t shining. Similarly, biomass-fired power plants use steam turbines to convert organic matter into energy, offering a renewable and potentially carbon-neutral alternative to fossil fuels. Even in nuclear power, steam turbines are indispensable, converting heat from nuclear reactions into electricity without combustion emissions.
Maintenance and lifecycle management of steam turbines are vital to ensuring their long-term performance and cost-effectiveness. Routine inspections, blade condition monitoring, vibration analysis, and lubrication system maintenance are part of comprehensive programs to prevent failures and extend equipment life. Modern diagnostic tools, including sensors and predictive analytics powered by artificial intelligence, enable operators to detect early signs of wear or imbalance, schedule timely maintenance, and avoid unplanned outages.
Looking forward, research into new materials such as ceramics and advanced metal alloys aims to further increase the operating temperatures and pressures steam turbines can withstand, pushing efficiencies even higher. Innovations in blade design, cooling technologies, and additive manufacturing are also poised to enhance turbine durability and performance. These technological advances, combined with growing emphasis on decarbonization and renewable integration, ensure that steam turbines will remain a cornerstone of energy infrastructure, evolving to meet the challenges of a sustainable energy future while continuing to provide reliable, large-scale power generation around the world.
Steam Turbine Efficiency: Maximizing Output
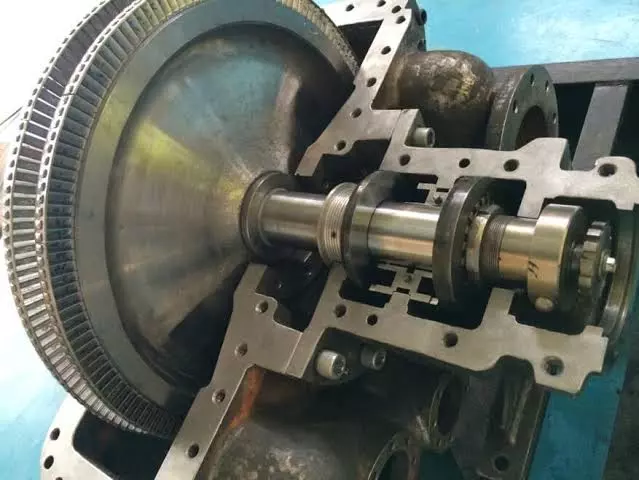
Steam turbine efficiency is a critical factor in power generation, directly influencing how much electrical energy can be produced from a given amount of fuel or heat input. Maximizing steam turbine efficiency involves optimizing the conversion of thermal energy in steam into mechanical work while minimizing energy losses throughout the process. The overall efficiency of a steam turbine is typically expressed as the ratio of useful mechanical or electrical energy output to the thermal energy supplied to generate the steam. Several key principles and engineering strategies contribute to maximizing this efficiency.
One fundamental way to improve steam turbine efficiency is by increasing the temperature and pressure of the steam entering the turbine. Higher inlet steam temperatures and pressures increase the available energy for expansion through the turbine stages, allowing more mechanical work to be extracted. Modern power plants achieve this by operating at supercritical or ultra-supercritical conditions, where steam behaves more like a gas with higher enthalpy, improving the thermodynamic efficiency of the Rankine cycle that governs steam turbine operation.
The turbine’s design itself also greatly impacts efficiency. Multi-stage turbines with carefully engineered blades enable gradual expansion of steam, optimizing the energy extraction at each stage while minimizing turbulence and flow losses. Blade profiles are aerodynamically shaped to reduce drag and increase lift, allowing steam to transfer its energy more effectively to the rotor. Advances in computational fluid dynamics (CFD) allow engineers to model and refine blade designs for optimal steam flow characteristics and minimal energy dissipation.
Another critical factor is reducing steam leakage and mechanical losses. Seals and labyrinth packing are used around the turbine shaft and casing to minimize steam escaping without doing work, preserving pressure and energy within the system. High-precision manufacturing and tight tolerances reduce frictional losses in bearings and rotating parts, contributing to improved mechanical efficiency.
Moisture content in the steam also affects turbine efficiency. As steam expands and cools through the turbine, it can condense into tiny water droplets that erode blades and reduce aerodynamic efficiency. To mitigate this, reheating stages are often incorporated, where steam is extracted mid-expansion, reheated in the boiler, and then reintroduced into later turbine stages at higher temperatures. This process reduces moisture formation and increases the average temperature at which work is extracted, boosting efficiency and prolonging turbine life.
Regenerative feedwater heating is another technique used to improve efficiency by recovering some of the thermal energy from steam extracted at intermediate turbine stages to preheat the boiler feedwater. This reduces the fuel required to generate steam, effectively recycling energy within the cycle and increasing the overall plant efficiency.
Condenser performance also plays a role, as maintaining a low exhaust pressure through effective condensation increases the steam expansion ratio and the amount of work extracted in the turbine. Efficient cooling systems that maintain low condenser temperatures enhance this effect, further improving turbine efficiency.
Finally, regular maintenance and monitoring ensure that the turbine operates at peak efficiency over its lifespan. Wear, fouling, and blade damage can degrade performance, so condition monitoring, vibration analysis, and timely repairs are essential to sustain high efficiency.
In summary, maximizing steam turbine efficiency involves a combination of thermodynamic principles, advanced materials, precise engineering, and operational strategies. By optimizing steam conditions, turbine design, and cycle integration, modern power plants can extract the maximum possible energy from steam, reducing fuel consumption and emissions while producing reliable electricity at competitive costs.
Improving steam turbine efficiency is an ongoing challenge that drives innovation in both design and operational practices. One of the most significant advancements in this area is the development of supercritical and ultra-supercritical steam turbines, which operate at pressures and temperatures above the critical point of water. At these extreme conditions, steam no longer undergoes a distinct phase change from liquid to vapor, allowing for more efficient energy transfer and reducing losses associated with condensation inside the turbine. Plants operating with ultra-supercritical steam conditions can achieve thermal efficiencies exceeding 45%, compared to around 33-35% for older subcritical units. Achieving these conditions requires advanced materials capable of withstanding intense thermal stresses and corrosion, often involving nickel-based alloys and sophisticated cooling techniques to maintain blade integrity.
Another area of efficiency improvement lies in turbine aerodynamics and blade design. Engineers use computational fluid dynamics (CFD) simulations to optimize blade shapes, angles, and surface finishes to reduce turbulence, pressure drops, and flow separation. The result is a smoother steam flow that transfers energy more effectively to the rotating shaft. Additionally, the number and arrangement of turbine stages are carefully engineered to extract the maximum work while preventing excessive moisture buildup. To further manage moisture, reheaters are incorporated in the steam cycle to boost steam temperature partway through expansion, maintaining dryness and protecting turbine blades from erosion.
