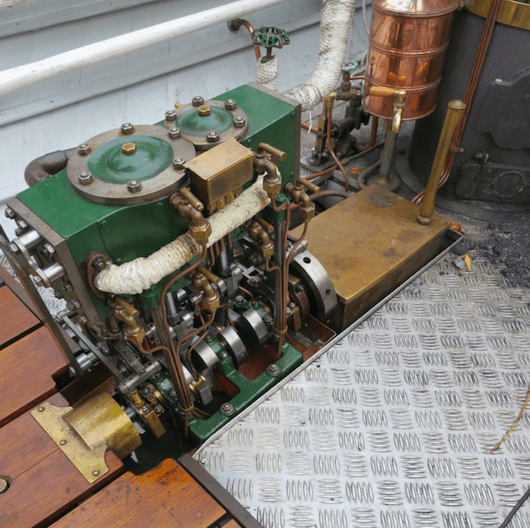
Buy or Lease Steam Engines for Your Project: A steam engine is a heat engine that performs mechanical work using steam as its working fluid. It was one of the key technologies driving the Industrial Revolution and laid the groundwork for modern mechanical engineering and thermodynamics.
Basic Working Principle
Steam engines operate on the Rankine cycle, where water is heated to create steam, which expands to perform mechanical work, and is then condensed back into water.
Key Steps:
- Boiling Water: Heat is applied to water in a boiler, producing high-pressure steam.
- Steam Expansion: Steam enters the engine’s cylinder or turbine, pushing a piston or turning a shaft.
- Exhaust and Condensation: The steam is then exhausted and typically condensed for reuse.
Main Components
- Boiler: Heats water to produce steam.
- Cylinder & Piston (or Turbine Blades): Where the steam does mechanical work.
- Condenser: Cools exhaust steam to water for reuse.
- Flywheel: Regulates engine speed and smooths power output.
- Valve Gear: Controls steam admission and exhaust.
Types of Steam Engines
a. Reciprocating Steam Engines
- Use pistons moving in cylinders.
- Common in early locomotives and stationary engines.
b. Steam Turbines
- Steam turns a rotor with blades.
- More efficient; used in power plants and ships.
c. Condensing vs. Non-Condensing
- Condensing Engines: Use condensers to improve efficiency.
- Non-Condensing (Back-Pressure) Engines: Exhaust steam is used for heating or industrial processes.
Historical Context
- 1712: Thomas Newcomen builds the first practical steam engine.
- 1769: James Watt improves efficiency with a separate condenser and rotary motion.
- 1800s: Steam powers factories, trains, ships, and early cars.
Advantages
- High torque at low speeds.
- Can use various heat sources (coal, biomass, nuclear, etc.).
- Suitable for large-scale power generation.
Limitations
- Lower efficiency compared to internal combustion engines or electric motors.
- High maintenance requirements.
- Bulky and heavy, especially reciprocating types.
Modern Applications
- Power Plants: Steam turbines generate most of the world’s electricity.
- Cogeneration Systems: Use back-pressure turbines for both electricity and process heat.
- Historic and Niche Uses: Steam locomotives (tourism), hobby engines, and educational purposes.
Recent Innovations
- Supercritical Steam Technology: Operates at higher pressures and temperatures for greater efficiency.
- Combined Heat and Power (CHP): Systems recover and use waste heat.
- Solar Thermal Plants: Use mirrors to heat water into steam.
Steam engines are heat engines that convert thermal energy from steam into mechanical work. They played a pivotal role during the Industrial Revolution, transforming industries, transportation, and manufacturing by providing a reliable and powerful source of energy. The fundamental principle behind a steam engine is the Rankine cycle. In this cycle, water is heated in a boiler to produce high-pressure steam. This steam then expands and pushes against mechanical components—typically pistons in early engines or turbine blades in modern ones—causing motion. After the steam has done its work, it is exhausted and, in many systems, condensed back into water to be reused.
The earliest steam engines were reciprocating engines, where steam pushed a piston back and forth within a cylinder. These pistons were connected to a crankshaft, converting linear motion into rotary motion suitable for driving machinery or wheels. James Watt made significant improvements to this design in the 18th century, including the addition of a separate condenser and the development of rotary motion capability, which greatly increased efficiency and utility.
As technology progressed, steam turbines became more prominent, especially in power generation. Unlike reciprocating engines, steam turbines use high-pressure steam to spin a rotor with attached blades. This method is smoother, more efficient, and better suited for continuous power production. Today, steam turbines remain central to electricity generation, particularly in thermal power plants that use fossil fuels, nuclear energy, or solar thermal energy.
Steam engines consist of several critical components: the boiler, which heats water; the cylinder or turbine, where steam does mechanical work; the condenser, which recaptures steam as water; and the valve gear, which controls the flow of steam. Flywheels and governors are used to regulate speed and maintain consistent operation.
There are two main categories of steam engines—condensing and non-condensing. Condensing engines use a condenser to create a vacuum that improves efficiency by lowering the exhaust pressure. Non-condensing engines, also known as back-pressure engines, exhaust steam at a pressure above atmospheric and are often used where the exhaust steam’s heat can be recovered for other purposes like heating or drying in industrial processes.
Despite their historical importance, steam engines have been largely replaced in transportation and small-scale applications by internal combustion engines and electric motors. However, they remain vital in large-scale power generation. Innovations such as supercritical and ultra-supercritical steam cycles have significantly improved efficiency by operating at higher temperatures and pressures. Additionally, combined heat and power systems and solar thermal technologies continue to use steam as a versatile and effective energy carrier.
Steam engine technology, though centuries old, remains a cornerstone of modern energy systems, evolving with new materials and engineering techniques to meet today’s energy challenges.
Steam engine technology is based on converting heat energy from steam into mechanical work. At its core, the system operates by heating water in a boiler to create steam, which then expands and exerts pressure. This pressurized steam is directed into a cylinder or turbine, where it pushes a piston or spins a rotor, creating motion that can be used to power machinery, vehicles, or generators. Once the steam has done its work, it is released—either into the atmosphere in simple systems or into a condenser where it’s cooled and turned back into water for reuse in more efficient setups.
The earliest practical steam engines were developed in the early 18th century, with Thomas Newcomen’s atmospheric engine being among the first. James Watt later introduced key improvements like the separate condenser and rotary motion, making steam engines more efficient and adaptable to industrial needs. These innovations allowed steam power to become the driving force behind the Industrial Revolution, revolutionizing manufacturing, mining, and transportation.
There are two main types of steam engines: reciprocating and turbine-based. Reciprocating engines use pistons that move back and forth, while turbines use high-speed rotating blades. Turbines are generally more efficient and are the standard in modern power plants. Depending on how steam is handled after expansion, steam engines can also be categorized as condensing or non-condensing. Condensing engines reuse steam by converting it back to water, improving efficiency. Non-condensing engines (also called back-pressure engines) release steam for heating or other industrial uses.
Steam engine systems include several key components: the boiler to generate steam, the engine or turbine to convert pressure into motion, a condenser (in closed-loop systems) to turn steam back into water, and various control mechanisms like valves, governors, and regulators to manage flow, pressure, and speed. Over time, these systems have been refined to handle higher pressures and temperatures, leading to better performance and lower fuel consumption.
Even though steam engines are no longer common in vehicles or small-scale use, they remain essential in power generation. Modern thermal power plants, whether fueled by coal, natural gas, nuclear reactions, or solar heat, often use steam turbines to generate electricity. Advances such as supercritical and ultra-supercritical steam cycles allow these plants to operate at higher efficiencies by using higher temperatures and pressures.
Steam technology also plays a role in cogeneration (combined heat and power systems), where steam produced for electricity generation is also used for industrial processes or space heating. In renewable energy, solar thermal power plants use concentrated sunlight to produce steam, offering a clean, sustainable alternative to fossil fuels.
In essence, steam engine technology is a foundational element of industrial engineering, and while its role has shifted, it continues to evolve and support critical infrastructure around the world.
Innovative Steam Engine Designs for Modern Applications
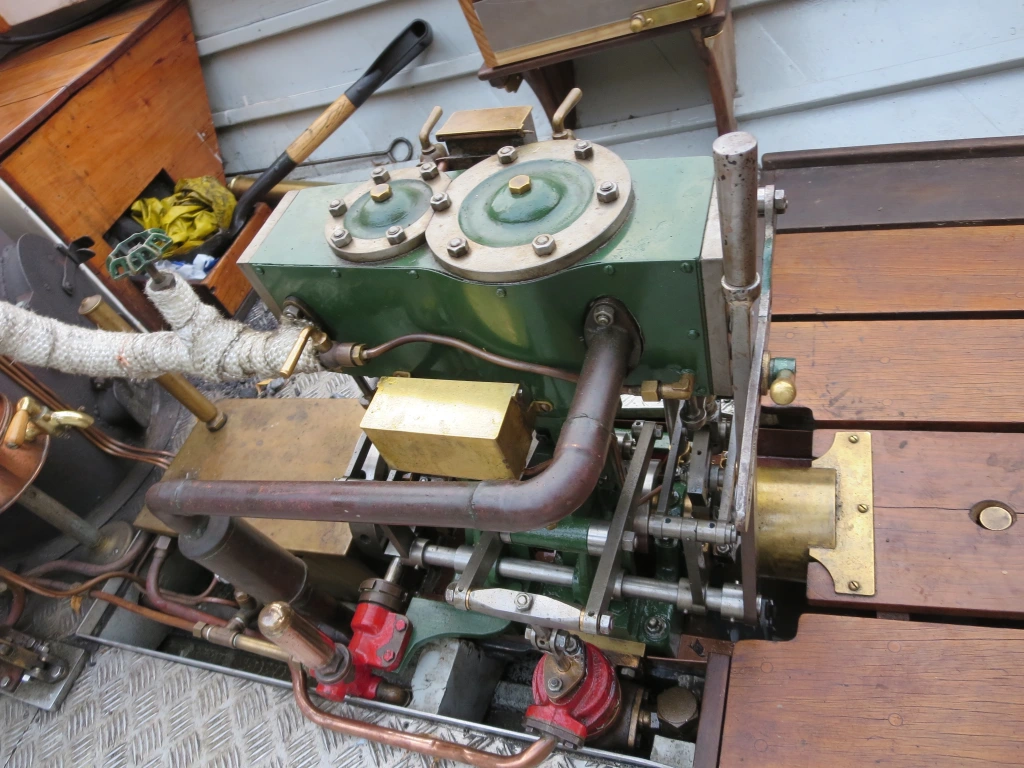
Innovative steam engine designs for modern applications focus on enhancing efficiency, sustainability, and versatility. While traditional steam engines laid the foundation for industrialization, contemporary designs adapt the core principles to meet current energy demands and environmental standards.
Modern steam engines are being reimagined with advanced materials, precision engineering, and integration into hybrid and renewable systems. One area of innovation is supercritical and ultra-supercritical steam cycles, which operate at temperatures and pressures beyond the critical point of water. These systems dramatically increase thermal efficiency in power plants, often exceeding 45%, and are commonly used in coal and nuclear power generation. Their advanced metallurgy and heat-resistant components allow for sustained operation under extreme conditions.
Compact steam engines are another area of exploration, especially in off-grid and portable energy systems. Small-scale steam engines designed for biomass or solar thermal inputs are being tested for rural electrification and micro-grid setups. These engines use organic Rankine cycles (ORC), where organic fluids with lower boiling points than water allow energy recovery from low-grade heat sources, such as industrial waste heat or geothermal energy. ORC-based engines can generate electricity where conventional systems would be inefficient or unfeasible.
Waste heat recovery steam engines are increasingly popular in industrial environments. These systems capture residual heat from manufacturing, internal combustion engines, or other thermal processes to generate steam and produce additional electricity. By converting otherwise lost energy into usable power, these systems reduce fuel consumption and emissions, contributing to sustainability goals.
In transportation, while steam power is largely obsolete in mainstream vehicles, niche applications are seeing a resurgence. Engineers and enthusiasts have developed modern steam-powered cars and motorcycles with closed-loop systems, rapid-start boilers, and computer-controlled valve timing. These prototypes often run on cleaner fuels and promise quiet operation, high torque, and reduced emissions, although they remain largely experimental.
Innovative designs also explore solar steam engines, which use concentrated solar power (CSP) systems to heat water and generate steam. These engines are used in regions with high solar irradiance for decentralized power generation and desalination. Some systems store thermal energy in molten salts, allowing for electricity production even after sunset—a key advantage over photovoltaic solar.
Another forward-thinking approach involves combined heat and power (CHP) systems using back-pressure steam turbines. These setups generate electricity while simultaneously providing steam or hot water for industrial processes, district heating, or building climate control. By utilizing the full energy content of the fuel, CHP systems can achieve efficiencies up to 80%.
Material science is also contributing to innovation. The development of ceramic coatings, corrosion-resistant alloys, and nano-structured surfaces helps steam engines operate at higher pressures and temperatures with reduced wear. Precision control systems and digital monitoring further enhance reliability, safety, and performance.
Although internal combustion engines and electric motors dominate most sectors, innovative steam engine designs still offer unique advantages in specific applications—especially where heat is abundant or where integrated thermal-electric solutions are needed. As the world shifts toward cleaner energy, steam technology, reinvented through modern engineering, continues to play a relevant and adaptive role.
Innovative steam engine designs today are reshaping how we think about this centuries-old technology by integrating modern materials, precision control, and alternative energy sources. Unlike the bulky and maintenance-heavy engines of the past, current designs are compact, efficient, and often tailored for specific roles in energy systems that prioritize sustainability and resource optimization. One of the most significant advances comes from the use of supercritical and ultra-supercritical steam cycles in power plants. These systems push water past its critical point, allowing for significantly higher efficiencies compared to traditional steam cycles. They operate at extreme temperatures and pressures, made possible through advances in metallurgy and thermal management, making them central to modern coal, gas, and nuclear plants striving to minimize fuel consumption and carbon emissions.
At smaller scales, engineers are developing steam engines for decentralized energy production, particularly in rural or off-grid areas. These compact engines often run on biomass or solar heat and are designed to generate electricity and useful thermal energy simultaneously. By using the organic Rankine cycle, which employs fluids with lower boiling points than water, these systems can extract energy from relatively low-temperature heat sources—such as industrial waste heat, geothermal sources, or solar collectors—turning otherwise wasted heat into productive power. The flexibility of these systems makes them ideal for remote communities, agricultural applications, or industrial facilities looking to improve energy efficiency and reduce reliance on fossil fuels.
In industrial settings, the concept of waste heat recovery has become a major driver of innovation in steam technology. Instead of allowing heat from engines, furnaces, or other processes to dissipate, new steam engine systems capture and convert it into usable energy. These setups not only enhance overall efficiency but also contribute to lower greenhouse gas emissions by making the most out of every unit of fuel. Some systems are even designed to retro-fit existing plants, offering a cost-effective path to greater energy sustainability.
While steam has largely disappeared from modern vehicles, enthusiasts and engineers continue to explore advanced steam-powered transportation. Some experimental cars and motorcycles now incorporate flash boilers, closed-loop systems, and digital controls, allowing for rapid startup times and precise steam flow management. These vehicles produce high torque with smooth, quiet operation and have potential niche uses in areas where emissions and noise are critical concerns. Though not likely to replace internal combustion or electric engines broadly, they highlight how steam can still compete when designed with today’s tools and expectations.
Another area of growth lies in solar thermal applications. Solar steam engines harness the power of concentrated sunlight to produce steam for electricity generation, water purification, or heating. Some systems use parabolic mirrors or heliostats to focus sunlight onto a central receiver, where water is heated to generate steam. With thermal storage mediums like molten salt, these plants can operate even when the sun isn’t shining, addressing the intermittency that plagues other renewable sources. This makes them especially useful in sunny regions where grid infrastructure is limited or where reliable clean energy is needed.
The integration of modern materials such as advanced ceramics, corrosion-resistant alloys, and nanostructured surfaces has greatly improved the durability and thermal performance of steam engine components. These materials can withstand the high temperatures and pressures necessary for modern applications while reducing maintenance needs and extending service life. Alongside this, computerized control systems have become standard in new designs, allowing real-time monitoring of pressure, temperature, flow rates, and efficiency metrics. Automation ensures optimal operation, safety, and adaptability to changing conditions, whether in a power plant or a micro-generator.
Altogether, steam engine innovation today reflects a blend of old principles and new possibilities. While it may not dominate headlines, this quiet reinvention continues to influence sectors ranging from renewable energy to industrial sustainability. As global demand for cleaner and more efficient energy grows, modern steam technology—refined, repurposed, and reinvented—remains an essential part of the solution.
As the world pivots toward sustainable and efficient energy solutions, steam technology continues to evolve in ways that complement modern environmental goals. A major trend is the development of hybrid systems, where steam engines are integrated with renewable energy sources like wind, solar, and geothermal. For example, some solar thermal power plants use steam engines not just for electricity generation but also for thermal storage, enabling 24/7 operation. The stored heat from the day is converted into steam and used to generate power during the night or on cloudy days, solving the intermittency issue that affects other renewable sources like solar and wind.
In industrial applications, steam engines are increasingly part of combined heat and power (CHP) systems, where the same steam is used for both generating electricity and providing heat to nearby facilities or homes. This dual-use approach enhances overall efficiency, often achieving efficiencies upwards of 80%, compared to the 30-40% typical of separate electricity and heating systems. It’s especially relevant in sectors like manufacturing, where excess heat is a byproduct of production processes that would otherwise go to waste. By capturing this heat and converting it into useful power, these systems help reduce both fuel consumption and environmental impact.
Waste heat recovery is also becoming more sophisticated with steam engines that can capture heat from a broader range of industrial sources. Advanced steam cycles, using materials designed for higher temperatures, can harness low-grade heat that was once considered unusable. These systems are particularly valuable in heavy industries such as steel, cement, and glass manufacturing, where large amounts of heat are generated. By converting this waste heat into power, these industries can significantly reduce energy costs while minimizing their carbon footprint.
Furthermore, small-scale steam engines using biomass or organic waste as fuel are gaining traction in rural areas and developing economies. Biomass-fueled steam engines can power isolated communities, small farms, or remote industries by providing both electricity and heat from locally available resources like wood, agricultural residue, or even waste from food production. These localized, renewable solutions help reduce dependence on imported fuels and offer a more sustainable path to rural electrification and economic development.
In the transportation sector, while the widespread use of steam engines in vehicles has long since passed, there are still niches where steam-powered designs are seeing renewed interest. Modern steam-powered boats, trains, and vehicles are being developed by enthusiasts and researchers looking to harness steam’s high torque and relatively simple mechanical design. These systems are increasingly powered by alternative fuels like biodiesel or ethanol, reducing the environmental impact associated with fossil fuels. While these applications remain experimental, they demonstrate the continuing potential of steam technology in settings that demand low emissions, quiet operation, or retrofitting older systems.
Another promising innovation lies in micro-scale steam engines, which can be used in residential and small-scale commercial applications. These engines often pair with small-scale biomass boilers or geothermal systems to provide off-grid power and heating. Micro steam turbines can generate power from small heat sources like wood stoves or waste heat from industrial processes, offering an efficient solution for decentralized energy production. As advancements in materials and digital control systems make these engines smaller, more efficient, and more cost-effective, they could be a viable option for areas where grid access is limited or unreliable.
The future of steam engine technology looks increasingly connected and intelligent. Smart systems that monitor and optimize performance in real-time, predicting maintenance needs and adjusting to changes in load or fuel type, are already becoming a standard in many modern applications. The integration of Internet of Things (IoT) sensors and artificial intelligence (AI) allows for better energy management, ensuring that steam engines are operating at peak efficiency while minimizing waste. These smart systems can adapt to changes in energy demand or supply conditions, improving the reliability and cost-effectiveness of steam-driven power systems.
Additionally, nanotechnology and advanced coatings are enabling steam engines to work in more demanding environments. Nanomaterials can significantly enhance the heat resistance, corrosion resistance, and thermal efficiency of steam engine components, ensuring that these systems can operate at higher temperatures and pressures for longer periods without degradation. This opens up possibilities for high-performance steam engines in power plants, industrial applications, and renewable energy systems that require a more durable and efficient approach.
With all of these advancements, it’s clear that while steam engines may have been an invention of the 18th century, their modern evolution shows that the technology still has a vital role to play in the future of energy generation. By combining time-tested principles with innovative materials, digital control systems, and alternative fuels, steam technology is not only adapting to the needs of modern industry but is also being reborn as a cornerstone of a more sustainable, efficient, and energy-conscious world.
Steam Engine Manufacturing Process
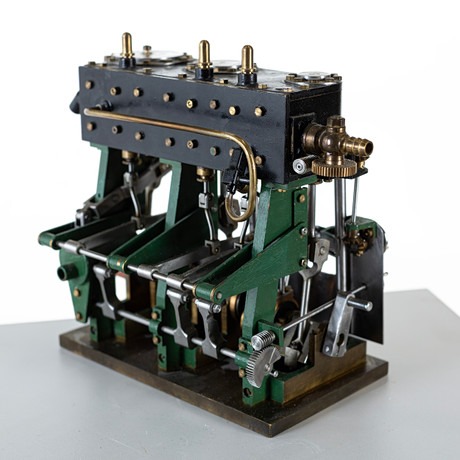
The manufacturing process of steam engines involves several stages, from the initial design and material selection to the final assembly and testing. Modern steam engines, whether used in power plants, industrial settings, or experimental designs, require precise engineering and high-quality materials. Below is a breakdown of the general process for manufacturing a steam engine:
1. Design and Engineering
The first step in manufacturing a steam engine is the design phase, where engineers and designers develop detailed plans and blueprints. This involves:
- Selecting the type of steam engine: Deciding whether it will be a reciprocating engine, a steam turbine, or another variation. The application of the engine (power generation, industrial use, transportation) will largely dictate the design.
- Engine parameters: Defining critical specifications, including the cylinder size, pressure, temperature, efficiency, and fuel type.
- Material selection: Choosing suitable materials for various components like the boiler (which must withstand high pressure), turbine blades, pistons, valves, and exhaust systems. High-strength alloys, corrosion-resistant materials, and heat-resistant coatings are commonly used.
- Computational fluid dynamics (CFD): Using simulations to optimize steam flow, heat transfer, and the mechanical motion of moving parts.
2. Material Procurement
Once the design is finalized, the required materials are sourced. The materials needed for steam engine construction include:
- Metals: Steel, cast iron, and special alloys for durability and strength under high-pressure conditions.
- Boiler materials: Copper, stainless steel, and alloys with high resistance to corrosion and heat.
- Heat-resistant coatings: These are used on turbine blades and other high-heat areas.
- Seals and gaskets: Made of rubber, synthetic materials, or metal, designed to prevent leaks in high-pressure steam systems.
3. Component Fabrication
The manufacturing process for the various steam engine components involves specialized fabrication techniques:
- Boiler Construction: Boilers are typically made from thick steel plates. The plates are cut, shaped, and welded together. Special attention is given to the integrity of the welds and the thickness of the walls to ensure they can withstand high pressures and temperatures. Pressure tests are conducted to verify the strength of the boiler.
- Cylinder and Piston Manufacturing: Cylinders are typically made from steel and are precision-machined to ensure a perfect fit with the pistons. Pistons are often cast and then machined to exact specifications. The cylinders are honed to a smooth finish to reduce friction and prevent leaks. The piston and cylinder are the heart of reciprocating steam engines, and their accurate alignment is crucial.
- Turbine Manufacturing: In the case of steam turbines, the manufacturing process is more complex. Turbine blades are made from high-strength alloys, often with a turbine casing made of a heat-resistant material. The blades are carefully shaped, and the turbine rotor is balanced to ensure smooth operation at high speeds.
- Valve Gear and Control Systems: The valves that regulate steam flow are precisely engineered and often made from high-strength materials like stainless steel. The valve gear is assembled with gears, shafts, and actuators that allow for the controlled admission and exhaust of steam at the correct times.
4. Precision Machining and Assembly
After all the components are fabricated, they undergo precision machining to ensure the correct fit and alignment. This is especially important for components like pistons, cylinders, valves, and turbine blades. CNC (Computer Numerical Control) machines are used to mill, grind, and drill components with extreme accuracy. The machining process includes:
- Cylindrical machining for the pistons and cylinders.
- Surface grinding for creating smooth, tight-fitting components.
- Heat treatment for hardening certain parts, such as valves and turbine blades, to increase their resistance to wear.
Once the individual components are manufactured, the engine begins the assembly process:
- Boiler and Pressure System Assembly: The boiler is connected to the pressure system, including pipes, valves, and safety mechanisms.
- Turbine or Cylinder Assembly: In steam turbines, the turbine blades are mounted on the rotor, and the casing is assembled. For reciprocating engines, the cylinder, piston, connecting rods, and crankshaft are assembled.
- Valve and Governor Systems: The valve gear, which regulates the admission of steam into the cylinder or turbine, is installed. A governor is also set up to regulate engine speed and maintain stable operation.
5. Welding and Joining
Various components of the steam engine are welded or bolted together to create a strong and leak-free structure:
- Welding: Especially important in boiler construction, where high-pressure components must be joined securely.
- Bolting and fasteners: Used for assembling parts that may require periodic disassembly, such as the turbine casing, pistons, and valve systems.
- Sealing: Gaskets and seals are used between mating surfaces to prevent steam leaks and maintain pressure integrity.
6. Testing and Calibration
Once the steam engine is fully assembled, it undergoes a series of rigorous tests:
- Pressure testing: The boiler and pressure system are subjected to high-pressure steam to ensure they can withstand the operational conditions without leaking or failing.
- Steam flow testing: For turbines and reciprocating engines, the flow of steam through the system is measured to ensure efficiency and correct operation. This may involve testing the power output, torque, and RPM.
- Efficiency testing: Efficiency is tested by monitoring fuel consumption, steam output, and overall performance under varying load conditions. Any inefficiencies are noted and adjustments are made.
- Safety checks: Steam engines are tested for safety, including the function of pressure relief valves, temperature sensors, and automatic shutdown systems.
7. Final Adjustments
After testing, any necessary adjustments are made to improve performance or resolve issues identified during testing. This may involve:
- Adjusting valve timing or steam flow for optimal performance.
- Fine-tuning governor settings to maintain constant speed.
- Replacing seals or gaskets if leaks are detected.
8. Painting and Coating
Once the engine is fully assembled and tested, it may undergo a final painting or coating process to protect against corrosion and give it an aesthetic finish. This is particularly important for external parts exposed to high temperatures or the elements, such as the boiler and turbine housing.
9. Installation and Commissioning
The steam engine is then transported to its installation site (such as a power plant, factory, or ship). Commissioning involves setting up the engine in its final location, connecting it to the power grid, and running a final round of operational tests. Engineers ensure that all connections are secure, safety systems are operational, and the engine is ready for long-term operation.
This entire manufacturing process requires a high level of precision, quality control, and specialized knowledge to ensure the engine performs safely and efficiently over a long lifespan. Modern steam engines are a result of years of engineering advancements and careful material selection, aimed at improving efficiency and minimizing the environmental impact of steam power.
The manufacturing process of steam engines is a complex and highly detailed procedure that blends traditional craftsmanship with modern technology. After the design phase is completed, the process begins with the procurement of materials suited to the specific needs of each component. Steel, cast iron, and high-strength alloys are essential for parts exposed to high pressure and heat, while specialized metals like copper and stainless steel are often used for areas like the boiler or components in direct contact with steam.
Once materials are sourced, the individual components are fabricated using various techniques. For example, boilers, which are central to steam engine operation, are constructed by carefully shaping and welding thick steel plates together. These plates are then subjected to rigorous pressure testing to ensure they can withstand the intense stress they will endure during operation. Precision machining is essential for the production of pistons, cylinders, and turbine blades. Each part is carefully shaped, drilled, and polished to exact specifications, often using computer numerical control (CNC) machinery to achieve the necessary level of accuracy. Components like cylinders are honed to achieve a smooth surface that minimizes friction, while pistons are often ground to ensure they fit precisely within their corresponding cylinders.
For turbine-based engines, the manufacturing process is even more intricate. Turbine blades, which are exposed to extreme temperatures, are often made from high-strength alloys that can endure the stress of high-speed rotation. These blades are shaped and tested for optimal aerodynamics and resistance to thermal expansion. The turbine rotor, which spins under the pressure of steam, is carefully balanced to prevent vibrations that could reduce performance or cause damage.
Once all parts are fabricated, the engine is assembled, starting with the installation of the boiler and pressure system. The boiler is connected to a complex network of pipes, valves, and pressure relief systems designed to maintain the integrity of the steam engine under high-pressure conditions. After the boiler is installed, components like the cylinder, piston, and turbine are put together, with each part carefully aligned to ensure smooth motion and efficiency. The valve gear, responsible for controlling the flow of steam into the engine, is also carefully installed and calibrated. A governor is added to regulate the engine’s speed, ensuring that it operates within the desired range.
Next, various components are welded or bolted together, with special attention given to sealing systems that prevent steam leaks. Gaskets and seals are used between joints to ensure a tight fit, which is crucial for maintaining pressure within the engine. These seals are often made from high-quality synthetic materials or rubber that can withstand the heat and pressure generated by the steam.
Once the engine is fully assembled, a series of testing procedures are conducted to ensure it meets safety and performance standards. Pressure tests are performed on the boiler and pressure system to check for leaks or weaknesses. The engine is then tested for efficiency, with steam flow and power output carefully monitored. Any inefficiencies or problems that arise during testing are addressed, whether it’s adjusting valve timing, optimizing steam flow, or fine-tuning the engine’s control systems.
After all tests are completed and any necessary adjustments are made, the engine undergoes a final round of checks, including safety inspections and fine-tuning of the governor system. The engine is then painted or coated to protect it from corrosion, particularly on components that are exposed to the elements, such as the boiler casing or the turbine housing. This coating helps ensure that the engine can operate effectively for many years, even in harsh environmental conditions.
Finally, the steam engine is transported to its installation site, where it undergoes commissioning. During commissioning, the engine is connected to its final infrastructure, whether it’s a power grid, industrial plant, or transportation system. A final set of operational tests is run to ensure that the engine functions as expected and is ready for long-term operation. Throughout this entire process, precision, attention to detail, and adherence to safety standards are paramount to producing a steam engine that performs efficiently and reliably under the demanding conditions it was designed for.
Once the steam engine is installed and commissioned, it enters its operational phase, where ongoing monitoring and maintenance become crucial for ensuring long-term performance. During its operation, the engine’s performance is continuously assessed through various parameters, such as temperature, pressure, steam flow, and power output. Modern steam engines often incorporate advanced sensors and control systems that allow operators to track these metrics in real time, providing a level of precision and reliability that earlier steam engines lacked.
In power plants or industrial applications, steam engines may operate continuously, so regular maintenance schedules are implemented to prevent wear and ensure the engine stays in peak condition. The steam boiler, which operates under high pressure and heat, is particularly prone to scaling, corrosion, and wear, which is why regular inspections are necessary. Boiler tubes are inspected for signs of erosion or fatigue, and water chemistry is carefully managed to prevent the formation of scale or sludge that could impair the heat exchange process.
Other components, such as pistons, cylinders, and valves, also undergo regular checks to ensure they are working properly. Pistons and cylinders are subject to wear over time due to the constant friction and pressure from the steam. To extend the life of these components, regular lubrication and careful inspection for signs of damage or misalignment are required. Valve seals are replaced as necessary to prevent leaks, and the valve gear system, which controls steam admission, may need periodic calibration to maintain precise timing and performance.
Turbine blades, in particular, require careful monitoring, as they are subjected to extreme heat and mechanical stress. Over time, turbines may experience thermal degradation, cracking, or erosion. To mitigate these issues, many modern steam turbines are equipped with sensors that monitor vibrations, temperature, and stress levels in real time. These sensors can detect potential problems early, allowing for preventive maintenance before any catastrophic failure occurs. Turbine blades may also need periodic polishing or resurfacing to maintain their aerodynamic properties and reduce friction.
The efficiency of steam engines is also affected by external factors, such as fuel quality or the thermal environment. In renewable or hybrid applications, where steam engines work alongside solar, geothermal, or biomass energy systems, there may be additional considerations regarding fuel consistency or the need to switch between different energy sources based on availability. In such cases, steam engines must be flexible enough to handle a variety of input conditions, and operators may adjust settings to optimize performance depending on the source of the heat.
As with any complex mechanical system, steam engines require routine monitoring of auxiliary systems, such as the condensate recovery system, pumps, and safety valves. A well-maintained condensate recovery system, for instance, ensures that water used in the steam cycle is recycled effectively, reducing the need for fresh water input and improving overall efficiency. Pumps, which maintain the flow of steam and water, need to be checked for wear and tear, and safety valves must be tested periodically to ensure they are functioning properly to release excess pressure if the system becomes dangerously pressurized.
The long-term performance of steam engines also depends on advances in materials and technology. New alloys and composites designed for high-temperature, high-pressure applications are continuously being developed, and these may be incorporated into older steam engines during maintenance or upgrade procedures. Materials such as ceramic coatings or nanostructured surfaces are increasingly being used to reduce friction, enhance durability, and improve thermal efficiency. These technological advances help steam engines maintain or even improve their performance over time, even as the operating conditions become more demanding.
Lastly, with the growing emphasis on sustainability and energy efficiency, steam engines are increasingly being integrated into broader energy management systems. In modern power plants or industrial operations, steam engines often operate in conjunction with other energy systems, such as combined heat and power (CHP) units, energy storage solutions, and renewable energy sources. The integration of steam engines with these systems requires advanced control strategies and software that can manage the interaction between multiple energy sources, ensuring that steam generation is optimized for the current energy demand while minimizing waste and emissions.
In summary, while the manufacturing process for steam engines is intricate and requires specialized knowledge and skill, the operation and maintenance phase is equally critical to ensure long-term efficiency and reliability. By combining rigorous maintenance schedules, real-time monitoring, technological advancements, and integration with renewable energy systems, modern steam engines can continue to be a valuable asset in a variety of industrial, commercial, and power generation applications, playing an essential role in today’s energy landscape.
Custom Steam Engine Solutions for Industry
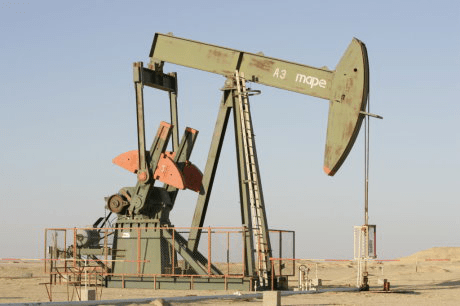
Custom steam engine solutions are increasingly being tailored to meet the specific needs of various industries, providing highly efficient and optimized systems that are suited for unique operational requirements. These solutions go beyond standard off-the-shelf steam engines, incorporating design modifications, specialized materials, and advanced technologies to meet the challenges and goals of particular industrial applications. Below are some examples of how custom steam engine solutions are being implemented across different sectors:
1. Industrial Power Generation
In large-scale industrial operations, custom steam engine solutions are used for power generation that complements the overall energy strategy. For industries like paper mills, chemical plants, or refineries, where substantial heat is produced during manufacturing processes, steam engines can be integrated into combined heat and power (CHP) systems. These systems not only generate electricity but also capture and reuse the thermal energy, significantly improving overall efficiency.
Custom steam engines in these environments are designed to handle specific heat loads, operate efficiently at varying pressure levels, and accommodate fluctuations in fuel availability. For instance, a custom steam turbine might be designed to operate in a wide range of thermal conditions, adapting to both conventional fossil fuels and renewable energy sources like biomass. Additionally, the turbines may be designed to handle corrosive or dirty feedwater, common in some industrial settings, ensuring the longevity and durability of components under harsh conditions.
2. Waste Heat Recovery
Many industries generate significant amounts of waste heat—from exhaust gases, industrial furnaces, or cooling processes—that can be harnessed for energy production. Custom steam engines are frequently used in waste heat recovery systems, where the engine is specifically designed to handle low-temperature or low-grade heat that would otherwise be wasted.
For example, industries like steel production, cement manufacturing, and glass making all produce vast quantities of waste heat during their processes. Custom steam turbines or organic Rankine cycle (ORC) systems are often engineered to extract energy from this waste heat, producing electricity or useful thermal energy. These engines are optimized to run efficiently at lower steam temperatures and pressures compared to traditional systems, allowing for energy recovery in conditions that would be inefficient for conventional steam engines. The flexibility in design helps to ensure that even small or intermittent sources of heat can be converted into useful power.
3. Renewable Energy Systems
As industries shift towards more sustainable energy solutions, custom steam engines are being integrated into renewable energy systems like geothermal, solar thermal, and biomass plants. In these applications, steam engines must be adapted to handle the variable nature of renewable energy sources.
For example, solar thermal plants utilize mirrors or lenses to focus sunlight and generate high temperatures, which can be used to produce steam. Custom steam engines are often designed with high-temperature materials and innovative cooling systems to ensure optimal performance, even under fluctuating solar conditions. In geothermal power plants, where steam or hot water is drawn from deep underground reservoirs, steam engines must be designed to handle not only the high pressure and temperature of the geothermal fluids but also the potential for minerals or gases in the steam that could cause corrosion or scaling. These custom solutions are tailored to meet specific geothermal resource characteristics, ensuring reliable operation and maximum efficiency.
4. District Heating Systems
Custom steam engine solutions are also valuable for district heating systems, where steam is used to provide heat to multiple buildings or facilities from a central plant. In this case, steam engines are designed to operate in large-scale, community-based energy systems that deliver heat to residential, commercial, and industrial customers. These engines are optimized for continuous operation, often with the ability to adjust heat and power output based on seasonal demand.
In some cases, steam engines are used to convert excess heat from industrial processes into steam for district heating, reducing the need for additional fuel consumption. Custom steam turbines or heat exchangers can be designed to efficiently manage varying temperatures and load demands, ensuring that customers receive reliable heating while minimizing energy waste.
5. Marine and Transportation Applications
Custom steam engine solutions have found niche applications in marine and transportation sectors, particularly in historical restoration projects or experimental designs. Steam engines can be integrated into ships, trains, and even electric vehicles that require a low-emission, high-torque power source.
In marine applications, for example, custom-designed steam turbines or engines can provide power to large vessels, where efficiency and durability are critical. These engines are tailored to work with specific fuel types such as biomass, biodiesel, or propane, making them a sustainable alternative for certain types of vessels. For train operators or operators of historic locomotives, custom steam engines can be modified to meet modern regulatory standards while maintaining the traditional steam power that offers high torque and low-speed operation.
6. Custom Solutions for Harsh Environments
Certain industries, such as mining, oil and gas, and marine operations, demand custom steam engines that can withstand harsh and extreme environments. Custom steam engines in these sectors are often designed with specialized materials that resist corrosion, abrasion, and extreme temperatures. In the oil and gas industry, for example, steam engines are frequently used in Enhanced Oil Recovery (EOR) methods, where steam is injected into reservoirs to reduce oil viscosity and improve extraction.
To withstand such conditions, custom steam turbines or reciprocating engines are engineered with heat-resistant alloys, enhanced lubrication systems, and protective coatings to prevent damage from corrosive materials such as hydrogen sulfide or saline water. These engines are also designed to operate reliably in remote or off-grid locations, with self-sufficient power and water treatment systems to handle the needs of the site.
7. Custom Steam Solutions for Small and Remote Applications
Custom steam engine solutions are also being employed in small-scale or off-grid applications, where a flexible, compact energy system is needed. For example, in rural or isolated communities where access to the electrical grid is limited, custom-designed steam engines can run on biomass, wood, or organic waste to provide both electricity and heat. These systems are optimized to operate with locally available fuels and can be scaled to meet the specific energy needs of the community.
Similarly, in the agricultural sector, steam engines are used in greenhouses, drying facilities, and irrigation systems, where they can provide both thermal energy for heating and power for mechanical operations like pumps or fans. Custom steam engines in these applications are designed for efficiency, easy operation, and low maintenance, providing farmers and rural communities with sustainable energy solutions.
8. Custom Solutions for Specialty Industries
In some niche applications, steam engines are customized to meet the needs of specialty industries that require unique energy solutions. Industries such as pharmaceutical production, food processing, and textile manufacturing often have specific temperature, humidity, and pressure requirements that can be met with custom steam engines. For instance, in pharmaceutical manufacturing, where precise temperature control is critical for sterilization processes, custom steam systems are designed to deliver exact steam conditions without overheating or underheating the materials being processed.
For textile mills, steam engines provide the power needed for various stages of production, including dyeing, finishing, and drying fabrics. In these settings, custom steam systems are engineered to accommodate the specific requirements of the production process, with a focus on energy efficiency and consistent steam delivery
In conclusion, custom steam engine solutions allow industries to take full advantage of the energy efficiency and operational flexibility of steam power while addressing the unique challenges they face. These tailored systems are designed to improve performance, reduce fuel consumption, and lower environmental impacts, while providing the reliability and durability needed for demanding industrial environments. Whether for power generation, waste heat recovery, renewable energy, or specialized applications, custom steam engines remain an essential tool for modern industrial solutions.
Custom steam engine solutions continue to evolve as industries demand higher efficiency, flexibility, and sustainability. These solutions allow companies to tailor their steam systems to meet very specific needs, optimizing their operations while reducing costs and environmental impact. One of the key advantages of custom solutions is the ability to design engines that can operate with a variety of fuels, including renewable and waste-based resources. This flexibility allows industries to lower their reliance on fossil fuels and reduce carbon emissions while still meeting their power and heating requirements.
In many applications, the adaptability of custom steam engines allows them to operate in conjunction with other energy systems, creating hybrid solutions that improve overall energy efficiency. For example, integrating steam engines with solar thermal or biomass systems can enable a facility to operate with a reduced carbon footprint while maintaining consistent power generation. These systems can be designed to switch between different heat sources or combine multiple sources of energy to ensure reliable operation, even in fluctuating conditions. This makes custom steam engine solutions not only more energy-efficient but also more resilient in the face of changing energy availability or environmental factors.
Moreover, the use of advanced materials and manufacturing techniques is helping to push the boundaries of what steam engines can do. With innovations in high-temperature alloys, coatings, and composites, steam engines can now handle more extreme conditions, extending their lifespan and improving their performance under high-pressure or high-temperature scenarios. These improvements also help reduce the need for frequent maintenance, which can lower operating costs and reduce downtime. Furthermore, custom steam engines are increasingly equipped with smart sensors and monitoring systems, allowing operators to track performance in real time. This enables predictive maintenance, where issues are identified and addressed before they lead to failure, further enhancing reliability and efficiency.
The integration of custom steam engine solutions into industrial processes also opens up new opportunities for energy recovery and waste heat utilization. Many industries generate large amounts of waste heat that can be captured and converted back into usable energy. Custom steam engines designed for waste heat recovery systems can extract energy from exhaust gases, cooling systems, or other sources of waste heat, improving overall plant efficiency. This is particularly useful in industries like steel production, cement manufacturing, or chemical processing, where high-temperature processes produce excess heat that would otherwise go to waste.
In addition to improving energy efficiency, custom steam engines can be optimized for specific operational characteristics, such as high torque at low speeds or the ability to start up quickly and ramp up to full power in a short amount of time. This is important for applications where load demands fluctuate rapidly or where power needs to be generated quickly in response to changes in production schedules or energy demand.
One of the key aspects of custom steam engine solutions is their ability to be highly tailored to the operational constraints and specific goals of a given industry. For example, some industries may require very precise steam quality and pressure for critical processes, while others might prioritize long-term operational stability under heavy load conditions. Custom steam engine designs can incorporate the necessary adjustments to meet these requirements, such as optimizing the steam cycle, enhancing heat transfer, and fine-tuning the control systems to manage pressure and temperature with greater precision.
As sustainability becomes an increasingly important focus across all sectors, custom steam engines are also being integrated into broader sustainability initiatives. In many cases, steam systems are designed to minimize energy consumption and maximize resource efficiency, often aligning with green building certifications or carbon-neutral goals. In the case of agricultural applications, custom steam systems can be designed to use organic waste materials or renewable resources like biomass, which further reduces environmental impacts. The ability to integrate these systems with energy storage solutions or power management systems can help industries ensure that they can meet both their operational and environmental goals.
Custom steam engine solutions are also playing a role in sectors where reliable backup power is critical. In facilities like hospitals, data centers, or emergency response centers, where power failure is not an option, steam engines can serve as reliable backup power sources. These engines can be designed to quickly respond to power outages, providing uninterrupted energy to critical systems until the main grid is restored. In some cases, these backup steam engines may be designed to operate in tandem with other backup systems, such as diesel generators or battery storage, to ensure seamless and reliable power delivery during outages.
Finally, as industries around the world continue to modernize and adopt new technologies, custom steam engine solutions will become increasingly sophisticated. With the advancement of artificial intelligence (AI), machine learning, and predictive analytics, steam engines will be able to self-optimize based on real-time operational data, improving performance over time without the need for manual adjustments. These developments will further enhance the versatility of steam engines, enabling them to integrate into smart grid systems, contribute to decentralized energy solutions, and support the transition toward more sustainable industrial practices.
In conclusion, custom steam engine solutions are helping industries to meet their unique energy and operational needs in increasingly efficient, sustainable, and cost-effective ways. By tailoring these systems to specific applications, industries can optimize their energy use, improve performance, and reduce environmental impact, all while ensuring reliability and operational efficiency. With continued advances in materials, technology, and design, the future of custom steam engine solutions is bright, offering even more opportunities for industries to embrace cleaner, more efficient, and more adaptable energy solutions.
As industries continue to seek more sustainable and cost-effective energy solutions, custom steam engine technologies are poised to evolve further, integrating even more advanced systems and features that can address the challenges of tomorrow’s industrial landscape. Several trends and innovations are expected to shape the future of custom steam engines and their applications.
One key area of growth is the integration of digital technologies into steam engine systems. The use of Internet of Things (IoT) devices, smart sensors, and real-time data analytics is allowing operators to closely monitor and optimize the performance of steam engines. By collecting data from multiple points in the system—such as pressure, temperature, steam flow, and component wear—these systems can continuously adjust to improve performance and efficiency. Additionally, advanced machine learning algorithms can analyze the vast amounts of data generated by these sensors to predict potential failures or performance declines before they occur, allowing for predictive maintenance. This approach reduces downtime, extends the lifespan of components, and minimizes the cost of repairs by addressing issues before they become major problems.
Another exciting development is the hybridization of steam engine systems with renewable and low-carbon technologies. Steam engines have traditionally relied on fossil fuels, but increasingly, they are being adapted to work in conjunction with solar thermal, geothermal, biomass, and other renewable energy sources. In these systems, steam engines can play a complementary role, either by providing backup power when renewable sources are unavailable or by converting waste heat from renewable processes into usable steam. This synergy not only maximizes the efficiency of renewable systems but also reduces reliance on non-renewable energy sources, helping industries meet ambitious carbon reduction goals.
The demand for decentralized and off-grid power generation is also driving the development of custom steam engine solutions. Remote or rural areas, where access to centralized electrical grids is limited or non-existent, are increasingly turning to independent energy systems. Custom steam engines are ideal for these settings, especially when paired with biomass, wood, or other locally available fuels. These systems can be designed to operate autonomously, generating electricity and heat for local communities, reducing energy costs, and providing a reliable power source even in regions with poor grid infrastructure. This trend is not limited to developing regions but is also gaining traction in industrial facilities or operations located in remote or harsh environments, such as oil rigs, mining operations, or military bases, where reliable power is critical.
Modular steam systems are another innovative development in custom steam engine solutions. These systems are designed with scalability and flexibility in mind, allowing companies to start with a smaller, more cost-effective solution and scale up as their energy needs grow. In these modular setups, multiple smaller steam engines can be linked together to create a larger power generation system, enabling better load management and improved efficiency across the facility. Modular systems also allow for easy maintenance and replacement of individual components without disrupting overall operations, which is particularly useful in industrial facilities where downtime can be costly.
As energy storage technologies continue to advance, custom steam engines are being integrated into hybrid systems that incorporate both battery storage and steam generation. In these configurations, steam engines can provide base-load power while batteries handle peak demand, ensuring that energy is consistently available even when renewable sources like solar or wind are intermittent. The combination of steam and battery storage not only enhances the overall efficiency of the system but also supports the grid by stabilizing fluctuations in energy supply and demand.
The growing emphasis on circular economy principles is also influencing the design of custom steam engines. Industries are increasingly focusing on resource efficiency and minimizing waste. Steam engines that can run on waste biomass or organic waste materials, for instance, contribute to a circular economy by converting discarded materials into valuable energy. Additionally, advancements in waste-to-energy technologies enable steam engines to recover energy from various waste products, such as municipal solid waste or industrial by-products. By incorporating waste streams into energy production, industries can minimize landfill waste while generating clean, renewable energy.
Another aspect of future custom steam engine design is thermal energy storage. This technology allows excess heat generated during off-peak hours to be stored and used when demand is high. Steam engines can be integrated with thermal energy storage systems to improve overall energy efficiency and balance supply and demand. This approach is particularly beneficial in industries that require high amounts of heat for processes, such as in the food processing, chemical, or textile industries. By capturing and storing thermal energy, these industries can avoid spikes in energy costs and reduce their environmental impact by using renewable heat sources when possible.
Environmental sustainability will continue to be a key driving factor for custom steam engine design. As governments and industries worldwide push for cleaner energy solutions, steam engines are being designed with advanced emissions control technologies to reduce pollutants such as nitrous oxides (NOx), sulfur oxides (SOx), and carbon dioxide (CO2). New materials, improved combustion techniques, and better integration with emission control systems allow for cleaner and more efficient operation. Additionally, the development of steam engines capable of using biogas or syngas—a gas derived from renewable sources such as organic waste—further reduces the carbon footprint of steam-based power generation.
As the cost of renewable energy technologies continues to decline and their efficiency improves, the adoption of custom steam engine solutions that rely on these technologies will expand. Custom systems that integrate solar concentrators, geothermal loops, and biomass conversion are expected to become more prevalent, providing industries with versatile, low-emission alternatives to traditional energy systems. These systems, which can include both thermal and electrical output, provide a continuous, renewable energy source while reducing reliance on fossil fuels and minimizing greenhouse gas emissions.
Finally, customization itself will continue to be an area of growth, with manufacturers offering highly tailored solutions to meet the unique needs of industries, from power generation to specialized manufacturing processes. Steam engines will become more efficient in terms of fuel consumption, heat recovery, and power output, while the integration of digital and smart technologies will allow for greater adaptability in real-time energy management. As industries demand more specialized applications—whether it’s for backup power, waste heat recovery, or specialized process requirements—custom steam engines will evolve to meet these demands, offering bespoke solutions that push the boundaries of performance, energy efficiency, and sustainability.
In conclusion, the future of custom steam engine solutions lies in the ability to adapt to emerging technologies, evolving energy demands, and the growing push for environmental sustainability. These engines will continue to serve as a cornerstone for industries seeking reliable, efficient, and clean energy sources. By leveraging innovations in digital technology, renewable energy, materials science, and energy storage, custom steam engines will not only remain relevant in the modern energy landscape but will also become integral to the transition toward cleaner, more sustainable industrial operations worldwide.
Types of Steam Engines: A Comprehensive Guide
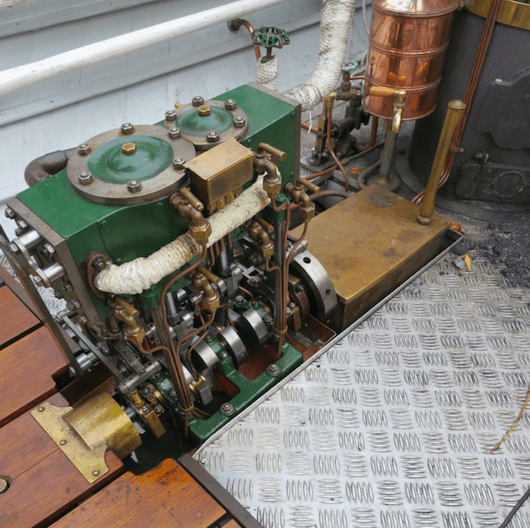
Steam engines, though rooted in the past, continue to be pivotal in various modern applications, and understanding the different types can help identify which is best suited for specific uses. These engines have evolved over time, incorporating new materials, technologies, and refinements. Below is a comprehensive guide to the various types of steam engines, highlighting their unique features and applications.
1. Reciprocating Steam Engines
Reciprocating steam engines are the most traditional type, where steam pressure is used to push a piston back and forth inside a cylinder. These engines convert the thermal energy of steam into mechanical work through linear motion, which is then converted to rotary motion using a crankshaft.
- Applications: Historically used in locomotives, steamships, and factory machinery. They remain in use in certain small-scale applications, such as in old-fashioned pumps, agricultural engines, and educational demonstrations.
- Advantages: Simple design, reliable, and easy to maintain.
- Disadvantages: Less efficient at high speeds, larger and heavier compared to other types.
2. Steam Turbines
Steam turbines are a more modern and efficient evolution of the steam engine. In this design, steam is directed at blades attached to a shaft, causing the shaft to spin. The spinning shaft is then used to generate mechanical energy.
- Applications: Predominantly used in power plants for electricity generation, marine propulsion (such as in large ships and naval vessels), and in some industrial applications like petrochemical processing.
- Advantages: Higher efficiency than reciprocating engines, especially at larger scales. Can generate large amounts of power with relatively compact designs.
- Disadvantages: Expensive to build and maintain, requires precise engineering.
3. Compound Steam Engines
Compound steam engines are a type of reciprocating engine that uses multiple cylinders to maximize efficiency. In these engines, steam is expanded in stages through multiple cylinders of varying sizes. The steam first passes through a high-pressure cylinder, then moves to progressively larger cylinders at lower pressures, extracting more energy at each stage.
- Applications: Often found in marine vessels, large industrial machinery, and early power plants.
- Advantages: More efficient than simple steam engines due to the multi-stage expansion of steam.
- Disadvantages: More complex design, requiring precise synchronization between cylinders.
4. Double-Acting Steam Engines
In a double-acting steam engine, steam is applied to both sides of the piston, allowing for a more continuous and efficient motion compared to a single-acting engine, which only uses steam on one side of the piston. This type of engine can provide power during both the forward and backward strokes of the piston.
- Applications: Used in various industries for pumping, lifting, and other heavy-duty tasks.
- Advantages: Greater power output for the same size, more continuous operation.
- Disadvantages: Slightly more complex due to the need for two valve systems.
5. Non-Condensing Steam Engines
Non-condensing steam engines, also known as atmospheric engines, exhaust steam into the atmosphere rather than condensing it into water. These engines operate at lower pressures and are less efficient than their condensing counterparts but are simpler in design and cheaper to construct.
- Applications: Early industrial applications, including pumping stations and in some vintage steam locomotives.
- Advantages: Simple design and lower maintenance costs.
- Disadvantages: Lower efficiency, greater fuel consumption.
6. Condensing Steam Engines
Condensing steam engines work by cooling the exhaust steam to create a vacuum or lower pressure, which allows the engine to extract more energy from the steam, increasing overall efficiency. This type of engine typically uses a separate condenser to cool the steam back into water, which can then be reused in the boiler.
- Applications: Commonly used in power plants, especially for electricity generation, as well as in large-scale industrial applications.
- Advantages: Higher efficiency, reduced fuel consumption, and lower operating costs.
- Disadvantages: Requires more complex equipment and maintenance, including the condenser.
7. Flash Steam Engines
Flash steam engines are a type of steam engine that uses flash steam, which is created by rapidly releasing steam from pressurized water. The water quickly vaporizes into steam when the pressure is released, and this steam can then be used to drive an engine. Flash steam engines are typically used in applications where a heat source is already available, such as in geothermal or biomass energy systems.
- Applications: Used in geothermal power plants, and also in some waste heat recovery systems.
- Advantages: Can efficiently use lower-grade heat sources, relatively compact design.
- Disadvantages: Limited to certain applications, especially where flash steam can be efficiently generated.
8. Locomotive Steam Engines
Locomotive steam engines are a type of reciprocating engine specifically designed for use in steam trains. These engines use a series of pistons and a driving wheel system to propel the train forward. A coal-fired boiler generates steam, which is then used to drive the pistons, translating into mechanical energy.
- Applications: Historical rail transportation, and still used in some tourist or heritage railways.
- Advantages: High torque at low speeds, ideal for pulling heavy loads over long distances.
- Disadvantages: Inefficient by modern standards, large fuel consumption, and high maintenance.
9. Steam-Driven Pumps
These are specialized steam engines designed specifically for pumping water or other liquids. They can be either reciprocating or rotary types, but the key feature is that they use steam power to move fluids.
- Applications: Often used in mining, irrigation, waterworks, and sewage treatment plants.
- Advantages: Can operate independently of electricity, suitable for remote areas.
- Disadvantages: Typically lower efficiency compared to modern electric pumps.
10. Rotary Steam Engines
Rotary steam engines use steam pressure to turn a rotor instead of a piston. These engines operate on a principle that differs from the traditional reciprocating engine, utilizing rotary motion to drive mechanical processes.
- Applications: Less common, but can be found in specialized industrial applications, such as in compressors, pumps, and small power plants.
- Advantages: Continuous, smooth operation, compact design.
- Disadvantages: Less efficient than other types at large scales, can be difficult to scale up.
11. Stirling Engines
Though not technically a steam engine in the traditional sense, Stirling engines work by expanding and contracting gas using heat. They are an example of external combustion engines that use heat to drive mechanical work. While they can run on steam, they are more commonly powered by air, biomass, or other heat sources.
- Applications: Used in small-scale, solar thermal power generation and experimental power applications.
- Advantages: High efficiency at small scales, environmentally friendly, and relatively low emissions.
- Disadvantages: Limited to smaller power applications, slower response times.
12. Rocket Engines (Steam Rockets)
Though largely obsolete and specialized, steam rocket engines were once used in rocketry experiments. They work by producing high-pressure steam, which is expelled through a nozzle to create thrust.
- Applications: Used historically for experimental propulsion, now of interest in niche aerospace applications.
- Advantages: Simple design, potential for high thrust at low altitudes.
- Disadvantages: Inefficient, impractical for modern space exploration.
Conclusion
Steam engines, despite the rise of more efficient and modern energy systems, continue to hold importance in niche applications, where they provide advantages in energy efficiency, fuel flexibility, and power generation. The type of steam engine used in any given application depends on several factors, including efficiency, operational requirements, space, and fuel availability. Whether for industrial power generation, transportation, or waste heat recovery, each type offers unique benefits tailored to specific needs. As industries continue to explore more sustainable energy solutions, some of these steam engine technologies may see a resurgence, particularly in renewable energy contexts, where steam cycles are combined with clean sources like geothermal or biomass.
As steam engine technology continues to evolve, more specialized and innovative applications are emerging. One of the key drivers for the continued development of steam engines is the push for cleaner and more sustainable energy sources. With the growing demand for renewable energy, steam engines are finding new life in geothermal and biomass power plants, where they can efficiently convert heat into mechanical energy, contributing to cleaner electricity generation. In geothermal power plants, steam extracted from the Earth’s natural heat sources is used to power steam turbines, driving electrical generators while minimizing environmental impact. Similarly, biomass plants use organic waste materials to generate steam, further reducing reliance on fossil fuels.
Another area where steam engines are making a comeback is in small-scale, off-grid power generation. As energy security becomes a priority in remote or underserved areas, steam engines offer a reliable, low-tech solution for communities that may not have access to electricity through conventional means. In these settings, flash steam engines, which can harness heat from locally available resources like wood or waste biomass, are particularly effective for providing energy in off-grid applications. These systems can operate independently, producing both heat and electricity, and they can often be scaled according to the specific needs of the community or facility.
The integration of combined heat and power (CHP) systems also presents new opportunities for steam engine technology. In a CHP system, steam engines or turbines generate both electricity and useful thermal energy, such as hot water or steam for industrial processes, space heating, or district heating networks. These systems are especially popular in industrial applications where there is a consistent demand for both heat and electricity, and they help maximize the overall efficiency of energy use. By capturing waste heat and using it in other processes, these systems can achieve efficiencies of 70% to 80%, significantly reducing fuel consumption and emissions.
With advances in digital control systems and sensor technologies, steam engines are also becoming smarter and more efficient. Modern steam engines can be equipped with sophisticated monitoring systems that track performance in real time, allowing operators to adjust settings for optimal efficiency. For instance, steam pressure, temperature, and flow can be continuously monitored, and adjustments can be made automatically to ensure the engine operates within ideal parameters. This allows for improved fuel efficiency, reduced emissions, and increased system reliability. Predictive maintenance technologies, powered by artificial intelligence and machine learning, further enhance the lifespan and reliability of steam engines by predicting when components need maintenance or replacement, reducing unplanned downtime and repair costs.
Another exciting development is the potential for integrating steam engines with solar power. In solar thermal power plants, mirrors or lenses focus sunlight onto a fluid (typically oil or water), which is then used to produce steam. This steam can then power turbines or engines, providing renewable electricity. Hybrid systems that combine solar thermal energy with steam engines offer an efficient way to store and utilize solar energy even when the sun isn’t shining, as the stored heat can be used to generate steam when needed, providing a continuous and reliable energy source.
While many of the traditional uses of steam engines, such as in steamships and trains, have been largely replaced by more efficient technologies, there is still a niche market for these applications, particularly in heritage railways and historical attractions, where steam engines offer a glimpse into the past. In these settings, they not only serve as a source of education and entertainment but also continue to capture the imagination of those fascinated by the history of industrialization.
At the same time, new types of steam engines are emerging, incorporating innovations such as microturbines or miniaturized systems that can be used for personal power generation or in remote monitoring applications. These smaller, more portable steam engines may use a variety of heat sources, including waste heat, and are ideal for applications where space is limited, and conventional power sources are unavailable or impractical.
The future of steam engine technology also points toward increasing integration with digital infrastructure. As industries move towards more automated and connected operations, steam engines are becoming part of larger smart grids and integrated energy systems. These systems can automatically adjust energy generation based on demand, enabling better overall management of power generation and consumption. The integration of steam engines into such smart networks allows for better synchronization between power sources, reducing waste and ensuring energy is used efficiently across multiple applications.
Ultimately, the ongoing advancements in steam engine technology promise to keep them relevant in the coming decades. While the core principles of steam engines remain grounded in centuries-old technology, innovations in materials, digital controls, renewable energy integration, and energy efficiency are expanding their applications. Whether for industrial processes, renewable energy systems, or small-scale off-grid power generation, the continued evolution of steam engine technology represents an exciting opportunity to improve sustainability, efficiency, and energy security across various sectors. As industries seek to reduce their environmental footprints and embrace more sustainable energy solutions, steam engines will remain a key player in the broader energy landscape, demonstrating their adaptability in the modern world.
As the world continues to focus on sustainability and energy efficiency, steam engine technology is adapting to meet new demands. One area where this adaptation is particularly evident is in the use of waste heat recovery systems. Industrial processes often generate significant amounts of heat that are released into the environment as waste. Steam engines, when integrated with waste heat recovery systems, can harness this waste heat to generate power, thereby improving the overall efficiency of industrial operations. These systems are especially useful in industries such as cement production, steel manufacturing, and chemical processing, where large amounts of heat are generated during production processes. By capturing and converting waste heat into useful energy, steam engines can reduce overall energy consumption and lower operating costs while minimizing the environmental impact of these industries.
Another exciting area of development is the use of advanced materials in the construction of steam engines. Over the years, improvements in material science have led to the development of stronger, more heat-resistant materials that can withstand higher pressures and temperatures. These advancements have allowed for more efficient steam engines, capable of operating at higher efficiencies and longer lifespans. Alloys such as stainless steel and titanium are increasingly being used to manufacture critical components of steam engines, such as boiler tubes, turbine blades, and heat exchangers. These materials offer improved durability and resistance to corrosion, which is particularly important in systems that use water or steam at high temperatures and pressures.
In addition to advancements in materials, additive manufacturing (3D printing) is starting to play a role in steam engine design and production. This technology enables the creation of highly customized and complex parts that are difficult or impossible to manufacture using traditional methods. Components such as turbine blades, pump impellers, and heat exchangers can now be designed with more intricate geometries that improve their performance and efficiency. 3D printing also allows for rapid prototyping, reducing the time it takes to test and iterate on new designs, which accelerates the innovation process and leads to more optimized steam engine solutions.
Hybrid steam engines, which combine the benefits of traditional steam power with other energy sources, are also gaining traction. For example, solar-assisted steam engines utilize both solar energy and steam generation to enhance overall system efficiency. In these systems, solar energy is used to preheat water or generate steam directly, reducing the amount of fuel needed to operate the steam engine. Such hybrid systems are particularly valuable in regions with high levels of sunlight, allowing industries to reduce their reliance on fossil fuels and lower greenhouse gas emissions. Similarly, biofuels are being used in steam engines to reduce the carbon footprint of power generation, particularly in agricultural or forestry applications where biomass is abundant and readily available.
The development of micro and pico steam engines is also opening up new possibilities for decentralized power generation. These small-scale steam engines can generate electricity in the range of a few kilowatts to several hundred watts, making them ideal for off-grid applications or for powering small machines and devices. Micro steam turbines are increasingly being used in remote research stations, isolated communities, and small-scale manufacturing operations, where access to centralized electricity is limited or nonexistent. These micro systems are highly adaptable and can be fueled by a wide range of sources, including wood, waste heat, and biomass, providing a reliable energy source in off-grid and rural areas.
The future of steam engine technology is also intertwined with the growing trend of decarbonization in industrial sectors. As part of the global push to achieve net-zero emissions, many industries are looking for ways to reduce their carbon footprint and transition to cleaner energy sources. Steam engines, especially when paired with carbon capture and storage (CCS) technologies, are seen as a viable solution to reduce emissions from power plants and heavy industries. In these systems, CO2 emissions from the combustion of fuels are captured before they are released into the atmosphere and stored underground or used in other industrial processes. By integrating steam engines with CCS technologies, industries can continue to utilize steam power while mitigating the environmental impact of their operations.
Another area of development is in the thermal energy storage (TES) field. By using steam engines in combination with TES systems, industries can store excess heat produced during off-peak hours and use it when demand is higher, thus balancing energy supply and demand more effectively. In these systems, heat is stored in materials such as molten salts or phase change materials, which can later be converted back into steam to power engines. This kind of system is particularly useful in solar thermal plants or concentrated solar power (CSP) plants, where heat is stored during the day and used to generate steam when the sun is not shining. This not only improves the efficiency of these plants but also helps stabilize the energy grid by providing a consistent power source, even during periods of intermittent solar generation.
The integration of steam engines with smart grid systems is another promising development. Smart grids are designed to optimize the distribution and use of electricity by allowing real-time monitoring, communication, and control of energy flows. By incorporating steam engines into these networks, they can be dynamically controlled to meet varying energy demands. For example, during peak demand periods, steam engines could be used to provide additional power to the grid, while during off-peak times, they could be ramped down or shut off. This flexibility enables better overall grid management and helps reduce energy waste.
Finally, hydrogen is emerging as a promising fuel source for steam engines, particularly in industries looking to reduce their dependence on fossil fuels. Hydrogen-powered steam engines work similarly to traditional steam engines, except that the hydrogen fuel is used to produce the steam. When burned, hydrogen produces only water vapor as a by-product, making it a clean and environmentally friendly alternative to coal, natural gas, or other fossil fuels. Hydrogen can be produced through electrolysis (splitting water into hydrogen and oxygen using electricity) using renewable energy sources, creating a truly green energy cycle. As hydrogen infrastructure continues to grow, steam engines fueled by hydrogen may play a significant role in industrial decarbonization efforts.
In conclusion, steam engine technology is far from obsolete. In fact, it is undergoing a transformation, driven by advancements in materials, renewable energy integration, and digitalization. From off-grid power generation to carbon capture and thermal energy storage, steam engines are finding new life in modern applications. As industries seek more efficient, sustainable, and adaptable energy solutions, steam engines will continue to be a cornerstone of energy production, particularly in niche applications and as part of hybrid systems. The combination of traditional steam engine principles with cutting-edge technologies will ensure that steam remains an important part of the energy landscape for years to come.
Steam Engine Parts and Components
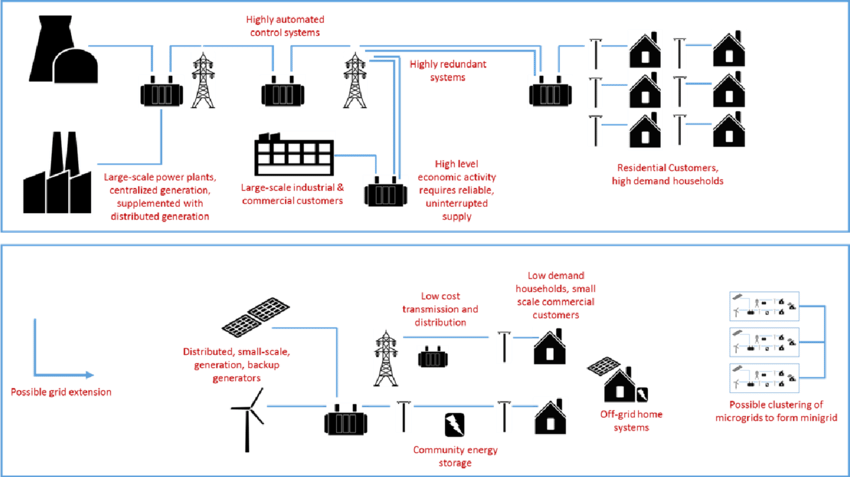
A steam engine consists of several critical parts and components, each of which plays a specific role in ensuring the engine functions properly. Understanding the components of a steam engine is crucial for both its design and operation. Here’s an overview of the key parts and their functions:
1. Boiler
The boiler is the component responsible for generating steam. It heats water to produce steam using heat from burning fuel such as coal, wood, oil, or gas. The boiler typically consists of a large, cylindrical chamber that houses pipes through which the fuel is burned. The heat generated from the combustion process is transferred to the water surrounding these pipes, converting it into steam.
- Function: Generates high-pressure steam for driving the engine’s mechanical components.
- Components: Firebox (where fuel burns), combustion chamber, and heat exchange surfaces (like tubes or coils).
2. Cylinder
The cylinder is where steam is introduced to create mechanical motion. The steam enters the cylinder, causing a piston to move. The cylinder is typically made of strong metal to withstand the pressure of steam. In some designs, multiple cylinders are used for efficiency (as in compound engines).
- Function: The cylinder houses the piston and acts as the chamber in which steam’s thermal energy is converted into mechanical work.
- Components: Cylinder casing, piston, piston rings, and valves for steam admission and exhaust.
3. Piston
The piston is a cylindrical piece that fits tightly inside the cylinder and moves back and forth (or reciprocates) when steam is applied. As steam enters the cylinder, it pushes the piston in one direction, and as the exhaust steam is vented, the piston returns in the opposite direction. This reciprocating motion is the foundation of how steam engines generate power.
- Function: Converts the thermal energy of steam into mechanical motion.
- Components: Piston rod, piston rings (to maintain a tight seal), and the piston head.
4. Crankshaft
The crankshaft converts the linear motion of the piston into rotational motion. As the piston moves back and forth, the crankshaft turns, providing the rotational force necessary to drive mechanical devices, such as wheels, gears, or turbines.
- Function: Converts the piston’s linear motion into rotary motion.
- Components: Crank, crankpins, and flywheel (in some designs).
5. Flywheel
The flywheel is a rotating mass attached to the crankshaft. Its purpose is to smooth out the fluctuations in speed caused by the reciprocating motion of the piston. The flywheel stores kinetic energy and helps maintain a consistent rotational speed.
- Function: Reduces speed variations and provides smooth, consistent power output.
- Components: Flywheel (a large, heavy disk or wheel).
6. Valves
Valves control the flow of steam into and out of the cylinder. In a basic steam engine, there are typically two valves: one to admit steam to the cylinder and one to exhaust steam after it has performed its work. In more complex designs, additional valves may be used to control the timing and distribution of steam.
- Function: Controls the timing and direction of steam flow into and out of the cylinder.
- Components: Steam admission valve, exhaust valve, valve gear mechanism (for controlling valve timing), and sometimes eccentric cams.
7. Valve Gear
The valve gear system is responsible for controlling the movement of the valves. It ensures that steam is admitted into the cylinder at the right time and that exhaust steam is released at the right time. The timing of valve operation is critical to the efficiency and performance of the steam engine.
- Function: Coordinates the timing of the valves’ movement to regulate the flow of steam.
- Components: Eccentric wheels, cams, and linkages.
8. Condenser
The condenser is used in condensing steam engines to cool exhaust steam and convert it back into water. This process creates a vacuum in the cylinder, allowing the engine to extract more energy from the steam, improving efficiency. The condenser is typically located outside the engine and consists of a series of tubes where steam is cooled by cold water.
- Function: Converts exhaust steam back into water and creates a vacuum to improve engine efficiency.
- Components: Condenser chamber, cooling tubes, and water circulation system.
9. Governor
The governor is a device used to regulate the engine’s speed. It adjusts the flow of steam into the engine based on the load or demand, ensuring that the engine operates at a steady speed. This is especially important in applications like power generation, where a consistent speed is necessary to synchronize with the electrical grid.
- Function: Regulates the engine’s speed to maintain a consistent output.
- Components: Centrifugal weights, throttle valve, and linkage.
10. Connecting Rod
The connecting rod is the link between the piston and the crankshaft. It transfers the linear motion of the piston to the crankshaft, converting the reciprocating motion into rotary motion.
- Function: Transmits the piston’s motion to the crankshaft.
- Components: Connecting rod, pin, and bearings.
11. Safety Valve
The safety valve is a crucial safety component designed to prevent the boiler from exploding due to excessive pressure. If the pressure in the boiler exceeds a preset limit, the safety valve opens to release steam and reduce pressure, ensuring the system remains within safe operational limits.
- Function: Prevents the boiler from exceeding its pressure limit and potentially causing a dangerous explosion.
- Components: Spring-loaded valve, pressure setting mechanism.
12. Ash Pan
In engines that burn solid fuel (such as coal), the ash pan collects the ashes and waste products that result from combustion. It is typically located beneath the firebox and needs to be emptied regularly to ensure efficient burning and safe operation.
- Function: Collects ash and other waste by-products from combustion.
- Components: Pan, ash door, and removal mechanism.
13. Water Pump
The water pump is used to supply water to the boiler to replace the water lost during steam production. In many steam engines, especially in locomotives, the water pump is powered by the steam engine itself.
- Function: Supplies water to the boiler to ensure a constant steam supply.
- Components: Pump body, piston, and valve system.
14. Smoke Stack (Chimney)
The smoke stack, or chimney, directs exhaust gases and smoke away from the engine or boiler. It is typically tall to ensure proper ventilation and to prevent the buildup of smoke in enclosed spaces.
- Function: Expels combustion gases and smoke safely from the engine.
- Components: Stack, flue, and damper.
15. Exhaust Pipe
The exhaust pipe carries the steam from the cylinder after it has done its work, directing it to the condenser or out into the atmosphere in non-condensing engines. In locomotives, the exhaust is often funneled into the smoke stack to create a visible plume.
- Function: Directs exhaust steam from the cylinder to the condenser or outside.
- Components: Pipe, joints, and valves.
16. Lubrication System
The lubrication system is essential to reduce friction between the moving parts of the steam engine, especially the piston, connecting rod, and crankshaft. Proper lubrication helps to reduce wear and tear, improve efficiency, and extend the life of the engine.
- Function: Reduces friction and wear between engine components.
- Components: Oil reservoir, oil pumps, and delivery lines.
Conclusion
Each part and component of a steam engine plays a crucial role in converting heat energy into mechanical work. From the boiler that generates steam to the valves that regulate its flow, each element is intricately designed to maximize efficiency and performance. Whether it’s a small-scale engine used for educational purposes or a large industrial turbine, understanding the parts and components of a steam engine is key to both its operation and maintenance.
In addition to the primary components of a steam engine, several supporting elements contribute to its overall performance and efficiency. The steam chest, for instance, is located above the cylinder and connects the boiler to the steam valve. It holds steam before it enters the cylinder and helps regulate steam distribution. The steam dome in the boiler, located at the top, collects and separates the steam from the water, ensuring only dry steam enters the engine.
The pressure gauge is an important instrument that provides real-time monitoring of the steam pressure within the boiler. This ensures that the system operates within safe pressure limits and allows operators to make adjustments as needed. Coupled with the thermometer, which measures the temperature of the steam, these gauges offer critical feedback for maintaining optimal engine performance.
Another essential part is the fuel supply system, which manages the introduction of fuel into the boiler. In older steam engines, especially in locomotives and ships, this was typically a manual process, with firemen stoking the furnace to maintain the proper fuel levels. Modern steam engines, however, may have automatic fuel feed mechanisms that ensure consistent and efficient combustion. These systems often include a fuel hopper or a fuel feed mechanism designed to optimize combustion and reduce the need for frequent refueling.
In some designs, a superheater is used to further enhance the efficiency of the steam engine. The superheater is a set of pipes or coils through which steam passes after leaving the boiler but before entering the cylinder. The superheater increases the steam’s temperature, making it more efficient at doing mechanical work by raising the energy content of the steam. This process helps improve engine efficiency by reducing the amount of fuel needed to generate the same amount of power.
The steam distribution system—comprising pipes, joints, and valves—ensures that steam flows properly to the cylinder at the correct pressure and temperature. The piping system also allows the exhaust steam to be directed out of the cylinder to either a condenser or into the atmosphere. Modern steam engines may use insulated pipes to minimize heat loss and maintain steam temperature as it travels from the boiler to the engine.
As steam engine technology has evolved, so has the importance of maintenance systems designed to keep these engines running smoothly. Regular maintenance is essential to ensure the longevity of the engine, prevent failures, and optimize performance. In industrial or large-scale applications, automated diagnostic systems are often integrated into the steam engine to monitor performance, detect potential issues, and alert operators about the need for repairs or adjustments.
In more modern setups, especially in power generation or district heating applications, heat exchangers are commonly used to transfer excess heat to other parts of the system, improving overall energy efficiency. These devices allow heat to be transferred from hot steam to water or other fluids, which can then be used in additional processes, such as heating or power generation. By recovering heat, steam engines can achieve higher efficiencies, especially in industrial or multi-use settings.
Steam engines, though relatively simple in concept, consist of a diverse array of components that must work in unison. The continued evolution of materials, automation, and technology has allowed for significant improvements in steam engine design, making them more efficient, reliable, and adaptable. While traditional steam engines may no longer dominate large-scale applications like they once did, their principles are still widely applied in various industries and remain relevant today due to their versatility and reliability. From heritage railways to modern power generation, these engines continue to prove that even centuries-old technology can evolve and thrive in new contexts.
The adaptability and continued relevance of steam engine technology can be attributed to ongoing innovations in efficiency, integration with modern systems, and diverse applications across different industries. In recent years, advancements in control systems have enhanced steam engine operation, allowing for more precise regulation of parameters such as pressure, temperature, and steam flow. These systems help optimize the performance of steam engines, making them more suitable for modern applications in energy generation, transportation, and manufacturing.
Automation in steam engine systems has become increasingly important, especially in large-scale industrial settings. Modern steam engines are often integrated with programmable logic controllers (PLCs) and distributed control systems (DCS), which automate many of the processes that were once managed manually. These automated systems can control the fuel feed, regulate steam pressure, and monitor various performance parameters. This has not only improved the efficiency of steam engines but also made their operation safer and more consistent, reducing the likelihood of human error and minimizing the need for manual interventions.
In industrial power generation, steam engines are commonly paired with other technologies to create combined heat and power (CHP) systems, which maximize the use of energy. In CHP systems, the waste heat from the steam engine’s exhaust is used to heat water or generate additional electricity, improving the overall energy efficiency of the system. These hybrid systems are particularly effective in industries like chemical processing, paper manufacturing, and food processing, where both power and heat are required for continuous operations. By utilizing both electricity and thermal energy, CHP systems help reduce operational costs and lower carbon emissions, making them an environmentally friendly choice.
Geothermal power plants also benefit from steam engine technology, as they use the natural heat from the earth’s interior to generate steam. This steam is then used to drive turbines that generate electricity, often in combination with steam engines. Geothermal energy is considered a sustainable and renewable energy source, and steam engines play a key role in converting the thermal energy from the earth into usable power. As the demand for clean energy solutions grows, the role of steam engines in harnessing geothermal resources is expected to increase.
Another innovative application is the use of supercritical steam engines in power plants. Supercritical steam is steam that has been heated beyond its critical point, meaning it is no longer a distinct liquid or gas. This superheated steam can be used to drive turbines at higher efficiency levels than traditional steam engines, as it operates at higher pressures and temperatures. Supercritical steam cycles are used in many modern coal, natural gas, and nuclear power plants, where they significantly improve thermal efficiency and reduce fuel consumption.
In the transportation sector, steam engines, though no longer in widespread use, are still celebrated for their historical significance and continue to inspire modern engineering. Heritage railways and steam-powered boats continue to operate in various parts of the world, offering a nostalgic connection to the past while demonstrating the enduring legacy of steam power. These engines, though not typically used for mainstream transportation today, serve as working museums, offering a glimpse into how industrial revolutions were powered and how steam engines shaped the development of modern infrastructure.
The military sector also benefited from steam technology in the past, and today, many of the principles that originated with steam engines have influenced the design of modern turbines and engines. For example, steam turbines are widely used in naval ships for propulsion, where they provide a reliable and efficient means of generating power. These engines, though often much more advanced than traditional steam engines, retain the same basic principles of steam generation and mechanical conversion of energy.
Beyond industrial and historical applications, steam engine principles are also being adapted for small-scale renewable energy projects. For instance, small steam turbines and engines are being used in rural or remote communities as part of off-grid power generation. These small systems can be powered by biomass, solar thermal energy, or waste heat, providing a flexible and renewable power source for isolated regions. This kind of distributed energy generation is particularly important in developing regions or in areas where connecting to the electrical grid is not feasible.
Looking to the future, steam engines are likely to remain a cornerstone of energy systems that emphasize sustainability and efficiency. Research is ongoing into how steam technology can be further optimized for use with renewable fuels and sustainable energy sources. For example, steam engines could play an integral role in carbon capture and storage (CCS) technologies, which aim to reduce greenhouse gas emissions by trapping carbon dioxide produced by industrial processes. By pairing steam engines with CCS systems, it may be possible to reduce the environmental impact of steam-based power generation while maintaining its usefulness as a reliable energy source.
Steam engine technology, while rooted in the industrial revolution, continues to evolve. The continuous efforts to improve efficiency, reduce emissions, and integrate with renewable energy systems ensure that steam remains a viable option in the modern energy landscape. Whether through new applications in waste heat recovery, renewable energy, or small-scale decentralized power generation, steam engines are proving their adaptability in an era of increasing technological sophistication. They offer a blend of traditional engineering with modern innovations, bridging the gap between the past and the future of energy production.
As industries and researchers continue to explore new methods of utilizing steam in environmentally friendly ways, steam engines are likely to remain a critical element in a variety of applications, contributing to the development of clean energy technologies and supporting sustainable industrial growth.
High-Efficiency Steam Engines for Power Generation
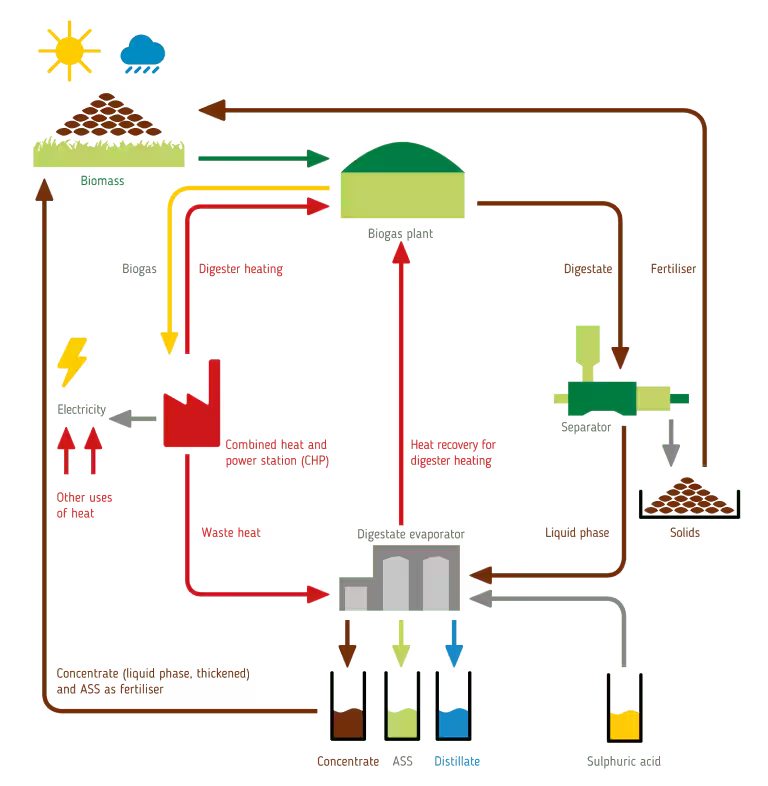
High-efficiency steam engines for power generation represent a crucial aspect of modern energy systems. As the demand for cleaner and more efficient power generation continues to rise, steam engines—particularly steam turbines—remain central to a variety of power plants. These systems are engineered to extract the maximum possible energy from steam while minimizing fuel consumption and reducing environmental impact. Here’s an overview of the key components and strategies for achieving high-efficiency steam engines in power generation:
Supercritical and Ultra-Supercritical Steam Cycles
One of the most significant advancements in steam engine technology for power generation is the development of supercritical and ultra-supercritical steam cycles. These cycles use steam at extremely high pressures and temperatures, significantly improving the efficiency of the power plant.
- Supercritical steam refers to steam that has been heated beyond its critical point, where there is no clear distinction between the liquid and vapor phases. This allows for higher energy transfer efficiency because the steam can expand more effectively in the turbine.
- Ultra-supercritical steam operates at even higher pressures and temperatures than supercritical steam. By increasing the temperature and pressure of the steam, ultra-supercritical cycles reduce the energy losses that occur during the expansion process in the turbine. This leads to even higher thermodynamic efficiency, making ultra-supercritical steam engines ideal for power plants that need to operate at a large scale while minimizing fuel consumption.
These high-efficiency cycles are especially important in coal-fired power plants and nuclear power plants, where increasing the efficiency of the steam cycle directly translates to reduced fuel requirements and lower emissions.
Regenerative Rankine Cycle
The Rankine cycle is the fundamental thermodynamic cycle used in most steam engines for power generation. It involves the boiling of water to create steam, which drives a turbine to produce mechanical power, followed by condensation back into liquid water to repeat the process. To improve efficiency, the regenerative Rankine cycle is often employed.
In a regenerative Rankine cycle, heat from the exhaust steam is used to preheat the feedwater before it enters the boiler. This process reduces the amount of fuel needed to heat the water to its boiling point, thus increasing the overall efficiency of the cycle. The feedwater heater serves as the key component in this cycle, improving thermal efficiency by recovering heat from the exhaust steam and using it to increase the temperature of the incoming water.
Combined Heat and Power (CHP) Systems
High-efficiency steam engines are also integrated into combined heat and power (CHP) systems, which simultaneously produce electricity and usable heat. By capturing and utilizing waste heat that would otherwise be lost, CHP systems achieve efficiencies that can exceed 80%, far higher than conventional power generation methods that only produce electricity.
In CHP systems, the steam engine’s exhaust is routed to heat exchangers or district heating systems to provide thermal energy for nearby industrial processes or residential heating. This waste heat recovery significantly improves the energy efficiency of the overall system, making it a sustainable option for power generation.
Steam Turbine Technology
Steam turbines are at the heart of most high-efficiency steam engines used in power plants. Modern steam turbines are designed with precision to extract as much energy as possible from the steam, ensuring minimal energy losses and optimizing overall performance. Here are some ways in which steam turbine technology is evolving to enhance efficiency:
- Multi-stage turbines: Modern turbines use multiple stages, with steam being expanded progressively across several turbine blades. This staged expansion allows for a more controlled and efficient conversion of steam energy into mechanical work.
- Advanced materials: New high-temperature alloys and ceramic coatings are being developed to withstand the extreme conditions of supercritical and ultra-supercritical steam cycles. These materials ensure that the steam turbine components remain strong and reliable, even at elevated pressures and temperatures.
- Improved blade designs: Advances in blade aerodynamics, materials, and cooling techniques enable steam turbines to operate at higher efficiencies by reducing friction and optimizing steam flow through the turbine stages.
Condensing and Non-Condensing Turbines
Steam engines used in power generation often fall into two categories based on whether they employ a condenser or not:
- Condensing turbines: These turbines are typically used in thermal power stations, where the exhaust steam is cooled and condensed back into water. The condenser creates a vacuum, which allows the steam to expand more fully in the turbine, thereby increasing efficiency. Condensing turbines are particularly effective when there is a demand for high electrical output.
- Non-condensing turbines: Also called back-pressure turbines, these are typically used in combined heat and power (CHP) systems where the exhaust steam is not condensed but instead used for district heating or industrial processes. Non-condensing turbines are ideal for smaller-scale applications where both heat and power are required.
Advanced Control Systems and Automation
To further enhance the efficiency of steam engines in power generation, modern control systems and automation play a crucial role. These systems monitor various parameters like steam pressure, temperature, and flow rate in real-time and adjust the operation of the steam engine accordingly. For example, in a high-efficiency power plant, the control system can optimize the fuel input based on the demand for electricity, ensuring that the steam engine operates at peak efficiency under varying loads.
Automated systems also help minimize wear and tear by adjusting the operation of the engine to reduce stresses on critical components. This not only increases efficiency but also extends the operational life of the steam engine and associated equipment.
Carbon Capture and Storage (CCS) Integration
One of the growing concerns with traditional steam engines, particularly in fossil-fuel power plants, is their contribution to carbon emissions. To address this, many modern steam engines are being integrated with carbon capture and storage (CCS) technologies, which capture CO2 emissions from the exhaust gases before they are released into the atmosphere.
- Pre-combustion capture involves capturing CO2 before fuel is burned, typically through gasification of coal or natural gas. The CO2 is then separated and stored.
- Post-combustion capture involves capturing CO2 from the flue gases after combustion. This process uses solvents or other materials to absorb the CO2 before the exhaust is released.
By capturing and storing carbon emissions, steam engines in power plants can operate more sustainably, even when burning fossil fuels. This is a key technology for helping countries meet international climate goals and reduce their carbon footprint.
Renewable Integration
Another exciting development is the integration of steam engines with renewable energy sources. For instance, biomass power plants use organic materials, such as wood or agricultural waste, to generate steam. These plants can use high-efficiency steam engines to convert the biomass energy into electricity and heat. This approach offers a renewable alternative to traditional fossil fuels and contributes to a circular economy.
In addition, concentrated solar power (CSP) systems use large mirrors or lenses to focus sunlight on a central receiver, where the heat is transferred to a working fluid (often water) that generates steam. This steam then drives a turbine to generate electricity. Steam engines in CSP systems can be highly efficient, especially when combined with thermal storage technologies, allowing power generation even when the sun is not shining.
Conclusion
High-efficiency steam engines are central to modern power generation, offering an effective and flexible way to produce electricity and heat with minimal environmental impact. With innovations such as supercritical and ultra-supercritical cycles, CHP systems, and advanced turbine designs, the efficiency of steam engines continues to improve. The integration of automation, carbon capture technologies, and renewable energy sources further enhances their role in sustainable power generation. As global energy demands grow and the need for cleaner, more efficient systems becomes more pressing, high-efficiency steam engines will remain an essential component in meeting these challenges while reducing the environmental footprint of power generation.
High-efficiency steam engines continue to evolve in response to growing energy demands and environmental concerns. One of the most significant advancements in steam engine technology is the integration of renewable energy sources and alternative fuels. This integration helps reduce dependence on fossil fuels while maintaining the core advantages of steam power. For instance, the use of biomass as a fuel for steam engines offers a renewable and carbon-neutral alternative to traditional coal and natural gas. Biomass power plants, which burn organic materials such as wood, agricultural waste, and dedicated energy crops, produce steam that can drive turbines and generate electricity. The use of these renewable resources helps mitigate the environmental impact of power generation and supports the transition to a more sustainable energy future.
Moreover, the flexibility of steam engines makes them well-suited for integration with various forms of energy storage systems. In some modern power plants, steam engines can operate alongside thermal energy storage systems, such as molten salt or phase-change materials, which store excess heat generated during periods of low demand. This stored thermal energy can later be used to generate steam when demand rises, allowing for more consistent and reliable power generation, even in the face of fluctuations in energy supply or demand. This integration enhances the grid stability and supports renewable energy sources like solar and wind, which can be intermittent.
In the broader context of energy transition, steam engines are also contributing to the development of low-carbon technologies. For example, when coupled with carbon capture, utilization, and storage (CCUS) technologies, steam engines can help mitigate the greenhouse gas emissions associated with conventional power generation. By capturing CO2 before it is released into the atmosphere, these systems can reduce the carbon footprint of steam-based power generation. Some advanced steam plants are also exploring the use of hydrogen as a fuel, with hydrogen combustion offering the potential for zero-emission steam generation. In this context, hydrogen-powered steam engines are an exciting area of research and development, offering a pathway to decarbonize industries that rely on steam power.
As steam engine technology continues to advance, digitalization and smart grid integration are playing an increasingly important role in improving operational efficiency and flexibility. The use of advanced sensor networks, real-time monitoring systems, and predictive analytics enables plant operators to optimize steam engine performance, anticipate maintenance needs, and reduce downtime. These technologies allow for a more responsive and adaptive approach to power generation, enabling plants to adjust to changing load demands, fluctuations in fuel prices, or disruptions in supply chains. This also helps ensure that power plants operate within optimal parameters, enhancing efficiency and extending the lifespan of critical components.
Another key trend is the modularization of steam engine systems, particularly in the context of smaller-scale or distributed power generation. Modular steam engines are being designed to allow for easy scalability and quicker deployment in various settings, from remote communities to industrial facilities. These modular systems can be customized to meet specific power needs, whether it’s for a small microgrid or a large-scale industrial operation. The ability to easily scale and adapt steam engine systems offers significant advantages in terms of cost-effectiveness, installation time, and operational flexibility, especially in developing regions where reliable access to the grid may be limited.
In addition to their traditional use in power plants, steam engines have found applications in district heating systems, where they provide a reliable source of heat for residential and commercial buildings. These systems are particularly beneficial in urban areas, where centralized heating plants can efficiently supply steam to multiple buildings. By utilizing high-efficiency steam engines, district heating systems can achieve substantial reductions in energy consumption and greenhouse gas emissions while providing a sustainable alternative to individual heating solutions like electric or gas boilers.