Beyond design, operational strategies are vital to maintaining high efficiency. Turbine load management ensures that the machine operates close to its optimal design point, as significant deviations can lead to inefficiencies and increased wear. Control systems monitor parameters such as steam temperature, pressure, and flow rate, adjusting valves and turbine speed dynamically to respond to grid demands without sacrificing efficiency. In some cases, predictive maintenance powered by sensor data and AI algorithms detects early signs of component degradation, allowing operators to perform repairs before efficiency drops or failures occur.
Thermodynamic cycle enhancements also contribute significantly. Regenerative feedwater heating systems capture steam from intermediate turbine stages and use it to preheat the incoming feedwater. This reduces the fuel required to convert water into steam by raising its temperature before entering the boiler, effectively recycling energy within the cycle and boosting overall plant efficiency. In addition, condensers that efficiently convert exhaust steam back to water while maintaining low back pressure enhance the turbine’s ability to expand steam fully, increasing mechanical work output.
Moreover, combined cycle power plants exemplify how integrating steam turbines with other technologies maximizes efficiency. In such plants, a gas turbine generates electricity and produces hot exhaust gases that feed a heat recovery steam generator (HRSG), which in turn produces steam for the steam turbine. By capturing and utilizing waste heat that would otherwise be lost, combined cycle plants can reach efficiencies over 60%, making them some of the most efficient thermal power generation systems available today.
Environmental regulations and economic pressures have also pushed for improvements in steam turbine efficiency. Lower fuel consumption not only reduces operating costs but also decreases greenhouse gas and pollutant emissions, aligning with global efforts to combat climate change. As a result, research continues into novel materials, coatings, and cooling methods that allow turbines to operate at even higher temperatures and pressures safely.
In summary, maximizing steam turbine efficiency involves an intricate balance of thermodynamics, advanced engineering, precise operation, and maintenance practices. By pushing the boundaries of material science, aerodynamic design, and system integration, modern steam turbines achieve remarkable efficiencies that support cleaner, more sustainable power generation while meeting the ever-growing global demand for electricity.
To further enhance steam turbine efficiency, ongoing research and development focus heavily on material science and manufacturing innovations. One of the critical challenges is the turbine’s exposure to extreme temperatures, often exceeding 600 degrees Celsius in ultra-supercritical units. At these temperatures, conventional steels can weaken or corrode, leading to reduced lifespan and efficiency losses. To combat this, advanced nickel-based superalloys are employed for turbine blades and components, offering exceptional strength and resistance to oxidation and creep deformation. Additionally, thermal barrier coatings (TBCs) are applied to protect surfaces from heat, allowing components to maintain mechanical integrity even under severe operating conditions. These materials enable turbines to operate safely at higher temperatures, directly improving thermodynamic efficiency.
Manufacturing techniques such as additive manufacturing (3D printing) are beginning to revolutionize turbine component production. This technology allows for highly complex blade geometries that were previously impossible or prohibitively expensive to create using traditional methods. Complex internal cooling channels can be embedded within blades, improving heat dissipation and allowing turbines to sustain higher inlet temperatures without damage. Additive manufacturing also supports rapid prototyping and customization, accelerating the development of next-generation turbine designs optimized for efficiency and durability.
Operational flexibility is another frontier where steam turbines are evolving. Historically, steam turbines were best suited for steady-state, base-load operation because rapid changes in temperature and pressure can cause thermal stress and mechanical fatigue. However, modern grids require power plants to ramp output up and down frequently to balance fluctuating renewable energy sources. Advances in control systems and turbine materials have enhanced load-following capabilities, allowing steam turbines to respond more quickly and safely to changing demands without significant efficiency penalties or damage risk.
Integration with digital technologies is playing an increasingly important role in efficiency optimization. Sensors embedded throughout the turbine continuously monitor vibration, temperature, pressure, and blade condition. Data analytics and artificial intelligence process this information in real time, enabling predictive maintenance that prevents unexpected failures and optimizes operating conditions. These smart systems help maintain peak efficiency by identifying subtle performance degradations early and recommending adjustments or repairs before losses escalate.
Furthermore, steam turbine efficiency improvements are closely tied to broader power plant cycle innovations. For example, advanced combined heat and power (CHP) systems utilize steam turbines not only for electricity but also for providing heat to industrial processes or district heating networks. By capturing and utilizing the turbine exhaust steam for heating rather than condensing it, CHP systems significantly increase total fuel utilization efficiency, sometimes exceeding 80%, far above typical electricity-only plants.
In the renewable energy sector, concentrated solar power (CSP) plants employ steam turbines driven by solar-generated steam. These systems often incorporate thermal energy storage, allowing steam turbines to generate electricity even when sunlight is unavailable. This capability enhances grid stability and increases the practical efficiency and reliability of solar thermal power generation.
Looking ahead, the development of hybrid cycles combining steam turbines with emerging technologies such as supercritical CO2 turbines or advanced energy storage systems promises to push efficiency boundaries even further. These hybrid systems aim to leverage the high energy density and rapid response of new technologies while utilizing the proven reliability and scalability of steam turbines.
Ultimately, steam turbine efficiency is a multifaceted challenge requiring ongoing innovation across materials, design, operation, and integration. Continuous improvements contribute to lowering the environmental impact of power generation, reducing fuel consumption, and supporting the transition toward cleaner, more sustainable energy systems worldwide. As global energy demands grow and decarbonization efforts intensify, the role of highly efficient steam turbines in providing reliable and affordable electricity remains indispensable.
Advanced Steam Turbine Materials
Advanced steam turbine materials are fundamental to pushing the limits of turbine performance, reliability, and efficiency, especially as modern turbines operate at increasingly high temperatures and pressures. The ability of turbine components to withstand extreme thermal, mechanical, and chemical stresses directly impacts not only efficiency but also durability and maintenance intervals. Over the years, materials technology has evolved from traditional steels to sophisticated superalloys and coatings specifically engineered for the demanding conditions inside steam turbines.
The primary challenge for turbine materials is to maintain mechanical strength and resist creep deformation—a slow, permanent shape change under stress at high temperature—while also resisting oxidation, corrosion, and thermal fatigue caused by the repeated heating and cooling cycles during operation. To meet these requirements, engineers have turned to nickel-based superalloys, which offer excellent high-temperature strength and corrosion resistance. These alloys are often used for turbine blades, nozzles, and other critical components exposed directly to high-velocity steam.
Nickel-based superalloys are complex mixtures of metals such as chromium, cobalt, aluminum, and titanium, which form a microstructure that maintains strength at elevated temperatures. They resist oxidation by forming a stable oxide layer on the surface, protecting the underlying material from further degradation. Additionally, the aluminum and titanium contribute to the formation of a gamma-prime (γ’) phase, a precipitate that significantly strengthens the alloy by hindering dislocation movement within the metal’s crystal lattice.
In addition to the base alloy composition, surface engineering techniques play a vital role in enhancing material performance. Thermal barrier coatings (TBCs) are ceramic layers applied to turbine blades and vanes to insulate the underlying metal from the hottest steam temperatures. These coatings reduce the thermal load on the metal, enabling turbines to operate at higher steam inlet temperatures without risking component failure. Common TBC materials include yttria-stabilized zirconia (YSZ), which offers low thermal conductivity and excellent stability under thermal cycling.
Moreover, advanced cooling methods complement these materials. Internal cooling channels within turbine blades allow cooler air, often bled from earlier compressor stages in combined cycle plants, to flow through the blade interior. This active cooling reduces blade metal temperatures and prevents overheating, extending component life and enabling more aggressive steam conditions that boost efficiency.
For other parts of the turbine, such as the rotor shaft and casing, high-strength steels and alloys are used that balance toughness, fatigue resistance, and manufacturability. Rotors, in particular, must withstand enormous centrifugal forces during operation and require materials with excellent mechanical properties and low susceptibility to cracking.
Another significant advancement is the use of powder metallurgy techniques to manufacture superalloy components. This method produces highly uniform microstructures with fewer defects, resulting in better mechanical properties and resistance to creep and fatigue. Powder metallurgy also allows for complex shapes to be produced more precisely, improving overall turbine aerodynamics and performance.
Looking to the future, research is exploring ceramic matrix composites (CMCs) and other ultra-high-temperature materials that could withstand even more extreme operating environments, potentially raising turbine inlet temperatures beyond current limits. CMCs combine the high-temperature resilience of ceramics with enhanced toughness and damage tolerance, offering the promise of lighter, stronger components that further improve efficiency and reduce cooling requirements.
In summary, advanced steam turbine materials are a cornerstone of modern turbine technology, enabling higher operating temperatures and pressures that directly translate to improved efficiency and power output. The interplay of superalloys, coatings, and cooling technologies allows turbines to perform reliably under extreme conditions, extending service life and reducing maintenance costs. As energy demands grow and environmental regulations tighten, continued materials innovation will be essential to maintaining the steam turbine’s role as a key technology in clean and efficient power generation.
Advanced steam turbine materials form the backbone of high-efficiency, high-performance turbine systems by enabling these machines to operate reliably under extreme thermal and mechanical conditions. The evolution of materials used in steam turbines has been driven by the push for higher operating temperatures and pressures, which directly improve thermodynamic efficiency but place immense stress on components. Traditional steels, while suitable for earlier designs, could not withstand the temperatures and creep stress associated with modern ultra-supercritical and advanced combined cycle operations. As a result, engineers began incorporating nickel-based superalloys that retain mechanical strength and resist oxidation at temperatures well above what conventional materials can endure. These superalloys are carefully engineered with elements like chromium, cobalt, molybdenum, and titanium to create a microstructure that can maintain its shape, resist deformation, and handle the corrosive nature of high-velocity steam over long periods.
One of the key features of these advanced materials is their resistance to creep, a slow, time-dependent deformation that occurs at high temperature and constant stress. This is critical for turbine blades, which must maintain their precise shape and structural integrity to ensure efficient energy transfer from steam to rotational energy. In addition to their internal strength, many turbine components are coated with thermal barrier coatings (TBCs) to insulate the base metal from direct exposure to extreme heat. These ceramic-based coatings, often made from yttria-stabilized zirconia, provide thermal insulation while also withstanding rapid temperature fluctuations during turbine start-ups and shutdowns. Their presence allows engineers to push steam temperatures even higher, extracting more energy per unit of fuel while extending the service life of the underlying materials.
Beyond coatings, component cooling also plays a crucial role in material performance. Many modern turbine blades are hollow and contain intricate internal cooling channels through which air or steam can pass, removing excess heat and protecting the metal from damage. This combination of high-performance alloys, protective coatings, and active cooling enables turbines to operate at steam temperatures exceeding 600°C, and in some advanced applications, approaching 700°C. These conditions yield significantly higher thermal efficiencies but would be impossible without advanced materials.
Manufacturing techniques have also evolved to support the use of these materials. Powder metallurgy allows for precise control over the grain structure and composition of superalloys, resulting in parts that are more uniform, durable, and resistant to fatigue and creep. Additive manufacturing, or 3D printing, is becoming increasingly important as well, enabling the production of complex geometries with internal features that enhance cooling or improve aerodynamics—features that would be difficult or impossible to create using traditional casting or forging techniques.
In large structural parts of the turbine, such as the rotors and casings, high-strength martensitic and ferritic steels are often used. These materials offer a balance of thermal resistance, strength, and toughness that ensures stability under the immense centrifugal forces generated during high-speed rotation. However, even in these areas, incremental improvements in alloy formulation and heat treatment continue to push performance boundaries.
Research is currently exploring the potential of ceramic matrix composites (CMCs), which are lighter than metals and can endure much higher temperatures without active cooling. These materials promise a paradigm shift in turbine design, potentially allowing for dramatically higher inlet temperatures and greater efficiency with fewer cooling losses. Though challenges remain in manufacturing, cost, and impact resistance, the future of steam turbine materials likely lies in such revolutionary technologies.
In essence, advanced steam turbine materials are not just passive components; they are enablers of progress, directly tied to efficiency, durability, and the environmental footprint of power generation. Their development reflects the intersection of metallurgy, thermodynamics, and engineering innovation, allowing steam turbines to meet modern energy demands while complying with stricter emissions and performance standards. As the energy sector evolves, these materials will continue to play a central role in unlocking new levels of performance and sustainability.
As steam turbine materials continue to advance, their influence extends beyond the physical integrity of turbine components to the economics of power generation and long-term operational planning. Materials that allow for higher efficiency translate directly into reduced fuel consumption per unit of electricity generated, which not only lowers operating costs but also significantly cuts greenhouse gas emissions. For power plants running on fossil fuels, this can mean compliance with stricter emissions regulations and improved competitiveness in energy markets increasingly focused on sustainability. Even in renewable or hybrid systems, such as those incorporating solar thermal energy or biomass, better materials enable greater thermal storage and generation capacity, supporting round-the-clock power delivery and grid reliability.