Looking forward, the role of steam engines in clean energy and sustainable power generation will continue to expand. As more industries and nations prioritize carbon neutrality and seek ways to reduce the environmental impact of their energy systems, steam engines—especially those operating on high-efficiency cycles and renewable fuels—will remain an essential part of the energy mix. The ability to adapt steam engines to utilize a wide range of fuels, including biomass, hydrogen, and synthetic fuels, positions them as a flexible and enduring technology in the global transition to a low-carbon economy.
Ultimately, high-efficiency steam engines will continue to serve as a vital component in addressing the challenges of climate change, energy security, and sustainable development. Their ability to convert heat into mechanical energy with minimal waste, combined with ongoing advancements in efficiency, automation, and integration with renewable energy sources, ensures that steam engines will remain a cornerstone of modern power generation. Whether for large-scale electricity generation, industrial processes, or localized energy systems, high-efficiency steam engines will continue to support global efforts to create a more sustainable, resilient, and efficient energy landscape.
As the global energy landscape continues to evolve, the role of high-efficiency steam engines will become increasingly significant in addressing both energy demands and environmental goals. One of the key aspects of this transition is the integration of steam engines with renewable energy systems. As more countries and industries strive for a decarbonized future, combining steam engines with renewable resources such as solar, wind, and geothermal energy offers a pathway to sustainable power generation. For example, solar thermal power plants use concentrated solar power (CSP) to generate heat, which is then converted to steam that drives turbines. This combination of solar energy and steam power offers a reliable and environmentally friendly alternative to conventional fossil-fuel-based power plants.
Additionally, geothermal energy is another renewable resource that pairs well with steam engines. Geothermal power plants tap into the earth’s natural heat to produce steam, which drives turbines to generate electricity. This process offers an efficient and consistent energy source that is not subject to the fluctuations of weather or time of day, unlike solar or wind energy. The integration of high-efficiency steam engines in geothermal power generation maximizes the potential of this sustainable energy source, providing a steady flow of electricity with minimal environmental impact.
Another exciting development is the use of hybrid systems that combine steam engines with other renewable technologies. For instance, biogas or waste-to-energy facilities can utilize organic waste materials, such as agricultural by-products or landfill gas, to generate steam. These renewable fuels can be burned in high-efficiency steam engines to produce electricity and heat. In such hybrid systems, the steam engine acts as a versatile workhorse, converting heat from various renewable sources into valuable power while minimizing reliance on fossil fuels.
Energy storage systems, such as battery storage and thermal storage, will also play an important role in the future of steam engine-powered systems. As intermittent renewable energy sources like solar and wind become more prevalent, efficient energy storage technologies will be crucial to ensuring a stable and reliable energy supply. Steam engines can be integrated with thermal energy storage systems, which store excess heat generated during times of low demand. This stored thermal energy can be used to produce steam when demand increases or when renewable energy supply drops, providing a constant and stable source of power.
In industries such as manufacturing, refining, and chemical production, steam engines will continue to serve as key components for both power generation and process heat. The flexibility of steam engines allows them to be used in diverse industrial applications where consistent and reliable steam is essential for processes like distillation, sterilization, drying, and material handling. By using high-efficiency steam engines, these industries can reduce energy consumption, lower operational costs, and improve overall productivity while also reducing their carbon footprint.
Furthermore, modular steam engine systems are gaining popularity as they offer advantages in terms of scalability and adaptability. Small-scale, modular systems are increasingly being deployed in remote and off-grid areas where conventional energy infrastructure is unavailable or unreliable. These modular systems can be easily scaled to meet specific energy demands and provide power for communities, industrial facilities, and even remote military bases. This adaptability is especially important in developing regions where decentralized and localized energy solutions are needed to support economic growth and improve living standards.
The ongoing trend of digitalization and smart technologies is also transforming steam engine operations. By incorporating Internet of Things (IoT) sensors, predictive maintenance, and machine learning algorithms, steam engine systems can be monitored and optimized in real-time to ensure maximum efficiency and performance. Predictive maintenance, for example, allows plant operators to anticipate equipment failure before it happens, reducing downtime and preventing costly repairs. Similarly, machine learning can be used to fine-tune system performance based on real-time data, improving the overall efficiency of the steam engine.
Another aspect of high-efficiency steam engines is their role in industrial decarbonization. As industries work towards reducing their carbon emissions, steam engines can be adapted to run on clean fuels like hydrogen. Hydrogen combustion offers the potential for steam production without producing carbon emissions. When hydrogen is used as a fuel source in steam engines, it burns cleanly, releasing only water vapor as a byproduct. This makes hydrogen-powered steam engines an ideal option for industries aiming to decarbonize their operations. In combination with carbon capture technologies, hydrogen-powered steam engines could contribute to a net-zero carbon future, enabling industries to continue their operations while meeting stringent environmental regulations.
In transportation, while steam engines have largely been replaced by more modern technologies like diesel and electric engines, there are still niche applications where steam engines have relevance. For example, some heritage railways and steam-powered ships continue to operate around the world, drawing tourists and enthusiasts alike. These operations, while not widespread, help preserve the historical significance of steam technology and demonstrate the enduring appeal of steam-powered systems. Additionally, steam technology is often celebrated in museums, educational settings, and exhibitions, offering a unique way to engage the public with the history of industrialization and the evolution of energy production.
Finally, global efforts to address climate change will increasingly rely on the integration of low-carbon technologies such as high-efficiency steam engines. As the international community works toward achieving carbon neutrality, steam engines that run on renewable fuels, hydrogen, or biomass will become more prominent in achieving energy targets and reducing global greenhouse gas emissions. The adoption of these technologies, combined with smart grid solutions and energy storage systems, will help balance power generation and consumption, ensuring that energy systems remain reliable, sustainable, and environmentally friendly.
In conclusion, high-efficiency steam engines will continue to play a vital role in the future of power generation, industrial applications, and renewable energy integration. The development of advanced steam cycles, automation, and digital monitoring will drive further efficiency improvements, making steam engines a cornerstone of modern energy systems. Whether in large-scale power plants, small modular systems, or industrial processes, these versatile and reliable engines will be integral in meeting the world’s energy needs while reducing environmental impact and supporting the transition to a low-carbon future.
Steam Engine Applications in Modern Industry
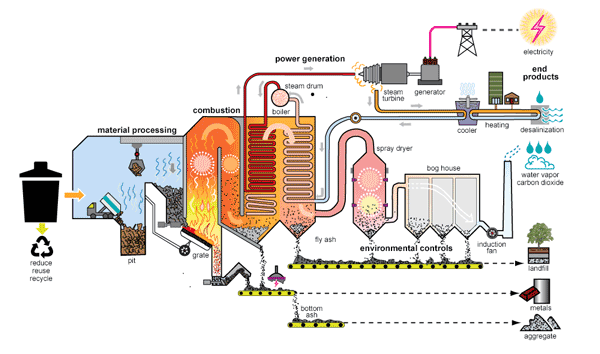
Steam engines, particularly in the form of steam turbines and steam boilers, continue to play a significant role in modern industrial applications. Despite the rise of newer technologies like gas and electric motors, steam engines remain integral in several industries due to their efficiency, versatility, and the high energy demands of certain industrial processes. Here are some of the key applications of steam engines in contemporary industry:
Power Generation
One of the most common applications of steam engines in modern industry is in power plants, where steam turbines are used to generate electricity. Power plants, particularly those fueled by coal, natural gas, and nuclear energy, rely on steam turbines to convert heat energy into mechanical energy, which is then used to drive a generator and produce electricity. In these facilities, water is heated to produce steam, which is then expanded through the turbine blades to generate power.
- Coal-fired power plants: Steam engines continue to be used in coal-fired power plants, although the industry is increasingly transitioning to cleaner energy sources.
- Nuclear power plants: Nuclear reactors use heat from nuclear fission to produce steam, which drives steam turbines. These plants provide a large, stable source of power.
- Renewable energy plants: Steam turbines are also used in geothermal and solar thermal power plants, where steam is produced using renewable heat sources.
Oil Refineries and Petrochemical Industry
In oil refineries and petrochemical plants, steam engines are employed for both power generation and process heat. Steam is used extensively in the refining process, such as for distillation, cracking, and heating. The large amounts of steam required for these processes are typically generated by steam boilers, which convert water into steam using the heat from burning fuels or through other processes.
Steam turbines are also commonly used to drive compressors, pumps, and other equipment in refineries and chemical processing plants. The ability to combine both mechanical power and heat in one system makes steam an efficient and flexible solution for these industries.
Food and Beverage Industry
In the food and beverage industry, steam is essential for many processing tasks, including cooking, sterilization, and drying. Steam is also used in pasteurization processes for beverages like milk, juice, and canned foods, where high heat is needed to eliminate harmful bacteria and extend shelf life.
- Steam boilers: Provide the necessary heat for these operations. They are used to heat water and generate steam, which is then directed into cooking and sterilizing equipment.
- Energy efficiency: Steam engines in food processing plants are often designed to be highly energy-efficient, helping reduce fuel costs and minimize environmental impact.
Textile Industry
The textile industry uses steam in various stages of fabric processing, including dyeing, finishing, and pressing. Steam provides the high temperature and moisture required for processes like steam setting, where heat and steam help to stabilize fabrics, particularly those made from synthetic fibers.
- Steam-powered machinery: Steam engines are used to drive the machinery that prepares textiles, such as looms, spinning machines, and pressing units.
- Boiler systems: Steam boilers generate the steam needed for these operations, which are typically integrated into the overall energy systems of textile mills.
Paper and Pulp Industry
The paper and pulp industry relies heavily on steam for both energy production and process heat. Steam engines and boilers are used throughout the pulp and paper production process, from cooking wood chips to creating paper sheets.
- Steam is used for cooking wood chips: In the pulping process, steam helps break down the raw material into fibers.
- Drying: After the paper has been formed, it must be dried using large steam-heated rollers.
- Energy generation: Steam turbines are often used to generate electricity from the heat produced in the process, making paper mills more energy-efficient and reducing their overall energy consumption.
Chemical Manufacturing
In the chemical industry, steam is essential for many operations, such as reaction heating, distillation, vaporization, and solvent recovery. Steam is also used to power the compressors and pumps that are integral to the smooth operation of chemical plants.
- Process heat: Steam is often used in reactors and vessels to provide the heat required for chemical reactions, such as in the production of fertilizers, plastics, and pharmaceutical products.
- Steam turbines: These are used to power various types of equipment, particularly when the plant needs to maintain a continuous and reliable energy supply.
Heating Systems
In large-scale district heating systems, steam engines play a key role in supplying heat to multiple buildings, such as residential complexes, schools, hospitals, and industrial facilities. Steam is generated centrally in a steam boiler plant and then distributed through a network of insulated pipes to provide heating and hot water.
- Combined Heat and Power (CHP): Many district heating systems use CHP plants, where a steam engine (usually a steam turbine) generates both electricity and heat from the same energy source. This dual-output system maximizes energy efficiency and minimizes waste.
Industrial Applications
Many other industries use steam engines for various applications that require large amounts of heat or power. For example:
- Cement plants: Steam is used for drying and heating raw materials during cement production.
- Metallurgical industries: Steam is used for heating processes, such as in the production of steel, where high temperatures are needed to refine metals.
- Mining operations: Steam engines can be used in mineral processing plants to power equipment, heat materials, or generate electricity.
Marine and Shipping Industry
Steam engines continue to play a role in the marine industry, particularly in steamships and certain cargo vessels. While they have largely been replaced by more modern diesel engines in most commercial shipping applications, steam engines are still used in some specialty vessels or historical ships.
- Naval applications: In the military, steam engines were historically used to power warships, although most navies have now switched to gas turbines or diesel-electric systems.
- Tourist ships: Many heritage or luxury tourist steamships still operate, maintaining steam engines as part of the traditional appeal.
Pharmaceutical Industry
In the pharmaceutical industry, steam is essential for sterilization and autoclaving processes, which are critical for ensuring the safety and purity of medical products. Steam is used to sterilize equipment, vials, and other tools used in drug production, ensuring that no contamination occurs during the manufacturing process.
- Steam autoclaves: Used to sterilize equipment by exposing them to high-pressure steam, which kills microorganisms and ensures sterility in pharmaceutical production.
- Energy generation: Steam engines are also used in pharmaceutical plants to generate the heat needed for various chemical processes and manufacturing steps.
Sustainable Applications
With the growing emphasis on sustainability, many industries are looking for ways to integrate renewable and low-carbon technologies into their steam engine systems. For example:
- Biomass-fired steam engines: Biomass, including wood chips, agricultural waste, and other organic materials, is used as a renewable fuel source for steam boilers and turbines.
- Waste-to-energy plants: These plants use waste materials, such as municipal solid waste, to generate steam and power.
- Hydrogen-powered steam engines: Hydrogen, as a clean fuel source, is being explored for use in steam engines, especially in industries aiming for carbon neutrality.
In these applications, steam engines help industries reduce their carbon footprint, lower energy costs, and make more efficient use of available resources.
Conclusion
Steam engines, despite being a technology rooted in the industrial revolution, remain highly relevant in modern industry. Their versatility and ability to provide both mechanical power and heat make them indispensable across a wide range of sectors, from power generation and manufacturing to food processing and chemical production. As industries strive for greater energy efficiency and sustainability, steam engines will continue to evolve, playing a key role in meeting the world’s growing energy needs while supporting efforts to reduce environmental impact and optimize resource use.
Steam engines continue to be a vital part of modern industrial operations, offering both versatility and efficiency across various sectors. In power generation, steam turbines are the cornerstone of traditional fossil-fuel-based plants, such as coal, natural gas, and nuclear power stations. These plants rely on steam engines to convert thermal energy into mechanical power, which then drives generators to produce electricity. More recently, renewable sources like geothermal and solar thermal plants have also adopted steam turbines, demonstrating their adaptability to sustainable energy solutions. The integration of steam systems with renewable energy sources, such as solar thermal and biomass, further highlights the role of steam engines in the transition to cleaner power generation.
In the oil refining and petrochemical industries, steam plays a crucial role in both power generation and process heating. Refining processes like distillation, cracking, and sterilization require large amounts of steam, which is typically generated by boilers fueled by natural gas, oil, or other sources. Steam engines also power compressors, pumps, and other essential equipment in these high-demand environments, contributing to the efficiency and smooth operation of chemical processes. The chemical manufacturing sector similarly relies on steam for reaction heating, distillation, and solvent recovery, where steam systems are used to regulate temperatures and facilitate necessary chemical transformations.
The food and beverage industry is another key area where steam is indispensable. It is used extensively in cooking, sterilization, pasteurization, and drying. Steam-powered machinery is essential for the manufacturing of food products that require precise temperature control, such as beverages, canned goods, and dairy products. For example, milk and juices are pasteurized using steam to kill harmful bacteria, which extends shelf life and ensures safety. Similarly, the textile industry utilizes steam for processes like dyeing, finishing, and pressing fabrics, where high temperatures and moisture are required to treat materials efficiently.
The paper and pulp industry uses steam in a variety of ways, from cooking wood chips to create pulp to drying the paper during production. Steam boilers are integral in these processes, providing consistent heat and allowing for the efficient use of energy in large-scale manufacturing. Steam turbines also contribute to energy generation in these plants, helping them optimize power usage and reduce overall energy costs. In the same way, the pharmaceutical industry uses steam for sterilization purposes, particularly in the form of steam autoclaves, which ensure that production equipment and materials remain free from contaminants.
The cement and metallurgical industries are heavy users of steam for heating and drying raw materials. In cement manufacturing, steam helps process materials at high temperatures, while in metal processing, it is used for heating and refining metals. Steam engines also power equipment in both of these sectors, making steam a vital part of their operations. Mining operations, similarly, rely on steam-powered systems for energy generation and for heat-intensive processing activities.
Steam engines are also integral to district heating systems in urban areas, where centralized steam production is used to provide heat and hot water to multiple buildings. These systems reduce the need for individual heating solutions and improve energy efficiency on a large scale. Combined heat and power (CHP) systems, which generate both heat and electricity from steam engines, have gained popularity due to their ability to maximize energy use and minimize waste.
In marine transportation, although steam engines have largely been replaced by more modern diesel and electric systems, they still play a role in certain heritage ships and luxury tourist vessels. These applications preserve the historical significance of steam-powered transportation while offering a unique experience for passengers.
Industries are increasingly turning to low-carbon technologies to reduce their environmental impact. Biomass-fueled steam engines and waste-to-energy systems provide a sustainable source of energy, using organic materials or waste products as fuel to generate steam. Hydrogen-powered steam engines are another promising development, offering the potential for clean energy with zero emissions when hydrogen is used as a fuel.
The integration of steam engines with digital technologies is another key trend. The use of smart sensors, real-time monitoring, and predictive maintenance tools has made steam engine systems more efficient and reliable. These innovations allow for greater control over energy use and system performance, reducing downtime and improving overall productivity. Industrial facilities can now optimize steam usage, predict when maintenance is needed, and ensure that equipment operates at peak efficiency.
As industries continue to focus on sustainability, steam engines are evolving to meet these demands. Their flexibility, ability to integrate with renewable energy systems, and the development of high-efficiency technologies make them a cornerstone of modern industrial operations. Whether it’s in power generation, food processing, or chemical manufacturing, steam engines are essential in keeping industries running smoothly while supporting the transition to cleaner, more efficient energy solutions. Their role will remain vital as industries seek ways to balance economic growth with environmental stewardship, ensuring that steam remains a key player in global efforts to reduce carbon emissions and promote sustainable energy practices.
As the focus on sustainability and energy efficiency continues to grow, steam engines are adapting to meet new demands. One of the most significant areas of development is the integration of advanced materials and designs to enhance the efficiency and performance of steam systems. The use of superalloys, ceramic composites, and high-temperature-resistant materials allows steam engines to operate at higher pressures and temperatures, improving the overall thermodynamic efficiency of the steam cycle. This development helps reduce fuel consumption and emissions, making steam engines more competitive with other power generation technologies in terms of efficiency and environmental impact.
The growing trend of decentralized energy systems also plays a crucial role in the continued relevance of steam engines. As industries and even communities look to become more energy independent, steam engines are being deployed in small-scale, modular power generation units. These systems can operate using renewable fuels or biomass, providing a sustainable and localized energy source. Microgrids and distributed generation systems, which rely on smaller, decentralized power generation sources, often integrate steam engines to provide reliable power and heat. Such systems offer flexibility, especially in remote or off-grid areas, where large-scale power plants are not feasible.
In addition, the role of steam engines in industrial decarbonization is becoming increasingly important. The push for industries to reduce their carbon footprint has led to the exploration of low-carbon fuels and green hydrogen for steam engine applications. Hydrogen combustion in steam boilers and turbines produces only water vapor as a byproduct, offering a clean alternative to conventional fossil fuels. Hydrogen-powered steam engines could significantly contribute to industrial decarbonization efforts, particularly in heavy industries that require high amounts of heat and power, such as steel manufacturing, cement production, and chemical processing.
As the world transitions to a more sustainable future, carbon capture and storage (CCS) technologies are being integrated with steam engine systems. By capturing and storing CO2 emissions from steam engines, particularly those in industrial settings, it becomes possible to reduce the environmental impact of these systems. In combination with clean fuels like hydrogen or biofuels, carbon capture technologies could enable steam engines to operate in a carbon-neutral or even carbon-negative manner, making them a critical component of global climate change mitigation strategies.
In the realm of transportation, while steam engines are no longer the predominant technology in mainstream applications like railroads or shipping, their legacy persists in niche areas. Heritage railways, steam-powered boats, and other vintage vehicles continue to capture the public’s imagination, with enthusiasts and tourists experiencing the charm and historical significance of steam-powered transportation. Beyond the nostalgic appeal, the technology continues to serve as a reminder of the engineering ingenuity that laid the foundation for modern industrial progress.
The research and development (R&D) landscape for steam engines is also thriving. Engineers are working on more advanced steam cycles, such as Rankine cycle modifications, that allow for higher efficiency at lower costs. These developments include improvements in heat exchangers, turbine designs, and thermodynamic cycles that enable steam engines to generate more power from less fuel. Furthermore, as industries demand better thermal management solutions, steam engines continue to evolve to meet the growing need for energy-efficient systems that minimize waste and increase output.
Looking to the future, steam engines will remain a key player in industries requiring high-temperature processes and large-scale power generation. The ability to use renewable fuels such as biomass, hydrogen, and waste products in steam engines ensures their continued relevance as the world shifts toward cleaner energy sources. Whether in heavy industry, energy production, or green technologies, steam engines are poised to play an essential role in the coming decades, driving innovation in energy systems that are both efficient and sustainable.
Additionally, the rising trend of circular economies is opening up new avenues for steam engines, particularly in the waste-to-energy sector. As industries and municipalities seek to make better use of waste materials, steam engines can convert these materials into useful energy, helping to close the loop on resource consumption. This approach not only supports sustainability efforts but also contributes to reducing landfill waste, offering a practical solution to urban waste management challenges.
Digitalization also plays a role in optimizing steam engine performance. With the integration of IoT (Internet of Things) devices, steam engines can be monitored remotely to provide real-time data on their performance. Predictive analytics can identify potential maintenance issues before they become critical, ensuring continuous operation and minimizing downtime. Advanced control systems can adjust operating parameters dynamically, improving the overall efficiency and lifespan of steam systems.
The flexibility of steam engines makes them well-suited for a variety of applications, whether for centralized power generation, industrial heat, or renewable energy integration. By optimizing these engines for modern needs, industries can leverage their capacity to meet stringent efficiency standards while reducing their environmental footprint. This adaptability will ensure that steam engines continue to play an important role in the global transition to more sustainable and resilient energy systems.
In conclusion, steam engines are not a relic of the past but an essential component of the future industrial landscape. With their ability to adapt to new fuel sources, improve efficiency, and integrate with emerging technologies, steam engines will continue to power industries, support renewable energy solutions, and contribute to decarbonization efforts. As innovation in materials, design, and digitalization continues, steam engines will remain a vital part of global efforts to create a sustainable and energy-efficient future.
Manufacturing Steam Engines for Renewable Energy
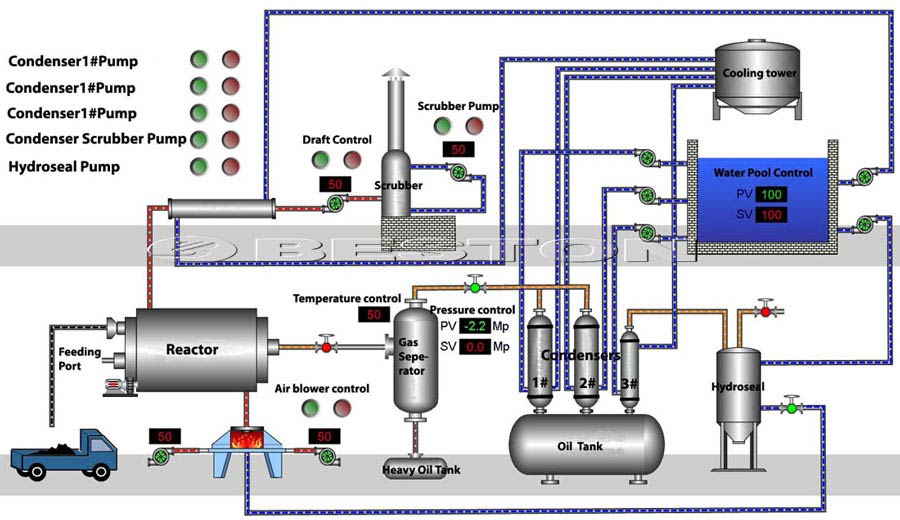
Manufacturing steam engines for renewable energy applications is an exciting and emerging area of interest, driven by the need for sustainable and efficient power generation solutions. As the world shifts toward cleaner, more sustainable energy systems, steam engines offer significant potential, especially when paired with renewable energy sources such as biomass, geothermal energy, and solar power. Here’s an overview of the manufacturing processes, technologies, and considerations involved in creating steam engines for renewable energy:
1. Biomass-Fueled Steam Engines
One of the most promising applications for steam engines in renewable energy is the use of biomass as a fuel source. Biomass includes organic materials such as wood chips, agricultural residues, and waste from food processing, which can be burned to produce steam. The steam then drives turbines or engines to generate electricity or provide heat for industrial processes.
- Fuel Selection: The first step in manufacturing steam engines for biomass applications is selecting the appropriate feedstock. Biomass needs to be carefully processed to ensure it meets the necessary specifications for efficient combustion. This can include drying, grinding, and pelletizing the biomass to ensure it burns consistently and efficiently.
- Boiler Design: The biomass is burned in a boiler, which generates the steam. Boilers for biomass need to be designed to handle the specific challenges of burning organic fuels, such as higher moisture content and ash production. Manufacturing such boilers requires advanced materials capable of withstanding high temperatures and corrosive elements found in biomass fuels.
- Steam Engine/Turbine Integration: The steam produced in the biomass boiler is used to drive a steam turbine or steam engine. Manufacturing these engines involves creating efficient, durable turbines that can operate effectively with the relatively lower energy density of biomass compared to fossil fuels. This often requires optimizing the steam cycle for maximum efficiency.
- Energy Efficiency: Manufacturing for renewable energy applications often includes the development of combined heat and power (CHP) systems. In CHP plants, steam engines not only generate electricity but also provide heat for industrial processes or district heating systems, maximizing the overall energy efficiency of the system.
2. Geothermal Steam Engines
Geothermal energy, which utilizes heat from the Earth’s interior, is another renewable energy source that pairs well with steam engine technology. In geothermal power plants, steam is generated from underground reservoirs of hot water or steam, which is then used to power steam turbines.
- Geothermal Wells: The geothermal process begins with the drilling of deep wells to access hot water or steam reservoirs. The steam from these wells is then brought to the surface and directed toward the steam turbine.
- Steam Turbines: The design and manufacturing of steam turbines for geothermal energy require specialized components that can handle high temperatures and pressures, which are common in geothermal systems. Materials used in these turbines must be highly resistant to corrosion and wear due to the presence of minerals and gases in geothermal steam.
- Low-Temperature Geothermal Systems: In certain locations, geothermal sources are not hot enough to produce steam directly. In these cases, binary cycle power plants are used, where heat from geothermal sources is transferred to a secondary fluid with a lower boiling point, which then produces steam to drive turbines. Manufacturing steam engines for these systems requires careful selection of materials and design to ensure efficiency.
3. Solar Thermal Steam Engines
Solar thermal power plants use concentrated sunlight to heat a fluid, which produces steam that drives a turbine to generate electricity. There are two primary types of solar thermal systems: parabolic trough and tower systems. Both use solar energy to generate steam, which then powers steam engines.
- Concentrated Solar Power (CSP): In CSP systems, mirrors or lenses concentrate sunlight onto a fluid, which is then heated to produce steam. The manufacturing of steam engines for CSP systems involves designing turbines and boilers that can operate efficiently with the heat produced from solar energy.
- Material Considerations: Solar thermal systems often operate at high temperatures, which means that materials used for steam engines and turbines must be able to withstand prolonged exposure to intense heat. This requires advanced alloys and heat-resistant coatings to ensure longevity and reliability.
- Integration with Energy Storage: Solar power can be intermittent, so many solar thermal plants incorporate thermal energy storage systems to store excess heat for later use. Steam engines in these systems must be able to work seamlessly with the storage units to ensure that power can be generated even when the sun isn’t shining.
4. Hydrogen-Powered Steam Engines
Hydrogen is a clean and renewable fuel that, when combusted, produces only water vapor as a byproduct. In hydrogen-powered steam engines, hydrogen can be used as the primary fuel source to generate steam.
- Hydrogen Production: Hydrogen can be produced from renewable energy sources through electrolysis, where electricity generated by wind, solar, or other renewable sources is used to split water into hydrogen and oxygen. This hydrogen can then be used as a fuel in steam boilers and turbines.
- Combustion and Steam Generation: Hydrogen is combusted in a boiler to produce steam, which drives the steam turbine. The design of these systems requires modifications to traditional steam engine technology to account for the specific combustion properties of hydrogen.
- Carbon-Free Power: Hydrogen-powered steam engines are seen as a potential solution for industries and sectors that require high-temperature heat or power but need to operate in a carbon-neutral manner. Their ability to burn clean hydrogen makes them an attractive option for decarbonizing heavy industries, such as steel production or cement manufacturing.
5. Manufacturing Considerations for Renewable Energy Steam Engines
When designing and manufacturing steam engines for renewable energy applications, several key factors must be considered to optimize their efficiency, reliability, and environmental performance:
- Materials: Steam engines used in renewable energy applications must be built from materials capable of withstanding high temperatures, pressures, and potential corrosive environments. For example, advanced superalloys, ceramic materials, and heat-resistant coatings are essential to ensure that steam engines maintain high efficiency over time and can resist the corrosive effects of certain renewable fuels like biomass or geothermal steam.
- Efficiency and Sustainability: The goal of manufacturing steam engines for renewable energy is to maximize efficiency and minimize environmental impact. This includes designing steam engines and turbines that extract as much energy as possible from the steam, reducing fuel consumption and emissions.
- Modularity: Many renewable energy projects, especially in off-grid locations, benefit from modular and scalable steam engine systems. Modular designs allow for easier integration into smaller or decentralized power plants, making it possible to tailor solutions to the specific needs of different communities or industries.
- Advanced Manufacturing Technologies: The use of additive manufacturing (3D printing) and precision casting techniques is becoming more common in the manufacturing of steam engine components. These technologies allow for the creation of complex parts with high precision and efficiency, reducing material waste and enhancing the performance of steam engines in renewable energy systems.
- Maintenance and Durability: Given that many renewable energy systems are intended to operate for long periods with minimal downtime, the design and manufacturing process must prioritize durability and ease of maintenance. Using advanced monitoring and control systems, combined with predictive maintenance technologies, can help optimize the lifespan and reliability of steam engines in renewable energy applications.
Conclusion
The manufacturing of steam engines for renewable energy applications offers a promising pathway toward achieving sustainable, low-carbon energy production. Whether powered by biomass, geothermal, solar thermal, or hydrogen, steam engines can play a vital role in the transition to cleaner energy systems. As technology continues to advance, the ability to integrate steam engines with renewable energy sources will expand, allowing for more efficient, reliable, and eco-friendly energy generation across industries. By leveraging advanced materials, innovative manufacturing techniques, and careful design considerations, steam engines will continue to be an essential tool in the global shift toward sustainable energy.
The evolution of steam engines for renewable energy applications is closely tied to the broader shift towards decarbonization and sustainable energy practices. As industries and governments work toward meeting carbon reduction goals, steam engines offer a reliable and adaptable technology that can support this transition across various sectors. One key advantage of steam engine technology is its ability to integrate with different renewable energy sources, such as biomass, geothermal, solar thermal, and hydrogen. Each of these energy sources has its own unique benefits and challenges, but when combined with steam engines, they can provide consistent, high-quality energy generation with lower environmental impact.
As the renewable energy landscape evolves, the role of steam engines in energy storage and off-grid applications becomes increasingly important. In areas where renewable energy sources like solar and wind are intermittent, steam engines can be used in combination with energy storage systems to ensure a steady supply of power. For example, solar thermal plants can store excess heat in molten salts or other thermal storage mediums, which can then be used to generate steam when the sun isn’t shining. This ability to operate on stored thermal energy enhances the stability and reliability of renewable power systems, making steam engines essential for balancing supply and demand.
The ability of steam engines to operate on a wide range of fuels—whether renewable or waste-derived—also makes them a valuable tool for addressing the challenges of waste management and resource optimization. Waste-to-energy technologies, which convert non-recyclable waste materials into heat and power, rely on steam engines to generate energy from municipal or industrial waste. This not only helps reduce landfill waste but also provides a cleaner alternative to traditional waste disposal methods like incineration. The versatility of steam engines in this context enables industries to adopt more sustainable practices by using waste materials as valuable resources for energy production.
As the global energy sector becomes more digitized, steam engines are also benefiting from advancements in smart grid technologies and real-time monitoring. The integration of digital tools like IoT sensors and predictive analytics allows for better management of steam systems, ensuring optimal performance and energy efficiency. This is particularly important in industrial settings, where downtime can be costly, and systems must operate continuously. The real-time data generated by these systems allows for proactive maintenance, which can extend the lifespan of steam engines and reduce operational costs.
With the rising interest in decentralized energy systems and microgrids, steam engines are being designed to function in smaller, more flexible power generation units that can be deployed in a variety of locations. These decentralized systems provide an alternative to traditional, centralized power plants, offering more localized energy solutions that can better meet the specific needs of communities, industries, and remote areas. The scalability of steam engines makes them an ideal fit for such systems, providing a sustainable energy source that can be easily adapted to different geographic and economic conditions.
At the same time, manufacturers are working on improving the overall efficiency and reliability of steam engines used in renewable energy systems. Advanced design techniques, including the use of high-efficiency turbines and supercritical steam cycles, are pushing the boundaries of steam engine performance. These innovations allow steam engines to extract more energy from the same amount of fuel, which is essential for making renewable energy systems more competitive with conventional fossil fuel-based power generation.
As global energy policies increasingly prioritize clean energy, steam engines will continue to play a significant role in meeting climate targets. The transition to low-carbon fuels, such as biofuels, green hydrogen, and waste-derived energy, will be vital for reducing the environmental impact of industrial sectors that rely on high-temperature processes, such as chemical manufacturing, steel production, and cement production. In these contexts, steam engines provide a cost-effective and technologically adaptable solution for generating the high heat and power required for these energy-intensive operations.
Moreover, as the need for industrial decarbonization grows, steam engines can help bridge the gap between traditional power generation systems and the new, low-carbon energy solutions required for a sustainable future. Hybrid systems that combine steam engines with electricity or natural gas turbines can be designed to provide flexible and efficient energy options that can adapt to varying loads and energy demands. This hybridization of technologies enables industries to reduce reliance on fossil fuels while maintaining reliable energy output.
The future of steam engines in renewable energy will also depend on continued research and development in materials science and manufacturing processes. As renewable energy systems push for greater efficiency, steam engines must meet new demands for higher operating temperatures, lower maintenance costs, and longer lifespans. Engineers are exploring next-generation materials that can withstand extreme operating conditions, including ceramic coatings and advanced composites that can improve heat transfer efficiency and extend the service life of steam turbines and boilers.
Looking forward, steam engines may also become integral to carbon capture technologies. By combining steam engine systems with carbon capture and storage (CCS) systems, it becomes possible to reduce CO2 emissions from industrial processes that rely on steam for power or heat. Steam engines can play a role in both power generation and carbon sequestration by providing the heat needed to drive CCS processes, further contributing to the decarbonization of industries.
In summary, steam engines are not only a historical technology but a vital part of the future energy landscape, especially in the context of renewable energy. Their adaptability, efficiency, and ability to integrate with a wide range of fuels and energy systems make them a key technology for sustainable power generation. As renewable energy sources continue to grow in importance, steam engines will play a central role in enabling industries to meet their energy needs while reducing environmental impacts. With continued innovation in materials, efficiency, and integration with digital technologies, steam engines are poised to remain a cornerstone of modern, low-carbon energy systems.
As renewable energy technologies continue to evolve, steam engines are expected to play an even greater role in a future where sustainable energy solutions are paramount. One of the areas where steam engines are increasingly relevant is in the integration with waste heat recovery systems. Many industrial processes generate excess heat that, if not captured, is released into the environment. By incorporating steam engines into waste heat recovery systems, this excess heat can be repurposed to generate electricity or supply thermal energy, providing an additional layer of efficiency to industrial operations. This kind of system is particularly useful in industries such as glass manufacturing, petrochemical production, and cement manufacturing, where high temperatures are necessary but can also be harnessed to power steam engines for further energy production.
The growing emphasis on energy storage technologies also complements the future role of steam engines in renewable energy applications. For instance, when combined with molten salt storage or high-temperature thermal storage, steam engines can generate power when renewable sources like wind or solar are not producing enough energy. By capturing excess energy during periods of high production and using it later when demand spikes or renewable generation is low, these systems can help stabilize the grid and reduce the need for conventional backup power, which often comes from fossil fuels.
Furthermore, advancements in smart grid systems are facilitating the seamless integration of steam engine-based power generation into larger renewable energy networks. By connecting steam engines to distributed energy resources (DERs), including solar, wind, and storage systems, these engines can contribute to a more resilient, flexible, and efficient energy grid. Through real-time monitoring and advanced control systems, steam engines can respond dynamically to grid demands, ensuring a steady supply of power while minimizing operational inefficiencies. The ability of steam engines to be used as part of a demand-response system will be particularly valuable as the energy transition continues to shift toward more decentralized and renewable energy sources.
The adoption of additive manufacturing (3D printing) for steam engine components is another innovative development that could shape the future of renewable energy steam engines. This technology allows for the creation of complex parts with intricate geometries that would otherwise be difficult or impossible to manufacture using traditional methods. Additive manufacturing enables the use of lightweight materials, precise tolerances, and complex heat exchangers, all of which are important for improving the performance and efficiency of steam engines in renewable energy applications. By reducing manufacturing time, costs, and material waste, 3D printing could revolutionize how steam engines are produced for renewable energy systems, making them more accessible and cost-effective.
In addition to traditional renewable energy applications, the concept of green hydrogen is gaining traction as a promising energy carrier for steam engine systems. Green hydrogen, produced using renewable electricity through electrolysis, can be combusted in steam boilers to produce steam for power generation. As hydrogen infrastructure grows and the cost of hydrogen production decreases, steam engines may become more widely used in hydrogen-based power plants, offering a carbon-neutral option for large-scale power generation.
The ongoing development of carbon-neutral fuels like biofuels and synthetic fuels further complements steam engine technology in the renewable energy landscape. These fuels, which are derived from renewable sources such as algae, plant oils, or agricultural waste, can be used in steam engines much like traditional fossil fuels, but without the associated carbon emissions. By shifting industries toward biofuel-powered steam engines, it’s possible to maintain industrial operations while significantly reducing the carbon footprint of power generation.
On the global stage, countries with abundant renewable resources, particularly in regions with high solar or geothermal potential, can benefit from steam engines as part of their energy mix. In developing countries, especially those with limited access to the grid, steam engines powered by biomass or waste could offer an affordable, localized energy solution. These systems provide a flexible, scalable, and low-maintenance option for power generation in remote areas, improving energy access and supporting economic development while maintaining environmental sustainability.
In highly industrialized regions, steam engines could help meet stricter emissions regulations and energy efficiency targets. Countries are increasingly adopting green building standards and industrial decarbonization initiatives, which incentivize businesses to reduce their environmental impact. Steam engines, when combined with renewable fuels or energy recovery systems, can provide a reliable means for industries to transition to cleaner energy solutions while maintaining their output and competitiveness in the market.
Moreover, the future of steam engine research and development (R&D) is focused on enhancing the thermal efficiency of these systems. Researchers are exploring ways to increase the thermal-to-electric efficiency of steam engines through innovations in supercritical and ultracritical steam cycles, which operate at higher pressures and temperatures than conventional systems. These high-performance cycles extract more energy from the same amount of fuel, reducing waste heat and improving overall efficiency. R&D into advanced turbine technology, superheated steam, and organic Rankine cycles (ORC) will push the limits of what steam engines can achieve in terms of efficiency, size, and cost-effectiveness, especially in renewable energy applications.
The introduction of AI-driven optimization is another technological leap for steam engines. By leveraging machine learning algorithms and big data analysis, steam engines in renewable energy settings can be optimized for maximum efficiency, based on real-time performance data, environmental conditions, and system demands. This predictive maintenance capability will allow operators to anticipate wear and tear before it becomes a major issue, reducing downtime and extending the operational life of steam systems.
As we move further into the 21st century, the combination of advanced steam engine technology, renewable energy sources, and digital tools will be essential in addressing the complex challenges of global energy demand and climate change. The flexibility, adaptability, and efficiency of steam engines make them a key piece of the puzzle in transitioning to a low-carbon, sustainable energy future.
In conclusion, the role of steam engines in renewable energy applications is poised to grow significantly. Whether they are being used in biomass plants, geothermal systems, solar thermal power generation, or waste-to-energy projects, steam engines are becoming increasingly important as industries look for sustainable ways to generate heat and power. The technological advancements in material science, digitalization, and energy storage are making it possible to harness the full potential of steam engines, ensuring their continued relevance in the future of clean energy. Through innovation and investment in sustainable manufacturing practices, steam engines can continue to evolve and contribute to a carbon-neutral future, supporting industries worldwide in their journey toward decarbonization.
Choosing the Right Steam Engine for Your Application
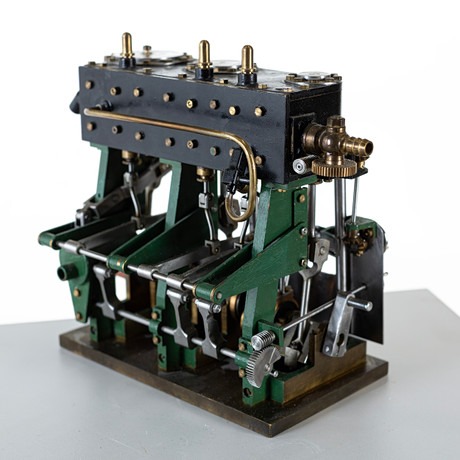
Choosing the right steam engine for a specific application involves several factors, as the efficiency, output, and reliability of the system must align with the intended purpose and the operational environment. Here’s a guide to help you understand the key considerations when selecting the ideal steam engine for your needs:
1. Type of Application
- Power Generation: If you need a steam engine for generating electricity, consider a high-efficiency steam turbine system. These are commonly used in large-scale power plants and often run in combined-cycle systems, where steam is used after a gas turbine.
- Industrial Applications: For manufacturing or industrial applications (such as in cement, steel, or chemical plants), you might need steam engines that can provide both power and heat. Combined Heat and Power (CHP) systems are ideal for this purpose, offering efficient energy use by converting both heat and electricity from steam.
- Heating and Small-Scale Energy: For smaller or off-grid applications, such as rural areas or small industries, biomass or solar-powered steam engines may be a better fit, offering distributed energy and the ability to use renewable sources.
- Transport and Marine Applications: For ships or other transport systems, steam engines designed for mobility and space efficiency are important. These systems need to be compact but robust, handling the rigors of transport while offering consistent power.
2. Energy Source
- Fossil Fuels: Traditional steam engines powered by coal, oil, or natural gas are often used in large-scale power plants or for heavy industrial applications. If you are considering a steam engine powered by fossil fuels, the availability of the fuel and regulatory concerns related to emissions must be considered.
- Renewable Fuels: If you’re looking for sustainable energy options, consider steam engines powered by biomass, waste-to-energy, solar thermal energy, or geothermal. These types of engines help reduce carbon emissions and can be integrated with waste heat recovery or energy storage systems for enhanced efficiency.
- Hybrid or Dual-Fuel Systems: Some systems offer flexibility with hybrid or dual-fuel capabilities, allowing you to switch between renewable and fossil fuel sources depending on availability and cost.
3. Size and Power Output
- Small-Scale vs. Large-Scale Needs: Consider the capacity needed. For smaller applications like residential or small industrial use, you might need a modular steam engine with low power output. Larger systems, like those in power plants or large industrial operations, require high-capacity steam turbines or combined systems that can handle large volumes of steam and generate substantial power.
- Customization: In some cases, the standard off-the-shelf steam engines may not meet the requirements. Custom-designed engines tailored to the specific needs of the facility, such as heat recovery systems or waste-to-energy plants, might be necessary.
4. Efficiency
- Thermal Efficiency: One of the most critical factors to consider is the efficiency of the steam engine. Engines that operate at higher temperatures and pressures will be more efficient, converting more of the fuel’s energy into usable power. Look for engines that incorporate superheated steam or combined cycles for maximum energy extraction.
- Fuel-to-Power Conversion: The fuel-to-power ratio is another important consideration. Ensure the engine is designed to efficiently convert the type of fuel you plan to use into steam, which drives the engine’s turbine or piston.
5. Maintenance Requirements
- Reliability and Durability: Steam engines used in industrial and power generation applications are often in use for long periods without interruption, so durability and reliability are paramount. Choose a steam engine from a reputable manufacturer with a proven track record of building long-lasting engines.
- Maintenance and Servicing: Maintenance schedules and ease of servicing should also factor into your decision. Some engines are designed for low maintenance, while others, particularly in complex systems, may require regular inspections, part replacements, and advanced diagnostics.
- Availability of Spare Parts: Consider the ease of obtaining spare parts. It is essential to choose a steam engine from a manufacturer with a strong supply chain and service network, especially for large-scale applications.
6. Environmental Impact
- Emissions and Pollution: For applications aiming to reduce carbon footprints or meet emissions regulations, consider using a steam engine that operates on renewable fuels or incorporates technologies that capture and reduce emissions, such as carbon capture systems. These technologies may help minimize environmental impacts and contribute to sustainability goals.
- Compliance with Standards: Ensure the steam engine meets any local or international standards regarding emissions, noise levels, and efficiency. Many countries have strict regulations in place to reduce air pollution and encourage the use of clean energy sources.
7. Operational Environment
- Temperature and Pressure Conditions: Depending on your application, the engine may need to operate in extreme conditions. High-pressure and high-temperature environments require specialized materials and engineering. For example, geothermal energy applications often involve steam at higher temperatures, requiring steam engines built to withstand such conditions.
- Corrosive or Harsh Environments: If the steam engine will be operating in a corrosive environment (e.g., marine applications, or regions with high saline levels), ensure the materials used in the engine are resistant to corrosion. This could involve the use of advanced alloys or protective coatings.
8. Cost and Budget
- Initial Investment vs. Long-Term Savings: The upfront cost of a steam engine can vary significantly depending on the complexity of the system and the energy source. While renewable energy-powered engines (such as biomass or solar thermal) may have higher initial installation costs, they often provide long-term savings through lower operational costs and potential subsidies or incentives.
- Operational Costs: Factor in the ongoing operational costs, including fuel consumption, maintenance, and labor. Energy-efficient steam engines can help reduce operational costs, making them more economical in the long run.
- Payback Period: For many businesses, the payback period of the investment is a key decision factor. Look for systems with a shorter return on investment (ROI) through reduced energy costs, increased efficiency, or waste reduction.
9. Integration with Existing Systems
- System Compatibility: Ensure the steam engine you choose is compatible with your existing power generation or industrial systems. For instance, integrating a steam engine into a combined-cycle system requires careful consideration of the design, layout, and control systems.
- Scalability: If you anticipate the need for expansion in the future, select a steam engine that is easily scalable or one that can be integrated with additional units as demand grows.
10. Supplier and Support
- Reputation of the Manufacturer: It’s important to choose a reputable manufacturer with experience in steam engine production. Established companies typically offer better engineering support, customer service, and training.
- Post-Purchase Support: Ensure that the manufacturer provides technical support, warranty services, and assistance with installation and commissioning. The availability of training programs for operators and maintenance personnel is also important for ensuring safe and efficient operation.
Conclusion
Selecting the right steam engine for your application is a complex process that involves balancing efficiency, fuel type, environmental considerations, and cost. By thoroughly understanding your energy needs, operational requirements, and long-term goals, you can make a well-informed decision. Whether you are in power generation, industrial manufacturing, or renewable energy, the right steam engine will help you optimize performance, reduce costs, and contribute to your sustainability efforts. Always keep in mind that long-term success depends on choosing an engine that matches the specific challenges and opportunities of your application.