In addition to improving performance and efficiency, advanced materials contribute to reducing unplanned downtime. Steam turbines operate under demanding conditions for extended periods, and materials that resist fatigue, corrosion, and thermal degradation extend maintenance intervals and reduce the likelihood of sudden failures. For instance, turbine blades made from modern superalloys may operate reliably for tens of thousands of hours before requiring inspection or replacement, even under the stresses of high-temperature, high-pressure steam. This reliability is essential in baseload and industrial applications where interruptions to power generation can have costly ripple effects.
Furthermore, the integration of materials science with digital monitoring technologies creates a feedback loop that helps continuously refine turbine operation. Sensors embedded in critical turbine components can measure temperature, vibration, strain, and corrosion in real time, feeding data into predictive maintenance systems that assess material condition. By monitoring how materials behave under actual field conditions, engineers can identify performance trends and failure precursors, allowing for early intervention and more accurate lifecycle assessments. This not only enhances safety but also supports more informed decisions about turbine upgrades, replacements, or retrofits.
Another benefit of material advancements is their role in enabling compact and modular turbine designs. As materials become stronger and more heat-tolerant, turbines can be designed with fewer stages or smaller footprints without sacrificing output. This opens up new applications for steam turbines in decentralized power generation, including industrial cogeneration, waste heat recovery systems, and portable or containerized units that can be deployed in remote or rapidly developing areas. In such contexts, the use of advanced materials makes high-efficiency power generation accessible in places where traditional large-scale infrastructure would be impractical.
It is also important to recognize the environmental advantages of more durable and efficient materials. Beyond reducing emissions from fuel combustion, long-lasting turbine components decrease the environmental burden of manufacturing and material extraction. Fewer replacements over the lifetime of a turbine mean fewer raw materials mined, less energy used in production, and less waste to dispose of. When combined with recycling and material recovery programs, this can significantly enhance the sustainability profile of steam power plants.
Global collaboration among materials scientists, turbine manufacturers, and energy providers continues to push the boundaries of what turbine materials can achieve. Major research initiatives are exploring not only higher temperature capability but also improved resistance to steam impurities such as silica, chlorides, and sulfates, which can cause pitting, erosion, and stress corrosion cracking. Future breakthroughs may include self-healing materials that can repair microcracks, or smart materials that change properties in response to environmental conditions to optimize performance dynamically.
Ultimately, the path forward for steam turbines will depend not only on advances in turbine architecture or control systems but also—perhaps most critically—on the continued evolution of the materials from which they are built. These materials are what allow turbines to safely and efficiently convert the raw heat of combustion or solar energy into the mechanical energy that powers the world. As global demand for cleaner, more efficient, and more reliable energy continues to rise, the role of advanced materials in enabling that transformation becomes ever more central and indispensable.
High-Efficiency Steam Turbines for Power Generation
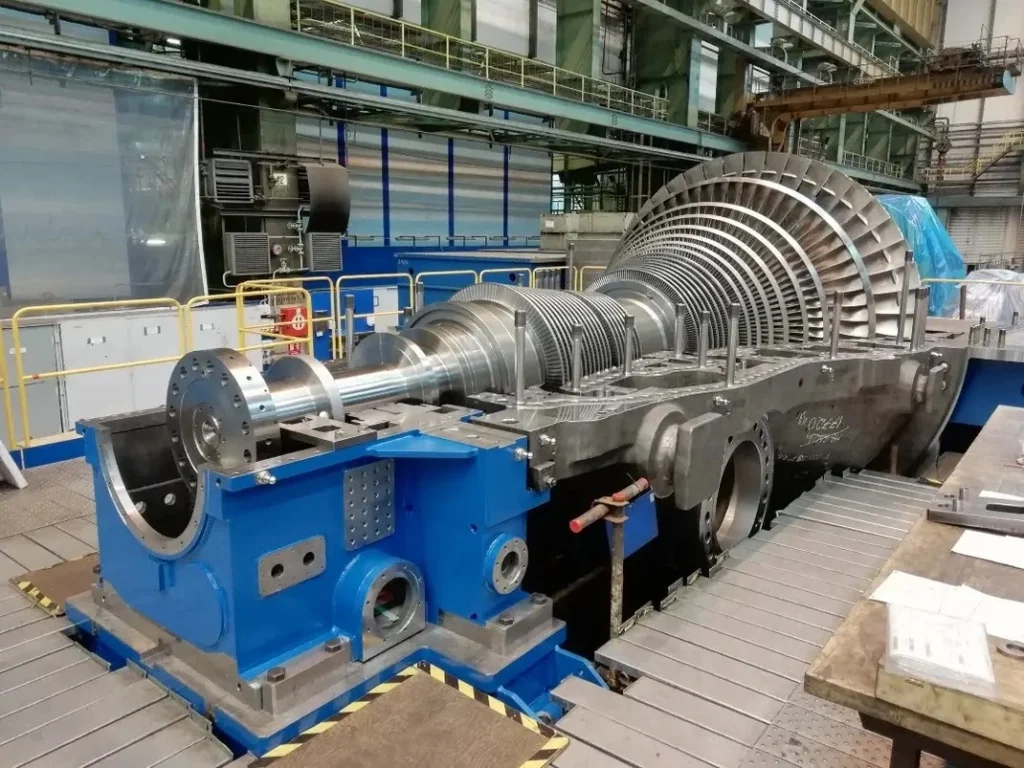
High-efficiency steam turbines represent a pinnacle of engineering in modern power generation, achieving remarkable thermal performance by combining advanced thermodynamic design, precision manufacturing, and cutting-edge materials. These turbines are crucial for maximizing energy output from a given amount of fuel—whether fossil-based or renewable—by extracting as much usable mechanical work as possible from high-pressure, high-temperature steam. In doing so, they play a central role in reducing fuel costs, lowering greenhouse gas emissions, and improving the overall sustainability and competitiveness of power plants.
One of the primary ways high-efficiency turbines achieve superior performance is through the use of ultra-supercritical (USC) steam conditions. While traditional subcritical turbines operate below the critical point of water (374°C and 22.1 MPa), USC turbines operate at pressures above this threshold and temperatures that often exceed 600°C. These extreme conditions increase the thermal efficiency of the Rankine cycle, reducing the amount of fuel required to produce a unit of electricity. Some state-of-the-art units are even moving toward advanced ultra-supercritical (A-USC) designs, where temperatures can reach 700°C or higher. Achieving and sustaining such conditions requires materials and components capable of withstanding extreme heat, pressure, and corrosion over prolonged periods.
The aerodynamic design of turbine blades is another critical factor in high efficiency. By optimizing blade profiles and spacing, engineers can minimize losses due to turbulence and steam leakage. Advanced blade shapes reduce drag and increase the velocity transformation from steam to rotational energy, directly improving the mechanical-to-electrical conversion process. Multi-stage turbines, where steam passes through a series of rotor and stator blades, are meticulously calibrated to extract incremental energy from the steam as it expands and cools. Each stage is designed to handle a specific pressure and temperature range to maintain ideal efficiency throughout the expansion process.
In addition, modern high-efficiency turbines often integrate reheating systems to further improve performance. In a reheat cycle, steam is partially expanded in the high-pressure turbine, returned to the boiler for reheating, and then sent through intermediate- and low-pressure turbines. This method helps reduce moisture content in the steam during later stages of expansion, preserving blade integrity and improving energy extraction. Reheating, combined with regenerative feedwater heating, contributes to raising the overall thermal efficiency of the plant to levels approaching or even exceeding 45% in coal-fired installations and up to 60% in combined cycle gas-steam configurations.
Precision manufacturing and tight tolerances also play a significant role in turbine efficiency. Advanced fabrication techniques, including computer-controlled machining and additive manufacturing, allow for exacting designs that minimize mechanical losses, ensure optimal flow paths, and reduce clearances where leakage could occur. Turbines are often housed in multiple-cylinder configurations (high-, intermediate-, and low-pressure units), each optimized for different stages of the expansion cycle. The use of axial exhaust designs, where steam exits along the turbine axis, further enhances flow dynamics and allows for more compact, efficient condenser layouts.
Control systems contribute to efficiency by continuously adjusting turbine operation to match load demands, environmental conditions, and fuel input. Modern digital control systems monitor turbine speed, pressure, vibration, and temperature in real-time, enabling fine-tuned operation that prevents overshoot, reduces wear, and extends component lifespan. Variable inlet guide vanes, adjustable extraction valves, and automatic startup/shutdown sequences help reduce energy waste and enhance responsiveness to grid requirements.
High-efficiency steam turbines are also essential in combined heat and power (CHP) and combined cycle systems. In CHP applications, excess thermal energy from the turbine exhaust is captured and used for district heating or industrial processes, dramatically increasing total system efficiency. In combined cycle plants, steam turbines are paired with gas turbines, using the gas turbine’s exhaust heat to produce steam that drives the steam turbine—making these installations among the most efficient forms of fossil-fuel generation.
Overall, high-efficiency steam turbines are a critical solution for sustainable, cost-effective electricity production. Whether installed in large baseload coal and gas plants, nuclear power stations, or industrial CHP facilities, they provide the power sector with a reliable means of reducing emissions while meeting increasing global energy demands. Continued innovation in materials, blade design, thermal management, and digital controls promises to push efficiency even higher in the coming decades, securing steam turbines’ role as a cornerstone of modern energy infrastructure.
As the global energy sector continues to prioritize decarbonization and operational efficiency, high-efficiency steam turbines are adapting to meet the demands of a more flexible and low-carbon grid. One of the emerging trends is the integration of these turbines into hybrid systems that combine renewable sources such as solar thermal or biomass with traditional steam cycles. In concentrated solar power (CSP) plants, for example, solar energy is used to produce high-temperature steam that feeds into a turbine much like in a conventional fossil-fueled plant. The efficiency of the turbine becomes critical in determining how much of the captured solar heat is converted into usable electricity. Similarly, in biomass plants where the steam is generated by burning organic matter, using high-efficiency turbines maximizes the energy value of what are often low-energy-density fuels.
Even in conventional fossil-fueled applications, turbine designs are evolving to support partial-load efficiency and rapid cycling, which are necessary traits in modern power systems dominated by variable renewable generation. Traditional base-load plants are being re-engineered to start and stop more quickly, run efficiently at reduced loads, and handle frequent thermal cycling. Steam turbines built with modern metallurgy and stress-resistant design features can now handle these variable operating profiles with minimal degradation, allowing them to complement intermittent solar and wind energy while maintaining high net efficiency.
In addition to operational flexibility, attention is being given to the life-cycle environmental impact of steam turbine installations. Manufacturers are adopting design-for-recyclability principles and reducing the carbon footprint of turbine production itself. The materials used—such as nickel alloys, advanced steels, and high-performance ceramics—are being selected not only for performance but also for longevity and eventual recyclability. As lifecycle emissions reporting becomes more standardized in the energy industry, turbines with lower embodied carbon and longer service lives will become more favorable choices for utilities and investors.
Maintenance strategies have also undergone a transformation alongside turbine design. Instead of relying solely on time-based overhauls, operators now use condition-based and predictive maintenance systems to monitor wear patterns, temperature excursions, and vibration anomalies in real time. This proactive approach minimizes downtime and ensures turbines continue to operate at peak efficiency throughout their service lives. By using machine learning algorithms trained on historical performance and failure data, operators can schedule maintenance with greater precision, reducing unnecessary part replacements and avoiding catastrophic failures. This not only saves money but also preserves the high efficiency of the turbine over decades of operation.
Furthermore, economic factors are driving the global proliferation of high-efficiency steam turbines. As carbon pricing and emissions regulations become more stringent, plants that can deliver more electricity per unit of fuel become more financially attractive. This is particularly true in regions with aging thermal infrastructure, where repowering older plants with advanced turbines allows operators to extend the life of existing facilities while achieving modern efficiency and emissions standards. In emerging markets, where energy demand is growing rapidly and infrastructure investments must deliver long-term value, the adoption of high-efficiency turbines represents a prudent way to future-proof new capacity.
These high-efficiency turbines also play a role in strategic energy reserves and backup systems. Because of their ability to ramp up and down rapidly without large losses in efficiency, they are increasingly used in peaking and reserve power roles, supplementing grids when renewable generation falters or demand spikes unexpectedly. The precision and reliability of modern steam turbine systems ensure that they can meet these demands without compromising on emissions goals or system stability.