When selecting a steam engine, it’s essential to evaluate the full lifecycle of the system, from design and installation to operation and eventual decommissioning. Often, the most significant costs aren’t just in the purchase but in ongoing operation and maintenance. This makes it imperative to factor in long-term operational savings and how the system will perform over time, including any potential challenges with wear and tear, system upgrades, or adjustments that might be necessary as fuel sources evolve.
Another aspect to consider is the flexibility of the steam engine in responding to changing energy demands. As industries increasingly prioritize energy resilience and the ability to rapidly adapt to fluctuating power needs, the response time and dynamic load management of the engine become critical. Steam engines that can handle variable loads without sacrificing performance are particularly valuable in environments with unstable or intermittent renewable energy sources like wind or solar power.
Emerging technologies in digitalization and automation are also making steam engines more sophisticated. Many modern systems come equipped with advanced sensors, control systems, and data analytics that allow for real-time monitoring of performance. This enables operators to predict when parts are nearing failure, optimize fuel consumption, and adjust operating conditions to achieve the best possible efficiency. These advancements allow steam engines to be integrated seamlessly into smart grids, contributing to a more responsive, efficient, and intelligent energy network.
In addition to operational considerations, future-proofing your steam engine investment is key. As energy policies and regulations evolve, there may be a need for upgrades or modifications to meet stricter emissions standards or to optimize for newer, cleaner fuel sources. Choosing a steam engine that can be easily retrofitted or modified for future upgrades can save significant costs down the line, as opposed to opting for systems that might become obsolete or difficult to adjust as technologies advance.
The economic environment and energy market trends also play a significant role in the decision-making process. As the global push for decarbonization intensifies, energy prices fluctuate, and government policies change, industries may need to stay agile. It’s important to keep an eye on emerging incentives, subsidies, and tax credits for renewable energy solutions or carbon reduction technologies, as these could significantly impact the cost-effectiveness of your investment in steam energy systems. Conversely, shifts in energy markets—such as price volatility in fuel sources like oil and gas—might make it more cost-effective to switch to a hybrid or renewable-powered steam engine solution.
The growing interest in decentralized energy systems also makes steam engines an attractive option for communities and industries that need localized solutions. With the rise of microgrids and distributed energy networks, steam engines can be used to create independent, small-scale power generation systems that reduce reliance on centralized utilities. These systems are particularly valuable in remote areas or regions where the existing grid infrastructure is either non-existent or unreliable. By investing in steam engine technologies for distributed energy, companies can improve their energy independence, reduce exposure to grid outages, and lower transmission losses.
As energy storage technologies like batteries and thermal storage continue to improve, steam engines can be part of a comprehensive energy solution. By combining steam engines with storage systems, you can optimize energy use by storing excess energy during periods of low demand and releasing it during peak times. This type of integration helps smooth out the natural fluctuations of renewable energy sources like wind and solar, ensuring a continuous, stable supply of power even when these sources are intermittent.
Ultimately, the future of steam engines lies in their ability to adapt to new energy demands, technological advancements, and environmental considerations. They will continue to be integral to the transition to sustainable energy, providing efficient, flexible, and reliable power solutions across industries. By selecting the right steam engine for your application, you’re not just meeting today’s energy needs but positioning yourself to thrive in a future that demands cleaner, more efficient, and resilient energy systems. As new innovations and designs emerge, steam engines will remain a crucial part of the diverse energy mix needed to power the world in an environmentally responsible way.
As the landscape of energy production continues to evolve, it’s essential to anticipate not only the technological trends but also the regulatory shifts that will shape the future of steam engines. Governments around the world are increasingly prioritizing carbon reduction, energy efficiency, and the transition to renewable energy. Many nations are setting ambitious carbon neutrality goals, often accompanied by stringent emissions standards for industrial processes and power generation. This means industries will face increasing pressure to adopt cleaner technologies, and steam engines powered by fossil fuels may become less viable unless adapted for low-carbon solutions, such as biofuels or carbon capture technologies.
One of the key benefits of steam engines in the context of industrial decarbonization is their ability to be retrofit with newer technologies. For instance, older fossil-fuel-powered steam engines can be adapted to run on biofuels, synthetic fuels, or hydrogen, helping companies meet emissions regulations while extending the operational life of their assets. This retrofit option is especially important in heavy industries, where switching to completely new technology might be too costly or logistically complex. The ability to modify existing systems for use with alternative fuels offers a flexible pathway for industries to transition gradually to cleaner energy sources.
In addition to fuel adaptability, another major focus of innovation in steam engines is the integration of energy efficiency technologies. Modern steam engines are increasingly designed with features that enhance their efficiency, such as improved heat exchangers, superheated steam cycles, and advanced turbine designs that maximize the amount of energy extracted from the fuel. These innovations are crucial in industries where energy consumption is high, and reducing waste heat is paramount for both cost savings and environmental impact. The next frontier of innovation will likely involve the development of systems that can recover even more heat from exhaust gases or incorporate systems like organic Rankine cycles (ORC), which can use low-temperature heat sources to generate additional power.
Another significant aspect of the future of steam engines is the development of smart technology. As the world increasingly moves toward the Industrial Internet of Things (IIoT) and digitalization, steam engines are becoming more connected, with real-time monitoring and predictive maintenance capabilities. This means that data analytics, coupled with artificial intelligence (AI), can be used to optimize performance, predict failures before they occur, and ensure that systems are always running at peak efficiency. These advancements are particularly important in industries where downtime is costly or operational continuity is critical. By adopting smart steam engine technologies, industries can drastically reduce maintenance costs, improve operational reliability, and increase the overall lifespan of their assets.
In parallel with digital advancements, the concept of energy resilience is becoming increasingly important. As more industries shift toward renewable energy sources, the need for energy systems that can operate independently or in hybrid configurations becomes paramount. Steam engines are well-suited for such applications, especially in the form of microgrids or distributed energy systems. These systems can incorporate various sources of renewable power, including solar, wind, biomass, or even geothermal, and use steam engines as part of the energy generation mix. By doing so, they provide a flexible, localized solution that can respond to energy demand fluctuations and provide backup power during emergencies or when the grid fails.
Steam engines can also play a role in the broader circular economy by supporting waste-to-energy processes. In a circular economy, where the goal is to minimize waste and maximize the reuse of resources, steam engines can help convert industrial waste into usable energy. For example, in municipal waste processing, steam engines can be used in waste incineration plants to generate electricity and heat from non-recyclable waste materials. Similarly, agricultural waste, such as crop residues or animal by-products, can be converted into biomass fuel for steam engines, turning waste materials into a valuable energy source. This not only supports sustainability goals but also helps industries comply with waste disposal regulations and reduces the environmental footprint of operations.
The adoption of green hydrogen as a clean fuel is another exciting possibility for the future of steam engines. As hydrogen production methods become more cost-effective and efficient, steam engines that can run on green hydrogen—produced using renewable energy sources like wind or solar—could become a crucial part of the global transition to a hydrogen economy. Green hydrogen, when combusted in steam boilers or used in fuel cells, produces zero carbon emissions and can be used in industries such as heavy transportation, steel manufacturing, and power generation. As a result, the potential for steam engines to be integrated into hydrogen-based energy systems will likely expand in the coming decades, providing an essential option for carbon-free energy production.
As the industry continues to innovate and adapt to changing energy landscapes, the future of steam engines will be shaped by cross-industry collaborations. For instance, the integration of steam engines with solar thermal or geothermal systems could enhance the overall efficiency and sustainability of these energy sources. By combining the thermal energy from these renewable sources with the power-generating capabilities of steam engines, industries can create hybrid systems that leverage the strengths of both technologies. Moreover, partnerships between technology companies, research institutions, and energy providers will be crucial for accelerating the development and commercialization of advanced steam engine systems for renewable energy applications.
In conclusion, the future of steam engines is bright, particularly in the context of renewable energy and sustainability goals. As the world transitions to cleaner energy systems, steam engines will continue to play a significant role in the energy mix, offering flexibility, efficiency, and adaptability to a wide range of applications. Whether through the integration of advanced technologies, the adaptation to new fuel sources, or their involvement in distributed energy systems, steam engines are well-positioned to be a key player in the global energy transition. By staying ahead of industry trends and embracing innovation, businesses can ensure that their steam engine systems remain relevant, efficient, and ready for the future.
Steam Engine Sales: Buy New or Refurbished Engines
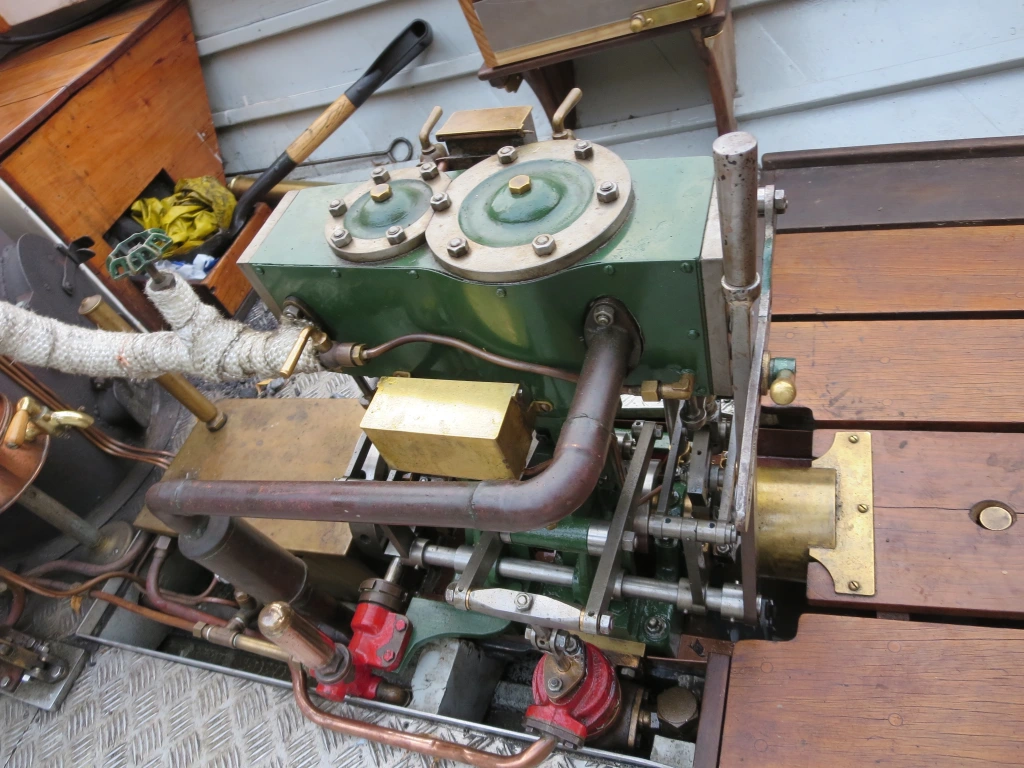
When deciding whether to buy a new or refurbished steam engine, several key factors should be considered, such as the cost, reliability, intended application, and long-term operational goals. Each option offers unique benefits and potential challenges, depending on your specific needs and business priorities. Here’s a detailed look at the decision-making process:
1. Cost Considerations
- New Steam Engines: The initial cost of purchasing a new steam engine is generally higher than a refurbished unit. However, a new engine comes with the benefit of the latest technology, manufacturing standards, and warranty coverage. If you need a steam engine that meets specific performance standards or if your operation requires highly specialized components, a new engine might be worth the investment despite the higher upfront cost.
- Refurbished Steam Engines: Refurbished engines are typically more affordable than new ones, as they are often reconditioned to a like-new condition. The cost savings can be substantial, especially for industries that are operating on a tight budget or need to replace older, worn-out equipment without incurring the high costs of new machinery. However, the savings should be weighed against potential risks related to the engine’s history, such as wear, the extent of refurbishment, and the remaining lifespan.
2. Performance and Efficiency
- New Steam Engines: New steam engines are designed with the latest advancements in materials, design, and energy efficiency. This means they are more likely to perform at higher efficiency levels, have lower emissions, and be equipped with modern control systems. For industries that demand peak performance or have strict regulatory requirements, a new engine offers assurance of reliable, high-performance operation.
- Refurbished Steam Engines: While refurbished engines can be restored to good working condition, they may not be as efficient as a new engine, especially if they are older models or if the refurbishment was not thorough. Efficiency improvements may be limited to the extent of the reconditioning process, and older engines may not have the latest energy-saving technologies, making them less cost-effective in the long run.
3. Warranty and Support
- New Steam Engines: Purchasing a new steam engine typically comes with a full warranty that covers defects, failures, and other issues for a set period. This gives you peace of mind knowing that you have manufacturer support should any problems arise. Additionally, manufacturers may provide installation, training, and regular maintenance as part of the purchase package, ensuring smooth operation from day one.
- Refurbished Steam Engines: Refurbished engines often come with limited or no warranty, depending on the supplier and the extent of refurbishment. While many reputable companies provide warranties for refurbished units, they may be shorter or less comprehensive than those for new engines. Without a robust warranty, the risk of additional costs arising from unforeseen issues is higher, and finding reliable support or parts may be more challenging.
4. Lead Time and Availability
- New Steam Engines: Depending on the manufacturer and customization requirements, new steam engines may take time to manufacture, test, and deliver. If you need a steam engine quickly to replace a malfunctioning system or meet tight project deadlines, the lead time for new units could be a critical factor. However, new engines are built to order, which means you can specify exact performance requirements and features.
- Refurbished Steam Engines: Refurbished engines are often readily available, which can significantly reduce lead time. If your operation is urgent or you need to replace an engine quickly, purchasing a refurbished unit may be the best option. Additionally, refurbished engines may be available for immediate delivery, especially if the supplier already has the exact model or specifications in stock.
5. Customization and Upgrades
- New Steam Engines: With new engines, you have the opportunity to customize the design and specifications to suit your exact needs. Modern steam engines can be configured with cutting-edge control systems, energy-saving features, and advanced materials designed for specific fuels or operating conditions. This is a significant advantage if you have very specific operational requirements or need features that are not available on older models.
- Refurbished Steam Engines: While refurbished engines can sometimes be customized, their options are more limited compared to new systems. Additionally, any upgrades or modifications made during the refurbishment process may not always meet the latest standards or provide the same level of performance as a newly designed engine. If you require specific features or performance levels, it may be harder to achieve this with a refurbished engine.
6. Reliability and Risk
- New Steam Engines: New steam engines come with the assurance of having never been used before, which generally means fewer issues with wear and tear, corrosion, and parts failure. With the manufacturer’s commitment to quality assurance, new engines are designed to last and are tested under controlled conditions to ensure they perform to the highest standards. This significantly reduces the risk of unexpected breakdowns and maintenance costs in the near future.
- Refurbished Steam Engines: While a well-refurbished engine can be reliable, its past history may influence its long-term reliability. Even after extensive reconditioning, there may still be some level of risk associated with older components or potential weaknesses that weren’t addressed during the refurbishment process. The key to minimizing this risk is to choose a reputable supplier with a proven track record for quality refurbishments and a history of delivering reliable equipment.
7. Sustainability and Environmental Impact
- New Steam Engines: New steam engines are typically designed with environmental considerations in mind, adhering to the latest standards for emissions and energy efficiency. For companies looking to align with sustainability goals, new steam engines often provide an opportunity to implement the most energy-efficient systems, reduce their carbon footprint, and contribute to the transition to clean energy.
- Refurbished Steam Engines: Purchasing a refurbished steam engine is inherently more sustainable in some ways, as it helps reduce waste and promotes reusing equipment. Refurbishing older engines extends their life, reducing the need for new manufacturing and the associated environmental impact. However, the environmental benefits may be offset if the refurbished engine is less efficient than a new, more advanced model.
8. Long-Term Maintenance and Operating Costs
- New Steam Engines: While the initial purchase price of a new steam engine may be higher, the long-term operating and maintenance costs may be lower due to the engine’s improved efficiency, reliability, and modern components. These engines typically have longer lifespans and are covered by warranties, reducing the likelihood of expensive repairs in the short term.
- Refurbished Steam Engines: Refurbished engines may have lower initial costs, but they can come with higher long-term maintenance and operating costs, especially if the refurbishment was not comprehensive. Older engines may require more frequent maintenance, replacement parts, and repairs, which can accumulate over time. This should be factored into the total cost of ownership, especially if your steam engine is expected to run for many years.
Conclusion
The decision to buy a new or refurbished steam engine ultimately depends on several factors, including your budget, performance needs, and operational requirements. If cost is a primary concern, and the engine will be used for less-demanding applications, a refurbished engine may offer an affordable and effective solution. However, if performance, efficiency, and long-term reliability are critical, especially in industries with high operational demands or stringent regulatory requirements, investing in a new engine could be the better option.
Before making a decision, it’s important to thoroughly assess the supplier’s reputation, warranty terms, and the extent of refurbishment for used engines. For those looking to balance cost savings with performance, a new engine with state-of-the-art features may offer the best return on investment in the long term, while refurbished engines can provide a cost-effective alternative if carefully selected and backed by solid warranties and support.
When weighing the decision between new and refurbished steam engines, it’s crucial to understand that the choice will also depend on the specific demands of your operation. For instance, if your business operates in a high-demand, high-precision environment, the precision and reliability of a new engine might outweigh the cost savings of a refurbished model. A new steam engine could also give you access to the latest innovations in control systems, materials, and automation, which could significantly improve operational efficiency and make your processes more future-proof.
Another important consideration is the support network associated with each type of purchase. With new engines, the manufacturer typically offers ongoing customer support, which can be invaluable for troubleshooting, maintenance, and performance optimization. This support often extends throughout the warranty period and can provide you with the necessary peace of mind in the early years of operation. In contrast, while many reputable companies offer strong support for refurbished engines, it may not be as extensive or reliable as that for new units. It’s essential to ensure that the supplier of a refurbished engine offers adequate service and parts availability, as well as a clear track record of high-quality refurbishment.
The operational environment is another key factor to consider. If your business operates in a harsh or extreme environment, a new engine built with modern materials and technologies might offer the durability and efficiency required. In industries like chemical processing, metallurgical production, or heavy manufacturing, where uptime is critical and failures can be costly, investing in a new engine that meets the latest safety, efficiency, and emissions standards could be worth the higher upfront cost.
Additionally, businesses that are part of a global supply chain or are highly dependent on their energy infrastructure may be inclined to invest in new engines to ensure they meet international performance standards and can easily be integrated into existing systems. In contrast, industries where operations are more localized and flexibility is key might find refurbished engines to be a more suitable choice, especially if the equipment can be customized and retrofitted for specific needs.
Another point to consider is regulatory compliance. Many regions have stringent environmental and safety regulations, and purchasing a new steam engine ensures that it will meet the latest environmental standards, including emission controls and energy efficiency targets. A new engine will likely be built with these regulations in mind, which is essential for industries that need to adhere to specific compliance standards. Refurbished engines, depending on their age and the scope of their refurbishment, may not always meet the latest standards, which could lead to additional costs in order to bring them up to code or fines for non-compliance.
For companies with a tight operational budget or those who need to replace aging equipment without disrupting production, refurbished engines present a viable option. They can offer reliable performance at a fraction of the cost of new engines, and if the refurbishment process is thorough, they can provide significant longevity and efficiency. However, it’s important to conduct a detailed assessment of the refurbishing process, including inspecting replacement parts, ensuring that key components have been replaced or upgraded, and confirming that the engine meets your operational needs.
The long-term cost of ownership is another crucial factor. New steam engines tend to have lower maintenance and operational costs in their early years, thanks to advanced features and modern manufacturing processes. Over time, however, the higher initial purchase price could still be justified by reduced downtime, fewer repairs, and greater fuel efficiency. On the other hand, refurbished steam engines may come with higher long-term maintenance costs due to the wear and tear of older components, although the initial savings might offset these potential future expenses. It’s essential to project the total cost of ownership over the life of the engine to understand which option will provide the best financial return in your specific context.
A final point to consider is technological upgrades. New engines often come with the ability to easily integrate with modern control systems and smart technologies, which can lead to improved monitoring and predictive maintenance. These features can optimize engine performance, reduce operating costs, and increase overall efficiency. Refurbished engines, depending on the model, may not always have this level of adaptability, or may require more significant modifications to take advantage of modern technological advancements. If your operations are moving toward automation or digitalization, a new engine might be the best fit to integrate seamlessly into these systems.
Ultimately, choosing between a new and refurbished steam engine is a decision that depends on balancing cost, performance requirements, and future needs. If your business requires the highest levels of efficiency, reliability, and long-term support, a new engine is likely the better choice. However, if cost is a primary concern and you have a trusted supplier capable of delivering high-quality refurbished equipment with strong after-sales support, a refurbished engine can be an excellent alternative. Regardless of your choice, it’s important to carefully assess your needs, the potential total cost of ownership, and the risks and benefits of each option to ensure you make the best investment for your business.
When considering whether to buy a new or refurbished steam engine, it’s also important to take into account the future-proofing of your equipment. As industries evolve and energy demands shift, the ability to adapt your steam engine to new technologies or fuel sources will be crucial. New steam engines are typically designed with this adaptability in mind, making them easier to integrate with upcoming advancements such as biofuels, hydrogen, or carbon capture systems. These types of upgrades might be more difficult or costly to implement in older, refurbished models, which may not have been designed to accommodate such future technologies. If your business is looking at long-term sustainability goals or planning to shift towards greener energy sources, a new engine could provide a better foundation for these transitions.
Additionally, if you are working in a growth industry or one that requires frequent scaling of operations, the flexibility and modern features offered by new steam engines can support this expansion more effectively. New systems tend to have higher capacities, improved energy output, and better performance metrics that will allow your operation to increase its production without sacrificing efficiency or requiring frequent overhauls.
On the other hand, refurbished steam engines can still be suitable for operations where rapid scaling isn’t a primary concern or for businesses looking to maintain smaller, more stable operations. If you’re in a sector where you’re not aiming for rapid technological upgrades or where older technologies are still effective, purchasing a refurbished engine could still deliver strong performance and reliability, especially if your requirements are well-matched to the engine’s existing capabilities.
There are also some logistical considerations to keep in mind, particularly around installation and downtime. New engines, while offering the latest technology, can sometimes require more complex installation processes, especially if they involve integration with new systems or modifications to existing infrastructure. This might result in longer downtime during the installation phase, which could disrupt operations in industries where continuous operation is critical. Conversely, a refurbished engine might be quicker to install or more compatible with existing systems, which can minimize downtime and make it easier to maintain business continuity.
In some cases, the availability of spare parts may also influence your decision. For new engines, parts and replacements are generally readily available, and manufacturers often provide clear channels for sourcing these components. Refurbished steam engines, however, may face challenges with parts availability, especially if the model is older or no longer supported by the manufacturer. It’s essential to ensure that the refurbished engine you are considering is supported by a reliable supply of parts, and that these parts are not prohibitively expensive or difficult to acquire. If you are purchasing a steam engine from a reputable seller, they may have already addressed these concerns, but it’s still a factor worth investigating thoroughly.
In terms of emergency repair needs, new engines tend to have the advantage. They typically come with comprehensive service packages and a network of trained technicians who are experienced with their specific models. This ensures that if something goes wrong, help is just a phone call away, and repairs can be carried out efficiently. For refurbished engines, while service might still be available, it may be more difficult to find specialized technicians, and response times may be slower, especially if parts are no longer in production.
Another angle to consider is the life cycle of the steam engine. New steam engines will typically have a longer expected lifespan compared to refurbished ones, particularly when factoring in the wear and tear that older engines might have already endured. While refurbished engines are restored to a like-new condition, they may still have aging components that could reduce their effective lifespan in comparison to a new engine that is starting fresh. For industries with long-term operational needs or those that intend to keep the equipment running for decades, investing in a new steam engine might ultimately be more cost-effective, as it will likely require fewer repairs and offer more years of reliable service.
When factoring in all of these considerations, the ultimate choice between new and refurbished steam engines will depend on a combination of financial goals, technical requirements, and long-term business strategy. For companies that are looking for the latest technology, reliability, and optimal performance, a new steam engine may offer the most value, even with its higher upfront cost. Conversely, if cost-efficiency, short-term needs, or reliability in less demanding environments are more important, a refurbished engine can provide excellent value with a shorter payback period.
Lastly, the environmental impact of both options should also be taken into account. New steam engines often come with higher energy efficiency and lower emissions, especially if they are built to meet modern environmental regulations. Refurbished engines, while potentially offering the benefit of sustainability by reusing old equipment, may not always meet the latest green standards unless significant upgrades are made during the refurbishment process. If your business has set specific carbon reduction goals or needs to comply with environmental regulations, investing in a new steam engine may be the better choice, particularly if it includes energy-efficient features such as advanced heat recovery systems, better combustion technology, or reduced emissions.
In conclusion, the decision between new and refurbished steam engines is not one-size-fits-all. It involves a thorough evaluation of your operational needs, financial situation, and long-term goals. Regardless of which option you choose, the key to making the best decision is understanding the total cost of ownership, the expected maintenance requirements, and the specific needs of your operation. By weighing these factors carefully and consulting with reputable suppliers, you can ensure that your steam engine investment supports the long-term success and sustainability of your business.
Expert Steam Engine Installation Services
Expert steam engine installation services are essential for ensuring that your system operates safely, efficiently, and reliably from the very beginning. Whether you’re setting up a new steam engine or replacing an older one, professional installation goes far beyond simply positioning the unit and connecting the pipes. It involves a detailed understanding of the engine’s mechanical, thermal, and control systems, along with precise calibration, alignment, and safety verification.
The process typically begins with a site assessment, where installation specialists evaluate the physical layout, foundation requirements, structural support, and environmental factors like ventilation, drainage, and proximity to power and steam lines. This early step helps to avoid future issues related to vibration, heat buildup, and misalignment. Based on this assessment, a customized installation plan is created to match the unique demands of your operation—whether it’s industrial, marine, power generation, or heritage-based.
During installation, experienced technicians handle tasks such as foundation anchoring, shaft and coupling alignment, boiler connection, valve setup, and integration with auxiliary systems like condensers, pumps, and control panels. They ensure that all components are not only installed correctly but also optimized for peak efficiency and long-term reliability. Proper alignment and torqueing of components are especially crucial to minimize wear and prevent future mechanical issues.
A critical part of expert installation is the commissioning process, which involves rigorous testing of the system under load conditions. This includes verifying pressure levels, temperature control, steam flow regulation, lubrication systems, and emergency shutdown procedures. Technicians monitor performance metrics and make adjustments to ensure that the steam engine performs within specified tolerances, and that it integrates seamlessly with the rest of the facility’s operations.
Safety compliance is another vital aspect. Professional installers ensure that all relevant codes, standards, and regulations are met—from ASME and ISO standards to local environmental and safety laws. This includes proper venting, pressure relief, insulation, and emergency stop systems, as well as documentation and operator training.
Furthermore, expert installation services often include post-installation support, such as maintenance planning, operational training, and access to technical resources. Many providers offer long-term service agreements, ensuring that any future issues are handled quickly by technicians who already understand your system’s design and layout.
Ultimately, investing in expert steam engine installation pays dividends in performance, longevity, and safety. A correctly installed engine minimizes downtime, maximizes energy efficiency, and protects your investment—whether it’s a new high-efficiency unit or a heritage restoration project. With the right team, you gain not just installation expertise, but a partnership that supports your steam power system over its entire life cycle.
Expert installation services also offer the advantage of minimizing costly delays and mistakes that can occur during complex mechanical setups. Because steam engines operate under high pressure and temperature, even minor errors in installation—such as improper pipe routing, misaligned shafts, or incorrect valve settings—can lead to serious performance issues or safety hazards. Professionals bring not only technical know-how but also the experience of having worked with a variety of steam engine types and site conditions, allowing them to anticipate and solve problems before they escalate.
Another critical benefit of professional installation is the ability to streamline integration with existing infrastructure. Many facilities operate with legacy systems or hybrid setups that require careful coordination between new and old components. An expert team will have the tools and understanding necessary to ensure seamless interfacing between the steam engine and auxiliary systems such as boilers, turbines, electrical generators, or factory automation platforms. This includes configuring sensors and control interfaces to enable real-time monitoring and diagnostics, which are essential for predictive maintenance and energy optimization.
In industries where downtime equals lost revenue, time is of the essence. Experienced installation teams understand this urgency and typically follow a well-coordinated schedule, often with modular installation techniques that reduce on-site work time. They also conduct pre-installation testing off-site when possible, ensuring that systems are verified before they arrive at your facility. This efficiency not only speeds up commissioning but reduces the risk of unplanned shutdowns during early operation.
An often-overlooked aspect of expert installation is training and documentation. Once the steam engine is in place and running, operators need to understand not just how to use it, but how to care for it. Professional installers provide detailed manuals, schematics, and logs, along with hands-on training sessions that cover everything from daily checks to emergency procedures. This knowledge transfer empowers your team to operate the system confidently and reduces your reliance on external support for routine matters.
There’s also the added advantage of warranty protection. Most manufacturers and refurbishers require professional installation as a condition for honoring equipment warranties. Attempting a DIY installation or using underqualified contractors can not only void these warranties but also leave your facility vulnerable to unexpected repair costs. By using certified installers, you protect your investment and gain direct access to technical support in case issues arise during the warranty period.
Furthermore, expert installation services often come with post-installation performance tuning. Once the engine has been operating under load for a set period, technicians may return to assess performance metrics and make fine adjustments to steam pressure, valve timing, or governor settings to enhance efficiency and reduce wear. This continuous improvement approach helps the engine settle into its operating environment and delivers long-term reliability and output consistency.
For industries operating in regulated environments—such as food processing, pharmaceuticals, or power generation—professional installation services also play a critical role in compliance. These teams ensure that all equipment is installed in accordance with local and national codes, perform necessary inspections, and help prepare documentation for audits or certifications. They may also conduct risk assessments and hazard analyses to ensure that the system is safe and compliant from the outset.
Ultimately, having experts handle your steam engine installation gives you a head start toward achieving operational excellence. The process isn’t just about bolting machinery into place—it’s about optimizing an entire energy system to function harmoniously with the rest of your operation. From mechanical alignment to digital integration, from safety compliance to hands-on training, the value of expertise becomes apparent not only in smooth day-one performance but in years of trouble-free operation that follow. The peace of mind you gain from knowing your system is in the hands of professionals is an intangible but powerful benefit, allowing you to focus on your business while your steam engine runs with precision, power, and purpose.
Beyond the technical and operational advantages, expert steam engine installation services also provide a significant strategic edge. Companies that rely on steam power as a core component of their operations—whether for electricity generation, industrial heating, propulsion, or process steam—must treat the installation phase as the cornerstone of long-term system health. A well-installed engine not only performs better out of the gate but sets the foundation for maximum lifecycle value. Professional installers understand this and work to ensure that every bolt, sensor, and control connection contributes to long-term reliability and cost-efficiency.
One often understated benefit is how expert installation services contribute to energy efficiency. Steam engines, by design, convert thermal energy into mechanical work, and any inefficiency in the setup—such as poor insulation, heat loss, or suboptimal steam routing—can significantly affect performance and energy costs. Installation professionals apply best practices like proper lagging of pipes, minimizing pressure drops, and optimizing condensate return systems. These details, though seemingly minor at the moment of installation, have a substantial cumulative impact on fuel consumption, emissions, and operational costs over time.
In many industrial settings, steam engines operate alongside complex systems such as CHP (combined heat and power) units, thermal storage, or distributed generation assets. Installing a steam engine within such an ecosystem demands not just mechanical skills but an integrated energy systems mindset. This means thinking in terms of total plant efficiency, ensuring that the steam engine installation complements the heat recovery, waste management, water treatment, and electrical grid infrastructure already in place. Professional installers are trained to see the broader picture and to work collaboratively with your engineering or plant management teams to create a fully optimized energy environment.
For custom or high-performance applications, such as in research facilities, luxury yachts, or heritage railway restorations, the level of precision and craftsmanship involved in installation becomes even more critical. Here, the aesthetics, acoustics, and historical authenticity of the steam engine may be just as important as its functional performance. Expert installers often bring artisanal skill and deep historical knowledge to these projects, ensuring the final result honors both form and function. This kind of bespoke installation can also involve custom machining, retrofitting of components, or integration with legacy controls—areas where only highly experienced professionals can truly deliver.
Moreover, as digital technologies continue to transform industry, expert installation increasingly includes the deployment of smart systems. These might involve sensors that monitor temperature, vibration, pressure, or performance trends, all feeding data into centralized dashboards for real-time analysis. Installing these technologies correctly from the outset ensures your system is future-ready, capable of integrating with IoT platforms, predictive maintenance software, and even AI-based performance optimization tools. Rather than retrofitting these features later, expert installers embed them into the system during commissioning, giving your operation a technological head start.
Long-term cost savings are another compelling reason to invest in top-tier installation services. The initial outlay for expert help may seem high compared to in-house or generic contractors, but the return on investment is almost always realized through reduced downtime, lower maintenance needs, fewer safety incidents, and higher operational efficiency. In essence, the cost of doing it right once is nearly always less than the cost of fixing it later—especially when dealing with powerful, high-pressure systems like steam engines.
Finally, there’s the intangible but valuable benefit of confidence. When your steam engine is installed by seasoned professionals, you can be certain that it has been calibrated, aligned, and tested to exacting standards. That confidence translates into peace of mind for your operators, trust for your stakeholders, and stability for your production schedules. It means fewer surprises, smoother audits, and a system that performs as it was designed to—day in, day out.
Whether you are installing a modern back-pressure turbine, a heritage piston engine, or a high-efficiency condensing unit, the quality of the installation will directly influence every operational and financial outcome that follows. Expert steam engine installation isn’t just a service—it’s a strategic investment in the durability, performance, and future-readiness of your entire operation.
Custom Steam Engine Manufacturing and Design
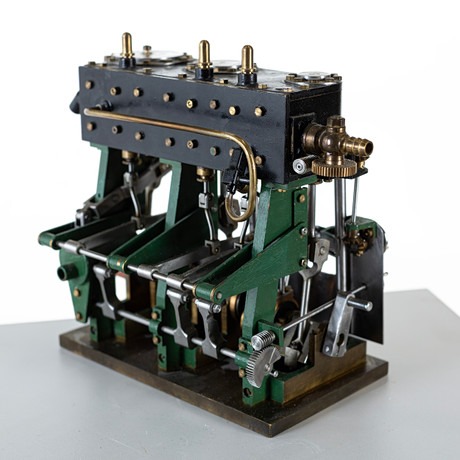
Custom steam engine manufacturing and design is a highly specialized field that combines mechanical engineering expertise with practical knowledge of thermodynamics, materials science, and often, legacy craftsmanship. Unlike off-the-shelf engines, custom steam engines are tailored to meet the exact requirements of a specific application—whether that’s powering a micro-scale generator, driving industrial equipment, supporting research in thermal systems, or restoring a historic locomotive or ship.
The process begins with a thorough consultation and needs analysis. Designers work closely with clients to understand the engine’s intended use, expected power output, operational environment, fuel source, and any spatial or technical constraints. Whether it’s a compact steam engine for educational demonstration or a large-scale unit for industrial power, every design starts with detailed calculations to determine optimal cylinder size, stroke length, boiler capacity, steam pressure, and mechanical efficiency.
Once the requirements are clear, engineers move into the design phase using CAD and thermal simulation tools to model the engine’s components and predict performance. Materials are selected not only for durability and thermal conductivity but also for corrosion resistance, pressure tolerance, and machining precision. In some cases, the design may incorporate modern enhancements like electronic governors, automated lubrication systems, or digital control integration, allowing the steam engine to operate more efficiently and safely in a modern industrial environment.
The manufacturing stage is where engineering vision becomes physical reality. Skilled machinists and fabricators craft each component—cylinders, pistons, crankshafts, valve gear, flywheels, and linkages—often using a combination of CNC precision machining and hand-finishing for critical fits. For historical reproductions or artistic projects, foundry work may be included, producing cast-iron or bronze components from custom molds. Boilers, the heart of any steam engine, are typically custom-fabricated and certified to meet pressure vessel codes, with options for vertical, horizontal, fire-tube, or water-tube configurations depending on the application.
Assembly requires exacting care, as even minor alignment or clearance issues can lead to performance losses or mechanical failure. Once assembled, the engine undergoes a comprehensive testing process, including cold fits, dry runs, hydrostatic pressure testing, and live steam trials. Engineers fine-tune timing, valve operation, and load behavior to ensure the engine performs as expected under real conditions.
Custom design also allows for unique features tailored to user priorities. In energy recovery applications, for instance, back-pressure or condensing turbines can be integrated to reclaim heat and improve total system efficiency. In marine or mobile applications, compact and lightweight construction may take precedence, using high-strength alloys and modular layout. For educational or demonstrative units, transparent cylinders or slow-speed gearing can be used to make the mechanics easily observable.
In all cases, the advantage of a custom steam engine lies in its alignment with the user’s exact goals—delivering not just raw power, but optimized performance, efficiency, and longevity. With proper design and craftsmanship, a custom-built steam engine becomes a robust, elegant, and dependable machine tailored to its task, capable of running smoothly for decades with proper care. Whether driven by passion, necessity, or innovation, custom steam engine design bridges the old and the new, keeping this timeless technology relevant and powerful in today’s world.
The beauty of custom steam engine manufacturing lies in the freedom it offers to adapt the technology to a wide array of applications and challenges. This adaptability is particularly important in industries where off-the-shelf solutions might not meet the unique operational needs, space constraints, or environmental conditions. Whether the engine needs to operate in a high-humidity environment, withstand extreme temperatures, or integrate with advanced digital controls, custom designs can be engineered to handle these specific demands.
For example, in industries where steam engines are used for combined heat and power (CHP) systems, a custom engine can be designed to not only generate mechanical power but also supply heat efficiently to adjacent processes, factories, or buildings. By optimizing the engine’s thermodynamic cycles and integrating heat recovery systems, the steam engine can achieve a higher level of energy efficiency than standard models. Such applications often require close collaboration between the manufacturer, engineers, and the end-user to fine-tune the system for the best balance of power and heat output, as well as integration with existing plant operations.
In research and development settings, custom steam engines may be built to allow detailed study of steam dynamics, fluid mechanics, and thermodynamics. Here, design flexibility can enable specialized features like variable-speed governors, transparent components, or instrumentation that allows for real-time monitoring of internal pressures and temperatures. These custom-built engines become essential tools for academic institutions or R&D departments working on advanced energy technologies or optimizing steam-based systems.
The aesthetic appeal of custom steam engines is another important aspect, especially in industries like heritage restoration or luxury applications. In such cases, the engine may be designed not only to operate efficiently but to retain or replicate the classic look and feel of historic steam technology. Restoration projects, such as those involving historic railroads, marine vessels, or steam-powered vehicles, require designers who understand the subtleties of vintage engineering. This includes crafting visually accurate components, using period-correct materials, and ensuring that the engine is capable of meeting modern performance and safety standards without compromising its historical integrity.
In terms of performance, custom steam engines can be tuned for specific fuel sources. Whether powered by coal, natural gas, biomass, or even renewable fuels like hydrogen or biogas, custom designs allow the combustion and steam-generation processes to be fine-tuned for efficiency, cost-effectiveness, and environmental impact. These custom solutions can help industries transition to more sustainable energy sources, reducing reliance on fossil fuels while maintaining the reliability and performance of traditional steam technology.
Space efficiency is also a key consideration for many custom projects. In confined spaces, such as those found in ships, offshore platforms, or remote industrial sites, the engine must be designed with careful consideration for compactness and modularity. Custom steam engines can be built with modular components that are easier to transport, assemble, and maintain in tight spaces, reducing the need for extensive infrastructure or costly modifications to the installation site.
Moreover, custom steam engines offer the potential for advanced control systems. Modern steam engines can integrate with smart technologies, allowing for precise monitoring and adjustments via digital interfaces. For industrial applications, this means connecting the steam engine to supervisory control and data acquisition (SCADA) systems, which enable operators to monitor and adjust parameters such as steam pressure, flow rate, and temperature remotely. These systems can also track performance over time, identifying trends and enabling predictive maintenance, reducing downtime and improving overall efficiency.
The custom manufacturing process doesn’t just stop at the engine itself but often extends to related equipment. For example, custom-designed boilers, turbines, and valve systems can be part of a larger bespoke steam power system, each component designed to fit together seamlessly. The result is an integrated system that performs at its highest potential, with each element optimized to contribute to the overall function of the engine.
Furthermore, the maintenance aspect of custom steam engines is often planned from the beginning. With specific design features tailored to ease servicing and component replacements, custom engines can be built for longevity and reliability. Features like easily accessible valve assemblies, inspection ports, or modular parts make it easier for maintenance teams to perform checks, replace worn components, and keep the engine in peak condition throughout its life cycle.
At the core of custom steam engine design is the ability to provide a solution that meets unique operational requirements. This flexibility is what sets custom-built engines apart from standard models, offering unparalleled value in situations where performance, efficiency, or visual design is critical. Whether for industrial use, energy generation, transportation, or historic restoration, custom steam engines can be crafted to deliver both functional excellence and lasting durability, ensuring that these powerful machines continue to play an important role in modern industries and historical preservation.
In addition to the core benefits of custom steam engine design and manufacturing, there’s also a significant focus on safety and compliance. Custom steam engines, especially those used in high-risk or regulated industries, are built to meet stringent safety standards and industry regulations. Engineers take extra care to integrate safety features such as pressure relief valves, temperature sensors, emergency shut-off mechanisms, and automatic shutdown systems to prevent accidents. These safety systems are tailored to each specific application, ensuring that the engine will perform safely under varying operating conditions, whether in a high-pressure industrial environment or a more controlled research setup.
The customization process also allows for the inclusion of advanced materials and innovative technologies that can extend the life of the engine while reducing operational costs. For example, engineers may choose to use ceramic coatings for high-wear components to improve their resistance to thermal cycling and corrosion. Advanced sealing systems can be designed to improve the engine’s overall efficiency by preventing steam leaks, which are critical for maintaining optimal performance and minimizing fuel consumption. Moreover, high-strength alloys and composite materials may be utilized for components that are subject to extreme mechanical stress or wear, ensuring the engine can operate effectively for many years with minimal downtime.
Another important aspect of custom steam engine manufacturing is the consideration of energy recovery systems. For many industrial applications, the steam engine doesn’t just provide mechanical power—it can also play a significant role in heat recovery. Integrating custom steam engines with systems such as heat exchangers and regenerative turbines allows industries to capture and reuse waste heat, significantly boosting energy efficiency. This process not only reduces fuel consumption but also minimizes environmental impact by lowering emissions. Custom design enables these energy recovery features to be seamlessly integrated with the steam engine, ensuring optimal performance across all operating conditions.
For applications that require transportable steam engines, such as in maritime or remote-area industries, manufacturers often design engines that are lightweight and portable, while still delivering the necessary power output. Modular systems allow components to be easily transported, set up, and reassembled on-site. This flexibility is particularly valuable in industries like offshore oil and gas extraction or in temporary installations where a mobile, yet powerful steam system is required. Such designs are built with easy transportability in mind, considering factors such as engine size, weight distribution, and ease of assembly to ensure smooth deployment in diverse environments.
Furthermore, the longevity and reliability of custom steam engines are a major selling point. Unlike mass-produced engines, which may have a shorter operational life or require frequent maintenance, custom-built engines are designed with a longer-term view. The components are selected to withstand the rigors of heavy use, and the engines are designed for easy servicing, reducing the likelihood of expensive and time-consuming breakdowns. Manufacturers often provide detailed maintenance schedules, offering customers guidance on how to optimize the performance and lifespan of their custom engine.
The integration of digital technologies into custom steam engine systems is also a growing trend. Smart steam engines can now be equipped with embedded sensors that provide real-time feedback on operational performance. These sensors can monitor variables like pressure, temperature, steam quality, and mechanical stress, feeding this data into centralized monitoring systems for analysis. Using these insights, operators can adjust parameters for optimal performance or predict when maintenance is needed, helping to avoid unplanned downtime and reducing long-term operating costs. Some modern steam engines even come with IoT (Internet of Things) capabilities, allowing for remote monitoring and control from anywhere in the world.
The versatility of custom steam engine design also extends to the retrofit and upgrade of existing systems. For industries that rely on older steam engines but need to improve performance, efficiency, or integrate new technologies, custom design and manufacturing services offer the opportunity to upgrade or replace individual components with modern, higher-performance parts. This can include retrofitting with newer, more efficient boilers, improved condensate systems, or integrating the engine with modern digital control systems. These upgrades not only extend the life of the engine but also improve energy efficiency and reduce emissions, making older systems more environmentally friendly and cost-effective.
Another advantage of custom steam engines is their role in sustainability efforts. Many industries today are focused on reducing their carbon footprints and increasing their sustainability. Custom-designed steam engines can be built with this focus in mind, incorporating energy-efficient features and using environmentally friendly materials wherever possible. Additionally, biomass and renewable energy sources are increasingly being integrated into steam systems, allowing industries to reduce their reliance on fossil fuels while maintaining reliable power generation.
Finally, post-manufacturing support is a vital part of custom steam engine services. Manufacturers often offer comprehensive post-installation support, including training, maintenance services, and troubleshooting assistance. This ensures that customers can maximize the performance of their engines and minimize any operational interruptions. Custom engine manufacturers may also provide spare parts tailored specifically for the designed systems, ensuring that replacements are readily available if needed, with minimal downtime for repairs.
In conclusion, the custom design and manufacturing of steam engines offer a multitude of benefits, including tailored performance, extended lifespan, energy efficiency, and integration with modern technologies. From power generation to research applications, marine propulsion, and industrial processes, custom-built steam engines are indispensable in industries that require high-performance, reliability, and sustainability. The combination of engineering expertise, advanced materials, and cutting-edge technologies ensures that these engines not only meet the specific needs of their applications but exceed them, offering long-term value and versatility across a wide range of industries.
Steam Engine Efficiency and Performance Optimization
Steam engine efficiency and performance optimization are critical elements in ensuring that these systems operate at their peak potential, both in terms of energy usage and mechanical output. As steam engines rely on converting thermal energy into mechanical power, the more efficiently this conversion happens, the less fuel is required, and the better the overall system performance becomes. Whether used for power generation, industrial processes, or transportation, improving steam engine efficiency directly correlates with reduced operational costs, fewer emissions, and extended engine lifespan.
Key Factors Influencing Steam Engine Efficiency
1. Thermodynamic Cycle Optimization:
At the heart of every steam engine is the thermodynamic cycle, which defines how efficiently heat is converted into mechanical energy. The Rankine cycle, commonly used in steam engines, involves heating water to create steam, using that steam to drive a turbine or piston, and then condensing the steam back into water to repeat the cycle. Optimizing each phase of the cycle can lead to significant improvements in efficiency.
For example, higher steam pressures and temperatures improve the efficiency of the Rankine cycle because they allow the engine to extract more energy from the same amount of fuel. Increasing the pressure of the steam and expanding the steam at a lower pressure in the turbine can boost mechanical output without needing more fuel. However, this comes with the need for stronger materials and a more robust design to handle the increased stress and temperature.
2. Boiler Design and Efficiency:
The boiler plays a pivotal role in a steam engine’s performance. Boilers are responsible for generating steam, and their design has a direct impact on the fuel consumption, steam pressure, and overall efficiency. A more efficient boiler minimizes heat loss during the combustion process and optimizes the steam generation process. Modern boilers often use economizers to recover waste heat from exhaust gases, improving efficiency by preheating incoming feedwater. Additionally, fluidized bed combustion and modulating burners are technologies used in advanced boilers to enhance combustion efficiency and reduce fuel consumption.