As the energy landscape shifts toward a more distributed, diversified, and decarbonized model, high-efficiency steam turbines are positioned not as relics of the industrial past, but as essential components of a balanced and resilient energy mix. They bridge the gap between renewable generation and the firm capacity required for grid reliability, and they do so with a continually improving balance of thermal efficiency, flexibility, and environmental performance. Innovations on the horizon—from new working fluids and closed-loop supercritical CO₂ cycles to integration with thermal energy storage—promise to further extend the capabilities of steam-based power generation. In this context, the high-efficiency steam turbine stands not as a finished product, but as an evolving technology platform with continued relevance in the pursuit of cleaner, more efficient global energy systems.
The continued relevance of high-efficiency steam turbines also lies in their adaptability to emerging fuels and decarbonized energy carriers. With the global push to reduce reliance on coal and natural gas, new efforts are exploring the use of alternative fuels such as hydrogen and ammonia in boilers and gas turbines that generate steam for power cycles. In these scenarios, the steam turbine becomes a key component in a cleaner thermal loop, where combustion emissions are drastically reduced or eliminated. For instance, hydrogen-fired boilers can produce high-temperature steam that feeds directly into advanced turbines designed to handle the specific temperature and pressure profiles of these new fuels. Although combustion of hydrogen or ammonia poses technical challenges, including flame stability and NOₓ emissions control, the steam turbine itself remains largely compatible with steam from a variety of sources, making it a flexible asset in the decarbonization roadmap.
Moreover, high-efficiency steam turbines are increasingly being used in industrial sectors beyond electricity production, including petrochemical processing, pulp and paper manufacturing, desalination, and large-scale carbon capture and storage (CCS) operations. In these applications, the turbine not only generates power but also provides mechanical drive for pumps, compressors, and other rotating machinery, or supports processes requiring high-grade thermal energy. In carbon capture plants, for example, integrating a steam turbine allows efficient recovery and reuse of thermal energy needed for solvent regeneration. The ability of steam turbines to deliver both power and process heat at various pressure levels makes them uniquely valuable in such complex, energy-intensive environments.
Another important advantage of modern steam turbines is their long operational lifespan, often exceeding 30 to 40 years with proper maintenance. This durability makes them a wise capital investment, particularly when matched with modular upgrade pathways. Many OEMs now offer retrofit and modernization packages that upgrade blade sections, seals, and control systems, effectively bringing older turbines up to current efficiency and emissions standards. These retrofits can result in efficiency gains of several percentage points, which, over the remaining life of the plant, translate to substantial fuel savings and emissions reductions. Because turbines represent a significant portion of capital expenditure in a power plant, the ability to extend their productive life is a major financial and environmental benefit.
The global expansion of district heating and cogeneration networks further underscores the strategic importance of steam turbines. In such systems, waste steam from the electricity generation process is repurposed for heating residential or industrial buildings, dramatically increasing overall system efficiency to as much as 80–90%. Steam turbines optimized for extraction or back-pressure operation are especially useful in these configurations. They allow operators to divert steam at specific pressure levels to meet heat demands without sacrificing electricity generation entirely. This dual utility not only reduces the need for separate heating infrastructure but also minimizes the environmental footprint of urban energy systems.
Education and workforce development also play a key role in the continued advancement of steam turbine technology. Universities and technical institutes around the world are training a new generation of engineers who are fluent in fluid dynamics, thermodynamics, materials science, and control systems—all vital disciplines for designing and operating high-efficiency turbines. Collaborative efforts between academia, industry, and government ensure that research findings on things like heat transfer, blade aerodynamics, and corrosion mechanisms are quickly translated into practical design improvements. As digital tools and simulation environments become more sophisticated, they allow for rapid prototyping and testing of turbine components under real-world conditions, accelerating the pace of innovation.
In summary, high-efficiency steam turbines are not only surviving but thriving as indispensable tools in the 21st-century energy landscape. Their ability to adapt to new fuels, support low-carbon technologies, integrate into industrial and urban energy systems, and deliver unmatched reliability and longevity cements their role in a sustainable energy future. Far from being a relic of the Industrial Revolution, the steam turbine is evolving with modern demands—becoming more intelligent, more efficient, and more integrated into the broader fabric of clean energy infrastructure around the world.
Innovations in Steam Turbine Blade Design
Innovations in steam turbine blade design have been pivotal in enhancing the efficiency, reliability, and operational flexibility of modern steam turbines. As turbines are expected to operate under increasingly demanding conditions—such as higher temperatures, pressures, and variable load cycles—engineers have focused on developing blades that not only withstand extreme environments but also extract more energy from the steam passing through the turbine. These innovations are grounded in advances in aerodynamics, materials science, computational modeling, and manufacturing technologies, each of which contributes to the performance and longevity of turbine blades.
One of the most significant developments in blade design has been the improvement of aerodynamic profiles. Engineers now employ sophisticated computational fluid dynamics (CFD) software to model and optimize the flow of steam across the blades. These simulations enable precise shaping of the airfoil section of each blade to minimize turbulence, reduce drag, and maximize the pressure drop across each stage. This leads to more efficient conversion of thermal energy into rotational energy. Three-dimensional blade designs, including twisted and tapered geometries, ensure optimal performance at each radial location on the rotor. These designs account for variations in steam velocity and pressure from hub to tip, resulting in improved stage efficiency and smoother flow characteristics.
Another critical area of innovation is in blade cooling and thermal management, especially in high-pressure and intermediate-pressure stages where temperatures can exceed 600°C. While blade cooling is more commonly associated with gas turbines, some advanced steam turbines also require mechanisms to reduce thermal stress and fatigue. Techniques include the use of thermal barrier coatings and internal cooling channels, which help dissipate heat more effectively. These features ensure that blades retain their mechanical integrity over long operational cycles, reducing the risk of failure and extending maintenance intervals.
The materials used in turbine blades have also undergone significant evolution. Traditional stainless steels are being replaced by high-strength alloys and advanced martensitic steels that offer superior creep resistance, fatigue strength, and corrosion resistance. For the most demanding conditions, nickel-based superalloys and single-crystal materials are being explored. These materials can maintain their strength and shape under prolonged exposure to heat and stress, which is crucial for maintaining tight clearances and minimizing blade deformation. In tandem with these materials, protective coatings such as chromium or aluminum-based films are applied to prevent oxidation and erosion caused by wet steam and contaminants.
Blade attachments and root designs have seen refinement as well. In modern turbines, blades are often attached to the rotor via fir-tree or dovetail root configurations that distribute stress evenly and allow for thermal expansion without compromising the mechanical lock. These attachment methods are designed to minimize fretting and wear, especially during startup and shutdown cycles. Blade tip seals and shrouds have also improved, reducing steam leakage around the blade tips and increasing overall stage efficiency. Some blades are connected by integral shrouds or lacing wires to suppress vibration and resonant frequencies that could lead to mechanical failure.