3. Heat Recovery and Condensation Systems:
A crucial part of optimizing steam engine performance is the recovery of heat from the exhaust steam and the efficient management of condensation. In traditional steam engines, steam is often allowed to condense, which results in a loss of potential energy. By installing condensers and implementing a closed-loop system, the condensation process can be managed more efficiently, recovering heat and improving the system’s overall thermal efficiency.
For example, in condensing steam engines, the exhaust steam is cooled and condensed back into water at a low pressure, which enhances the energy extracted from the steam and reduces the energy needed for reheating. The recovered heat can be utilized for preheating the feedwater, which lowers the fuel required to reach the desired steam pressure.
4. Turbine or Piston Efficiency:
In steam engines, whether the power is harnessed via a steam turbine or piston system, the efficiency of these components is crucial. Turbines, especially in power generation systems, benefit from precise blade design that reduces energy losses due to friction and steam turbulence. Optimizing the clearances and turbine stages allows for better steam flow and more effective energy extraction.
In piston engines, valve timing and piston design play a key role in maximizing the conversion of steam energy into mechanical work. Variable valve timing (VVT), a concept borrowed from modern internal combustion engines, can be used in steam engines to adjust the timing of steam intake and exhaust based on engine load, optimizing performance across different operating conditions.
5. Lubrication and Maintenance:
Proper lubrication is essential for maintaining the efficiency and longevity of steam engines. Friction between moving parts, such as pistons, valves, and shafts, leads to energy losses and excessive wear. Implementing automated lubrication systems and regular maintenance schedules ensures that friction is minimized, allowing for smoother operation and less energy waste. Routine maintenance, including cleaning boilers and checking for steam leaks, ensures the system runs at peak performance.
6. Advanced Control Systems and Automation:
The integration of smart technologies and automated control systems can further enhance steam engine efficiency. Modern steam engines often feature sensors that monitor parameters such as temperature, pressure, and steam flow. These sensors feed data into a central control system, which can make real-time adjustments to optimize performance. For example, by adjusting the fuel-to-steam ratio or valve settings automatically, the system can maintain peak efficiency under varying loads and environmental conditions.
In larger systems, like combined heat and power (CHP) installations, advanced control systems can adjust both the electricity generation and heat recovery processes based on real-time energy demands, optimizing overall system efficiency and reducing waste.
7. Fuel Type and Combustion Optimization:
The type of fuel used in the steam engine has a direct impact on both its thermal efficiency and environmental footprint. Traditional coal or oil-based steam engines can be optimized by enhancing the combustion process—for instance, using flue gas recirculation or oxygen-enriched combustion. These methods improve the efficiency of the combustion process by increasing the flame temperature and reducing the formation of nitrogen oxides (NOx), which are harmful pollutants.
However, many modern steam systems are increasingly transitioning to biomass and renewable energy sources. Custom-designed boilers can be built to accommodate these new fuels, allowing for a more sustainable energy source while maintaining high performance.
8. Waste Heat Recovery and Integration with Other Systems:
In industrial settings, steam engines can be part of a larger energy ecosystem. By integrating the steam engine with other systems, such as organic Rankine cycle (ORC) systems, heat exchangers, or even thermal energy storage systems, waste heat can be effectively captured and reused. This reduces fuel consumption by ensuring that all available energy is utilized, improving the overall system efficiency.
9. Optimization for Load and Operating Conditions:
Steam engines must be designed and optimized for a wide range of operational conditions, including variable loads and start-stop cycles. Optimizing the system for the specific operational requirements can significantly improve performance. For example, load tracking capabilities allow the steam engine to adjust its output based on real-time demand, preventing overproduction and reducing unnecessary fuel consumption.
10. Performance Monitoring and Continuous Improvement:
To keep the steam engine performing optimally over its entire operational life, continuous monitoring and performance evaluation are essential. With the help of modern IoT-enabled sensors and data analytics, steam engine operators can track performance trends and identify any areas where efficiency could be improved. Predictive maintenance tools can forecast when parts are likely to fail, allowing for proactive repairs and upgrades that prevent downtime and keep the engine running efficiently.
Conclusion
Optimizing the efficiency and performance of steam engines is a complex, multifaceted task that involves improving each element of the steam generation and power conversion process. By refining boiler design, enhancing heat recovery, optimizing the thermodynamic cycle, incorporating advanced materials, and utilizing modern control systems, significant improvements in efficiency can be achieved. Whether the goal is to reduce fuel consumption, minimize emissions, or extend the operational lifespan of the engine, careful attention to detail at every stage of the design, operation, and maintenance phases ensures that steam engines continue to provide reliable, cost-effective power in both industrial and modern applications. With the continued integration of advanced technologies and sustainable practices, steam engines will remain a valuable part of the global energy landscape for years to come.
Optimizing steam engine efficiency goes beyond just the individual components and their respective roles. It’s also about the integration of advanced engineering principles and modern technology to enhance performance across the entire system. Each adjustment made at the component level, whether it’s in the boiler, turbine, or control system, has a ripple effect on the overall efficiency, leading to reduced fuel consumption, minimized waste, and lower operational costs.
The utilization of alternative energy sources is also a growing factor in improving steam engine performance. As the world moves towards more sustainable energy solutions, incorporating biomass, solar thermal, and geothermal energy sources into steam engine systems becomes an attractive option. These renewable energy sources can be used to produce steam in a more environmentally friendly manner, cutting down on greenhouse gas emissions and reducing dependence on fossil fuels. Custom-built systems are designed to adapt to these energy inputs, allowing for a more flexible, green approach to steam engine operations.
For instance, integrating biomass boilers into steam engines is a common practice in industries focused on sustainability. Biomass, being a renewable source, can significantly reduce the carbon footprint of a steam-powered system while providing an efficient means of generating heat. Similarly, solar thermal collectors can preheat the water fed into the boiler, improving the overall thermal efficiency and reducing the amount of conventional fuel required to reach the desired steam pressure.
Operational flexibility is another key factor in performance optimization. Steam engines used in applications that experience varying load demands, such as in power generation or heavy industry, benefit from systems that can adjust their output dynamically. Advanced variable-speed control systems ensure that the engine operates efficiently at different loads, minimizing fuel consumption during periods of low demand and ramping up efficiency when full power is required. The ability to modulate steam output based on real-time requirements also means the engine can operate at optimal levels without unnecessary strain or wasted energy.
One of the most significant benefits of continuous optimization in steam engine performance comes from the ability to extend the lifecycle of the equipment. Regular maintenance, along with the integration of predictive maintenance tools, helps detect and address issues before they lead to system failures. Technologies like machine learning algorithms can predict when certain parts—such as valves or gaskets—are likely to wear out based on operational data. This allows for more precise scheduling of maintenance, preventing unscheduled downtime and reducing the need for costly emergency repairs.
Another important aspect of enhancing performance is the reduction of heat losses at every stage of the process. While modern steam systems are designed to recover as much heat as possible, even small improvements can have a noticeable impact. For instance, insulation on pipes and components can prevent heat from escaping, ensuring that more of the energy from the steam is converted into useful mechanical work rather than lost as heat. This is particularly important in systems that operate continuously, where even slight reductions in energy waste can lead to substantial savings over time.
The design of the exhaust system is another area where performance improvements can be made. By ensuring that the exhaust steam is fully condensed and all residual heat is captured, steam engines can operate more efficiently. In systems where steam is used for power generation, the integration of combined heat and power (CHP) systems means that waste heat is repurposed for heating or industrial processes, further boosting overall efficiency and minimizing waste. This synergy not only improves energy efficiency but also adds value by providing heating or process steam without requiring additional fuel input.
With the advent of smart technologies and data analytics, the monitoring and optimization of steam engines have become more advanced. Real-time data collection from sensor networks integrated into the steam engine components allows for constant monitoring of steam pressure, temperature, and flow rates. This data is then analyzed to identify trends, inefficiencies, and potential areas for improvement. Machine learning algorithms can sift through vast amounts of data to predict the optimal operational parameters based on specific conditions, adjusting the engine’s operations accordingly. For instance, in a system where steam pressure fluctuates due to varying load conditions, an intelligent system can dynamically adjust the flow rates and pressure to maintain the most efficient operational point.
The role of digital twin technology in steam engine optimization is also worth mentioning. By creating a virtual replica of the steam engine, engineers can simulate different operating conditions and assess the impact of potential adjustments or upgrades. These digital simulations enable engineers to predict how modifications—such as changes to boiler pressure, steam temperature, or turbine blade design—will affect overall efficiency, performance, and fuel consumption before any physical changes are made. This predictive capability significantly reduces trial-and-error approaches, making the optimization process more precise and cost-effective.
Additionally, advancements in materials science have led to the development of more durable and efficient components for steam engines. For instance, high-temperature alloys and advanced ceramics can now withstand the extreme conditions inside a steam engine, increasing the lifespan of key parts like pistons, valves, and turbine blades. These materials not only extend the service life of the engine but also improve efficiency by reducing wear and tear, which can cause energy losses and decreased performance over time. The use of carbon composites in certain parts also contributes to reducing the weight of the engine while maintaining strength, allowing for more efficient operation, especially in mobile applications.
The synergy between efficiency and environmental sustainability has become a driving force in the development of modern steam engines. As industries strive to meet stricter environmental regulations and reduce their carbon footprint, steam engine optimization plays a key role in achieving these goals. By focusing on improving fuel efficiency, reducing emissions, and utilizing renewable energy sources, steam engines can continue to be a viable option for power generation, industrial processes, and transportation.
In conclusion, optimizing steam engine efficiency and performance involves a multi-faceted approach that takes into account not only the mechanical and thermal processes but also the integration of modern technologies, advanced materials, and data-driven insights. Whether through improving the thermodynamic cycle, utilizing renewable energy sources, or adopting advanced monitoring and control systems, the goal is always to maximize energy conversion, minimize waste, and ensure that steam engines remain a reliable, sustainable, and cost-effective option for a wide range of applications. The continuous advancements in design, materials, and digital technologies ensure that steam engines can evolve and adapt to meet the demands of modern industries, all while offering better efficiency and performance than ever before.
Further advancements in steam engine efficiency and performance can be achieved by focusing on the continual refinement of combustion processes. In traditional steam engines, combustion efficiency is crucial for generating the necessary heat to produce steam. However, modern innovations such as pre-combustion air staging, flame stability systems, and low NOx burners can be incorporated into custom steam engine designs to ensure a cleaner, more efficient combustion process. By optimizing the air-to-fuel ratio and improving the combustion chamber design, it’s possible to burn fuel more completely, reducing excess fuel consumption and limiting harmful emissions.
Additionally, the integration of combined cycle systems can further improve steam engine efficiency. In a combined cycle system, the steam engine can be paired with a gas turbine or other power generation technologies. The heat from the gas turbine’s exhaust gases is fed into the steam engine’s boiler, allowing it to generate additional power from the waste heat. This type of system can result in efficiency improvements of up to 50% over standalone steam power systems, as it utilizes waste heat that would otherwise be discarded. These combined systems are especially beneficial for large-scale power generation and are commonly used in combined heat and power (CHP) facilities.
Operational optimization also plays a role in maximizing steam engine performance. In industries where steam engines are used for batch processes or intermittent power generation, it’s important to adapt the engine’s operation to the unique requirements of the process. For example, the steam engine may be optimized for quick startup times and variable output to match fluctuating production needs. By monitoring demand and adjusting the steam production and mechanical output accordingly, steam engines can operate more efficiently in environments where consistent load demands are not guaranteed.
Furthermore, steam engine performance can be enhanced by minimizing steam leakage throughout the system. Steam leakage often occurs at pipe joints, valves, or worn seals, leading to a significant loss of heat and pressure, which in turn reduces overall efficiency. Regular maintenance and timely replacement of sealing components can prevent leaks, ensuring that the system operates at peak thermal efficiency. In some cases, the integration of zero-leakage technologies and automated sealing systems can drastically reduce this loss, maintaining the system’s integrity and boosting its performance.
Innovative heat transfer technologies are also critical for improving steam engine efficiency. For example, the use of advanced heat exchangers can increase the rate of heat transfer between the combustion gases and the feedwater, resulting in faster steam generation and lower fuel requirements. Plate heat exchangers and double pipe systems are among the modern solutions being adopted in steam engine systems to optimize heat transfer. These technologies ensure that more energy is transferred to the water or steam, increasing system efficiency.
The development of advanced turbine blade coatings has made it possible to enhance steam engine performance under high temperatures. Thermal barrier coatings and ceramic coatings can significantly improve the durability of turbine blades, preventing them from degrading or losing efficiency due to extreme thermal stresses. These coatings also enhance the turbine’s ability to withstand high temperatures, allowing it to operate at higher steam pressures and temperatures, which ultimately boosts overall system efficiency.
Another area of focus is the integration of steam engines with energy storage systems. In systems where steam engines are used intermittently or in combination with renewable energy sources like solar or wind, the integration of thermal energy storage (TES) can provide a buffer to ensure consistent and reliable steam generation. By storing excess heat during periods of low demand and utilizing it when demand spikes or renewable generation is low, TES systems can balance load and reduce the reliance on supplementary fossil fuels.
One of the more exciting trends in steam engine optimization is the potential for additive manufacturing (3D printing) to play a role in creating more efficient steam engine components. By using 3D printing techniques, it’s possible to manufacture highly complex parts that are lighter, stronger, and more heat-resistant. This allows for more precise engineering of components, such as turbine blades and heat exchangers, improving both performance and durability. 3D printing also enables manufacturers to produce parts with better geometries that can improve steam flow and heat transfer efficiency, leading to reduced energy losses and better overall system performance.
Additionally, hybrid systems that combine steam engines with other power generation methods, such as solar-thermal systems, are becoming increasingly viable for a wide range of applications. In hybrid systems, steam engines act as backup or supplementary power sources when solar energy is insufficient or during times of peak demand. This not only increases system reliability but also maximizes the use of renewable energy, reducing the reliance on conventional fuel sources and minimizing environmental impact.
Energy audit programs and real-time performance analytics are powerful tools in monitoring steam engine operations, helping operators identify inefficiencies and areas for improvement. These audits focus on tracking energy use, steam generation rates, and the performance of individual components, enabling precise adjustments to improve efficiency. Performance data can be sent to centralized systems where predictive maintenance algorithms detect patterns that may indicate system inefficiencies, allowing operators to intervene before a potential failure occurs.
The evolving role of digital twins in steam engine performance optimization represents a significant leap forward in predictive maintenance and operational efficiency. Digital twins are virtual models of the steam engine system that simulate its operation in real time. These models enable engineers to test various operational scenarios, predict performance outcomes, and optimize the system before any physical modifications are made. Digital twins can also help track and predict when maintenance is required, ensuring that the engine is always running at its highest efficiency.
The overall trend is clear: steam engine efficiency can be enhanced through a combination of advanced materials, intelligent control systems, integrated renewable energy sources, and cutting-edge manufacturing techniques. By focusing on optimizing every aspect of the steam engine—whether through better fuel combustion, more efficient heat transfer, or innovative technologies like 3D printing and digital twins—industries can continue to benefit from the reliability and versatility of steam engines while pushing the boundaries of what’s possible in terms of energy efficiency, performance, and sustainability.
Maintenance and Repair Services for Steam Engines
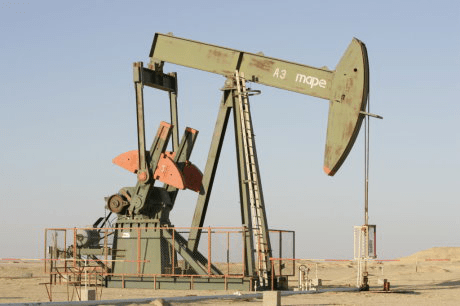
Maintenance and repair services for steam engines are crucial for ensuring that these systems operate efficiently and reliably over time. Given the complex nature of steam engines, regular maintenance is necessary to prevent unexpected breakdowns, maximize the lifespan of components, and optimize performance. Whether in industrial applications, power generation, or transportation, a well-maintained steam engine ensures safe, effective, and energy-efficient operation.
Preventive Maintenance
Preventive maintenance involves scheduled checks and inspections that help identify potential issues before they develop into major problems. This approach focuses on keeping the steam engine in optimal condition and addressing wear-and-tear before it leads to a failure. The key components of preventive maintenance for steam engines include:
- Boiler Inspection and Maintenance:
The boiler is one of the most critical components of a steam engine, as it generates the steam needed for power generation. Regular inspection and maintenance of the boiler are essential to ensure it operates safely and efficiently. This includes checking for:- Corrosion or scale buildup inside the boiler, which can reduce efficiency and lead to equipment damage.
- Water quality checks to ensure proper water chemistry and prevent scaling or fouling.
- Safety valves and pressure controls to ensure they function correctly.
- Burner performance to ensure the combustion process is optimized.
- Turbine and Piston Maintenance:
Turbines or pistons are the mechanical components responsible for converting steam energy into mechanical energy. Regular checks should include:- Lubrication systems to prevent excessive friction, which can lead to overheating and component failure.
- Valve timing and valve seals to ensure they are working efficiently.
- Turbine blade inspection to check for wear or damage, especially in high-pressure turbines.
- Piston ring and cylinder inspection to check for signs of wear, damage, or leaks.
- Condensate System Checks:
The condensate system is responsible for cooling the steam and returning it to water form to be reused in the boiler. Regular maintenance involves:- Checking condensers for proper heat exchange efficiency.
- Inspecting drainage systems for leaks or blockages.
- Ensuring that pumps are operating correctly and not losing efficiency.
- Steam Pipe Inspection and Maintenance:
Steam pipes are used to transport steam from the boiler to the engine or turbine. It’s essential to inspect pipes for:- Leaks or signs of corrosion, which can cause energy losses or safety hazards.
- Thermal insulation to ensure that energy is not lost through uninsulated or damaged sections of pipe.
- Control Systems and Sensors:
Modern steam engines are equipped with various sensors and control systems that regulate temperature, pressure, and flow rates. Regular testing and calibration of these systems ensure optimal operation. Checks should be done to:- Verify pressure, temperature, and flow sensors are calibrated correctly.
- Ensure the control valves are responding accurately to changes in load and operating conditions.
- Test the safety shutdown systems to ensure the engine stops safely in case of malfunction.
- Steam Engine Vibration Monitoring:
Excessive vibrations can indicate problems with alignment, wear, or unbalanced components. Monitoring vibrations regularly can help identify issues like:- Misaligned shafts or bearings.
- Imbalanced rotors or turbine blades.
- Loose or worn-out couplings or mounts.
Corrective Maintenance
Corrective maintenance is performed when a fault or failure occurs, requiring repairs to restore the steam engine to its proper functioning state. This may be scheduled after an inspection reveals damage or when an issue arises unexpectedly during operation. Key areas of corrective maintenance include:
- Boiler Repairs:
Boilers often require repair due to issues such as corrosion, erosion, or scaling. Common repairs include:- Welding or replacing damaged boiler tubes.
- Repacking or replacing seals and gaskets to prevent steam leaks.
- Replacing burners if they are no longer functioning efficiently.
- Turbine or Piston Repairs:
Repairs to turbines or pistons may involve the following:- Blade replacement or resurfacing due to erosion or damage.
- Re-aligning turbines or pistons if misalignment is detected.
- Replacing piston rings that have become worn or damaged.
- Seal replacement for valves to prevent leaks and improve efficiency.
- Condensate System Repairs:
Repairing leaks, replacing worn-out parts, or cleaning the condensate system can involve:- Cleaning condensers and removing debris or scale buildup that hinders heat exchange.
- Replacing condensate pumps that have failed or are not operating efficiently.
- Fixing leaks in the condensate return piping.
- Pipe and Valve Repairs:
Pipe and valve repairs typically involve:- Replacing or sealing leaks in pipes that may have corroded or suffered physical damage.
- Repairing or replacing valves that have become worn out or are not operating correctly.
- Upgrading valve actuators and other control mechanisms to improve reliability.
- Control System Repairs:
Issues with the control systems can affect the steam engine’s ability to respond to changing loads or operating conditions. Repairs in this area may include:- Calibrating or replacing faulty sensors.
- Replacing damaged control valves or actuators.
- Reprogramming or replacing malfunctioning controllers.
- Vibration Troubleshooting:
Corrective action for excessive vibrations might involve:- Balancing rotating parts like turbines or flywheels.
- Re-aligning shafts or bearings to correct misalignment.
- Replacing or tightening loose components that could be causing abnormal vibrations.
Emergency Repairs and Downtime Management
In the event of a steam engine failure, prompt repair services are essential to minimize downtime and prevent further damage. This often involves emergency repairs, which should be handled by trained technicians who can quickly diagnose the issue and provide solutions. Emergency repairs might include:
- Emergency Boiler Repairs:
If the boiler experiences a sudden failure, such as a cracked tube or pressure vessel rupture, quick repairs are necessary to prevent further damage. Emergency welds, temporary seals, or replacement parts might be required until permanent solutions can be implemented. - Turbine or Engine Failures:
Sudden failures in turbines or other engine components require swift identification of the problem, such as worn-out bearings, misalignment, or internal damage. Technicians will often need to disassemble parts of the engine to repair or replace damaged components quickly. - Steam Leaks or Pressure Loss:
In cases where there’s a sudden steam leak or a significant loss of pressure, technicians will need to isolate the faulty section of piping or equipment and repair the leaks. This often involves replacing worn gaskets, damaged seals, or corroded pipes.
Spare Parts and Component Replacement
Maintaining a supply of spare parts is essential for minimizing downtime and ensuring that maintenance and repairs can be performed quickly. Some steam engine parts, such as gaskets, seals, and valves, may require frequent replacement due to wear and tear, while other parts, like turbine blades or boiler tubes, may only need to be replaced once every several years, depending on usage.
OEM (original equipment manufacturer) parts should be used whenever possible to ensure compatibility and optimal performance. Aftermarket parts can sometimes offer a more affordable option, but it’s important to assess their quality and compatibility to avoid performance issues.
Training and Technical Support
Proper maintenance and repair of steam engines also depend on having skilled technicians who understand the intricate workings of these systems. Manufacturers or specialized service providers often offer training programs for operators and maintenance personnel to ensure they have the necessary skills to keep steam engines in top working condition. Technical support services can also be critical, especially for troubleshooting complex issues, performing diagnostics, and obtaining expert guidance on optimizing steam engine performance.
Conclusion
Effective maintenance and repair services for steam engines are essential to keeping these powerful systems running smoothly and efficiently. A well-planned preventive maintenance program can catch issues before they escalate, while corrective maintenance addresses problems that arise unexpectedly. Emergency repairs are critical for minimizing downtime, and a steady supply of spare parts ensures that repairs can be done promptly. With the right combination of expertise, technology, and proactive strategies, steam engines can continue to perform reliably in a wide range of industries and applications.
Maintaining steam engines is not only about keeping them operational but also ensuring their performance stays optimized over time. One of the fundamental aspects of effective maintenance is the ability to anticipate potential failures before they occur, which can drastically reduce the impact of downtime and repair costs. The key to achieving this lies in establishing a robust maintenance plan that includes predictive maintenance based on data-driven insights. Advances in technology, such as sensor-based monitoring and IoT connectivity, allow for real-time data collection from the engine’s components. This data can be analyzed to detect patterns, such as increased vibrations, temperature shifts, or pressure fluctuations, that could signal potential issues before they become critical.
For instance, vibration analysis can help detect misalignment or imbalance in rotating parts like the turbine or crankshaft. When a specific threshold is exceeded, this could indicate wear, friction, or improper alignment, leading to more extensive damage if left unaddressed. Similarly, temperature monitoring can provide early warnings for overheating components or issues in the boiler, allowing operators to take corrective action before the problem worsens.
Another important aspect of maintaining steam engines is ensuring that the lubrication system is functioning properly. Over time, lubrication can degrade, and the buildup of contaminants can result in increased friction and accelerated wear on moving parts. Regular inspection of oil filters and ensuring proper lubrication levels can prevent such issues. In many modern systems, automated lubrication monitoring can signal when levels drop or when oil quality deteriorates, prompting timely refills or changes.
The design of the steam engine itself plays a significant role in long-term maintenance needs. Engineers and manufacturers continually look for ways to improve component durability, reducing the frequency of repairs. Using advanced materials, such as heat-resistant alloys, and employing coatings that can withstand high temperatures and pressures, extends the lifespan of parts like turbine blades, seals, and bearings. Additionally, modular designs allow for easier replacement and repair of individual components without having to dismantle the entire system, which is essential for minimizing downtime in industries that rely on steam power.
Maintaining efficient combustion processes within the steam engine is crucial for both performance and safety. As the combustion chamber deteriorates, it can lead to incomplete burning of fuel, producing excess soot or unburned particles, which can compromise the overall efficiency of the steam cycle. Cleaning the combustion chamber, inspecting burners, and checking for fuel consistency are important tasks to ensure that the fuel is burned efficiently. Proper combustion also impacts emissions, and in industries where environmental regulations are stringent, ensuring that steam engines operate within legal limits is essential to avoid penalties.
The steam turbine or piston in a steam engine also requires attention. Over time, the blades or pistons may experience wear, which can affect the engine’s power output. Turbine blades may need to be sharpened or replaced due to erosion from high-pressure steam or mechanical stresses. Similarly, piston rings and cylinders should be inspected for signs of wear or damage. Small imperfections in the piston ring can lead to steam leaks, reducing engine efficiency and increasing fuel consumption.
Ensuring that the steam condenser and heat exchangers remain clean and free of obstructions is also essential for maintaining efficiency. Condensers often deal with scale buildup or corrosion due to prolonged exposure to hot steam and water. Regularly checking and cleaning these components can prevent a decrease in the heat transfer efficiency, which in turn ensures the steam engine operates at its peak.
In addition to the mechanical aspects, systematic testing of safety features is vital for ongoing steam engine maintenance. Components like pressure relief valves, emergency shutdown systems, and alarm systems should be routinely tested to ensure they function correctly under stress. These safety measures help protect against catastrophic failures, which can result in significant damage to the engine or even pose safety risks to operators.
The role of training for operators and maintenance personnel cannot be overstated. Properly trained staff can perform daily checks and maintenance tasks with greater efficiency, spotting issues early on and responding appropriately. Manufacturers often provide detailed training sessions, including how to troubleshoot common problems and interpret system diagnostics. Additionally, ongoing education ensures that maintenance teams stay up-to-date with the latest technologies and methodologies for optimizing steam engine performance.
Inventory management for spare parts is another important consideration. Steam engines often require parts that may need to be replaced on a regular basis, such as gaskets, seals, and control valves. Ensuring that a stock of critical components is readily available reduces the risk of extended downtime when parts are needed. Some organizations also utilize supply chain management tools that allow for better tracking of spare parts, ensuring that replacements are ordered before stock levels run low.
One of the growing trends in steam engine maintenance is the implementation of remote monitoring. Many modern steam engines come equipped with remote diagnostics capabilities that allow for monitoring and analysis of the engine’s health from a distance. This technology enables engineers to access performance data in real-time, receive alerts about potential problems, and even perform remote troubleshooting. Remote monitoring helps identify issues early on, which means fewer on-site visits and quicker resolution times. It also allows for predictive maintenance where specific components are replaced or serviced based on usage patterns, ensuring that maintenance efforts are both proactive and cost-effective.
The importance of environmental considerations in steam engine maintenance is also gaining prominence. Industries that rely on steam power must ensure that their systems comply with environmental regulations regarding emissions, water usage, and waste management. Routine checks and maintenance procedures that address emission control systems, such as scrubbers and filters, are important to reduce the environmental footprint. Similarly, water treatment systems used in the boiler and condenser cycles must be monitored to prevent contamination and ensure that wastewater discharge meets regulatory standards.
Another area of focus is the upgrading or retrofitting of older steam engines with newer technologies. Over time, some steam engine designs may become outdated or less efficient compared to more modern systems. Retrofitting a steam engine with new components, such as improved turbine blades, better boilers, or more efficient control systems, can enhance its performance and extend its useful life. While retrofitting can be an expensive investment upfront, the long-term gains in efficiency and reliability can make it a worthwhile solution for older systems.
Finally, ongoing performance audits are essential to maintaining a steam engine’s efficiency. These audits involve a detailed examination of the engine’s operating data and performance history, including fuel consumption, steam production, and system efficiency. By continuously analyzing performance trends, it becomes easier to identify areas for improvement and plan for future upgrades or maintenance needs. Regular performance audits help ensure that steam engines remain productive and cost-effective over their entire operational life.
In conclusion, maintaining and repairing steam engines requires a proactive, data-driven approach that involves regular inspection, predictive maintenance, and continuous performance monitoring. By keeping components in top condition, addressing issues before they lead to significant failures, and investing in new technologies, industries can maximize the efficiency, reliability, and longevity of their steam engines.
To further enhance the long-term sustainability of steam engines, businesses can implement a more holistic approach to their maintenance and operation. This may include adopting sustainable practices that focus not only on the machine’s operational efficiency but also on reducing the environmental impact throughout its lifecycle.
One of the emerging areas in steam engine optimization is the integration with renewable energy sources. As industries strive for greener, more sustainable operations, steam engines are being coupled with alternative energy systems, such as solar, wind, and biomass. These renewable energy sources can help provide supplemental power to steam engines, reducing the reliance on fossil fuels and lowering greenhouse gas emissions. For example, in industrial applications, a steam engine can use waste heat from a solar thermal system, making the entire process more energy-efficient. Similarly, biomass-based systems, where steam engines run on organic waste products, provide a renewable energy source and help reduce waste disposal issues.
Another area of development is cogeneration systems, where steam engines are used to simultaneously produce both power and heat. These systems increase the overall efficiency of energy usage by utilizing the waste heat from the steam engine to provide hot water or heating for other processes. In certain industries, this not only makes the steam engine more energy-efficient but also reduces the need for separate heating systems, thereby lowering operational costs and reducing the carbon footprint.
Moreover, the design of steam engines is evolving toward greater modularity and customization. Modern steam engines can be designed to fit the specific needs of different industries, ensuring they are not overbuilt or underutilized. These custom systems help reduce energy waste and improve cost-effectiveness. In some cases, modular designs allow for easy scaling depending on the operational demand, where parts can be added or removed without extensive reconfiguration. This is particularly beneficial in industries with fluctuating energy needs, where flexibility in operation can result in significant savings and more efficient energy management.
Digital technologies are also playing a crucial role in advancing steam engine operations. The integration of smart sensors, Internet of Things (IoT) technologies, and cloud computing allows for real-time monitoring of steam engine performance from any location. The data collected from various sensors can be stored and analyzed to detect anomalies in the system, such as unexpected pressure drops or temperature variations. The use of predictive analytics based on historical data helps identify potential maintenance needs well in advance, which reduces unplanned downtimes and optimizes maintenance schedules.
In addition, artificial intelligence (AI) is becoming increasingly important in steam engine optimization. AI algorithms can be applied to predict performance issues, optimize fuel consumption, and automate parts of the maintenance process. For example, AI can analyze the data from sensors and maintenance logs to predict when a component is likely to fail, allowing maintenance teams to act proactively. This approach not only improves engine performance but also extends the useful life of the equipment.
The growing use of 3D printing in steam engine manufacturing and repair is another significant advancement. 3D printing allows for the creation of complex, custom parts quickly and at a fraction of the cost of traditional manufacturing methods. This is particularly useful when replacing parts that are no longer in production or hard to source. Additionally, 3D printing can be used to create prototypes for new parts or test designs, allowing engineers to experiment with innovative materials and configurations to enhance the engine’s performance or efficiency.
Advanced materials science continues to push the boundaries of what steam engines can achieve. The development of superalloys that can withstand higher temperatures and pressures extends the lifespan of steam engine components. These materials are often more resistant to corrosion and erosion, reducing the frequency of repairs. Research into ceramic coatings is also being explored, as these coatings can enhance the thermal efficiency of steam engines by providing better insulation and protection for critical components like turbine blades and boiler walls.
Furthermore, the operation and management of steam engines are evolving with the increasing emphasis on sustainability and corporate responsibility. Many industries are moving towards energy management systems that track not only steam engine performance but also the overall energy consumption and carbon footprint of an entire facility. These systems enable organizations to optimize energy usage and adopt energy-saving strategies, such as reducing steam production when demand is low or using excess energy for other purposes within the facility. Additionally, incorporating green certifications and adhering to sustainability guidelines can improve a company’s reputation and ensure compliance with environmental regulations.
Fleet management is another critical area where technology is playing a key role. For industries operating multiple steam engines across different sites, centralized fleet management software can help monitor and coordinate maintenance, performance, and operations. This software can aggregate data from multiple machines and locations, providing operators and managers with a clear picture of system health and identifying trends or areas that need attention. Predictive maintenance tools integrated into these systems can alert teams about upcoming issues, ensuring that no equipment is left unchecked or neglected.
As industries face increasing pressure to reduce emissions and energy consumption, steam engine manufacturers are working toward creating low-emission steam systems that meet stringent environmental standards. By improving combustion processes, using cleaner fuels, and employing advanced filtration and emission reduction technologies, the environmental impact of steam engines can be significantly minimized. Some of these systems include carbon capture technologies, which capture CO2 emissions from the steam cycle and store them or convert them into useful products, further reducing the carbon footprint.
Regulatory compliance also remains a major consideration in steam engine operation and maintenance. Adhering to both local and international standards for emissions, safety, and performance is critical. For example, steam engines in power generation or industrial facilities must meet strict safety regulations, ensuring that operators are not exposed to dangerous conditions such as excessive heat, pressure, or toxic fumes. Regular audits and inspections are required to ensure compliance with these regulations, and failure to adhere to them can result in fines, equipment shutdowns, or even legal repercussions.
Looking ahead, integration with modern energy grids will likely become more common, especially with the rise of smart grid technologies. Steam engines may become part of distributed energy systems where they provide backup power during peak demand times or when renewable energy sources like solar or wind are not producing enough electricity. In this model, steam engines can act as a stabilizing force, ensuring that the grid remains reliable and resilient, especially as it becomes more dependent on variable energy sources.
In conclusion, maintaining and optimizing steam engines in the modern industrial landscape involves a combination of advanced technologies, sustainable practices, and a deep understanding of the system’s mechanics. By embracing predictive maintenance, digital tools, renewable energy integration, and advanced materials, industries can enhance the efficiency, reliability, and environmental performance of their steam engines. As technology continues to evolve, steam engines will remain a key component in energy generation and industrial processes, but their operation will continue to adapt to the demands of a more sustainable and interconnected world.
Steam Engine for Home Use: Efficient Energy Solutions
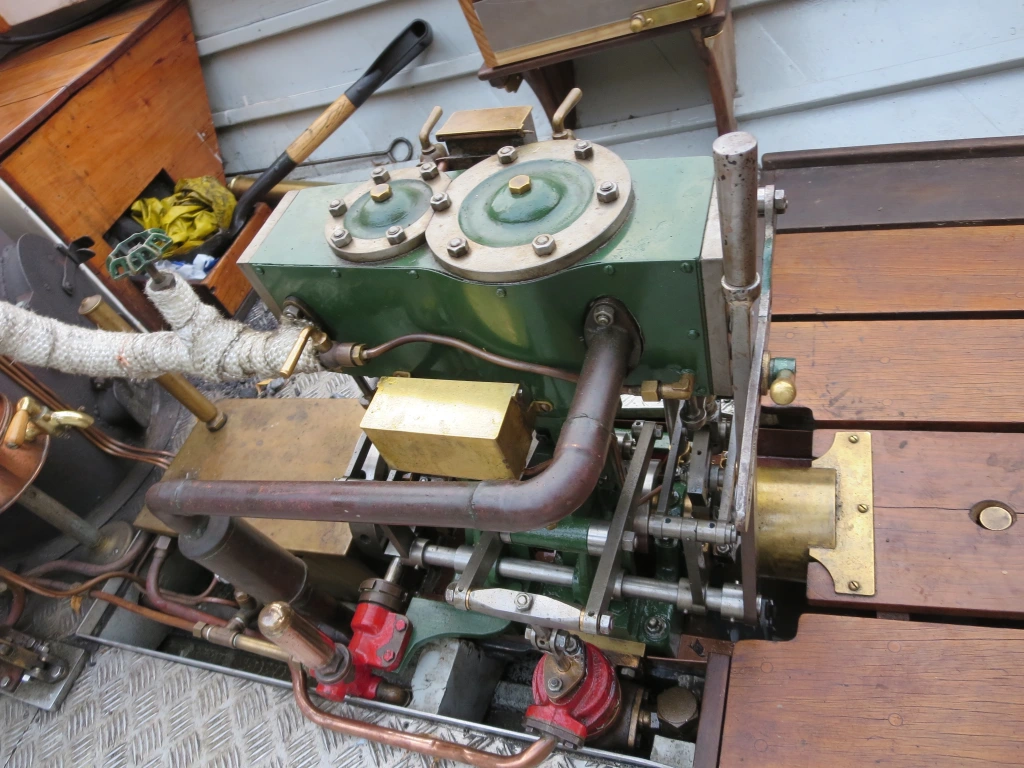
Steam engines for home use are an intriguing option for individuals seeking alternative and sustainable energy solutions. While steam engines are more commonly associated with industrial applications, there are a growing number of innovations that allow for their use in residential settings. These systems offer a way to generate electricity or provide heat for homes, often utilizing renewable resources or waste heat from other systems. By adopting steam engine technology for home use, homeowners can reduce their dependence on traditional energy sources, lower energy bills, and contribute to a more sustainable future.
Benefits of Steam Engines for Home Use:
- Renewable Energy Integration: One of the most appealing aspects of steam engines for home use is their compatibility with renewable energy sources like solar, biomass, and geothermal energy. Homeowners can install steam engines that run on renewable fuels, such as wood pellets or biomass, turning waste materials into useful energy for heating or electricity generation. By utilizing waste products like agricultural or forestry residues, homeowners can reduce their carbon footprint while generating power or heat.
- Heat Generation: Steam engines, particularly those integrated with combined heat and power (CHP) systems, are well-suited for producing both electricity and heat. These systems capture the heat generated by the steam process and use it to warm the home or provide hot water. The ability to combine heat and power in one unit makes steam engines highly efficient, as they make use of the full energy potential of the fuel.
- Cost Savings: While the initial investment in a steam engine for home use can be high, the long-term savings can be significant. By generating your own electricity or heat, you can reduce your reliance on the power grid and cut monthly utility bills. In rural or off-grid locations, a small-scale steam engine can serve as a reliable energy source, providing homeowners with independence from utility companies. Additionally, excess electricity produced by the steam engine can be sold back to the grid, providing an additional income stream through net metering programs.
- Sustainability: Steam engines for home use align well with the increasing focus on sustainability and environmental responsibility. With the ability to use biomass and waste products, homeowners can lower their reliance on fossil fuels and contribute to reducing greenhouse gas emissions. Furthermore, by improving energy efficiency through the combination of heat and power generation, homeowners can maximize the use of available resources while minimizing waste.
- Low Emissions: Unlike traditional fossil fuel-based heating systems, modern steam engines designed for home use can be optimized to burn cleaner fuels with reduced emissions. By using biomass, such as wood chips or pellets, or integrating carbon capture technologies, emissions can be minimized, ensuring that the system adheres to stringent environmental regulations.
Key Considerations for Steam Engines in Residential Applications:
- System Size and Scalability: Steam engines for home use are generally smaller than industrial systems, but size can still vary significantly depending on the energy needs of the household. The size of the engine required will depend on factors such as the home’s square footage, energy consumption, and whether the system is intended for heating, power generation, or both. Homeowners need to ensure that the steam engine they choose can meet their needs without being oversized, which would lead to wasted energy and unnecessary costs.
- Fuel Supply and Storage: A critical consideration when using a steam engine for home use is ensuring a steady and reliable fuel supply. If using biomass, homeowners will need a consistent source of fuel, whether that’s wood pellets, agricultural waste, or another form of organic material. Depending on the location and fuel type, homeowners may need to invest in fuel storage solutions to ensure they have enough material for the system to operate efficiently throughout the year.
- Maintenance: While steam engines are known for their durability, regular maintenance is essential to keep them running efficiently. This includes checking boiler pressure, cleaning combustion chambers, and inspecting components like turbines, valves, and pipes. The complexity of a steam engine system means that periodic servicing by trained technicians may be required, so homeowners need to be prepared for both regular maintenance and occasional repairs.
- Installation: Installing a steam engine in a home requires a significant amount of planning and professional expertise. The installation process can be complex and requires specialized knowledge in steam technology, piping, and electrical integration. It’s crucial to work with experts who can design and install a system that maximizes efficiency and complies with local safety codes and regulations. Depending on the scale of the project, homeowners may also need to consider the structural integrity of their property to accommodate the system.
- Water Management: Since steam engines operate using water in the form of steam, water management is an important consideration. Homeowners must ensure they have access to a consistent and reliable water supply, as well as systems for water treatment and condensation recovery. The system should be designed to recycle water efficiently, reducing consumption and minimizing waste.
- Regulations and Permits: Before installing a steam engine, it’s important to check local regulations and zoning laws. In some regions, there may be restrictions on the use of certain fuels or emissions levels. Homeowners must also ensure that their system meets safety standards and complies with building codes. Obtaining the necessary permits and approvals from local authorities will help avoid legal issues and ensure the system is safe to operate.
Types of Steam Engines for Residential Use:
- Biomass Steam Engines: Biomass steam engines are particularly popular for residential use due to their ability to use renewable organic materials as fuel. These systems can burn wood pellets, agricultural waste, or other biomass materials to generate steam, which is then used to drive a turbine or produce heat. Biomass steam engines are ideal for homeowners who have access to large quantities of waste wood or agricultural byproducts.
- Geothermal Steam Engines: Geothermal steam engines take advantage of the heat from the Earth’s core to produce steam. A geothermal heat pump can be used to extract heat from the ground, which is then converted into steam for power generation or heating purposes. While the initial installation of geothermal systems can be costly, they provide a highly sustainable and low-maintenance energy source in the long term.
- Solar-Powered Steam Engines: Solar steam engines use concentrated solar power (CSP) to generate steam. Mirrors or lenses are used to focus sunlight onto a boiler, where water is converted into steam. These systems are most effective in regions with abundant sunlight. Solar steam engines can be used for both residential power generation and heating, and they offer a completely renewable energy solution when combined with storage technologies.
- Miniature Steam Turbines: Small-scale steam turbines are another option for homeowners looking for a way to generate electricity from steam. These turbines can be driven by a variety of fuels, including natural gas, wood pellets, or even waste heat from industrial processes. While they may not be as common in home use, their ability to produce power in a compact form makes them an intriguing option for off-grid applications.
Future of Steam Engines in Homes:
As technology continues to evolve, steam engines for home use will become more efficient and accessible. Innovations in materials, automation, and renewable energy integration are driving down costs and making these systems more user-friendly. The future of home steam engines will likely involve smart systems that integrate seamlessly with other home energy management solutions, providing homeowners with full control over their energy generation, consumption, and savings.
Additionally, as the world continues to focus on reducing carbon emissions and transitioning to sustainable energy sources, steam engines, particularly those powered by biomass or renewable fuels, will play a more significant role in decentralizing energy production. For homeowners looking to reduce their environmental impact and increase energy independence, steam engines present an exciting and practical solution.
The potential for steam engines in residential applications extends beyond just heating and power generation. As energy demands grow and sustainability becomes a key priority, homeowners are seeking out alternative, renewable, and energy-efficient technologies. Steam engines offer a unique solution for achieving both energy independence and reducing environmental impact. In addition to the traditional applications of producing heat and power, innovative advancements in steam engine technology are opening up new possibilities for home use.
For instance, waste-to-energy systems are becoming increasingly popular for residential use. By converting household waste, such as food scraps, garden waste, or even plastic, into steam and subsequently electricity, homeowners can generate their own power while reducing the volume of waste sent to landfills. These systems are particularly useful in urban or suburban settings where waste management can be a significant challenge. By leveraging a steam engine’s ability to burn waste for energy, homeowners can reduce their reliance on external waste collection services while benefiting from the energy generated.
Moreover, integrated home heating systems that use steam engines could work in synergy with other advanced home technologies. Smart home integration allows homeowners to manage not just their heating or power generation but also how and when energy is used. For instance, when energy demand is low, a steam engine can store excess heat for later use, helping to balance out the fluctuations in power demand that are common in homes powered by intermittent renewable energy sources like solar or wind. This way, a steam engine could contribute to overall home energy management, reducing reliance on the grid during peak demand periods, and cutting down on energy costs.
Additionally, with the growing interest in green building technologies, steam engines could be integrated into passive house designs or other energy-efficient homes. These buildings are built to be as energy-efficient as possible, and integrating a steam engine that generates both power and heat could significantly reduce a home’s overall energy footprint. Such designs might feature highly insulated walls, solar panels, geothermal heating, and steam-driven systems, working in harmony to maintain optimal energy use.
Another trend is the growing interest in off-grid living, particularly in rural or remote areas where access to conventional power grids may be limited. Steam engines can offer a reliable and independent source of energy, making off-grid living not only feasible but also sustainable. Homeowners can set up their own steam-powered systems, relying on locally sourced renewable fuels, such as wood chips, agricultural waste, or even biogas from a household composting system. This autonomy is particularly valuable for those seeking self-sufficiency in both energy and resource management.
While residential steam engines are not yet widespread, the advancements in smaller-scale systems, coupled with the drive for renewable energy adoption and sustainability, make them a highly viable option for the future. As interest in steam engines for home use grows, the costs of installation and operation are expected to decrease, thanks to increased manufacturing efficiencies and improvements in technology. New, more efficient designs will be developed, with easier installation processes, smaller footprints, and lower maintenance requirements.
With innovative engineering and growing environmental awareness, steam engines could eventually become a staple in residential energy systems, contributing to net-zero homes and helping homeowners take control of their energy production. The increased use of smart technologies, better fuel sourcing, and a shift toward localized energy production will further enhance the appeal of steam engines in households that prioritize sustainability, cost-effectiveness, and independence.
By integrating steam engines into modern homes, homeowners could harness the benefits of clean energy, while reducing carbon emissions and reliance on fossil fuels, making a positive contribution to a more sustainable future. With the right investment in research and development, residential steam engines have the potential to revolutionize how homes generate and use energy, setting a new standard for eco-friendly living.
As the demand for sustainable and renewable energy sources continues to grow, the role of steam engines in residential applications is likely to expand further. Advances in miniaturization and modular design will play a significant role in making steam engines more accessible to homeowners. These smaller, scalable systems could be easily integrated into existing homes, making them a viable option for a broader range of users. Additionally, these modular designs would allow homeowners to customize the system based on their specific energy needs, ensuring that the steam engine is both efficient and cost-effective.
Another promising development in residential steam engine technology is the use of advanced thermodynamic cycles. By improving the efficiency of the steam cycle through innovations such as regenerative heating, superheating, or Rankine cycle modifications, homeowners could extract more energy from the same amount of fuel. These advances would enable steam engines to produce more electricity or heat with less fuel, further enhancing their appeal as a low-carbon energy source.
The integration of battery storage with steam-powered systems could also unlock new potential. While steam engines themselves can produce reliable energy, pairing them with energy storage solutions like batteries would allow homeowners to store excess energy generated during the day for use at night or during periods of high demand. This would help smooth out the intermittent nature of renewable energy production, ensuring a continuous and stable supply of power. The ability to store energy would also make steam engines more adaptable to a variety of energy needs, whether for heating, power generation, or both.