Another area of focus is moisture-resistant design for low-pressure blades. In the final stages of a turbine, steam expands and condenses into water droplets, which can cause erosion and pitting on blade surfaces. Modern blade profiles and surface treatments are engineered to minimize droplet impingement and reduce erosion damage. In some cases, special coatings or surface texturing is applied to resist pitting and improve water shedding. These enhancements are essential for extending blade life in wet steam environments, such as those found in nuclear or biomass-powered turbines.
Advanced manufacturing techniques have enabled many of these design innovations to be realized. Additive manufacturing (3D printing), precision casting, and five-axis CNC machining allow for the creation of complex blade geometries that were previously difficult or impossible to produce. These methods offer tighter tolerances, better material utilization, and faster prototyping, all of which contribute to improved blade quality and consistency. Furthermore, non-destructive testing and real-time inspection technologies are used during production to ensure that each blade meets exacting standards before it is installed in a turbine.
Digital design tools are also reshaping how blades are developed and validated. Through digital twins—virtual replicas of physical components—engineers can simulate real-world operating conditions and monitor blade performance over time. Data collected from sensors embedded in operating turbines feeds into these models, allowing for predictive maintenance and performance optimization. As these technologies mature, they will further reduce downtime, improve reliability, and refine the next generation of blade designs.
Collectively, these innovations in steam turbine blade design are helping operators achieve higher efficiency levels, lower operating costs, and longer service intervals. As global energy systems move toward decarbonization, high-performance blades will be critical in making steam turbines compatible with cleaner fuels and more dynamic operating modes. The future of steam turbines lies not just in larger or more powerful units, but in the meticulous design and performance of each blade—ensuring that every rotation of the rotor delivers maximum value with minimum loss.
The evolution of steam turbine blade design continues to be shaped by the dual pressures of performance demands and environmental responsibility. One emerging area is the application of smart blade technology, where sensors are embedded directly into the blade material to monitor parameters like temperature, stress, vibration, and strain in real time. These embedded sensors allow operators to detect early signs of material fatigue, unbalanced loads, or thermal distortion long before they become critical. By enabling continuous health monitoring of individual blades, this technology reduces the reliance on scheduled maintenance and shifts focus toward condition-based interventions, which are more cost-effective and minimize downtime. The information gathered from smart blades can also feed into AI-driven analytics platforms that predict failures or performance drops, allowing for intelligent maintenance planning across entire fleets of turbines.
Another promising innovation lies in adaptive blade geometry. While fixed-blade designs have served well for over a century, research is progressing into blades that can alter their shape slightly in response to changing load conditions or steam velocities. Using materials with shape-memory characteristics or incorporating small actuators, these blades could adapt their camber or angle of attack to optimize steam flow at different operating points. Although still largely in the experimental stage, adaptive blades represent a step toward more responsive and versatile turbine systems, capable of maintaining high efficiency across a wider load range.
As global power systems move toward more flexible generation to accommodate variable renewables like solar and wind, the ability of steam turbines to operate efficiently at part load becomes increasingly important. Blade design innovations that reduce losses during partial-load operation are crucial. For example, variable stator vanes upstream of the rotating blades can adjust the steam flow path to maintain optimal angles of incidence, reducing flow separation and energy dissipation. These vane and blade configurations are refined using advanced modeling techniques that account for fluid-structure interaction and transient heat transfer, ensuring stable operation under highly dynamic conditions.
In parallel, sustainability goals are influencing blade design from a life-cycle perspective. Turbine manufacturers are beginning to consider the recyclability of blade materials, ease of disassembly, and reduction in rare or hazardous substances. New alloy compositions are being developed that maintain high performance while being easier to recycle or process at end-of-life. In addition, innovations in blade coatings are reducing the need for frequent replacement. Self-healing or erosion-resistant coatings extend the operational life of blades in wet steam and contaminated environments, significantly reducing the material waste and emissions associated with maintenance and replacements.
High-fidelity digital simulation continues to push blade design to new limits. Virtual prototyping allows for exhaustive testing of new blade profiles, materials, and stress responses under a wide range of simulated conditions. These digital environments can model everything from steam flow turbulence to thermal gradients and creep over decades of simulated operation. Combined with rapid prototyping technologies like direct metal laser sintering or electron beam melting, this simulation-to-production pipeline shortens the development cycle and allows for custom blades tailored to specific plant conditions. Customization, in turn, leads to marginal but cumulatively significant efficiency gains when scaled across multiple turbines or a large generating fleet.
The development of ultra-supercritical and advanced ultra-supercritical steam cycles has also intensified the focus on blade design. These cycles operate at pressures above 250 bar and temperatures exceeding 600°C, conditions that push blade materials and geometries to their limits. Blades must resist not only high thermal stress but also oxidation, creep, and fatigue over long periods. This has led to the adoption of forged and directionally solidified materials that maintain grain structures aligned with primary stress paths, offering superior resistance to failure. In some cases, hollow blades with internal channels have been considered for steam turbines—mimicking successful designs in gas turbines—to manage thermal expansion and improve strength-to-weight ratios.
Ultimately, the steam turbine blade is no longer a simple mechanical component but a highly engineered, multifunctional system optimized for aerodynamics, thermal resilience, material science, and data integration. It embodies the convergence of traditional mechanical engineering with modern computational tools, smart materials, and digital diagnostics. As energy systems around the world continue to evolve, the steam turbine blade will remain at the heart of this transformation—quietly turning under high pressure and temperature, extracting every possible unit of work from the steam that drives it, and doing so with a level of intelligence, efficiency, and durability never before imagined.
Looking forward, one of the most intriguing frontiers in steam turbine blade design is the incorporation of bio-inspired engineering—designs modeled after structures and systems found in nature. For example, engineers are exploring blade surfaces with micro-textures or riblets modeled after shark skin, which have been shown to reduce drag in fluid flow. These microstructures can guide steam flow more efficiently across the blade surface, minimizing boundary layer separation and improving aerodynamic performance. In turbulent sections of the turbine, such passive flow control methods offer a low-cost way to enhance efficiency without adding mechanical complexity.
Another nature-inspired innovation is the use of fractal geometries in blade trailing edges or shroud configurations. These complex patterns can help dissipate energy more smoothly, reducing wake losses and suppressing noise—a benefit in both operational efficiency and environmental compliance. While these concepts were once considered too intricate for practical manufacturing, modern additive manufacturing now makes it feasible to produce them with high precision and consistency.