Hybrid systems that combine steam engines with other technologies, such as solar thermal systems, wind turbines, or heat pumps, are another exciting development. By integrating steam engines with these complementary technologies, homeowners can create a more resilient and versatile energy system that can operate effectively in a variety of weather conditions and energy demand scenarios. For example, during periods of low sunlight or wind, the steam engine could take over and continue to provide heat or power, while during peak solar or wind energy production, the renewable system could supply the bulk of the energy needs. This flexibility ensures that the home remains energy-efficient, even when one form of energy production is unavailable.
The future of residential steam engines also involves smart grid integration. With the rise of smart grids that enable bidirectional communication between energy producers and consumers, homeowners with steam engines could participate in demand-response programs. This means they could adjust their energy consumption based on grid demand, either reducing or increasing their energy production depending on what is needed. For instance, during peak times, the steam engine could contribute excess power to the grid, helping to stabilize it and potentially generating additional income for the homeowner through feed-in tariffs or other incentive programs. Conversely, during times of low demand, the homeowner could reduce their energy production, conserving resources and lowering costs.
Another important trend is the development of advanced fuels for steam engines. While traditional fuels like coal or natural gas have been used for steam generation, there is growing interest in alternative fuels that are cleaner and more sustainable. Hydrogen, for example, is emerging as a promising fuel for steam engines, particularly when used in a hydrogen steam cycle. Hydrogen can be produced through renewable methods such as electrolysis powered by solar or wind energy, offering a completely clean fuel source for steam engines. The use of hydrogen in steam engines would significantly reduce carbon emissions and contribute to the development of a hydrogen-based economy, offering a potential solution for both home and industrial energy needs.
Additionally, advancements in steam engine materials are also making these systems more efficient and durable. New materials, such as ceramic composites and high-performance alloys, are being developed to withstand the extreme temperatures and pressures found in steam engines. These materials can improve the performance of the steam cycle, increase the lifespan of critical components, and reduce the frequency of maintenance and repairs. As these materials become more affordable and widely available, they will further reduce the cost of installing and maintaining steam engines for home use.
The carbon-neutral potential of steam engines is another factor driving their future adoption. As more homeowners and industries seek ways to reduce their carbon footprints, steam engines that run on renewable fuels like biomass or hydrogen offer a compelling alternative to fossil fuel-based energy systems. The ability to generate heat and power with minimal carbon emissions positions steam engines as a critical component of a sustainable energy ecosystem, especially as global efforts to combat climate change intensify.
Steam engines for residential use also have the potential to bring communities together in ways that traditional energy sources cannot. For instance, small-scale steam engines could be part of a community-based energy system where multiple homes or buildings share a steam engine to generate heat or power collectively. These systems could operate on a cooperative model, with households contributing to the fuel supply and sharing the energy produced. This kind of localized energy generation could reduce the overall cost of energy for individual households and foster a sense of shared responsibility for sustainability.
As governments around the world introduce stricter regulations on energy consumption and emissions, there will likely be increased incentives for homeowners to invest in energy-efficient technologies like steam engines. These incentives could take the form of tax credits, grants, or low-interest loans, making steam engine systems more affordable and attractive to homeowners. Additionally, the global push for net-zero emissions by 2050 is expected to drive significant investment in clean energy technologies, accelerating the development and commercialization of steam engines as a viable option for residential energy production.
In the long term, residential steam engines could play an integral role in the transformation of global energy systems. By enabling decentralized energy production and local resilience, these systems could reduce the strain on traditional energy grids and improve the reliability of power supply, especially in remote or underserved areas. As technology advances and public awareness of the benefits of clean energy grows, steam engines could become a key pillar of sustainable home energy solutions, providing homeowners with an efficient, eco-friendly, and cost-effective way to meet their energy needs.
Understanding the Mechanics of Steam Engine Design
Understanding the mechanics of steam engine design is crucial for both engineers and enthusiasts who wish to comprehend how these powerful machines operate. At its core, a steam engine converts heat energy from steam into mechanical energy through a series of components that work together to harness the power of steam. To understand how this process unfolds, it’s important to break down the design and mechanical workings of a steam engine, focusing on its main parts and their function.
Key Components of Steam Engine Design:
- Boiler:
The boiler is the heart of the steam engine, where heat is applied to water to generate steam. It consists of a large vessel that contains water, which is heated by burning fuel (such as coal, oil, or biomass) in a furnace. The heat causes the water to evaporate, turning it into steam under pressure. The boiler is designed to withstand high temperatures and pressures, and it plays a key role in determining the efficiency of the steam engine. - Steam Cylinder:
The steam cylinder is where steam is introduced into the engine to do work. The steam is directed into the cylinder under pressure and causes a piston to move back and forth within the cylinder. This reciprocating motion is the primary form of mechanical energy that powers the steam engine. The size and shape of the steam cylinder, as well as the amount of pressure applied to the steam, determine the force and efficiency of the engine. - Piston and Piston Rod:
The piston is a critical part of the steam engine. It is a metal disc that fits snugly inside the steam cylinder and moves in response to the steam pressure. As steam enters the cylinder, it pushes the piston, creating a linear motion. This piston is connected to a piston rod, which transfers the mechanical motion of the piston to the crankshaft or another mechanical system that converts it into usable power. - Crankshaft:
The crankshaft is connected to the piston rod and converts the reciprocating motion of the piston into rotational motion. As the piston moves back and forth, the piston rod turns the crankshaft. The crankshaft is a crucial component that powers machinery or provides rotational motion for driving other equipment, such as wheels or generators. - Valve Mechanism:
The valve mechanism controls the flow of steam into and out of the steam cylinder. It directs steam to one side of the piston and exhaust steam out of the cylinder on the opposite side. The valve system is typically driven by the crankshaft through a system of gears or cams. It ensures that the steam engine operates efficiently by managing the timing of steam admission and exhaust. Common valve mechanisms include slide valves, poppet valves, and rotary valves. - Flywheel:
The flywheel is a large, heavy wheel attached to the crankshaft. Its primary purpose is to store energy during the engine’s power strokes and release it during non-power strokes. This helps maintain a smooth, continuous operation by compensating for the periodic nature of the piston’s motion. The flywheel is crucial for stabilizing the speed of the engine, especially in older designs where the engine’s power output could fluctuate significantly. - Condensers:
In some steam engines, especially those used for power generation, the condenser is used to cool and condense the exhaust steam from the engine. This allows for the recovery of heat from the exhaust steam and can help improve the engine’s overall efficiency. The condenser is a key component in closed-loop systems, where steam is continually recycled back into the boiler after being condensed. - Governor:
The governor is a device that helps regulate the speed of the steam engine to ensure it doesn’t operate too fast or too slow. It automatically adjusts the amount of steam entering the engine based on the speed of the engine’s rotation. This ensures a consistent performance level and protects the engine from damage due to excessive speed. There are different types of governors, such as centrifugal governors, which adjust steam flow in response to the engine’s speed. - Exhaust System:
The exhaust system directs the used steam out of the engine after it has completed its work. This system includes the exhaust port, exhaust pipe, and any additional cooling or condensing systems, such as a water tank or radiator. The efficient removal of exhaust steam is critical for the engine’s performance, as it helps prevent excessive pressure buildup and reduces energy loss.
How the Steam Engine Works:
- Heat Generation:
The steam engine begins its operation by burning fuel (e.g., coal or wood) in the boiler to heat water. The boiler raises the water to a boiling point, turning it into high-pressure steam. This steam is then directed into the steam cylinder. - Steam Admission:
The steam valve opens to admit steam into the steam cylinder. The steam pressure pushes the piston, causing it to move along the cylinder. The piston’s motion is linear, and this motion is transferred to the crankshaft through the piston rod. - Mechanical Motion:
The crankshaft, which is attached to the piston rod, converts the reciprocating motion of the piston into rotational motion. This rotational motion can be used to drive machinery, such as a pump, generator, or vehicle wheels, depending on the application of the steam engine. - Exhaust Steam:
After the steam has done its work, it must be expelled from the cylinder. The exhaust valve opens to release the used steam out of the cylinder. In a traditional steam engine, this exhaust steam is vented into the open air, but in more modern systems, a condenser might be used to capture and cool the exhaust steam for reuse. - Cycle Repeats:
The process repeats in cycles. As steam is admitted to the cylinder, the piston moves, and then the steam is exhausted, allowing the piston to return. This continuous cycle produces mechanical energy, which can be used to do work.
Design Variations and Their Impact:
While the basic mechanics of a steam engine remain relatively consistent, there are several variations in design that influence the performance, efficiency, and application of steam engines:
- Single vs. Double Acting:
A single-acting steam engine uses steam to move the piston in one direction, while a double-acting steam engine uses steam on both sides of the piston, creating more power with each cycle. - High-Pressure vs. Low-Pressure:
High-pressure steam engines operate at higher steam pressures, allowing them to generate more power and operate more efficiently. Low-pressure engines are simpler but less powerful. - Compound Engines:
Compound steam engines use multiple cylinders with different sizes, where steam is expanded in stages to extract more energy from the same amount of steam. This increases efficiency compared to a simple steam engine that uses a single cylinder. - Radial vs. Inline Configurations:
Steam engines can have different configurations depending on the application. Radial engines (where cylinders are arranged in a circular pattern) are often used in aircraft, while inline configurations (where cylinders are arranged in a straight line) are common in stationary or industrial engines.
Conclusion:
Steam engine design is an intricate process that combines thermodynamics, mechanical engineering, and material science to create a powerful machine capable of converting heat into mechanical energy. By understanding the key components of steam engine design, engineers can optimize performance, increase efficiency, and adapt steam engines for various applications, from industrial power generation to transportation. Advances in modern steam engine technology, such as improved materials, sophisticated valve mechanisms, and hybrid systems, continue to enhance the performance and potential of steam engines, ensuring their relevance even in today’s renewable energy landscape.
The basic principle behind the steam engine is the conversion of heat energy from steam into mechanical energy. This energy transformation happens through a series of steps, where components like the boiler, steam cylinder, and piston work together to harness the power of steam. The steam engine operates through a repeating cycle where heat is generated, steam is introduced into the cylinder, the piston moves, and then the exhaust is released. The engine’s ability to perform mechanical work comes from this continuous cycle of steam expansion and condensation.
As the steam engine works, it generates power, which is transferred through the crankshaft to drive machines or vehicles. The mechanical work produced by the engine can be used to turn wheels, pump water, or generate electricity. This fundamental process, while simple, requires careful engineering to ensure that the engine operates efficiently and safely.
One of the key factors in the performance of a steam engine is the amount of pressure at which the steam is generated. Higher steam pressure allows for more energy to be harnessed from the steam, making the engine more powerful and efficient. Over time, engineers have developed various strategies to manage this pressure and extract as much energy as possible from the steam. Innovations in valve systems, such as poppet valves or slide valves, have improved the timing and flow of steam into the engine, which directly impacts the engine’s efficiency.
The design of the boiler plays a critical role in the overall performance of a steam engine. Boilers must be constructed from materials capable of withstanding the extreme temperatures and pressures created by the steam. As the steam engine industry evolved, so did boiler technology, with early designs often being prone to catastrophic failures. Modern boilers incorporate multiple safety features, such as pressure relief valves and automatic shutdown systems, to prevent accidents and ensure stable operation.
Steam engines are also influenced by the materials used in their construction. Over the years, advancements in alloys and ceramics have allowed for better heat resistance and structural integrity, leading to longer lifespans for components like pistons, valves, and cylinders. The development of these materials has significantly increased the reliability and performance of steam engines, enabling them to operate in more demanding environments, such as power plants or heavy-duty industrial applications.
The efficiency of a steam engine can be further enhanced by improving the design of the condenser. In traditional steam engines, the exhaust steam is simply released into the atmosphere. However, more modern systems incorporate condensers to cool the exhaust steam and convert it back into water, which can then be reused in the boiler. This closed-loop system reduces water consumption and increases overall energy efficiency. Condensers also help to maintain the engine’s pressure, allowing it to operate at more optimal levels.
Governors, which regulate the speed of the engine, also play a key role in maintaining the engine’s stability. By automatically adjusting the steam flow based on the speed of the engine, governors ensure that the engine runs smoothly without over-revving or underperforming. These devices are especially important in industrial settings, where maintaining a constant speed is crucial for the proper functioning of machinery.
Over time, steam engine designs have adapted to meet changing energy demands. For example, compound steam engines, which use multiple cylinders for different stages of steam expansion, offer higher efficiency compared to simpler engines. These engines reduce energy loss by utilizing steam more effectively, which is why they are commonly used in power plants and large industrial applications. Similarly, modern steam turbines, which are a derivative of steam engines, have revolutionized the power generation industry by improving efficiency and output.
As steam engine technology continues to evolve, it has found applications not only in traditional industries but also in emerging sectors like renewable energy. Modern innovations in steam engine design aim to increase efficiency, reduce emissions, and integrate renewable fuel sources, such as biomass and hydrogen, into the system. This adaptability has made steam engines a viable option for future energy solutions, especially in remote areas where access to traditional grid power may be limited.
The continued refinement of steam engine technology, from the choice of materials to advanced thermal cycles, holds significant promise for improving the efficiency and sustainability of energy systems. By utilizing cleaner fuels, optimizing performance, and incorporating modern control technologies, steam engines are poised to remain an essential component of both industrial power generation and future green energy systems. Whether through advanced hybrid systems, solar-assisted steam generation, or even hydrogen-powered steam engines, the future of steam engines is full of innovative possibilities that can contribute to a more sustainable energy future.
The future of steam engine technology is increasingly focused on improving energy efficiency, reducing emissions, and adapting to renewable energy sources. As global attention turns toward more sustainable and eco-friendly solutions, steam engines are being reimagined to meet the demands of modern energy systems. The ability of steam engines to operate on a variety of fuels, including biomass, solar energy, and even hydrogen, makes them incredibly versatile. This flexibility positions steam engines as a key technology in the transition to renewable energy systems.
In particular, hydrogen steam engines are gaining attention as a potential solution for reducing carbon emissions. Hydrogen, when used as a fuel, produces only water vapor as a byproduct, making it a clean alternative to traditional fossil fuels. Research into hydrogen-powered steam engines is still ongoing, but it holds the promise of reducing the carbon footprint of industrial applications while still harnessing the power of steam technology. These engines would work similarly to traditional steam engines but would rely on the combustion of hydrogen to generate steam instead of coal or natural gas.
Additionally, the integration of solar thermal energy into steam engine designs is a promising area of innovation. Solar thermal systems collect sunlight and convert it into heat, which can then be used to generate steam. When combined with steam engines, these systems have the potential to provide clean, renewable energy for a variety of applications, including electricity generation, industrial heating, and even residential power production. The ability to use solar energy to generate steam represents an exciting opportunity for reducing reliance on nonrenewable resources and contributing to a more sustainable energy future.
Biomass steam engines are another area of development that is growing in popularity. Biomass, which includes organic materials like wood, agricultural waste, and even algae, can be burned to produce steam. The combustion of biomass releases carbon dioxide, but because the carbon dioxide emitted is offset by the carbon absorbed by the plants during their growth, the overall carbon impact can be much lower than that of fossil fuels. Biomass steam engines can play a significant role in reducing the carbon footprint of industries that require large amounts of heat and power, especially in regions where biomass resources are abundant.
Steam engines are also benefitting from the incorporation of smart technologies and advanced automation systems. Modern sensors, controllers, and computer systems allow for real-time monitoring and adjustment of engine performance. These smart systems can optimize steam flow, pressure, and temperature to ensure that the engine is always operating at peak efficiency. Moreover, predictive maintenance systems, which use machine learning and AI to analyze performance data and predict when maintenance is needed, can help reduce downtime and lower operational costs.
Furthermore, energy storage systems are becoming increasingly important in optimizing steam engine performance, especially in hybrid setups. Combining steam engines with battery storage or other forms of energy storage allows for the capture of excess energy when the engine is producing more power than needed. This stored energy can then be used during times when energy demand exceeds production, ensuring a stable and continuous supply of power. The integration of energy storage also enhances the feasibility of using steam engines in off-grid or remote locations where traditional energy infrastructure is unavailable.
In terms of industrial applications, steam engines are seeing renewed interest in microgrid systems. Microgrids are localized networks of energy generation and distribution that can operate independently from the main power grid. They are particularly useful in remote areas, industrial complexes, or critical infrastructure where reliability and resilience are essential. Steam engines, particularly those powered by renewable sources like biomass or solar, are ideal candidates for inclusion in microgrids because they can provide a stable and reliable power source that can operate autonomously.
Steam engines can also play a key role in district heating systems. These systems, which provide heat to multiple buildings or even entire neighborhoods, can be powered by steam engines running on renewable fuels. Instead of relying on individual heating units in each building, district heating systems distribute heat from a central source, which can reduce energy consumption and increase efficiency. When powered by sustainable energy sources, these systems can significantly lower the carbon emissions associated with heating.
As steam engine technology advances, modular designs are becoming more common. These modular systems are flexible and scalable, making them suitable for a wide range of applications. Whether used in small-scale residential setups, large industrial complexes, or as part of renewable energy systems, modular steam engines can be customized to meet specific energy needs. This adaptability is a key advantage, as it allows steam engines to be deployed in diverse settings and applications without the need for completely new designs.
Additionally, the push toward sustainability is driving the development of more efficient steam cycles. New cycle designs, such as the Rankine cycle, have been optimized over the years to extract more energy from steam while reducing waste heat. Advanced superheating and regenerative heating techniques are being incorporated into modern steam engines to increase efficiency further. By preheating the incoming steam or using superheated steam at higher temperatures, engineers can generate more mechanical energy from the same amount of fuel, resulting in lower fuel consumption and reduced environmental impact.
One of the significant challenges facing the future of steam engine technology is the need for cost-effective manufacturing and widespread adoption. While steam engines have existed for centuries, many of the latest innovations require significant investment in research and development, as well as new manufacturing techniques. As these technologies mature, however, economies of scale will likely reduce the cost of production, making steam engines a more accessible option for a wider range of industries and applications.
The transition to renewable energy sources and the increasing focus on reducing greenhouse gas emissions will continue to drive innovation in steam engine technology. Whether through the use of alternative fuels like hydrogen and biomass, the integration of solar thermal energy, or the application of advanced automation and energy storage systems, steam engines are likely to remain an important part of the global energy landscape. The continued evolution of steam engine design holds promise for a future where these machines contribute to sustainable power generation, reduce environmental impacts, and provide reliable energy solutions for industries and homes alike.
Eco-Friendly Steam Engine Solutions
Eco-friendly steam engine solutions are becoming increasingly relevant as the world focuses on sustainable energy and reducing environmental impacts. Steam engines, traditionally powered by coal or other fossil fuels, are now being reimagined with a focus on renewable and low-emission energy sources. Innovations in steam engine technology, along with the integration of cleaner fuels and energy recovery systems, are helping create eco-friendly solutions that contribute to reducing the carbon footprint while maintaining the power generation capabilities of steam engines.
A key element in developing eco-friendly steam engine solutions is shifting the fuel sources used to generate steam. While coal and oil have been the dominant fuels historically, there are now several alternative fuel sources that can power steam engines in a more environmentally responsible way.
- Biomass as a Fuel Source:
Biomass, which includes organic materials like wood, agricultural residues, and even algae, can be used to generate steam. Biomass combustion releases carbon dioxide, but it is considered more eco-friendly than fossil fuels because the carbon dioxide released during combustion is offset by the carbon absorbed by the plants as they grow. Biomass steam engines, therefore, help maintain a more balanced carbon cycle and are a renewable energy source that can reduce reliance on fossil fuels. Biomass is particularly attractive for use in regions where there is an abundance of agricultural waste or forest residues. - Solar Thermal Steam Generation:
Solar energy can also be harnessed to generate steam. Solar thermal systems use mirrors or lenses to concentrate sunlight, generating heat that is then transferred to water to create steam. This steam can then power a steam engine to generate electricity or drive mechanical processes. Solar thermal systems are particularly useful in areas with high sun exposure, making them a viable option for renewable energy in many parts of the world. The integration of solar energy with steam engines offers a sustainable solution for power generation, reducing dependence on fossil fuels while utilizing the abundant energy of the sun. - Hydrogen-Powered Steam Engines:
Hydrogen, when used as a fuel, produces only water vapor as a byproduct, making it a clean alternative to traditional fossil fuels. Hydrogen-powered steam engines are an emerging solution for reducing emissions in industrial processes. These engines operate similarly to conventional steam engines but burn hydrogen to generate steam. Hydrogen is a renewable energy carrier, and when produced using green energy sources (e.g., through electrolysis powered by wind or solar), it can provide a zero-emission solution for steam engine applications. The challenge with hydrogen-powered steam engines lies in the infrastructure needed for hydrogen production, storage, and distribution, but advancements in these areas are helping bring this solution closer to reality. - Waste Heat Recovery Systems:
Another eco-friendly aspect of steam engine technology is the use of waste heat recovery systems. In industrial settings, steam engines often produce excess heat that is not fully utilized in the steam generation process. By capturing and reusing this excess heat, steam engines can operate more efficiently, reducing the need for additional fuel and minimizing overall emissions. Heat recovery systems, such as regenerators and heat exchangers, can capture exhaust heat and reuse it to preheat water or steam, improving the engine’s efficiency and reducing fuel consumption. This approach helps lower the environmental impact of steam engines by maximizing energy utilization and minimizing waste. - Closed-Loop Steam Systems:
In traditional steam engines, steam is often vented after it has done its work, releasing energy into the atmosphere. However, more modern steam engines incorporate closed-loop systems, where the exhaust steam is cooled, condensed back into water, and reused in the boiler. This process significantly reduces water consumption and increases the overall energy efficiency of the system. The reduction in water waste is particularly important in regions where water is a scarce resource. By capturing and recycling steam, closed-loop systems also minimize heat loss and emissions, making steam engines more eco-friendly. - Combined Heat and Power (CHP) Systems:
Combined Heat and Power (CHP) systems, also known as cogeneration systems, are an efficient way to utilize steam engines for both power generation and heating. In a CHP system, the steam engine generates electricity while also providing heat for industrial processes or district heating. This dual-purpose approach maximizes the energy output of the steam engine, reducing fuel consumption and improving overall efficiency. CHP systems can significantly reduce greenhouse gas emissions, particularly when powered by renewable fuels or when integrated with heat recovery systems. - Smart Technologies and Automation:
Integrating smart technologies into steam engine systems can also contribute to their eco-friendliness. Modern steam engines are equipped with sensors, controls, and automation systems that optimize engine performance in real time. These systems can adjust steam flow, pressure, and temperature to ensure that the engine is operating at its most efficient level, reducing fuel consumption and minimizing emissions. Additionally, predictive maintenance systems can monitor the health of steam engine components and identify potential issues before they lead to energy losses or equipment failure, further improving efficiency and reducing environmental impact. - Sustainability Certifications and Standards:
As the demand for eco-friendly technologies grows, there is increasing pressure on manufacturers to meet sustainability standards and certifications. Steam engine manufacturers are adopting green standards and certifications to ensure that their products meet environmental and sustainability goals. These certifications may focus on emissions reductions, energy efficiency, and the use of renewable materials. By adhering to these standards, steam engine manufacturers can offer more environmentally responsible solutions to industries and end-users who are committed to reducing their carbon footprint.
By adopting these eco-friendly steam engine solutions, industries and power generation facilities can reduce their environmental impact, improve operational efficiency, and move toward a more sustainable energy future. The shift from fossil fuels to renewable energy sources, coupled with advances in steam engine design and technology, has the potential to make steam engines a critical component of the global transition to clean energy. With continuous innovation, steam engines will continue to evolve, contributing to the decarbonization of energy systems and supporting a more sustainable world.
The transition to eco-friendly steam engine solutions is transforming the way industries generate and utilize power. By adopting sustainable practices, these systems are helping to reduce environmental impact while maintaining or even improving their energy efficiency. A key part of this transformation is the integration of alternative fuel sources that help lower the carbon footprint of steam engines. Biomass, for instance, offers a renewable and carbon-neutral fuel source. The burning of organic material like wood or agricultural waste to generate steam produces lower emissions than fossil fuels, making it an effective solution for industrial heating and power generation.
In addition to biomass, the potential for solar thermal steam generation continues to gain traction. Using concentrated solar energy to produce heat and then convert it into steam is a promising way to harness abundant natural resources, especially in regions with high solar exposure. This type of energy generation minimizes the environmental impact, providing a clean, renewable power source. When combined with steam engines, solar thermal systems can help decarbonize power generation by replacing fossil fuels with sunlight.
Hydrogen-powered steam engines are another innovative solution, driven by the need for cleaner energy sources. Hydrogen, as a fuel, burns cleanly, producing only water vapor as a byproduct. When used in steam engines, hydrogen can provide an emission-free alternative to conventional fuels. The challenge lies in scaling up hydrogen production, storage, and distribution infrastructure, but as these systems develop, hydrogen-powered steam engines have the potential to play a significant role in reducing industrial emissions, particularly when produced from renewable sources like wind and solar energy.
Another key strategy in making steam engines more eco-friendly is the development of waste heat recovery systems. Industrial processes often generate excess heat that can be captured and reused in steam engines to generate power. This type of heat recovery reduces the need for additional fuel input, improving energy efficiency while reducing emissions. By recycling exhaust heat, steam engines can run more efficiently, requiring less fuel to produce the same amount of work. This principle is applied not only in power plants but also in industrial settings, where minimizing waste is critical for both operational efficiency and environmental sustainability.
The adoption of closed-loop steam systems is also contributing to the reduction of water consumption and heat loss in steam engines. In traditional systems, once the steam has performed its work, it is released into the atmosphere. In contrast, modern closed-loop systems capture and condense the exhaust steam, returning it to the boiler for reuse. This process reduces water consumption, which is crucial in areas where water is a scarce resource. It also minimizes energy waste, contributing to better efficiency and lower environmental impact.
The integration of combined heat and power (CHP) systems is helping industries maximize the utility of steam engines. By simultaneously generating electricity and providing heat for industrial processes or district heating, CHP systems improve energy efficiency and reduce the overall environmental impact. These systems help decrease the need for separate heating units, thus reducing energy consumption and associated emissions. Furthermore, when powered by renewable fuels such as biomass or hydrogen, CHP systems become even more eco-friendly by contributing to both power generation and thermal energy in an environmentally responsible manner.
Incorporating smart technologies into steam engines enhances their performance and sustainability. Automation systems, real-time monitoring, and control algorithms allow for precise adjustments in steam flow, pressure, and temperature, optimizing energy use and preventing waste. Predictive maintenance systems further improve efficiency by identifying potential issues before they result in equipment failure or energy loss. These advanced technologies not only increase the lifespan of steam engine components but also ensure that engines are operating at their highest possible efficiency.
As sustainability becomes a driving force in manufacturing and energy generation, steam engine manufacturers are increasingly adopting green certifications and environmental standards. These certifications ensure that the steam engines meet stringent emissions, energy efficiency, and sustainability criteria. By adhering to these standards, manufacturers are producing engines that have lower environmental impacts, helping industries meet their own sustainability goals.
The eco-friendly revolution in steam engine design also extends to the materials used in construction. Advances in materials science are helping to improve the durability, heat resistance, and efficiency of steam engine components. Lightweight, high-performance alloys and composite materials are now being used in pistons, cylinders, and boilers, allowing steam engines to run at higher pressures and temperatures without compromising safety or efficiency. These material innovations make steam engines more durable, longer-lasting, and less prone to wear and tear, ultimately reducing the need for replacements and minimizing the environmental impact associated with manufacturing and disposal.
The shift toward eco-friendly steam engines also supports the broader trend of decentralizing energy production through microgrids. These local energy networks are designed to operate independently from the central grid, and steam engines powered by renewable sources can play an essential role in providing reliable and sustainable energy to remote or off-grid communities. By incorporating renewable energy sources into these microgrids, steam engines can contribute to energy resilience in regions where access to conventional grid power is limited or unreliable.
In the future, steam engines will continue to evolve, contributing to a cleaner, greener energy landscape. Their ability to operate on renewable fuels, combined with cutting-edge technologies like smart automation, waste heat recovery, and CHP systems, makes them a sustainable option for industries worldwide. By focusing on energy efficiency, low emissions, and innovative fuel sources, steam engines will play a vital role in helping meet global energy demands while minimizing environmental impacts. With ongoing research and technological advancements, steam engines are poised to remain a key player in the transition to a more sustainable energy future.
As the world continues to transition towards cleaner and more sustainable energy solutions, the role of steam engines in various sectors is evolving rapidly. The incorporation of steam engines into industrial decarbonization strategies is gaining significant momentum. Industries that rely heavily on high-temperature heat processes, such as cement production, steel manufacturing, and chemical production, are exploring how steam engines can be utilized in combination with renewable energy sources to replace the need for traditional fossil-fuel-based heating systems.
One of the most significant developments in this area is the concept of hybrid energy systems, which combine steam engines with renewable power sources like solar, wind, and biomass. These systems offer the ability to generate electricity while also providing heat for industrial processes. For example, a steam engine powered by solar thermal energy could generate both power and heat for industrial facilities, reducing reliance on fossil fuels and enabling industries to meet their carbon reduction targets more efficiently.
Energy storage solutions also complement steam engine technology in hybrid systems. Energy storage, whether through batteries, pumped hydro storage, or thermal storage, allows for energy generated by renewable sources to be stored and used during periods of high demand or low generation. This storage capacity helps ensure that steam engines can run efficiently and consistently, regardless of fluctuations in the availability of renewable energy. For instance, excess energy from solar panels during sunny days could be stored and used to generate steam at night or during cloudy weather, ensuring a continuous and stable power supply.
Moreover, the development of low-carbon fuels, such as biofuels, green hydrogen, and synthetic fuels, offers further promise for steam engine applications. Biofuels, derived from plant and animal matter, provide a renewable alternative to fossil fuels. They can be used to power steam engines with a lower carbon footprint than traditional fuels. Green hydrogen, produced through electrolysis powered by renewable energy sources, can be another sustainable fuel option. When burned in steam engines, green hydrogen releases no greenhouse gases, making it an ideal fuel for eco-conscious industries. Similarly, synthetic fuels produced from captured carbon dioxide and renewable energy can power steam engines while contributing to efforts to reduce atmospheric CO2 levels.
At the same time, circular economy principles are gaining traction in steam engine manufacturing. In the circular economy, the focus is on reducing waste and maximizing the reuse and recycling of materials. Steam engine manufacturers are increasingly exploring ways to design engines with longer lifespans, using more durable and recyclable materials. This approach reduces the environmental impact of production and disposal, aligning with global efforts to reduce waste and encourage resource efficiency. By designing engines that can be easily disassembled and recycled at the end of their life cycle, manufacturers can contribute to reducing landfill waste and reducing the need for raw material extraction.
In parallel, advancements in carbon capture and storage (CCS) technology could make steam engines even more environmentally friendly. By capturing the carbon emissions produced by burning fossil fuels or biofuels in steam engines, and then storing them underground or using them for other industrial processes, CCS can further reduce the carbon footprint of steam engines. Though still in the early stages of commercialization, CCS has the potential to make fossil-fuel-powered steam engines more viable in a low-carbon economy by reducing their contribution to global warming.
Another important factor influencing the future of steam engines is the integration of digital technologies. The rise of Internet of Things (IoT) devices, big data analytics, and artificial intelligence (AI) is revolutionizing how steam engines are monitored, controlled, and optimized. IoT sensors can continuously monitor the performance of various steam engine components, providing real-time data on parameters such as pressure, temperature, and steam flow. This data can be analyzed to optimize performance, reduce energy consumption, and extend the lifespan of the engine. AI-powered predictive maintenance tools can detect signs of wear or malfunction before they result in costly repairs or downtime, ensuring that steam engines operate at their highest efficiency.
With the global shift towards decarbonizing transportation, steam engines are also finding new applications in sectors like marine transport. Shipping companies are exploring how steam engines can be powered by renewable fuels such as biofuels or ammonia, offering a potential solution for reducing emissions from maritime vessels. The versatility of steam engines, combined with renewable fuel sources, could provide a cleaner alternative to conventional diesel-powered ships, which are significant contributors to global greenhouse gas emissions.
In terms of urban energy systems, steam engines are increasingly seen as a viable option for district heating systems. These systems, which provide heat to entire neighborhoods or districts, can benefit from steam engine technology, especially when combined with renewable energy sources. Steam engines powered by waste heat, biomass, or solar thermal energy can provide efficient heating solutions for urban areas while helping to reduce the carbon emissions associated with traditional heating methods, such as natural gas or coal. District heating systems powered by renewable steam engines can also support more energy-efficient, low-carbon communities, contributing to the decarbonization of urban infrastructure.
The continued evolution of steam engines is not only about improving their efficiency or reducing their carbon footprint but also about making them more adaptable to different energy demands. As industries increasingly turn to steam engines to power their operations, there is a growing need for flexible, modular steam engine designs that can be easily adjusted to meet specific power and heat requirements. Modular systems allow steam engines to be scaled up or down to suit the size and complexity of different applications, from small-scale residential systems to large industrial plants.
The future of steam engine technology holds great promise in supporting the global transition to renewable and sustainable energy systems. With advancements in fuel technology, hybrid systems, waste heat recovery, and digital optimization, steam engines are poised to remain an important tool in reducing carbon emissions and increasing energy efficiency across industries. As the technology continues to evolve, steam engines will play a key role in helping industries, power plants, and even individual homes meet the growing demand for clean, reliable, and efficient energy in a rapidly changing world.
Steam Engine Integration into Power Plants
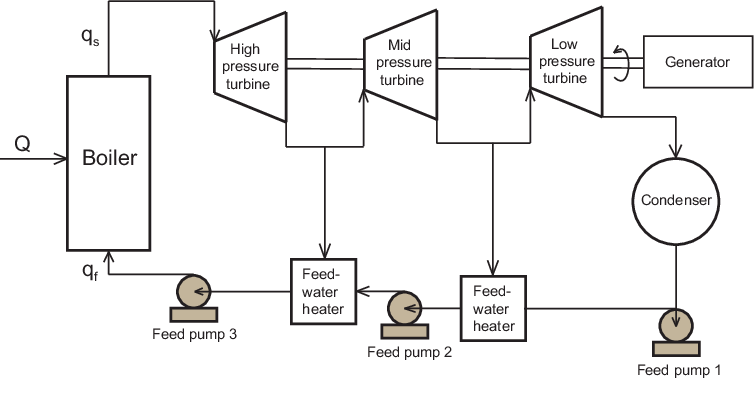
The integration of steam engines into power plants is a fundamental aspect of modern energy production, particularly in settings where high efficiency and sustainability are priorities. Steam engines have long been a staple in the energy sector, primarily used in the form of steam turbines to generate electricity. These turbines convert thermal energy into mechanical energy by expanding steam under high pressure, which then drives a generator to produce electrical power. While traditional steam turbines in power plants often run on fossil fuels like coal, natural gas, and oil, the industry is undergoing a significant transformation, moving towards cleaner, more sustainable energy sources.
Modern Power Plant Configurations and Steam Engines
The role of steam engines in power plants is typically centered around their ability to efficiently convert heat into electricity. The configuration of a modern power plant usually involves a combination of steam generation, steam turbines, and condensers in a highly integrated system. Here’s a closer look at how steam engines fit into these configurations:
- Fossil-Fuel Power Plants (Traditional Steam Turbines): In fossil-fuel-based power plants, steam is generated by burning coal, natural gas, or oil to produce high-pressure steam in a boiler. The steam is then directed to a steam turbine, where it expands and drives a generator to produce electricity. After passing through the turbine, the steam is condensed back into water in a condenser and returned to the boiler for reuse in a closed-loop system. Modern coal and natural gas plants can operate at higher efficiencies by utilizing advanced steam turbines, which operate at higher pressures and temperatures. These plants typically use a Rankine cycle, a thermodynamic cycle that maximizes the conversion of heat into useful work by utilizing steam. The integration of combined cycle systems (combining gas and steam turbines) further increases efficiency in modern power plants. In a combined cycle, a gas turbine generates electricity using high-temperature exhaust gases, which are then used to produce steam for a steam turbine. This allows the plant to extract more energy from the same amount of fuel, significantly improving overall efficiency.
- Renewable and Low-Carbon Steam Generation: As the world moves toward renewable energy sources, the integration of steam engines into power plants powered by biomass, solar thermal energy, and geothermal energy is gaining momentum.
- Biomass Power Plants: Biomass-based power plants use organic material like wood, agricultural residues, and dedicated energy crops to produce steam. The steam then drives a steam turbine to generate electricity. These power plants function similarly to fossil-fuel plants but with the advantage of utilizing renewable resources. Biomass steam engines can play a role in reducing carbon emissions, as the carbon released during combustion is offset by the carbon absorbed during the growth of the biomass.
- Solar Thermal Power Plants: Solar thermal power plants harness the sun’s energy by using mirrors or lenses to concentrate sunlight and produce heat. This heat is transferred to a fluid, which is used to generate steam that powers a steam turbine. Solar thermal power plants can provide a consistent source of energy by storing thermal energy in large thermal storage tanks, allowing steam turbines to continue generating electricity even when the sun is not shining.
- Geothermal Power Plants: Geothermal energy, derived from the heat of the Earth’s core, is another renewable energy source used to generate steam for power plants. In geothermal power plants, steam is extracted from underground reservoirs of hot water or steam and used to drive a steam turbine. These plants are highly sustainable, as they utilize the Earth’s natural heat, which is continuously replenished.
- Cogeneration and Combined Heat and Power (CHP): The concept of cogeneration (or combined heat and power, CHP) integrates the steam engine technology in power plants to simultaneously produce both electricity and usable heat. In industrial settings, this approach is highly efficient because the heat generated during the steam production process, which would normally be wasted, is instead captured and used for heating purposes. This could be for heating water in district heating systems or for industrial processes like chemical production, refining, or manufacturing. Cogeneration systems are typically integrated with steam turbines, which help extract thermal energy and convert it into mechanical energy for power generation. These systems reduce the need for additional fuel inputs and are particularly useful for industries with both heating and power needs. In the context of power plants, CHP systems contribute to a more sustainable energy model by maximizing energy utilization and minimizing waste.
- Waste Heat Recovery Systems: Modern power plants often incorporate waste heat recovery systems that utilize steam engines to capture and reuse exhaust heat from gas turbines, combustion engines, or industrial processes. This recovered heat is then used to produce steam, which drives a steam turbine for additional power generation. These systems are an integral part of combined cycle plants, as they increase overall plant efficiency by converting heat that would otherwise be lost into usable energy. Waste heat recovery systems are particularly effective when integrated with gas turbines and other high-temperature systems that produce large amounts of exhaust heat.
- Nuclear Power Plants: Nuclear power plants are another example of steam engine integration in power generation. In nuclear reactors, uranium or other nuclear materials undergo a controlled reaction that generates heat. This heat is used to produce steam, which drives a steam turbine to generate electricity. While nuclear power plants rely on nuclear fission rather than combustion, the basic principles of steam generation and turbine operation are similar to those used in traditional fossil-fuel power plants. The advantage of nuclear power is its ability to provide large-scale, low-carbon energy, though concerns around safety, waste disposal, and public perception remain challenges to widespread adoption.
Challenges and Opportunities in Integrating Steam Engines into Power Plants
Despite the many benefits, integrating steam engines, especially those powered by renewable or low-carbon sources, into power plants poses several challenges:
- Efficiency: One of the primary advantages of steam turbines in power plants is their ability to operate efficiently, particularly in high-pressure, high-temperature environments. However, achieving optimal efficiency in steam generation, especially with renewable energy sources, requires advanced technologies, such as heat recovery systems, thermal storage, and high-performance materials. The integration of these systems requires significant upfront investment in infrastructure and R&D.
- Fuel Availability: For renewable and low-carbon steam generation, such as biomass and solar thermal systems, the availability of fuel can be a limiting factor. Biomass plants, for instance, require a consistent and sustainable supply of organic materials. Solar thermal plants are dependent on sunlight, which can vary by location and time of day. Overcoming these challenges involves careful planning of fuel supply chains, geographic location analysis, and the use of complementary energy storage systems.
- Infrastructure and Integration: The integration of steam engines into power plants, especially those relying on renewable energy, often requires the modification of existing infrastructure. For example, retrofitting a fossil-fuel-based power plant to accommodate renewable steam generation might involve significant redesigns of boilers, turbines, and steam handling systems. Additionally, the transition to renewable energy sources requires investments in grid infrastructure to ensure that power can be delivered reliably and efficiently to consumers.
- Regulatory and Environmental Considerations: Power plants using steam engines must comply with a variety of environmental regulations regarding emissions, waste management, and water usage. While renewable and low-carbon steam generation systems generally have a smaller environmental footprint, careful monitoring and management are still necessary to minimize the impact on the local environment.
Despite these challenges, the potential for steam engines to be integrated into diverse power generation systems is vast. With advancements in technology, fuel sources, and energy storage systems, steam engines will continue to play a crucial role in the global shift toward sustainable and reliable energy production. Whether through the use of biomass, solar thermal energy, or cogeneration, steam engines remain a versatile and essential component of modern power plants, contributing to a more sustainable and energy-efficient future.
The integration of steam engines into power plants is rapidly evolving, especially as industries and governments seek to meet growing energy demands while reducing their carbon footprints. The global push for cleaner energy sources has resulted in the development of advanced steam engine technologies that leverage renewable energy and low-emission fuels. Power plants incorporating steam engines are now increasingly designed with efficiency, sustainability, and energy security in mind.
One key aspect of this transition is the shift toward renewable energy sources. The focus has expanded from traditional fossil fuels to harnessing natural resources such as the sun, wind, and geothermal heat. These renewable sources, paired with steam engine technology, offer great promise in providing low-carbon alternatives to conventional power generation. By utilizing renewable heat sources like solar thermal systems, power plants can generate steam without the need for carbon-intensive fuels. The integration of steam engines in solar thermal plants helps to harness concentrated sunlight and convert it into usable thermal energy. This process can produce consistent power generation, even during cloudy days or at night, by storing heat in thermal storage systems.
Similarly, geothermal energy offers a sustainable method of generating steam through the Earth’s natural heat. Geothermal plants, using steam extracted from underground reservoirs, drive steam turbines to produce electricity. This form of energy is largely unaffected by weather conditions, offering a stable, long-term energy source that reduces dependency on fossil fuels. In regions with abundant geothermal resources, these systems can significantly contribute to energy supply while minimizing environmental impact.
Waste heat recovery is another area where steam engines are proving invaluable. Many industrial processes and power plants produce significant amounts of excess heat, which is often vented into the atmosphere. By integrating steam engines into these systems, this wasted heat can be captured and converted into additional electricity. This process not only improves overall energy efficiency but also reduces the need for extra fuel consumption, thereby decreasing emissions and operational costs. In industries such as cement production, steel manufacturing, and chemical processing, waste heat recovery systems powered by steam engines are becoming increasingly common, providing substantial savings in both energy and environmental impact.
Moreover, hybrid energy systems are being explored, where steam engines work in combination with other technologies like gas turbines or combined cycle systems. This hybrid approach optimizes energy production by allowing plants to use both thermal energy and electricity from different sources, ensuring that power generation is both reliable and efficient. In some cases, a combination of steam engines and gas turbines allows for combined heat and power (CHP) systems, which deliver both electricity and useful thermal energy for industrial processes or district heating systems. This approach maximizes the use of fuel by capturing the exhaust heat from gas turbines and using it to generate steam for additional power, resulting in a highly efficient energy system.
As carbon capture and storage (CCS) technologies advance, steam engines will also play a role in enabling the capture and reduction of emissions from fossil-fuel-based plants. CCS systems capture carbon dioxide (CO2) emissions from the combustion of fossil fuels before they are released into the atmosphere. Once captured, CO2 can either be stored underground or repurposed for industrial uses such as in the production of synthetic fuels. For steam-powered plants, the integration of CCS can significantly reduce the carbon footprint, making it easier for these plants to meet stricter emission regulations while continuing to provide electricity.
Another growing area of interest is the integration of smart grid technologies into power plants using steam engines. Smart grids enable more efficient and flexible energy distribution, allowing for real-time monitoring and management of electricity demand and supply. By integrating steam engines with smart grid systems, power plants can better match production to demand, optimize energy use, and reduce waste. This integration is especially important as power generation becomes more decentralized, with local and renewable sources becoming increasingly common. Steam engines equipped with IoT sensors can help ensure that these systems operate efficiently by providing real-time data on performance metrics such as steam flow, pressure, and temperature, which can then be analyzed for optimization.
The ability of steam engines to provide reliable and continuous energy, especially when combined with energy storage systems, makes them a key component in maintaining grid stability. For example, thermal energy storage allows steam-powered plants to store excess heat during periods of low demand and release it when demand spikes. This energy storage capability is crucial for balancing supply and demand, especially as the energy grid becomes more dependent on intermittent renewable sources such as wind and solar. Steam engines, in combination with energy storage technologies, help ensure that energy is available when needed, supporting the overall resilience of the grid.
The flexibility of steam engines also extends to their application in microgrids, which are smaller, localized energy systems that can operate independently of the main grid. Microgrids, often powered by renewable sources such as solar, wind, and biomass, can integrate steam engines for power generation and thermal applications. By incorporating steam engines, microgrids can provide reliable, off-grid energy solutions for remote areas or critical infrastructure, ensuring energy security in regions that may be vulnerable to power outages.
In terms of economic impact, the integration of steam engines into power plants can lead to long-term cost savings for both utilities and consumers. Although the initial investment in advanced steam engine systems and renewable energy technologies can be significant, the long-term operational savings through higher efficiency, reduced fuel costs, and lower maintenance needs can be substantial. Furthermore, the transition to renewable-powered steam engines can help companies and governments meet climate targets, qualify for green incentives, and build resilience against rising energy costs in the future.
The future of steam engine integration in power plants is also closely tied to the development of next-generation materials and technology. For instance, advances in high-temperature alloys and superalloys can enable steam engines to operate at higher temperatures and pressures, further improving efficiency and expanding the range of renewable fuels that can be used. The use of advanced sensors, AI algorithms, and machine learning for predictive maintenance and real-time optimization will continue to drive the operational efficiency of steam-powered plants, ensuring that these systems operate at peak performance while reducing downtime and costly repairs.
In summary, the integration of steam engines into power plants is evolving from a traditional energy generation model to a more sustainable, efficient, and flexible approach. By harnessing renewable energy sources, improving efficiency through advanced technologies, and incorporating waste heat recovery and energy storage systems, steam engines are playing an essential role in the transformation of the global energy landscape. As industries, governments, and power producers strive for cleaner and more efficient energy solutions, steam engines will remain a cornerstone of power generation, offering a proven, adaptable, and increasingly eco-friendly technology for the future.
The evolution of steam engine integration into power plants continues to be shaped by advancements in technology and global energy demands. As the push for sustainable energy grows, industries are focusing on creating systems that not only provide reliable power but also contribute to the decarbonization of the energy sector. The integration of steam engines into these modern power plants is an integral part of this process, offering both flexibility and sustainability in how we generate and distribute energy.
One of the significant advantages of steam engines in power plants is their scalability. Unlike some other forms of power generation, steam engines can be scaled up or down depending on the size and needs of the facility. This versatility allows for a range of applications, from small-scale plants that serve isolated communities or industrial operations to large-scale facilities that contribute to national grids. This scalability is increasingly important as decentralized energy systems become more common, allowing communities to generate and consume energy locally while reducing transmission losses and improving energy security.
In the context of distributed energy generation, steam engines also lend themselves well to microgrid systems. Microgrids, powered by local renewable sources like wind, solar, or biomass, can benefit from the ability of steam engines to provide not just electricity but also heating solutions for industrial processes and residential buildings. This approach supports the creation of energy-autonomous regions, contributing to both energy resilience and sustainability. Steam engines can complement these microgrids by providing reliable power during periods of low renewable generation or when energy demand spikes.