Advancements in nanotechnology are also beginning to impact blade surfaces. Nano-coatings engineered to repel water and dirt can help mitigate erosion and fouling in low-pressure blades where steam starts to condense. These coatings not only preserve blade integrity but also maintain a smooth surface for optimal steam flow. Self-cleaning and anti-corrosive properties are particularly valuable in environments where the steam contains dissolved salts or impurities, such as in geothermal applications or biomass-fueled plants.
From a systems perspective, blade design is becoming increasingly integrated into holistic turbine optimization. Instead of designing blades in isolation, they are now co-optimized with casing geometry, shaft dynamics, steam path layouts, and even the control logic that governs steam admission and extraction. This whole-system approach ensures that every element works synergistically, avoiding mismatches between blade performance and overall turbine behavior. It also allows for more efficient integration with plant-level systems, such as heat recovery loops, condensers, and district heating networks.
Digital twin technology is further closing the gap between blade design and real-world performance. By creating a virtual replica of each blade that updates continuously based on sensor data, engineers can monitor how minor manufacturing deviations or site-specific operating conditions affect performance over time. This dynamic modeling allows for fine-tuning operational parameters like inlet pressure or load dispatch strategies to keep turbines running at peak efficiency. In the future, it’s conceivable that turbines will use machine learning to adjust blade angles or valve positions in real time based on live performance feedback—effectively turning a passive mechanical system into an adaptive energy machine.
Finally, the push for carbon neutrality is shaping how steam turbine blades are assessed from a sustainability standpoint. Environmental impact assessments now factor in not only operational emissions but also the embodied carbon in manufacturing, transporting, and maintaining turbine components. This has led to efforts to localize blade production using regional supply chains and low-emission manufacturing techniques. Lifecycle analysis is guiding choices in materials and coatings, helping designers select options that balance performance with environmental responsibility. These shifts are aligning steam turbine technology with the broader goals of the energy transition, ensuring that even the most advanced machinery contributes to a more sustainable energy future.
In essence, the future of steam turbine blade design is a convergence of precise engineering, smart data systems, and ecological awareness. Blades will continue to evolve from purely mechanical parts into intelligent, adaptive, and environmentally conscious components that play a vital role in the global energy system. As the world demands cleaner, more flexible power generation, it is the refinement of these seemingly simple components—the steam turbine blades—that will quietly enable some of the biggest leaps in efficiency, reliability, and sustainability.
EMS Power Machines
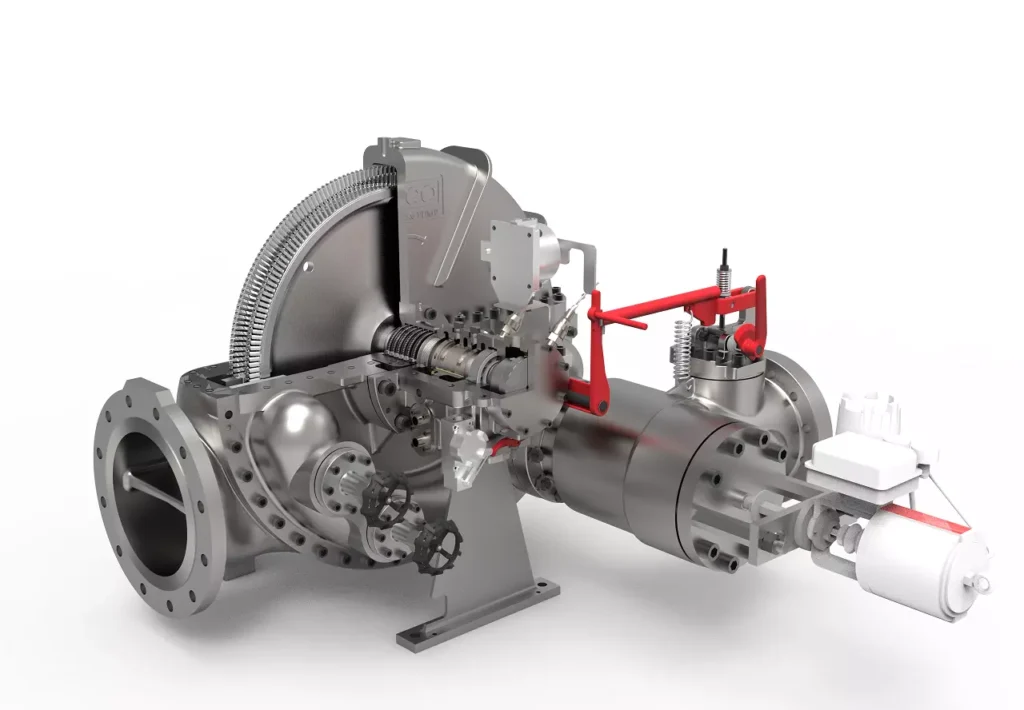
We design, manufacture and assembly Power Machines such as – diesel generators, electric motors, vibration motors, pumps, steam engines and steam turbines
EMS Power Machines is a global power engineering company, one of the five world leaders in the industry in terms of installed equipment. The companies included in the company have been operating in the energy market for more than 60 years.
EMS Power Machines manufactures steam turbines, gas turbines, hydroelectric turbines, generators, and other power equipment for thermal, nuclear, and hydroelectric power plants, as well as for various industries, transport, and marine energy.
EMS Power Machines is a major player in the global power industry, and its equipment is used in power plants all over the world. The company has a strong track record of innovation, and it is constantly developing new and improved technologies.
Here are some examples of Power Machines’ products and services:
- Steam turbines for thermal and nuclear power plants
- Gas turbines for combined cycle power plants and industrial applications
- Hydroelectric turbines for hydroelectric power plants
- Generators for all types of power plants
- Boilers for thermal power plants
- Condensers for thermal power plants
- Reheaters for thermal power plants
- Air preheaters for thermal power plants
- Feedwater pumps for thermal power plants
- Control systems for power plants
- Maintenance and repair services for power plants
EMS Power Machines is committed to providing its customers with high-quality products and services. The company has a strong reputation for reliability and innovation. Power Machines is a leading provider of power equipment and services, and it plays a vital role in the global power industry.
EMS Power Machines, which began in 1961 as a small factory of electric motors, has become a leading global supplier of electronic products for different segments. The search for excellence has resulted in the diversification of the business, adding to the electric motors products which provide from power generation to more efficient means of use.