Another key area of focus in steam engine integration is the growing importance of energy storage solutions. Steam engines powered by renewable sources like solar thermal or biomass often require energy storage systems to mitigate fluctuations in energy production. For example, during sunny days, a solar thermal plant may produce more energy than is needed, so excess heat can be stored in thermal storage systems for later use. When combined with steam engines, these energy storage solutions help to smooth out the intermittency of renewable power, ensuring a continuous and reliable energy supply. Energy storage can also play a role in stabilizing grid systems that incorporate both renewable and conventional sources, reducing the reliance on fossil fuels during peak demand periods.
As countries increasingly implement carbon pricing mechanisms, emission reduction targets, and stricter regulations on environmental impact, the role of steam engines in power plants as carbon-neutral or low-carbon solutions will continue to grow. A key driver in this transition is the development and use of green hydrogen. Green hydrogen is produced by using renewable energy to split water into hydrogen and oxygen via electrolysis, and this hydrogen can be used as a fuel to generate steam. When burned in steam engines, green hydrogen produces only water vapor, making it one of the most promising fuels for decarbonizing energy systems. Integrating steam engines with green hydrogen systems presents a compelling solution for industries looking to meet net-zero emissions goals.
In addition to green hydrogen, biofuels continue to be a promising alternative fuel for steam engines. Derived from organic materials such as plant matter or algae, biofuels offer a renewable energy source that can significantly reduce the carbon footprint of power plants. As the technology for producing and refining biofuels improves, it will become increasingly cost-effective to replace traditional fossil fuels with these cleaner alternatives. For steam engines in power plants, biofuels provide a bridge between fully renewable energy sources and the infrastructure already in place for conventional steam generation. This transition can happen without requiring extensive retrofitting, making biofuels an attractive solution for reducing carbon emissions in existing power plants.
The continued development of advanced materials for steam engines is another critical factor in the integration of steam engine technology into modern power plants. High-efficiency steam engines require materials capable of withstanding extremely high temperatures and pressures, and as these materials improve, steam engines can operate at even higher efficiencies. New developments in superalloys, ceramic materials, and composite materials allow steam turbines and other components to function at higher operating conditions, increasing the overall energy efficiency of the system. These advancements will help power plants reduce fuel consumption and improve the economic viability of renewable steam generation technologies.
As smart technologies continue to revolutionize industries, the integration of digitalization and IoT-based monitoring systems in steam-powered power plants is becoming increasingly common. Data-driven analytics are used to optimize the performance of steam engines by monitoring real-time data on turbine performance, steam flow, temperature, and pressure. These systems enable predictive maintenance, identifying potential issues before they cause system failures, reducing downtime, and improving the lifespan of critical equipment. Additionally, artificial intelligence (AI) and machine learning algorithms can be applied to streamline plant operations by improving steam generation processes, optimizing fuel usage, and minimizing emissions.
With global energy demand expected to rise steadily, steam engine technology in power plants will play a crucial role in providing energy for various sectors, from residential electricity generation to heavy industries. The flexibility, scalability, and adaptability of steam engines make them an essential component of any sustainable energy strategy. By integrating renewable energy sources, energy storage, hybrid systems, and advanced digital technologies, steam engine systems can provide reliable, affordable, and environmentally responsible power.
One of the emerging fields that could shape the future of steam engine technology in power plants is nuclear fusion. While fusion power is still in the experimental stages, its potential to provide virtually unlimited and clean energy makes it an exciting prospect for steam engine applications. If nuclear fusion reactors become commercially viable, they could generate vast amounts of heat to drive steam engines in power plants, potentially revolutionizing energy production and further reducing reliance on fossil fuels. Steam engines powered by fusion energy could deliver significant contributions to global decarbonization goals by providing clean, baseload power to the grid.
Furthermore, the integration of steam engine systems into district heating networks is expected to grow in the coming years. These systems use steam engines to generate heat, which is then distributed to homes and businesses within a specific area. By utilizing waste heat or renewable energy to generate steam, district heating systems powered by steam engines can help communities reduce their reliance on natural gas or oil for heating. This is particularly important for urban areas, where heating is a major contributor to energy consumption. By coupling steam engines with renewable energy systems like geothermal, biomass, or solar thermal, district heating networks can become an even more sustainable solution for cities looking to reduce their carbon emissions.
The ongoing innovation and development of steam engine technology continue to drive its potential for sustainable energy generation. As energy systems become more complex, decentralized, and reliant on renewable sources, steam engines will remain a central technology for integrating these resources into existing power plants and ensuring that they contribute to a cleaner, more efficient energy future. Whether through the adoption of new fuels, hybrid systems, advanced materials, or digital monitoring technologies, steam engines are poised to play a key role in the global energy transition. Their adaptability, scalability, and potential for integration with renewable energy sources position them as a reliable and important technology for meeting the world’s future energy needs.
Commercial Steam Engine Sales for Large Projects
Commercial steam engine sales for large projects require a thorough understanding of both the specific needs of the project and the capabilities of steam engine technology. These large-scale projects may include power plants, industrial processes, district heating systems, or renewable energy installations, all of which need custom steam engine solutions that align with the unique demands of each application. In these settings, steam engines must provide high levels of reliability, efficiency, and scalability, while adhering to environmental and economic standards.
Key Considerations for Commercial Steam Engine Sales:
- Application and Industry-Specific Needs: Large projects demand steam engines that are tailored to the specific requirements of the industry. For example:
- Power Generation: Steam engines for power plants must handle large volumes of steam, operate at high pressures and temperatures, and integrate with other systems like turbines and boilers. These engines must also be adaptable to renewable sources such as geothermal, biomass, or solar thermal.
- Industrial Applications: Industries like chemical processing, cement production, paper mills, or refineries require steam engines to power machinery, provide heating, or support other process applications. Steam engines in such settings often operate in high-demand, high-temperature environments.
- District Heating: In urban or industrial settings, steam engines can provide heat for district heating networks, supporting heating needs for residential, commercial, and industrial facilities.
- Size and Scalability: The scale of the project significantly impacts the type of steam engine required. For large projects, steam engines must be designed to provide consistent power generation while maintaining scalability. This could mean purchasing large steam engines with substantial output capacities or configuring a series of smaller units that can be modularly expanded to meet future demand.
- Fuel Type and Sustainability: Choosing the appropriate fuel type for a steam engine is crucial for optimizing performance and meeting sustainability goals. The fuel used can include traditional fossil fuels, renewable sources like biomass or biogas, or even green technologies such as green hydrogen. Commercial projects focused on reducing their carbon footprint may prefer engines designed to run on cleaner fuels or integrate with waste heat recovery systems to maximize efficiency.
- Customization and Engineering Design: Custom steam engines are often needed for large projects to ensure they meet specific design and operational requirements. Customization might include modifications to:
- Heat exchangers for better heat retention or transfer
- Control systems for real-time monitoring and automation
- Boiler pressure specifications to optimize energy production and meet regulatory standards
- Noise reduction and other safety features
- Durability and maintenance schedules suited for the project’s lifespan
- Energy Efficiency and Performance: Energy efficiency is a top priority in large-scale steam engine sales, as it directly affects operational costs and environmental impact. Modern commercial steam engines are designed to extract the maximum amount of energy from the steam generated. Features such as condensing turbines, heat recovery systems, and advanced materials help optimize performance. The efficiency of a steam engine can be further enhanced by integrating it into systems that use excess heat for other processes or by coupling it with combined heat and power (CHP) systems.
- Long-Term Reliability and Maintenance: For large-scale projects, long-term reliability is critical. Steam engines used in these projects typically operate in harsh environments, requiring robust construction and the ability to withstand extended periods of high-pressure and high-temperature operations. Manufacturers often offer extended warranties and service contracts, ensuring that steam engines maintain peak performance over many years. Predictive maintenance technologies, such as sensors and AI-powered monitoring, can also help optimize the engine’s performance and identify potential issues before they result in costly downtimes.
- Regulatory Compliance and Environmental Considerations: Large-scale projects often have to meet stringent regulations, including emissions standards, environmental impact assessments, and efficiency certifications. Steam engines sold for these projects must comply with local and international standards, including those related to emissions of carbon dioxide (CO2), nitrogen oxides (NOx), and other pollutants. Manufacturers must ensure that their steam engines are built to minimize emissions and operate within the prescribed limits set by governing bodies. Additionally, sustainability certifications, such as those for renewable energy or energy efficiency, can enhance the commercial viability of steam engines in green energy projects.
- Cost Considerations: The initial cost of purchasing and installing steam engines for large projects can be significant, but the long-term benefits of operational efficiency and reduced fuel consumption can offset these costs. Financial considerations for large projects typically include:
- Initial capital investment
- Ongoing operational costs (fuel, maintenance, repairs)
- Return on investment (ROI) based on energy savings
- Government incentives or grants for green energy projects
- Financing options such as leasing or long-term service agreements
- Installation and Commissioning: Installing steam engines for large-scale projects requires expert engineering and management to ensure the system is properly integrated into the overall plant. This includes transportation, site preparation, and alignment with existing systems. Installation services often come with a detailed project plan, from the initial feasibility study to final commissioning. The commissioning process includes thorough testing of the system under full load to ensure all components are functioning as intended.
- Partnerships and Supplier Support: Working with experienced steam engine manufacturers or suppliers is essential for large projects. These partnerships can provide comprehensive support, including:
- Design and engineering consultation
- On-site project management
- Training for operators and maintenance teams
- Post-sale customer service and ongoing support
- Spare parts supply
Conclusion:
The sale of commercial steam engines for large projects involves more than just the transaction of equipment; it requires tailored solutions, a deep understanding of the project’s goals, and long-term support to ensure the steam engine continues to meet the project’s needs. Successful integration of steam engines into large-scale energy generation systems, industrial processes, and renewable energy projects relies on a combination of advanced technology, customization, efficiency, and sustainability. As industries move toward greener energy solutions, steam engines remain a vital part of the energy infrastructure, providing reliable and efficient power generation for large commercial projects across various sectors.
For large-scale commercial steam engine sales, the decision-making process involves not just the technical specifications but also long-term strategic goals. Buyers must carefully evaluate the lifecycle costs of steam engines, including installation, operation, and maintenance. Since these engines are expected to serve for decades, it’s crucial to choose a solution that aligns with future growth, energy efficiency trends, and regulatory compliance. Furthermore, these projects often require collaboration between multiple stakeholders, including engineers, project managers, government bodies, and financial backers.
One of the primary drivers in choosing steam engines for large projects is the balance between cost-effectiveness and sustainability. While the initial investment for a high-performance steam engine may be substantial, the operational savings in terms of fuel efficiency and reduced maintenance costs make it a favorable choice in the long term. The ability to integrate these engines into a broader sustainability plan, such as carbon-neutral initiatives or green building certifications, adds to their commercial appeal. Many clients are looking for steam engines that can help them meet both energy production goals and environmental targets.
In large industrial applications, steam engines often serve as the backbone of operations. These engines can power essential machinery, heat processes, and provide the energy required for various industrial applications. Their ability to integrate with existing infrastructure and boost the efficiency of energy-intensive processes is highly valued in industries such as manufacturing, petrochemicals, and pulp and paper production. Moreover, steam engines can be integrated into combined heat and power (CHP) systems, which maximize energy use by producing both electricity and heat from the same fuel source, further improving efficiency.
As industries increasingly focus on energy transition and decarbonization, steam engine sales are aligning with renewable energy efforts. The shift from fossil fuels to renewable sources, like biomass, solar thermal, and geothermal energy, is paving the way for steam engines that can operate efficiently with low-carbon fuels. Buyers are now seeking solutions that are adaptable to renewable energy sources, offering the flexibility to transition over time as part of a long-term sustainability strategy. In addition, with the rise of green hydrogen as a fuel for industrial-scale steam generation, there is a growing market for engines that can operate on this clean and renewable resource.
Integrating steam engines into large projects also brings operational benefits. When properly maintained, these engines can run efficiently for decades, providing reliable baseload power for various industries. In addition to their primary role of power generation, they can also provide thermal energy to heat systems or support industrial processes like drying, distillation, and evaporation. By utilizing waste heat recovery technologies, steam engines can further reduce energy consumption, allowing for more sustainable operations that align with industry best practices.
Another benefit of steam engine systems is their flexibility in combining with other technologies. For instance, steam engines can work seamlessly with gas turbines, combined-cycle systems, or energy storage technologies to optimize energy output. Hybrid systems like these are gaining popularity as they help balance the intermittency of renewable sources like wind and solar. They also improve grid stability by providing reliable power during periods of high demand or when renewable generation is low.
While traditional steam engine designs still play a role, there is increasing demand for cutting-edge technologies that improve overall system performance. Advances in materials science, such as the development of superalloys that can withstand higher temperatures and pressures, are making steam engines more efficient than ever. Additionally, the use of advanced sensors and AI-driven analytics allows operators to monitor engine performance in real-time and predict maintenance needs, thereby minimizing downtime and improving the lifespan of the equipment.
For large commercial projects, choosing the right steam engine manufacturer is essential. Established manufacturers often offer a suite of additional services, such as custom engineering, installation support, training, and after-sales service. The expertise of the manufacturer in handling large-scale, complex installations can significantly impact the success of the project. Whether it’s ensuring smooth integration with existing systems or helping clients navigate regulatory approvals, the support provided by the supplier can be a decisive factor in the selection process.
Moreover, some companies may offer financing options or leasing programs to help offset the initial capital costs associated with purchasing steam engines. These financing structures can make steam engine solutions more accessible to large projects, particularly those in developing regions or industries with limited upfront capital. The ability to finance steam engine purchases or enter into long-term service agreements can provide clients with the flexibility to manage their budgets while still investing in high-quality, efficient energy solutions.
For governments and organizations pursuing large-scale infrastructure projects, steam engines represent a reliable and proven technology that can contribute to energy security and economic growth. As part of a comprehensive energy strategy, steam engine solutions can support national energy policies aimed at reducing greenhouse gas emissions, increasing the adoption of renewable energy, and improving overall energy efficiency across various sectors. By offering a robust solution for industrial power generation, heating, and even district-wide energy needs, steam engines help ensure that energy is delivered efficiently and sustainably to meet growing global demands.
Ultimately, commercial steam engine sales for large projects hinge on meeting the specific needs of the client while offering a flexible, scalable, and efficient energy solution. With advancements in technology, fuel options, and system integration, steam engines continue to provide a reliable, cost-effective energy source for industries looking to optimize their operations and contribute to a greener future. Whether through conventional steam generation, the use of renewable fuels, or integration with hybrid energy systems, steam engines remain an essential part of the energy landscape for large-scale commercial projects.
As the demand for cleaner, more sustainable energy grows, the role of steam engines in large-scale commercial projects is evolving to meet the expectations of industries and governments alike. The growing push for net-zero emissions and sustainable development goals (SDGs) is driving further innovation in steam engine technology, allowing for more energy-efficient, eco-friendly, and cost-effective solutions that align with global environmental targets.
For many large-scale projects, particularly in industries like manufacturing, mining, food processing, and refining, steam engines are indispensable for providing not just electricity but also thermal energy needed for various processes. The ability of steam engines to produce both power and heat (via combined heat and power (CHP) systems) is a significant advantage in projects looking to maximize energy efficiency. This dual-purpose functionality helps reduce energy waste, lower fuel consumption, and decrease overall emissions, thus creating a more sustainable and cost-effective energy solution.
The integration of digital technologies into steam engines is also becoming a significant trend in large commercial projects. The incorporation of smart sensors, Internet of Things (IoT) devices, and AI-driven analytics into steam engine systems is transforming the way operators manage and monitor these systems. Real-time data allows for predictive maintenance, where potential issues are identified and addressed before they lead to expensive downtime or system failures. Digital tools can also optimize steam generation processes, adjusting the operation of the engine based on energy demand, temperature, and pressure readings, thereby increasing overall efficiency.
In many cases, big data and advanced modeling are used to design steam engine systems that operate at peak performance. These technologies help identify the optimal operating conditions and potential areas for improvement, such as adjusting fuel input or modifying the system to reduce waste. By integrating machine learning into system monitoring, steam engine technology can continuously learn and adapt to new operational patterns, ensuring that the system operates as efficiently as possible, even in the face of fluctuating demands or external conditions.
Hybrid energy systems, which combine traditional steam engines with other forms of energy generation such as solar, wind, or battery storage, are also gaining popularity in large projects. These systems allow businesses to create a more resilient and flexible energy infrastructure, capable of handling peak demand while reducing reliance on fossil fuels. Steam engines can serve as a reliable baseload power source, while renewable technologies provide the additional energy needed during periods of high renewable generation or lower energy demand. The ability to seamlessly integrate steam engines with renewable energy sources is especially important in areas where grid infrastructure is limited or where energy independence is a priority.
The role of steam engines in hybrid systems extends beyond the traditional power generation sector. They are increasingly used in off-grid and remote locations where access to conventional power sources is limited. For industries operating in remote areas, such as mining or oil extraction, steam engines offer a reliable and efficient means of power generation and heating. These systems can be powered by local biomass or waste materials, reducing transportation costs and making use of otherwise discarded resources. This approach, known as circular economy, not only lowers costs but also aligns with sustainability objectives by reducing waste and lowering carbon emissions.
Energy storage technologies, such as thermal storage, are also being integrated with steam engine systems to create more dynamic energy solutions. By storing excess energy generated during times of low demand or high renewable output, these systems can release stored energy when needed, ensuring a continuous and reliable power supply. This is particularly beneficial for balancing the intermittency of renewable energy sources like solar or wind. For example, steam engines powered by solar thermal energy can use molten salt storage systems to store heat collected during the day and use it to generate steam and electricity during the night or on cloudy days.
As part of a comprehensive energy strategy, steam engines are being considered for use in district energy systems that provide heating and cooling to large communities or industrial zones. In these systems, steam engines can be used to generate steam, which is then distributed via a network of pipes to supply heat or hot water for buildings, industrial processes, and even electricity generation. The integration of steam engines with geothermal energy or waste heat recovery systems offers an environmentally friendly and cost-efficient approach to meeting the heating needs of urban or industrial centers.
Moreover, carbon capture and storage (CCS) technologies are being explored as a way to reduce emissions from steam engine systems, particularly in projects relying on fossil fuels or industrial waste. By capturing the carbon dioxide produced during combustion and storing it underground or utilizing it in other industrial processes, CCS can help mitigate the environmental impact of steam engine systems. As more industries face pressure to meet stricter emissions regulations, the adoption of CCS technologies is likely to grow, further contributing to the sustainability of steam engine-powered facilities.
Another key consideration in the commercialization of steam engines for large-scale projects is local economic impact. For many developing nations or emerging markets, steam engine technology provides an opportunity for energy independence and job creation. The development and installation of steam engines, along with the infrastructure to support them, often involve the creation of new jobs in fields such as engineering, manufacturing, maintenance, and project management. Additionally, the ability to generate energy locally can reduce the reliance on imported fuels, contributing to energy security and the stabilization of local economies.
As steam engines continue to evolve and adapt to the demands of modern energy systems, their role in large commercial projects will only grow. Whether for power generation, heating, or process energy, steam engines remain an integral part of the energy landscape, offering reliable, efficient, and adaptable solutions for businesses and governments seeking to meet the challenges of sustainability, economic growth, and energy security. The ongoing integration of renewable energy sources, digital technologies, and energy storage systems will continue to shape the future of steam engine technology, ensuring that it remains a viable and effective solution for large-scale energy projects for years to come.
4o mini
Steam Engines in Marine Transport and Shipping
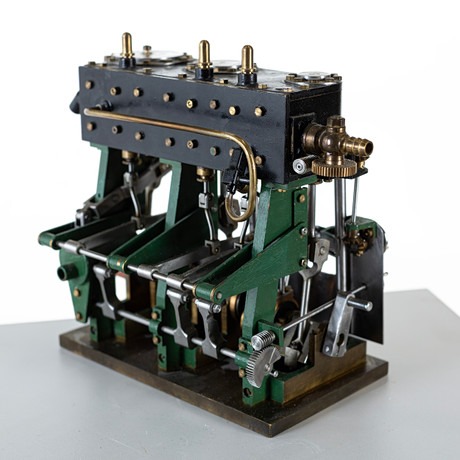
Steam engines have a long history in marine transport and shipping, dating back to the early 19th century when steam-powered vessels revolutionized the shipping industry. Although modern shipping vessels primarily rely on diesel engines or turbines, steam engines continue to play a role in specific areas of marine transport, offering a reliable and efficient means of propulsion in some contexts. Today, steam engines are used mainly in smaller vessels, specialty ships, and retrofitted vessels, although advancements in marine technology and the shift toward more sustainable energy solutions are transforming their role.
Steam engines in marine transport operate based on the same fundamental principles as those in land-based applications, converting the energy stored in steam into mechanical energy that drives the ship’s propeller. Here’s how they’ve evolved and how they are used in marine applications today:
Historical Context and Early Development:
In the early days of steam-powered marine transport, steam engines replaced traditional sail power and opened up new possibilities for international trade and travel. The first successful steamship, the Clermont, was designed by Robert Fulton and launched in 1807, marking the beginning of steam propulsion in shipping. These early steamships were primarily used for passenger transport and cargo shipping on rivers and across oceans.
The steam engine provided several advantages over sailing vessels, including:
- Independence from wind conditions, allowing ships to travel more reliably and at a steadier pace.
- Faster transit times, significantly reducing travel durations.
- Increased safety and reliability, particularly in areas with unreliable or unpredictable winds.
By the late 19th and early 20th centuries, steam-powered ships became commonplace, including in the form of ocean liners, which were designed for transoceanic voyages, and cargo ships that could transport goods across vast distances.
Modern Uses of Steam Engines in Marine Transport:
Although steam engines are no longer the primary power source for most large commercial ships, they are still used in certain types of vessels and marine applications.
- Steam Turbines in Large Passenger Ships and Military Vessels: Steam turbines, which operate on the principles of steam engines, continue to be used in some large ships, especially in military vessels like aircraft carriers and in luxury cruise liners. These steam turbines are highly efficient at generating the large amounts of power required for such massive vessels. In these cases, the steam engine is often used in a reheat cycle that generates electricity and provides propulsion. The efficiency of steam turbines in large vessels is especially notable when they are powered by nuclear reactors, as is the case in some naval vessels. The nuclear steam turbine system generates steam through nuclear fission, driving turbines that produce electricity and provide propulsion, all while offering nearly limitless range.
- Hybrid and Retrofits for Energy Efficiency: As the maritime industry increasingly seeks to reduce carbon emissions and improve fuel efficiency, some ships have been retrofit with hybrid steam systems, combining conventional propulsion with steam or heat recovery technologies. In certain applications, waste heat recovery systems can capture excess heat from engines, boilers, and other shipboard systems and convert it into steam for propulsion or electricity generation. This improves overall energy efficiency, especially in large vessels that require substantial energy to maintain high speeds.
- Specialty and Historical Ships: Some specialty vessels, such as historic steamships, museum ships, or tourist cruises, continue to use steam engines to maintain historical authenticity. These ships, often used for tourism or public display, are sometimes powered by original steam engines or modern reproductions. The use of steam engines in these ships is largely for educational or heritage purposes, preserving maritime history.
- Small, Commercial, and Private Vessels: While less common, steam engines are still found in smaller, private vessels, particularly in regions where they are seen as a novelty or in areas where access to modern fuel sources is limited. Steam launches or steam-powered riverboats are sometimes used for recreational purposes, where the engine offers a nostalgic appeal or unique method of propulsion.
- Sustainable Shipping: With the growing concern over environmental impact, steam engines and turbines are being reconsidered in the context of sustainable shipping. Some experimental projects explore the potential of biofuels, liquefied natural gas (LNG), and even hydrogen as alternatives to traditional fossil fuels in steam propulsion systems. The goal is to reduce greenhouse gas emissions while leveraging the efficiency of steam engines. For instance, steam engines that run on biomass or bio-oil are being tested as potential solutions for greener shipping operations.
Advantages of Steam Engines in Marine Transport:
- Proven Technology: Steam engines are a well-understood and reliable technology that has been used in marine transport for centuries. Their operational principles are simple, and they require less maintenance than more modern internal combustion engines in certain applications.
- Fuel Flexibility: Unlike diesel engines, which require specific fuels, steam engines can use a variety of fuel sources, including coal, oil, biomass, and even waste heat. This flexibility is valuable in regions with limited access to specific fuel types or in projects where sustainable fuels are desired.
- Energy Efficiency: Steam engines, particularly in larger vessels, can offer high efficiency in generating power and providing heat for onboard systems. When integrated with modern systems like heat recovery steam generators (HRSGs), steam engines can significantly reduce energy waste, especially in systems that require consistent high-power output.
- Reduced Emissions: With the right fuel and heat recovery systems, steam engines can offer lower emissions in comparison to traditional marine propulsion methods, particularly in hybrid configurations that combine renewable energy with steam power.
Challenges and Limitations:
- Size and Weight: Traditional steam engines are bulky and require a significant amount of space and infrastructure for steam generation, making them less practical for smaller vessels. This is one of the reasons that modern diesel engines and gas turbines have largely replaced steam engines in commercial shipping.
- Maintenance: While steam engines are simple in design, their operation requires continuous monitoring and maintenance of boilers, pressure systems, and steam turbines. Keeping steam engines running efficiently over time requires skilled labor and regular inspections.
- Efficiency and Fuel Consumption: Traditional steam engines, especially in smaller vessels, can be less fuel-efficient compared to modern internal combustion or electric engines. However, when steam engines are used in combination with modern systems or as part of a hybrid model, their efficiency can be improved.
- Capital and Operational Costs: Installing and maintaining steam engines on modern vessels can be costly, particularly when compared to newer propulsion technologies. However, for specific applications or retrofitting older ships, steam engines may still offer cost benefits over time.
Future of Steam Engines in Marine Transport:
The future of steam engines in marine transport may be linked to innovations in clean fuel technologies, hybrid systems, and integrated energy management systems. As the maritime industry increasingly shifts toward low-carbon, sustainable operations, steam engines may find new applications in eco-friendly shipping solutions. With advances in fuel diversification, waste heat recovery, and energy-efficient steam systems, steam engines may once again play a significant role in modern shipping, particularly in niche or specialized markets.
Additionally, the continued exploration of hydrogen-powered steam systems, as well as potential carbon capture and storage (CCS) technologies, may allow steam engines to remain relevant in the pursuit of a more sustainable maritime industry.
In recent years, there has been a renewed interest in exploring the potential of steam engines in marine transport, particularly in the context of cleaner energy and sustainability. This interest is driven by the growing demand for alternative power solutions that reduce the environmental impact of traditional shipping fuels. While steam engines may never replace diesel or gas turbines in large-scale commercial shipping, they are being revisited as part of the transition to more sustainable maritime operations.
One significant area of exploration is the use of hydrogen as a fuel for steam-powered systems. Hydrogen, when used as a fuel in steam engines, can produce water vapor as a byproduct, significantly reducing harmful emissions like carbon dioxide. The potential for hydrogen-powered steam engines in shipping lies in their ability to provide zero-emissions propulsion, making them a promising option for green shipping initiatives. The challenge, however, lies in the production, storage, and transportation of hydrogen fuel at a scale sufficient for commercial vessels. Nevertheless, research into hydrogen fuel cells, which can be integrated with steam engines or used in hybrid systems, continues to progress, promising to make steam-powered ships more eco-friendly in the future.
Another avenue of interest is biofuels. Biofuels made from renewable sources, such as algae, plant oils, or even waste materials, can potentially replace traditional fossil fuels in steam engines. Biomass-derived biofuels can be used in place of coal or oil, offering a more sustainable and less polluting alternative for steam propulsion. While biofuels are not yet as widely available or cost-competitive as traditional fuels, their use in steam engines could represent a step toward reducing the shipping industry’s reliance on fossil fuels and lowering greenhouse gas emissions.
The adoption of waste heat recovery systems also offers an opportunity to enhance the efficiency of steam-powered marine vessels. By capturing excess heat from exhaust gases, boilers, or engines, waste heat recovery systems can generate additional steam, improving overall fuel efficiency. These systems are especially beneficial in vessels with high power demands, such as cruise ships, container ships, or tankers, where the integration of a steam engine with a heat recovery system could lead to significant energy savings.
Moreover, advancements in materials science are enabling the development of more efficient and durable steam engines. The creation of superalloys and high-performance coatings allows steam engines to operate at higher temperatures and pressures, increasing their efficiency and extending their operational lifespan. These innovations are especially important for marine applications, where steam engines must operate under demanding conditions, including high levels of vibration, corrosion from seawater, and the need for continuous, long-duration operation.
In smaller or specialized vessels, such as tourist ships or historic steamers, steam engines may see a resurgence as part of a niche market. For example, eco-tourism operations, particularly in regions with strong historical ties to steam-powered transport, could benefit from retrofitting old steamships or building new ones powered by sustainable fuel sources. These ships could serve as both tourist attractions and practical solutions for transporting visitors in environmentally sensitive areas.
Furthermore, steam engines are being integrated into hybrid systems that combine multiple sources of energy. These hybrid systems may combine steam power with solar panels, wind turbines, or battery storage systems to create more efficient and eco-friendly vessels. Solar or wind power can generate electricity to charge batteries or directly support onboard systems, while steam engines provide the backup power required for propulsion or to generate electricity when renewable sources are unavailable. Hybrid models can significantly reduce the amount of fuel required, cut emissions, and enhance the overall sustainability of maritime operations.
The growing interest in sustainable shipping and zero-emissions goals is pushing the industry toward the adoption of greener technologies, and steam engines could play a vital role in that transformation. As international regulations become more stringent regarding carbon emissions and environmental impact, particularly under the International Maritime Organization (IMO) guidelines, shipping companies may turn to a combination of steam, renewable energy, and innovative fuel sources to meet these standards. These technologies could help companies reduce their carbon footprints while maintaining the reliable, robust power generation capabilities that steam engines are known for.
One of the main hurdles in the adoption of steam engines in modern marine transport, however, is the high capital investment required to develop, retrofit, and maintain these systems. While steam engines themselves are reliable and durable, the infrastructure needed to support their operation — such as boiler systems, fuel storage, and maintenance — can be costly. Additionally, steam engines generally require more space on board the vessel, which can limit their application in modern ship designs that prioritize cargo capacity and efficiency.
However, with the increasing push for decarbonization in the maritime sector and the need for alternative energy solutions, steam engines may find a resurgence in specific applications. The focus on sustainable shipping is likely to drive further innovation in steam engine technology, including new combustion methods, fuel types, and system integration strategies that improve overall performance.
Moreover, governments and international bodies are beginning to support the development of alternative fuels and technologies for the maritime industry, including subsidies or incentives for research and development of cleaner energy systems. These efforts, combined with the growing market demand for sustainable solutions, may create a more favorable environment for the adoption of steam engines in modern shipping, particularly in specialized or hybrid systems.
In conclusion, while steam engines are no longer the dominant force in marine transport, their ability to adapt to new technologies and fuel sources positions them as a valuable part of the ongoing evolution of the maritime industry. By integrating steam propulsion with cleaner fuels, hybrid systems, and renewable energy sources, steam engines could help propel the shipping sector into a more sustainable future, contributing to both energy efficiency and environmental goals. As innovation continues in the realm of clean maritime technologies, steam engines may once again prove their worth as a reliable and eco-friendly solution for modern marine transport.
As the maritime industry continues to explore cleaner, more efficient technologies, steam engines could increasingly be integrated into alternative power solutions for ships, especially when combined with cutting-edge technologies such as artificial intelligence (AI) and machine learning. AI-driven systems can optimize the operation of steam engines, managing fuel usage, boiler efficiency, and steam pressure in real-time, allowing for smoother operations and reduced energy consumption. By integrating data analytics with steam propulsion systems, ships could run at optimal performance, adjusting parameters dynamically based on environmental conditions, cargo weight, and other factors, maximizing fuel efficiency and reducing emissions.
Another important area for the future of steam engines in marine transport is the exploration of closed-loop systems that can minimize energy loss. By using technologies like thermal energy storage (TES) systems, excess heat produced by the engine could be stored and later used to generate additional steam when needed, further improving the energy efficiency of the system. This closed-loop concept aligns well with modern goals of minimizing waste and making the most out of every resource, especially in terms of heat energy that would typically be lost to the environment.
Furthermore, steam engines have the potential to contribute to the development of sustainable maritime supply chains. As shipping industries strive to reduce their carbon footprints, integrating steam engines into supply chain logistics could offer a greener solution for the transportation of goods across the globe. For instance, steam-powered bulk carriers or tankers could use biofuels, hydrogen, or waste-derived fuels, which can be locally sourced, reducing the carbon footprint of shipping and contributing to regional economies.
Carbon capture technologies could also be paired with steam engines in an effort to meet greenhouse gas emission reduction targets. Since steam engines, particularly in industrial applications, produce carbon dioxide during combustion, integrating carbon capture and storage (CCS) technologies could help sequester the CO2 emissions from the engine. These systems capture the carbon dioxide produced by the combustion of fuels and store it underground, preventing it from entering the atmosphere and contributing to climate change. As pressure increases for the maritime sector to adhere to global climate agreements, integrating CCS with steam engines may become an essential part of the decarbonization process for ships.
Moreover, as smart port infrastructure becomes more widespread, the integration of smart grids and energy storage systems could further enhance the role of steam engines in marine transport. With smart ports, vessels can potentially connect to shore-based systems to recharge batteries, plug into renewable energy sources, or even transfer heat to shore-based facilities, reducing the reliance on onboard fuel and improving overall operational efficiency. The adoption of microgrids powered by renewable energy at ports could also support steam-powered ships, making it easier to fuel vessels sustainably while docked.
The development of eco-friendly antifouling technologies for steam-powered vessels is another important consideration. Marine growth, such as barnacles and algae, can accumulate on ship hulls, increasing fuel consumption as ships struggle to maintain speed. New antifouling coatings or bio-based materials can reduce this drag and, in turn, reduce fuel consumption. By pairing these advancements with more efficient steam propulsion systems, shipping companies could see a significant reduction in energy consumption and an improvement in overall environmental performance.
In terms of regulatory support, governments and international maritime organizations are increasingly emphasizing the transition toward cleaner maritime operations. The International Maritime Organization (IMO) has set ambitious targets for reducing greenhouse gas emissions from international shipping, with goals such as reducing the sector’s carbon intensity by 40% by 2030. This regulatory pressure is expected to accelerate the development and adoption of green shipping technologies, including hydrogen-based steam propulsion, biofuel-powered steam engines, and other alternative fuel options.
Green financing initiatives are also playing a role in this transformation. As private and institutional investors look to fund sustainable projects, companies in the maritime sector may be able to access green bonds or other funding mechanisms that incentivize the adoption of environmentally friendly technologies, including retrofitting existing vessels with modern steam systems. This could make the initial capital investment required to develop and install steam propulsion systems more feasible, particularly in the face of the rising cost of fuel and the need for cost-effective energy solutions.
Public-private partnerships (PPPs) could further facilitate the development of steam-powered marine transport solutions. Governments, research institutions, and private companies could collaborate to accelerate the adoption of hybrid steam technologies and provide subsidies for fleets making the transition to greener, more sustainable operations. With strategic incentives, the maritime sector could overcome the cost barriers associated with implementing steam engines and expand their role in sustainable maritime transport.
Looking ahead, the future of steam engines in marine transport is intertwined with the larger goal of achieving net-zero emissions in the maritime sector. While steam engines may not replace diesel or gas turbines in all shipping applications, their role in sustainable shipping could grow through innovations in fuel technology, energy efficiency, and integration with renewable energy sources. As the global shipping industry faces pressure to reduce its carbon footprint, steam engines may offer a reliable and adaptable solution, particularly in hybrid systems, energy recovery applications, and as a backup power source for eco-friendly ships.
Ultimately, the long-term success of steam engines in marine transport depends on the continued development of complementary technologies and the willingness of the maritime industry to embrace new, sustainable energy systems. As the world seeks to move away from fossil fuels and mitigate climate change, steam engines could once again play a crucial role in shaping a more sustainable and energy-efficient maritime future.
Steam Engine Applications in the Automotive Industry
The automotive industry, as we know it today, is primarily driven by internal combustion engines (ICE), electric motors, and hybrid systems. However, steam engines played a significant role in the early development of the automobile, and while they have been largely replaced by more efficient and compact technologies, there are still niche applications and opportunities where steam engines could have a role in modern automotive technologies.
Historical Context and Early Use:
The earliest vehicles powered by steam engines date back to the late 19th and early 20th centuries. Before gasoline-powered engines became dominant, steam-powered automobiles were among the most innovative and widely used forms of transportation. These early steam cars were often seen as a potential alternative to the emerging internal combustion engine (ICE) technology, and several companies developed steam-powered vehicles during this time.
The Stanley Steamer, one of the most famous steam-powered cars from the early 1900s, was well-known for its performance and speed. Steam cars, like the Stanley Steamer, had some distinct advantages over internal combustion vehicles of the time, including:
- Smooth and quiet operation: Steam engines ran smoothly, without the vibrations and noise associated with early gasoline engines.
- Fewer moving parts: Early steam engines were simpler in design, with fewer components than the internal combustion engines of the time.
- Potential for rapid acceleration: Steam engines could produce peak power almost instantly, making steam cars capable of quick acceleration, a characteristic that garnered attention in competitive racing.
Despite their benefits, steam cars were limited by several factors:
- Size and weight: Steam engines were bulky and required large boilers to generate the steam needed for operation, which made the vehicles heavier and less practical for everyday use.
- Slow refueling times: It could take several minutes to build up enough steam to get the vehicle moving, making steam-powered cars less practical than the quick-starting gasoline engines.
- Limited range: Although steam cars could have a long range, they needed a constant source of water and fuel, which made them less convenient for long-distance travel compared to gasoline-powered cars.
By the 1920s, the internal combustion engine, with its higher energy density, greater efficiency, and convenience, had largely supplanted steam engines in the automotive industry. As a result, steam-powered vehicles faded from the mainstream, and innovations in gasoline and diesel engines led to the rise of modern vehicles.
Modern Applications of Steam in the Automotive Industry:
While the steam engine has been mostly phased out in mainstream automotive applications, there are still some unique uses and research into how steam power could be integrated into modern vehicles or influence new technologies.
1. Steam Hybrid Systems:
In recent years, there have been discussions about integrating steam technology into hybrid propulsion systems. The concept would involve using a steam engine in combination with an internal combustion engine or electric motor. Such systems could provide additional power during acceleration or provide a backup power source for charging electric batteries. The hybrid steam system could also take advantage of waste heat generated by the combustion engine or the brakes to produce steam, which could then be used to assist with propulsion or to power ancillary systems.
This integration could potentially offer the following benefits:
- Energy recovery: Similar to how some hybrid vehicles capture energy through regenerative braking, a steam engine could recover energy from the exhaust heat of the vehicle’s engine, turning it into steam power for additional propulsion.
- Improved fuel efficiency: By utilizing steam as an auxiliary power source, vehicles could reduce their reliance on conventional fuels and increase overall efficiency.
- Reduced emissions: When combined with renewable energy sources or low-carbon fuels, a hybrid steam system could help reduce the environmental footprint of vehicles.
2. Steam Turbines for Specialized Applications:
In some specialized applications, such as heavy-duty trucks or off-road vehicles, steam turbines could be used for additional power generation. These vehicles, which require a substantial amount of power for propulsion or for running equipment, might benefit from the high efficiency of steam turbines. Hybrid turbine-steam systems could be an interesting option for these applications, allowing vehicles to harness waste heat from engines and exhaust systems, turning it into useful steam power.
3. Experimental Steam-Powered Cars:
Some engineers and inventors are experimenting with steam-powered cars or steam-driven electric generators as a way to explore alternative, environmentally friendly power sources. These vehicles often serve as prototypes or experimental vehicles rather than commercially viable production models. Innovations in materials, energy storage, and steam generation technology have made it easier to develop smaller, more efficient steam systems, which could potentially be used in cars for educational purposes, exhibitions, or as part of eco-friendly car challenges.
4. Waste Heat Recovery and Steam Generation:
In modern vehicles, one of the primary areas of focus for energy efficiency is the recovery of waste heat, especially from engine exhaust. Some companies and research teams are investigating thermoelectric generators or organic Rankine cycles (ORC), which use waste heat to generate steam and power additional systems on the vehicle. This could allow vehicles to make better use of the heat energy that would otherwise be lost to the environment. Steam could be used to power auxiliary components or be converted into electricity to charge the battery in electric or hybrid vehicles.
5. Public Interest and Nostalgia Projects:
There is also a niche market for steam-powered vehicles driven by enthusiasts, collectors, or historical preservationists. These steam-powered vehicles are typically used for display or exhibitions, and some are still in operation in limited capacities for tourism or eco-friendly promotional events. The vintage steam-powered cars may not be practical for everyday use, but they hold sentimental and historical value, showcasing the early years of automotive innovation.
6. Sustainability and Green Technologies:
As the automotive industry moves toward greener, more sustainable solutions, there may be a renewed interest in steam engines powered by renewable energy. For instance, solar power, biomass, or geothermal energy could be harnessed to create steam for automotive propulsion. While still in the early stages, such ideas could be part of the future of sustainable, low-carbon transportation.
The interest in zero-emissions vehicles and the reduction of carbon footprints may lead to further exploration of alternative energy sources, including steam. If technology progresses to the point where efficient steam engines could be miniaturized and adapted for automotive use, steam-powered vehicles might once again become viable, albeit in niche markets.
Challenges to the Adoption of Steam Engines in Modern Automobiles:
Despite the intriguing potential for steam in modern vehicles, several challenges make the widespread adoption of steam-powered automobiles unlikely in the near future:
- Size and Complexity: Steam engines are bulky, and modern cars are designed for compactness, efficiency, and ease of use. The space and infrastructure needed for a steam engine, including a boiler, heat exchangers, and fuel supply, would take up valuable space in an already cramped vehicle.
- Fuel Requirements: Steam engines traditionally rely on external fuel sources, such as coal, oil, or biomass. With the focus shifting toward electric vehicles (EVs) and hydrogen fuel cells, the need for traditional fuel sources for steam power may become obsolete in the mainstream automotive market.
- Efficiency and Emissions: While steam engines are efficient in certain applications, they tend to produce more emissions compared to the modern internal combustion engine or electric motor. For steam power to be viable in the modern world, it would need to be combined with renewable energy sources or advanced emissions-reducing technologies.
- Technology Integration: Integrating steam engines into a modern car’s electrical systems, drive train, and energy management systems presents significant technical challenges. The complexity of modern automobiles makes it difficult to integrate steam engines in a way that offers tangible benefits without undermining the car’s overall efficiency.
Conclusion:
While steam engines were once a key player in automotive history, their role in the modern automotive industry is mostly relegated to niche applications and historical interest. However, as the industry continues to explore alternative and sustainable energy sources, steam engines may find a place in hybrid or experimental systems, particularly in applications focused on energy recovery, waste heat utilization, or eco-friendly vehicles. For now, steam engines are unlikely to replace traditional internal combustion or electric motors in passenger vehicles, but they could still play a role in specialized projects or as part of green energy initiatives, representing a blend of historical innovation and future sustainability.
In addition to the niche uses mentioned above, steam engines could play a role in the broader automotive sector if technological advances were to address their current limitations. One area where steam power could make an impact is in small, lightweight vehicles. Imagine compact vehicles designed for low-speed, short-distance travel, such as city cars or personal transport pods, where the size and complexity of the steam engine could be minimized. These vehicles could use a small-scale steam engine paired with renewable energy sources like solar or wind power to generate steam efficiently. This combination would reduce the need for traditional fuel and contribute to lowering the carbon footprint of daily commutes.
The concept of mobile power generation using steam engines could also be explored for specific purposes such as emergency response vehicles or recreational vehicles (RVs), where the energy demands are moderate, and the use of renewable fuel sources would be more feasible. These vehicles could utilize steam for auxiliary power systems, such as heating or backup energy generation, especially in remote or off-grid areas where access to traditional power sources might be limited.
Another potential application lies in sustainable urban transport systems. As cities worldwide push for greener transportation, incorporating steam engines in public transport networks such as buses or light rail systems could be explored. By using biofuels, hydrogen, or waste heat recovery systems, steam-powered public transport could be part of an integrated approach to cutting urban carbon emissions. In some cases, the infrastructure already in place, such as steam generation plants, could be retrofitted to support these systems, thus providing an innovative solution for more sustainable urban mobility.
Additionally, advancements in microtechnology and nanoengineering may open the door to more efficient steam engine designs. The miniaturization of components could result in steam engines that are compact enough to fit into smaller vehicles without compromising performance or energy efficiency. This could lead to entirely new vehicle designs where steam provides the main propulsion or assists with energy recovery.
The idea of steam-powered autonomous vehicles is another interesting avenue. With the rise of self-driving cars and the move toward more automated systems, a steam-powered vehicle could serve as part of an autonomous fleet, operating in specific contexts like delivery services or tourist shuttles. The ability to integrate steam engines into an autonomous vehicle fleet could offer both sustainability and energy resilience since steam engines are not reliant on the same infrastructure that gasoline-powered or even battery-powered vehicles need. They could be particularly beneficial in scenarios where long-range travel or off-grid operation is required.
Although it is unlikely that steam engines will make a full-scale comeback in the mainstream automotive industry, they can still be leveraged in hybrid technologies that combine traditional internal combustion engines with renewable energy sources or steam for auxiliary power. The eco-conscious car market is a growing segment where innovation thrives, and companies looking to differentiate themselves in terms of green technology may experiment with steam propulsion as part of their future designs.
As the automotive industry continues to face the challenge of reducing emissions and improving energy efficiency, the exploration of diverse propulsion technologies will remain a focal point. While the internal combustion engine and electric vehicles are likely to dominate, steam engines could have a valuable role to play in specific use cases, such as hybrid systems, energy recovery applications, and eco-friendly transportation options. With ongoing research into alternative fuel sources, advanced heat recovery technologies, and energy storage solutions, steam engines could evolve into a small but significant piece of the puzzle in creating more sustainable and energy-efficient vehicles for the future.
Ultimately, while the revival of steam engines in mainstream passenger cars may not be feasible, their potential in specialized applications or alternative fuel systems offers an intriguing prospect. As the automotive world shifts toward cleaner and more efficient technologies, exploring steam power’s role in that transition might yield surprising solutions, especially in the context of sustainable energy and the development of next-generation transportation systems.
As we continue to look towards the future, it’s important to recognize that alternative propulsion systems like steam engines, despite their historical limitations, are part of the larger conversation around sustainability and energy diversity. Modern research is focusing on improving the efficiency of energy conversion, reducing waste, and integrating multiple energy sources in a way that can meet the evolving demands of transportation, especially in the context of reducing global carbon emissions.
One of the key areas where steam engines could still find a place is in the research and development of renewable energy systems. The synergy between steam technology and emerging energy sources like biomass, geothermal, or solar power could result in efficient hybrid systems that offer clean, sustainable energy for automotive use. For example, using biomass to generate steam offers the possibility of creating a low-carbon power source that could be used to propel vehicles, either directly or indirectly through combined heat and power (CHP) systems. The development of advanced biofuels could also play a significant role in ensuring that steam engines run more efficiently while lowering their environmental impact.
Further, the concept of green hydrogen is becoming a significant part of discussions around sustainable transport solutions. While hydrogen fuel cells are already being considered as a viable option for passenger vehicles, using hydrogen as a fuel to generate steam for propulsion could be another path forward. Hydrogen steam engines would produce only water vapor as a byproduct, making them an environmentally friendly alternative to fossil fuels.
In a broader sense, integrating steam power in transportation infrastructure, such as train systems, could offer efficiency improvements over traditional diesel or electric engines. Many regions still rely on diesel-powered trains, which contribute to air pollution and high carbon emissions. Replacing or retrofitting these systems with steam-powered engines, perhaps running on renewable fuel or electric hybrid systems, could significantly reduce the environmental impact of rail transport. This could especially be relevant in areas where electrification of rail systems has not been financially viable.
Another aspect to consider is the potential for hybrid steam-electric vehicles, where steam power could act as a backup system when battery power is low, providing additional range or power when needed. Such systems could be particularly useful in remote areas or regions with limited access to traditional charging infrastructure. For example, in electric RVs or campers, steam could be used for heating, hot water, and emergency power generation during off-grid travel, making it a unique off-grid solution in a way that aligns with the growing interest in sustainable, off-the-grid living.
As we look at the growing market for urban mobility solutions, the rise of electric scooters, bicycles, and micro-mobility vehicles opens new possibilities for integrating steam power. While a steam engine in these small-scale vehicles might not make practical sense, microscale heat engines and small-scale thermoelectric generators could be used to harness waste heat from the vehicle’s motors or external sources. Such technologies could be leveraged for on-demand steam generation, possibly providing an innovative power source for micro-transportation vehicles.
The idea of modular steam power is another forward-thinking approach. In this scenario, vehicles could be equipped with small, modular steam engines that can be swapped out or upgraded based on evolving needs. For example, a truck with a small steam engine could be used to provide auxiliary power, heating, or cooling while on long hauls. As this modular system evolves, it could offer significant operational flexibility, reducing the dependency on centralized power sources and allowing vehicles to operate more independently, especially in remote locations.
Looking beyond individual vehicles, we can also consider how fleet operators and public transport systems might benefit from steam-powered technologies. Large commercial vehicles, such as freight trucks or buses, could use steam engines for energy recovery and auxiliary power generation. Instead of relying solely on diesel or electricity, fleets could incorporate steam systems to supplement energy needs and reduce overall fuel consumption. In some cases, sustainable biomass or waste-to-energy systems could be used to power the steam engines, providing an entirely carbon-neutral transportation solution. By integrating these systems into fleet management software, operators could optimize energy use, reducing costs and minimizing environmental impact.
For autonomous vehicles, the adoption of steam engines could be considered in the context of vehicle-to-grid (V2G) systems. Here, steam-powered vehicles could interact with smart grids to provide electricity back to the grid when not in use, storing and distributing energy as part of a decentralized network. In such a scenario, steam could contribute to both the vehicle’s operational energy needs and serve as a grid balancing tool, offering additional storage and flexibility to renewable energy sources like wind and solar.
The ongoing advancements in automotive technology and the increasing demand for sustainable solutions will undoubtedly influence the role of steam engines in the automotive sector. While it is unlikely that steam power will replace electric or internal combustion engines for the vast majority of consumer vehicles, there is potential for its integration into hybrid systems, energy recovery solutions, or specialized vehicles. The push for carbon neutrality and energy efficiency could lead to the continued exploration of alternative propulsion systems, with steam engines playing a niche yet crucial role in certain applications.
As the automotive industry continues to transition toward cleaner, more sustainable technologies, the integration of diverse power sources will remain a critical part of the conversation. Steam engines, although no longer the dominant force they once were, still hold promise in areas where their unique attributes—such as the ability to recover waste heat, provide auxiliary power, or operate on renewable fuels—can contribute to the development of smarter, greener transportation systems.
In the end, the path forward for steam engines in the automotive industry will likely be shaped by the need for innovative solutions that balance energy efficiency, environmental sustainability, and the practical demands of modern transportation. Whether through hybrid systems, waste heat recovery, or specialized vehicles, steam-powered technologies might find a small but meaningful place in the automotive landscape as we continue to explore more eco-friendly and technologically advanced means of moving people and goods.
Historical Evolution of Steam Engine Technology
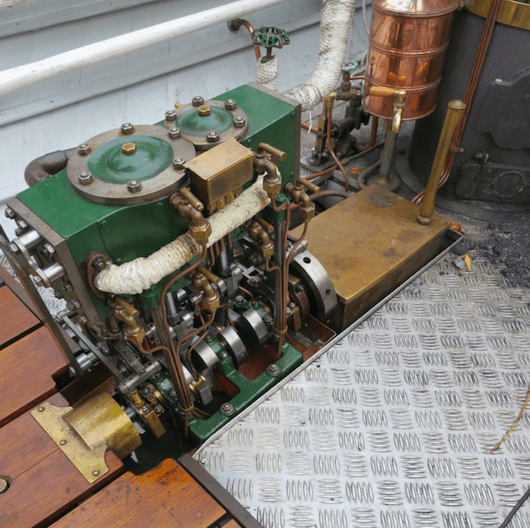
The historical evolution of steam engine technology is a story of innovation, trial and error, and eventual transformation into one of the most pivotal developments in human history. From its earliest beginnings as a primitive device to its dominance in the Industrial Revolution and beyond, the steam engine has had a profound impact on nearly every aspect of modern life, shaping industries, transportation, and society as a whole.
Ancient Concepts and Early Predecessors
The idea of harnessing steam power dates back to antiquity, though it wasn’t until the 17th and 18th centuries that practical steam engines began to emerge. The concept of using steam for mechanical work was explored in ancient civilizations, but it was only in the early modern period that technology advanced sufficiently to make steam engines viable.
- Hero of Alexandria (c. 10–70 AD): One of the first recorded ideas for a steam-powered machine came from the Greek engineer Hero of Alexandria, who designed a simple device called an aeolipile. This device was essentially a steam-powered rotary engine that used the force of steam escaping from a rotating sphere to produce motion. While it was a fascinating concept, it was not practical for industrial use and remained a curiosity rather than a functional machine.
The Early Modern Era: Birth of the Steam Engine
In the 17th century, the development of steam engines began in earnest, fueled by advances in both science and engineering.
- Thomas Savery (1698): Often considered one of the first to patent a steam-powered device for practical use, Thomas Savery was an English engineer who developed a machine known as the Savery engine. This was essentially a steam pump designed to remove water from flooded mines. While the engine had significant limitations (including a risk of explosion), it marked a critical step in the development of steam power as a useful tool in industry.
- Thomas Newcomen (1712): Building on Savery’s work, Thomas Newcomen developed a more practical and safer steam engine, known as the Newcomen engine. It was an atmospheric engine, meaning it used steam to create a vacuum that drove a piston. The Newcomen engine was primarily used to pump water out of coal mines, a critical task during the early stages of the Industrial Revolution. Though it was far from efficient, it laid the foundation for later improvements.
The Industrial Revolution: Refining Steam Power
The Industrial Revolution, beginning in the late 18th century, witnessed rapid advancements in steam engine technology. These improvements were crucial in driving industrialization, transforming economies, and changing the way people lived and worked.
- James Watt (1765): Perhaps the most famous name associated with steam engine development, James Watt was a Scottish inventor and mechanical engineer who made significant improvements to the Newcomen engine. In 1765, Watt introduced the concept of the separate condenser, which dramatically increased the efficiency of steam engines. Prior to Watt’s innovation, steam engines had to cool and reheat the piston in each cycle, which wasted a great deal of energy. Watt’s separate condenser allowed for continuous operation without wasting heat, making his design far more efficient and practical for industrial use. Watt also introduced the rotary motion feature to the steam engine, which allowed it to power machines in addition to simply pumping water. His improvements enabled steam engines to be used in a wide variety of industries, from textile mills to factories and transportation. By 1776, Watt’s engines were widely used in steam-powered mills, and he was awarded a patent for his design in 1781.
- Watt’s Engine and Factory Expansion: Watt’s innovations spurred the widespread adoption of steam engines in industry. Factories, mills, and foundries adopted steam engines as the primary source of power, significantly increasing production capacity and efficiency. The demand for Watt’s engines grew rapidly, and the manufacturing of steam engines became a major industry in itself.
Steam Engine’s Role in Transportation
While steam engines were initially used primarily in industry, their application in transportation had an equally transformative impact on the world.
- Richard Trevithick (1801): Richard Trevithick, a British engineer, is often credited with developing the first steam-powered locomotive. In 1801, he built a high-pressure steam engine that could generate more power and was smaller and more efficient than previous designs. Trevithick’s locomotive, known as The Penydarren locomotive, was capable of pulling a train, though it was not widely adopted due to its high fuel consumption and mechanical problems.
- George Stephenson (1814-1825): While Trevithick had the vision, it was George Stephenson, another British engineer, who refined the steam locomotive and made it commercially viable. In 1814, Stephenson built the Locomotion No. 1, which became the first steam-powered train to carry passengers and freight on a public railway in 1825. His work culminated in the construction of the Liverpool and Manchester Railway, the world’s first fully operational railway line that used steam-powered locomotives. Stephenson’s engine, The Rocket (1829), achieved speeds of up to 30 miles per hour, which was a revolutionary feat for its time.
- Steamships: Steam engines were also applied to naval transportation. In 1807, Robert Fulton, an American engineer, successfully launched the Clermont, the world’s first commercially successful steam-powered passenger vessel. Steamships revolutionized transportation across bodies of water, as they were faster and more reliable than traditional sailing ships. Over time, steamships replaced sailing vessels on many major maritime routes, contributing to global trade expansion and travel.
Expansion and the Steam Age
By the mid-19th century, steam engines were being used in a wide variety of applications. The industrial revolution had created new industries, and steam power was central to these developments. Factories, mills, and mines all relied heavily on steam engines. The creation of the steam turbine in the 1880s by Charles Parsons was another pivotal development, allowing steam engines to generate electricity more efficiently. This led to the widespread use of steam in power plants, providing electricity to homes, businesses, and industries.
- Steam in Agriculture and Manufacturing: Steam engines were used not only in factories but also in agriculture and construction. For example, steam-powered tractors, combines, and threshers helped revolutionize farming by allowing for more efficient plowing, planting, and harvesting. Similarly, steam-powered machines were integral to construction, helping build the infrastructure that fueled the further industrialization of the world.
Decline and Legacy of the Steam Engine
By the early 20th century, steam engines began to face competition from internal combustion engines, which were smaller, more efficient, and better suited to automotive and aviation applications. The development of electric motors also provided a more efficient alternative for many applications.
Despite the rise of these newer technologies, steam engines remained an important part of industry, particularly in power generation. Steam turbines, in particular, continued to be used in electrical power plants throughout the 20th century and are still used today in many parts of the world for generating electricity.
In the realm of transportation, the steam locomotive eventually gave way to diesel and electric trains, though steam engines continued to be used in some regions well into the 20th century.
While the steam engine is no longer the driving force behind most industries and modes of transport, its legacy remains. The engine’s invention laid the foundation for many of the technologies that followed. Steam power not only revolutionized industry but also society, transforming economies, improving standards of living, and contributing to the birth of the modern world. Today, steam engines are largely confined to historical preservation and niche applications, but their profound influence on engineering, transportation, and energy generation continues to resonate across multiple industries.
The story of the steam engine is far from just a tale of the past—it’s one that continues to inspire modern engineering and innovation. While steam power no longer dominates industrial or transportation sectors in the same way it did during the Industrial Revolution, its legacy still holds significant value in the realms of both historical significance and practical applications.
The most prominent legacy of the steam engine is its role in facilitating the transition from manual labor and animal power to mechanized production. As steam engines enabled factories to operate efficiently, they played a fundamental role in the rapid industrialization that spurred economic growth and technological advancement. This shift led to the creation of urban centers as industries concentrated around steam-powered machinery, which offered unprecedented efficiency in production. Entire economies, particularly in Europe and North America, were transformed as steam engines powered everything from cotton mills to ironworks, shaping the rise of modern manufacturing processes.
Moreover, the steam engine spurred advances in engineering, fostering the development of new materials, machine tools, and techniques for precision manufacturing. This foundation laid the groundwork for subsequent innovations, including the electric motor, internal combustion engines, and even nuclear power generation. The skills and knowledge gained from designing, operating, and improving steam engines contributed to the development of broader engineering fields, impacting everything from aerospace to automotive engineering.
In addition to the fields of manufacturing and transport, the steam engine’s influence is felt in the evolution of power generation. The steam turbine, an invention that built upon the principles of the steam engine, remains a cornerstone of electric power generation. Despite the rise of more energy-efficient and cleaner technologies in recent decades, steam turbines continue to play a role in power plants worldwide, particularly in coal, nuclear, and natural gas plants. These turbines convert thermal energy into mechanical energy, driving generators that produce electricity on a massive scale. This is a direct legacy of steam power, which continues to serve as a workhorse of the energy industry.
In the transportation sector, while steam locomotives have largely been replaced by diesel and electric engines, steam-powered trains are still celebrated and preserved for their historical value. Many heritage railways across the globe operate steam locomotives, providing an educational experience about the technology that revolutionized transport during the 19th century. In addition, steam engines have found their niche in certain tourist destinations where rail enthusiasts and history buffs can experience the romance and power of these machines.
Though steam power has been replaced in many mainstream industries, it still finds a place in niche applications. For instance, in some remote areas or off-grid systems, where access to electricity or traditional fuel sources may be limited, small-scale steam engines have been explored as viable power sources. These engines can run on renewable resources, such as biomass, and can help provide essential energy for communities, workshops, or farms without reliance on fossil fuels.
In the modern era, steam engine designs continue to capture the imagination of those interested in alternative energy solutions. The principles of steam power are being revisited in the context of sustainability and green technologies. Concepts like solar thermal power plants use mirrors or lenses to concentrate sunlight to generate steam, which drives turbines to produce electricity. This is an example of how steam generation can be integrated with renewable energy sources to produce power in an eco-friendly manner. Geothermal energy, another renewable resource, often uses steam to drive turbines in power plants, demonstrating that steam’s role in energy generation is still very much alive in the modern energy landscape.
Furthermore, the steam engine’s mechanical simplicity has made it an appealing topic for makers, tinkerers, and hobbyists who enjoy building and experimenting with old technologies. DIY steam engine kits and designs are popular among people fascinated by retro engineering and model-making. These miniature steam engines are often used in educational contexts to teach about the principles of thermodynamics and energy conversion, continuing the tradition of steam engines being tools of discovery and learning.
In the broader context of sustainable development, the legacy of steam engines is now being integrated with the development of hybrid energy systems. By combining steam technology with renewable energy solutions such as solar, wind, and biomass, modern innovations in steam power are being used to create more efficient, decentralized energy systems. For example, in emergency power generation and remote area operations, the use of modular steam-powered generators could prove to be a low-cost, flexible solution.
The advancements in materials science also play a role in reviving interest in steam engines. Modern alloys and composites could be used to build lighter, more durable steam engines that are capable of higher efficiency than their 19th-century predecessors. This evolution in materials could allow steam engines to find use in specialized industrial processes or even contribute to mobile power generation in environments where access to electricity is limited.
Lastly, while steam engines may no longer be the cutting-edge technology they once were, their historical significance cannot be overstated. The impact of steam power on the development of modern industrial society is immense, laying the foundation for technologies and systems that have shaped the world we live in today. In fact, many of the innovations that we now take for granted, such as electricity, automobile transportation, and mass production, owe their origins to the humble steam engine. The development of steam technology was the spark that ignited the Industrial Revolution, and its influence continues to reverberate throughout modern technology, culture, and the global economy.
The steam engine, once a symbol of industrial power, remains an enduring symbol of human ingenuity and progress, demonstrating how early inventions continue to influence and shape the future.
How to Optimize Your Steam Engine for Peak Performance
Optimizing your steam engine for peak performance involves a combination of mechanical tuning, thermal efficiency improvements, and regular maintenance. Whether you’re working with a vintage model, a custom-built engine, or a modern industrial steam unit, small adjustments and informed upgrades can result in major gains in output, reliability, and fuel economy. The first key factor is steam quality—dry, high-pressure steam is far more effective than wet or low-pressure steam. Ensuring your boiler is properly sized and insulated helps maintain consistent steam pressure and minimizes energy loss. Using a superheater, where applicable, can increase steam temperature and thus efficiency, allowing the engine to extract more work from each pound of steam.
Another critical area is valve timing and cut-off control. Adjusting the timing of valve events allows you to better control steam admission and exhaust, which directly impacts power output and efficiency. Shorter cut-off settings are ideal for light loads as they use less steam, while longer cut-offs provide more torque when needed. Variable cut-off mechanisms, such as those in compound or Corliss engines, allow for real-time optimization depending on load conditions. Additionally, make sure the valves and piston rings are properly seated and lubricated to reduce mechanical losses.
Insulation and heat recovery also play a major role. All steam lines, cylinders, and the boiler itself should be well insulated to prevent heat loss. Recovering waste heat from the exhaust steam using a feedwater heater or economizer increases overall system efficiency. Similarly, using a condenser at the exhaust side of the engine can significantly boost thermal efficiency by reducing back pressure, thereby enabling the engine to extract more energy from the steam.
Lubrication and friction reduction are often overlooked but essential to peak performance. Using high-quality, steam-rated lubricants reduces wear and energy losses in bearings, pistons, and other moving parts. Keep all moving components clean, aligned, and free of carbon or scale buildup. Speaking of which, water quality is another crucial factor—use treated feedwater to prevent scaling and corrosion in the boiler and steam passages. Scale build-up not only reduces heat transfer but can also lead to pressure drops and mechanical failures.
From a control and automation standpoint, integrating modern sensors and instrumentation can help you monitor steam pressure, temperature, engine speed, and load in real time. This data can be used to fine-tune your engine operation and anticipate maintenance needs before problems arise. Even on older engines, retrofitting with digital gauges or analog sensors can give you a clearer picture of what’s going on internally.
Regular inspection and maintenance routines are essential. Check for leaks, cracks, worn seals, and ensure that all fittings are tight. Periodic inspection of the boiler, safety valves, and piping not only maintains performance but ensures safe operation as well. Performing routine blowdowns on your boiler helps remove sediment and impurities, which can otherwise clog valves and reduce efficiency.
For larger or industrial applications, consider upgrading components like turbines, injectors, and governor systems with more efficient modern alternatives. Even a small upgrade in boiler efficiency or turbine design can lead to significant long-term savings. Also, ensure your load profile is well matched to the engine’s design; running a large engine under low load continuously is inefficient, just as pushing a small engine past its capacity can reduce lifespan and output.
In essence, achieving peak performance from your steam engine is about balancing thermal efficiency, mechanical reliability, and smart monitoring. With thoughtful design tweaks, consistent upkeep, and a clear understanding of your engine’s operational needs, you can dramatically increase output while reducing fuel consumption and mechanical stress. Whether you’re powering a factory, a generator, or a custom-built steampunk marvel, the same principles of thermodynamics and good engineering apply.
This process of optimization isn’t just about maximizing numbers on a gauge—it’s about creating a smooth, responsive, and efficient machine that works in harmony with its environment and load demands. A well-optimized steam engine will not only consume less fuel or water, but will also operate more quietly, start up more reliably, and require fewer emergency repairs over time. The cumulative effect of minor improvements—like tighter piston ring seals, cleaner steam lines, or better insulation—can translate into major performance gains when compounded across daily operation.
The key is paying attention to the nuances. For instance, how quickly does your engine respond to a sudden load change? Does it bog down or pick up cleanly? How stable is your boiler pressure under various loads? Are you seeing excessive condensate in the cylinder or exhaust? These subtle signs often tell a bigger story. Tuning your engine should always be an iterative process: observe, tweak, test, repeat. A good steam engineer develops an intuitive sense of how the system behaves, and can often detect performance drops by sound, vibration, or the rhythm of the exhaust beat.
Fuel type and combustion quality play an important role too. Whether you’re burning coal, wood, oil, or biomass, maintaining a clean, consistent fire with proper draft control ensures that heat is being effectively transferred into the boiler rather than wasted up the flue. Poor combustion leads to incomplete heating, which in turn creates low-quality steam and deposits soot or clinker in the boiler tubes, reducing efficiency and increasing wear. Automated or mechanically assisted firing systems can help regulate this in larger setups, but even hand-fired engines benefit greatly from proper stoking techniques and draft tuning.
It’s also worth considering the broader energy ecosystem around the engine. If your steam engine is part of a co-generation system, where both mechanical work and heat are utilized (for instance, powering machinery while also heating a facility), efficiency isn’t just about the engine itself—it’s about how waste heat and energy are used elsewhere. Capturing exhaust steam for heating or converting it to hot water means less energy wasted and more value extracted from every BTU of fuel.
Ultimately, optimizing your steam engine is about understanding the relationship between heat, pressure, motion, and resistance. It’s a conversation between fire and water, metal and motion. Each component, from the firebox to the flywheel, contributes to the symphony of operation. A finely tuned engine doesn’t just perform well—it feels alive. It responds when called upon, and rests quietly when the job is done. That kind of performance doesn’t come by accident; it comes from attention, care, and a deep respect for the machine and the forces it harnesses.
When a steam engine is operating at peak efficiency, you’ll notice more than just smooth mechanics and steady power—there’s a certain elegance in how everything comes together. The engine breathes, in a way, with rhythmic precision. You can hear it in the even cadence of the exhaust, feel it in the steady vibration of the frame, and see it in the absence of excessive steam leaks or jolting motions. This level of refinement isn’t just about mechanical parts working properly—it’s about harmony between the fuel, water, pressure, and load.
One of the often-underappreciated aspects of optimizing a steam engine is environmental adaptability. Different climates, altitudes, and even water sources can impact performance. In humid environments, for instance, managing condensation becomes more important. In high-altitude areas, boiler pressure dynamics and draft behavior may shift due to lower atmospheric pressure. These aren’t just theoretical issues—they affect real-world performance. The most optimized engines are those that have been adapted thoughtfully to their operating context, whether that means modifying boiler controls, insulating more aggressively, or recalibrating valve timing for slightly different pressure curves.
Sound tuning is another subtle but powerful indicator of engine health and optimization. An experienced operator can detect if the timing is slightly off, if there’s blow-by around the piston, or if exhaust steam isn’t fully expanding—all just by listening. It’s this deep sensory relationship between operator and machine that makes steam so compelling even today. It’s not just about numbers or output—it’s also about feel. An engine that “feels right” usually is right.
As technology continues to evolve, there’s also potential for blending traditional steam engines with modern digital monitoring tools. Compact sensors, microcontrollers, and software analytics can give you deeper insight into performance trends, fuel usage, and maintenance patterns. These tools don’t need to replace the art of operation, but they can supplement it—offering data that helps confirm intuition, or spotting issues before they become expensive problems.
And then there’s the human element. Even the best-built and finely tuned steam engine is only as good as the hands and eyes that manage it. Skill, patience, and experience make a tremendous difference. Optimizing a steam engine isn’t a one-time task—it’s an ongoing relationship. Like a musical instrument, it needs to be played, adjusted, and respected. That’s what turns a piece of machinery into something almost poetic—a living symbol of precision, power, and the enduring relevance of well-crafted engineering.
Streamlining Steam Engine Production with Automation
Streamlining steam engine production with automation brings a significant transformation to what was once a labor-intensive, craft-driven process. Modern manufacturing technologies now allow for faster, more precise, and more cost-effective production of steam engines—without compromising on quality or reliability. By incorporating automated CNC machining, robotics, and digital design tools, manufacturers can standardize critical components like cylinders, pistons, valve gears, and flywheels with incredibly tight tolerances, improving both performance and interchangeability.
Computer-aided design (CAD) and computer-aided manufacturing (CAM) platforms enable engineers to model entire steam engine assemblies down to the smallest gasket or bolt. This reduces design errors, speeds up prototyping, and ensures each part fits perfectly in the broader system. Automation also simplifies custom configurations for different applications—whether for marine use, industrial backup systems, or small-scale renewable energy projects—by allowing modular component production and flexible assembly lines.
Automated robotic arms can handle repetitive and heavy tasks such as casting preparation, part polishing, welding of boiler components, or assembling crankshafts, freeing skilled workers to focus on quality assurance, testing, and innovation. These systems reduce human error, speed up production cycles, and improve workplace safety by minimizing exposure to high-heat or high-pressure fabrication environments.
3D printing is increasingly being used to produce complex geometries for non-critical components, rapid prototypes, and tooling jigs, allowing steam engine builders to iterate designs more quickly and with lower upfront cost. Combined with IoT-enabled quality control systems—like laser scanning, thermal imaging, and real-time vibration analysis—automation helps identify inconsistencies or defects early in the production process, increasing yield and reliability.
With demand for custom steam engines in niche markets like heritage rail, green energy, and industrial redundancy systems, automation makes it feasible to produce short runs efficiently. Automated scheduling, inventory tracking, and predictive maintenance systems ensure a smoother supply chain and reduce downtime, making steam engine manufacturing more scalable and sustainable.
Ultimately, automation doesn’t replace craftsmanship—it amplifies it. The traditional knowledge of metallurgy, thermodynamics, and mechanical behavior is still essential. But when paired with modern manufacturing tools, that knowledge translates into cleaner builds, better engines, and a production process that meets both legacy performance expectations and modern efficiency demands.
As automation becomes more integrated into steam engine production, the entire lifecycle of the engine—from concept to commissioning—becomes more efficient and precise. This means fewer delays, less waste, and tighter quality control at every step. Imagine a production floor where CNC machines are continuously fabricating high-tolerance valve components while a digital twin of the entire engine is being tested virtually for stress points and thermal expansion, even before a single part is physically assembled. That’s not just faster—it’s smarter.
Automated production also makes it easier to maintain consistency across batches. One of the historical challenges in steam engine manufacturing was variation—minor differences in fit, finish, or alignment from one unit to the next. With today’s automated measuring systems and process controls, every bore, thread, and seat can be machined to identical specifications, ensuring that replacement parts and upgrades can be dropped into existing engines without modification. This kind of repeatability was once impossible with purely manual methods, and it’s especially useful for industries requiring dependable long-term performance under demanding conditions.
There’s also a significant impact on workforce efficiency. Instead of a large team of machinists, welders, and assemblers working long hours on a single build, a leaner, more specialized team can manage a wider range of tasks with the help of programmable machinery. Skilled technicians shift their focus to overseeing automated systems, troubleshooting issues, and applying their knowledge where it adds the most value—like interpreting data trends or fine-tuning prototypes.
Automation even extends into the testing and commissioning phase. Engines can now be hooked up to sensor arrays and diagnostic systems during their first test runs, generating precise performance data in real time. Pressure curves, thermal efficiency, vibration frequencies, and torque delivery are captured digitally, allowing engineers to make data-backed adjustments. This creates a feedback loop between design and performance that improves every generation of engine.
With the right infrastructure, manufacturers can even track each engine beyond delivery—monitoring wear patterns, fuel usage, and operational cycles. This kind of telemetry, once reserved for high-end aerospace or automotive systems, is now available in steam technology thanks to integration with smart control systems. It opens the door to predictive maintenance models, where parts are replaced based on actual wear rather than arbitrary schedules, minimizing downtime and maximizing uptime.
All of this ultimately leads to a redefinition of what steam engine production can be. It’s no longer a slow, one-at-a-time process bound by hand tools and blueprints on paper. It’s a fluid, adaptable, and intelligent system that honors traditional engineering principles while embracing the tools of modern manufacturing. This convergence doesn’t just preserve steam technology—it makes it more viable, more sustainable, and more competitive in today’s energy-conscious, performance-driven world.
The integration of automation also opens the door to collaboration and innovation on a global scale. With cloud-based design systems and digital fabrication tools, teams in different parts of the world can co-develop engine components, simulate system behaviors under diverse environmental conditions, and iterate rapidly based on real-time data. This digital interconnectedness fosters not only faster development but also the cross-pollination of ideas—where insights from the aerospace sector, for example, might inform improvements in steam valve geometry or thermal insulation strategies.
In this evolving landscape, manufacturers can adopt just-in-time production strategies, reducing the need for extensive warehousing and minimizing capital tied up in inventory. Components are made as they’re needed, to exacting specifications, and fed directly into the assembly process with minimal delay. The ability to track each part through barcode scanning or RFID tags ensures full traceability, from raw material to final engine installation, which is a crucial factor in industries where safety and compliance are critical.
Automation also supports the development of hybrid systems, where steam engines are paired with other technologies to optimize energy use. For example, a steam engine might work alongside solar or wind systems in microgrids, kicking in during periods of low renewable output. In such setups, digital controls enabled by automation help regulate load sharing, system synchronization, and response times. These hybrid configurations wouldn’t be as seamless or responsive without the precision and adaptability provided by modern automation systems.
Even education and training benefit from this evolution. Virtual reality and augmented reality can be used to simulate engine assembly, maintenance, and operation, giving engineers and technicians hands-on experience without needing access to a physical machine. These immersive training tools not only reduce the learning curve but also enhance safety by allowing users to experience potential failure scenarios in a controlled environment.
By removing repetitive tasks and allowing more human attention to be focused on design, innovation, and refinement, automation turns steam engine production from an artisanal process into a scalable, future-ready enterprise. And perhaps most importantly, it ensures that steam technology remains not just a historical curiosity or a niche application, but a relevant, reliable, and increasingly green option in a world that demands both performance and sustainability. Through automation, the steam engine steps confidently into the 21st century—not as a relic, but as a reinvented powerhouse.
Reconditioned Steam Engines
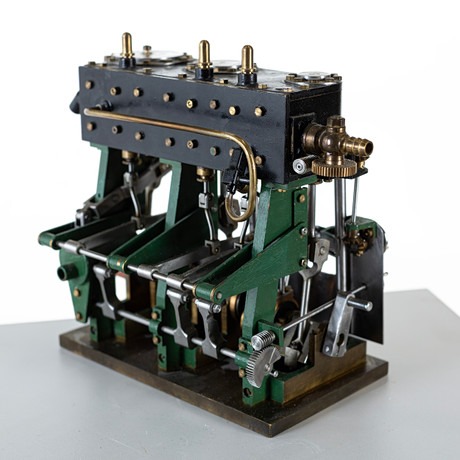
Reconditioned steam engines offer a unique balance between historical craftsmanship and modern performance. These are not just restored antiques—they’re revitalized machines that have been carefully dismantled, inspected, and upgraded to meet today’s operational standards while retaining their original integrity. The process of reconditioning typically begins with a complete teardown, where every component is evaluated for wear, corrosion, cracks, and fatigue. Parts that are beyond service limits are either replaced with exact replicas or machined anew using modern materials that often exceed the strength and durability of the originals.
The goal is to preserve the heart and soul of the original engine while bringing it up to spec for safety, reliability, and in many cases, regulatory compliance. Bearings are re-babbitted or replaced, pistons are re-machined for proper seal and balance, and valve gear is recalibrated to ensure smooth and efficient timing. Cylinders may be honed or sleeved to restore proper clearances. Gaskets, seals, and fasteners are replaced with modern equivalents that improve pressure retention and reduce maintenance needs.
Boilers, the most critical and heavily regulated part of any steam system, undergo rigorous hydrostatic testing and, if necessary, re-tubing or retubing. In some cases, original boilers are replaced entirely with new ones built to current pressure vessel codes while preserving the external appearance and mounting compatibility of the old unit. Safety valves, injectors, and feedwater systems are typically updated to more efficient, more responsive models.
Reconditioned engines are particularly attractive for applications where cost, aesthetics, or heritage are important. Heritage railways, agricultural expos, steampunk events, and even small-scale power generation projects often seek out these engines because they combine the character of the past with the functionality needed for present-day use. And since much of the engineering knowledge from the steam era remains valid today, many reconditioned engines continue to run for decades with proper upkeep.
The value proposition is strong: a reconditioned engine usually costs far less than a new build, especially when it comes to custom or large-scale models. Lead times are shorter, too, since many of the major castings and parts already exist. What’s more, each reconditioned unit carries a story—whether it powered a sawmill a century ago or ran on a narrow-gauge line in the mountains, there’s history built into the metal.
What’s changed is how these engines are reconditioned. Instead of relying purely on hand-fitting and visual inspection, modern shops use ultrasonic testing, laser measurement, digital modeling, and CNC machining to ensure tolerances and alignments are perfect. The result is a steam engine that not only runs like new—it often runs better than new, with less vibration, higher efficiency, and tighter controls.
In a world where sustainability and reuse are gaining more attention, reconditioning steam engines also aligns with environmental values. It keeps valuable materials in use, avoids the need for energy-intensive new casting and forging, and offers a way to celebrate and extend the life of beautifully engineered machines. Whether used for practical power or nostalgic display, a reconditioned steam engine is a testament to the enduring relevance of good design, smart repair, and mechanical artistry.
Reconditioned steam engines also provide an excellent opportunity for those in the industrial or niche markets to experience the charm and reliability of older technology while avoiding the often-high costs and extended timelines of building a new engine. For many, this is particularly appealing when looking to power smaller facilities, generate backup power, or provide reliable mechanical energy in remote areas. These engines often offer a level of self-sufficiency that can be difficult to achieve with more modern technologies.
In addition to industrial applications, reconditioned steam engines are often used in educational settings, offering students and apprentices the chance to work on and understand the mechanics of a bygone era while applying contemporary knowledge to improve efficiency. Museums, historical sites, and even some small tourist operations also make use of these engines, as they provide a living demonstration of engineering ingenuity and the transition from the steam era to modern power systems.
A key aspect of reconditioning is the customization options available. Many reconditioned engines are modified or upgraded to meet the specific needs of their new owners. For example, modern controls, instrumentation, and safety systems can be added to ensure that the engine runs optimally within current safety regulations. This kind of customization ensures that while the engine retains its historic charm, it also benefits from the safety, automation, and monitoring tools available today.
In some cases, older steam engines are reconditioned for very specialized applications. For instance, engines originally designed for locomotive service may be repurposed to power generators, pumps, or even agricultural equipment. This type of repurposing not only gives new life to engines but also makes them more adaptable to contemporary needs, especially in areas where electricity may not be readily available or where there is a desire to maintain low-tech, off-grid power sources.
Beyond just the engine itself, the broader infrastructure that supports the reconditioned steam engines often undergoes modernization. For example, boiler systems may be fitted with newer, more efficient fuel burners, reducing emissions and improving combustion efficiency. Similarly, auxiliary systems like condensate return lines, feedwater heaters, and exhaust systems are frequently updated to improve overall thermal efficiency, making the steam engine not just a relic but a functional component of a larger, more energy-efficient system.
Finally, the appeal of reconditioned steam engines is not just technical—it’s emotional. There’s something special about keeping an old piece of machinery running, especially when that machine has a history or a story attached to it. Whether it’s an engine that powered a local factory or one that served a particular community, the process of reconditioning and reviving it connects people to the past. It speaks to a time when craftsmanship, mechanical ingenuity, and labor were the foundation of modern industry. For those who understand and appreciate these machines, a reconditioned steam engine isn’t just a machine—it’s a piece of living history, kept alive through care, skill, and dedication.
Why Choose Steam Engines for Industrial Efficiency
Choosing steam engines for industrial efficiency can seem counterintuitive in a world dominated by electric motors, gas turbines, and other modern technologies. However, steam engines bring a unique set of benefits that continue to make them a viable and often efficient choice for certain industrial applications, particularly in settings where reliability, flexibility, and sustainability are priorities.
One of the primary advantages of steam engines is their ability to run on a wide variety of fuels, including coal, biomass, oil, and even waste materials. This makes them incredibly versatile and adaptable, especially in industries where access to clean electricity or natural gas is limited or costly. In remote or off-grid locations, steam engines offer a reliable energy source when other options might not be feasible.
Steam engines are also known for their longevity and robustness. When properly maintained, they can continue to operate efficiently for decades, providing a long-term return on investment. This is particularly valuable for industries that rely on heavy machinery running 24/7, such as in mining, agriculture, and manufacturing, where durability and consistent performance are critical. Unlike some modern engines, steam engines tend to be simpler in design, which can make them easier to repair and maintain with readily available parts, further extending their operational lifespan.
Additionally, steam engines can be highly efficient in terms of thermal energy conversion, especially when combined with cogeneration systems. Cogeneration, or combined heat and power (CHP), allows steam engines to produce both mechanical power and useful thermal energy, which can be used for heating or additional processes within the same facility. This dual-output capability makes steam engines especially attractive in industries with high thermal energy demands, such as chemical manufacturing, paper mills, or food processing plants.
Another key advantage is their scalability and adaptability. Steam engines can be configured to meet a wide range of power requirements, from small-scale applications in workshops or remote locations to large, industrial-scale operations in factories or power plants. This flexibility means that businesses can invest in steam-powered systems tailored to their specific needs, whether that’s a small, standalone engine or a large, multi-engine setup integrated into a broader industrial infrastructure.
From a sustainability perspective, steam engines offer an environmentally friendly option when fueled by renewable sources. Biomass-fired steam engines, for example, contribute to a closed-loop energy system where organic waste is converted into usable power, reducing the need for fossil fuels. Similarly, waste-to-energy steam plants can help mitigate waste disposal issues by turning industrial byproducts or municipal waste into power, providing both environmental and cost benefits.
Finally, there’s a certain level of reliability and predictability in steam engine operations that appeals to industries requiring high levels of consistency. Steam engines can maintain stable output over long periods, with fewer interruptions from fluctuating fuel prices or grid instabilities. In industries where downtime costs are significant, such as in mining or manufacturing, having a reliable steam engine can be a key factor in maintaining production schedules and minimizing losses.
While modern technology has introduced numerous alternatives, steam engines remain a competitive option for industrial efficiency in specific contexts. Their ability to operate on diverse fuel types, offer dual-purpose energy output, provide long-term reliability, and contribute to sustainability makes them an attractive choice for certain industries that value resilience, adaptability, and efficiency over pure innovation or trend-driven solutions.
Moreover, steam engines are relatively low-maintenance compared to some modern technologies. Their mechanical simplicity means there are fewer complex electronic components that can fail or require costly repairs. While they do require regular attention to components like the boiler, valves, and bearings, these are parts that have been well understood for centuries, with clear procedures for care and upkeep. This makes steam engines particularly appealing in industries where downtime can lead to significant financial losses or where specialized technical skills may be harder to come by.
In certain applications, steam engines can also be integrated into systems with other energy sources, creating hybrid setups that increase efficiency. For example, in a facility that utilizes both renewable energy sources and a steam engine, the steam engine can act as a backup system or be used during times when renewable energy production is low. The ability to seamlessly integrate with other power sources increases operational flexibility, ensuring that energy needs are met consistently without relying on a single source.
Steam engines can also offer more predictable and consistent power delivery compared to some renewable energy systems, like wind or solar, which can be intermittent. This predictability is especially crucial in industries that require constant, uninterrupted power. In such scenarios, steam engines act as a stabilizing force, providing a steady baseline of energy that can be supplemented by other power sources when needed.
Another notable factor is their relatively low environmental footprint, especially when compared to more polluting fossil fuel alternatives. In an era when industries are under increasing pressure to reduce their carbon footprints, steam engines powered by biomass, for example, contribute to carbon neutrality. The process of converting organic waste into steam energy reduces the need for landfills and helps manage waste while also generating power.
Additionally, as industries seek ways to reduce their reliance on external energy grids, steam engines can be part of a self-sufficient power solution. In remote locations or areas with unreliable electrical infrastructure, steam engines provide an independent power generation method that allows operations to continue smoothly. For industries like agriculture, mining, or remote manufacturing, being able to generate their own power using locally available fuel sources is a major advantage.
As energy costs continue to rise, businesses are increasingly turning to steam engines as a cost-effective alternative. With the rising price of natural gas and electricity in certain regions, investing in a steam engine can offer long-term savings. Additionally, steam engines are often less affected by energy price fluctuations, as they can be fueled by a wide range of sources, many of which are more stable in price or even waste materials that would otherwise incur disposal costs.
The adaptability and versatility of steam engines make them a practical solution for many industries seeking to balance efficiency, sustainability, and cost-effectiveness. While they may not be the first choice in every application, their ability to deliver dependable, long-term power with relatively low maintenance makes them an attractive option for businesses that require resilience and flexibility in their energy solutions.
In addition to their practicality and adaptability, steam engines also offer a level of control that other power generation systems may not provide. Operators can fine-tune steam pressure, temperature, and flow rates, adjusting the system’s performance to meet fluctuating demands or specific operational requirements. This level of customization can be crucial in industries that need precise control over energy output, such as in chemical processing, manufacturing, or even in certain research and development environments where consistency is key.
Furthermore, steam engines are inherently versatile in the types of mechanical processes they can drive. Beyond electricity generation, steam engines can be used to directly power mechanical equipment, such as pumps, compressors, and crushers, offering a reliable and efficient power source for a variety of industrial applications. This flexibility enables industries to integrate steam power into various stages of their operations, creating a more streamlined and self-contained energy system.
Another advantage is the robust energy storage capabilities of steam systems. Steam engines can store thermal energy in the form of pressurized steam, which can be held in reserve and released as needed. This makes it possible to store energy produced during off-peak times and use it during periods of high demand, providing a buffer against sudden energy shortages or fluctuations in supply. This energy storage potential helps improve the resilience of industrial operations, allowing them to continue functioning smoothly even in the face of changing conditions.
The use of steam engines in industrial efficiency also aligns with current trends in sustainability and circular economy practices. By utilizing waste heat recovery systems, steam engines can make use of otherwise wasted energy from industrial processes, reducing overall energy consumption and improving the efficiency of the entire system. In industries where high temperatures are involved—such as metalworking or glass manufacturing—steam engines can harness waste heat to generate additional power or provide heating for other processes, turning what would be waste into a valuable resource.
The ability to repurpose existing steam engines also contributes to sustainability. Rather than discarding outdated or inefficient systems, reconditioning and upgrading steam engines allows industries to extend the lifespan of these machines, reducing the environmental impact of manufacturing new engines. By combining modern engineering practices with older technology, companies can meet the growing demand for sustainable energy solutions without sacrificing the performance or reliability of their equipment.
As industries continue to focus on reducing their environmental impact and improving energy efficiency, steam engines present a viable and often overlooked solution. Their ability to operate on a variety of fuels, offer high energy efficiency, provide reliable and consistent power, and adapt to the unique needs of different industries makes them an enduring option in the pursuit of industrial sustainability. While newer technologies may dominate the market, the benefits of steam engines remain clear, especially for industries seeking an efficient, flexible, and cost-effective energy source.
Steam Engine for Cogeneration Systems
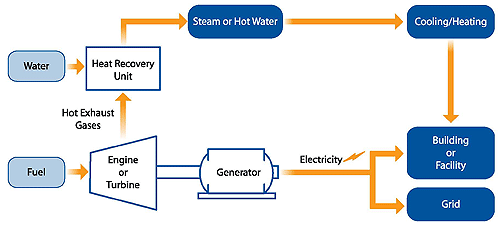
Steam engines play a vital role in cogeneration systems, where they are used to simultaneously produce both electrical power and useful thermal energy, typically in the form of steam or hot water. This process, known as combined heat and power (CHP), increases the overall efficiency of power generation, as it makes use of both the electrical and thermal energy produced by the engine. Unlike traditional power generation methods, which often waste a significant portion of the thermal energy as heat, steam engines in cogeneration systems can capture and utilize that heat for other industrial processes or heating needs.
One of the primary benefits of using steam engines in cogeneration systems is their ability to operate on a wide variety of fuels, including renewable resources like biomass, municipal waste, or even byproducts from industrial processes. This makes them particularly valuable in industries that generate their own waste heat or in facilities located in areas where conventional electricity sources are either expensive or unreliable. In these contexts, steam engines can be integrated into existing operations to recover waste heat and reduce dependence on external power grids.
In a cogeneration system, steam engines are typically paired with a boiler, which generates the steam used to drive the engine. The engine, in turn, powers a generator to produce electricity. The waste heat from the engine, which would otherwise be lost, is captured and utilized for heating purposes—either to provide hot water, space heating, or to power additional industrial processes such as drying, distillation, or chemical reactions. This dual-use approach can significantly reduce energy consumption, lower operating costs, and decrease greenhouse gas emissions by making more efficient use of the fuel consumed.
The energy efficiency of cogeneration systems can be quite high—often exceeding 80%, compared to the typical efficiency of traditional power plants, which may only reach 30% to 40%. This efficiency is particularly important in industries with high energy demands, such as paper mills, chemical plants, food processing facilities, and manufacturing plants. For these industries, cogeneration systems powered by steam engines can provide a reliable, cost-effective energy solution, enabling them to meet both their electrical and thermal energy needs while minimizing waste and reducing environmental impact.
In addition to industrial applications, steam engines in cogeneration systems are also used in district heating networks, where excess thermal energy is distributed to nearby buildings for heating. This provides a sustainable alternative to traditional heating methods, such as gas-fired boilers, and helps reduce emissions associated with residential and commercial heating.
The scalability of steam engines also allows for flexibility in cogeneration system design. Small-scale steam engines can be used in localized cogeneration setups, such as those in factories or remote areas, while larger, more powerful steam engines can be employed in centralized systems that serve large industrial parks, communities, or even municipalities. This adaptability makes steam engines a viable option for a wide range of applications, from small, off-grid systems to large, complex industrial operations.
Furthermore, steam engines in cogeneration systems can be easily integrated with other technologies, such as solar or wind power, to create hybrid energy systems. In such setups, steam engines can provide a steady, reliable base-load power supply when renewable energy sources are intermittent, helping to stabilize the grid and ensure a continuous power supply. This combination of renewable and thermal energy sources is especially appealing for industries seeking to reduce their carbon footprint and improve energy efficiency.
The use of steam engines in cogeneration systems is also beneficial from an economic standpoint. By utilizing both electricity and thermal energy, businesses can reduce their reliance on external energy providers, resulting in lower energy bills. Additionally, some regions offer financial incentives, such as tax credits or subsidies, for businesses that implement CHP systems, further improving the cost-effectiveness of steam engine-powered cogeneration solutions.
In summary, steam engines in cogeneration systems provide an efficient and sustainable energy solution for industries that require both electrical and thermal energy. By capturing and reusing waste heat, these systems improve overall energy efficiency, reduce fuel consumption, and lower operational costs. With their versatility, scalability, and ability to run on a variety of fuels, steam engines remain an important tool in the pursuit of energy-efficient, environmentally-friendly industrial practices.
Moreover, the integration of steam engines into cogeneration systems helps improve energy security, especially in regions with unreliable electricity grids or where energy supply disruptions are common. In these cases, steam engines can offer a more stable and independent source of power, reducing the vulnerability of industries to external power outages or energy price fluctuations. This autonomy is crucial for industries that rely on continuous operation, where even short periods of downtime can lead to significant losses in production or productivity.
In addition, steam engines provide a high degree of control over energy production. The flexibility to adjust steam pressure and temperature allows operators to fine-tune performance based on immediate needs, whether it’s for increased electricity generation or meeting thermal demands. This adjustability ensures that the system remains optimized under varying conditions, which is particularly useful in industries with fluctuating energy needs. For example, during peak production times, the system can prioritize electricity generation, while during off-peak times, it can focus on providing thermal energy for heating or other processes.
Another important consideration is the environmental benefit of steam engine-based cogeneration systems. By reducing reliance on separate, dedicated power generation and heating systems, these setups help minimize the overall carbon footprint of an operation. Steam engines fueled by biomass, for example, can create a closed-loop system, where organic waste materials are converted into usable power, significantly lowering emissions compared to conventional fossil fuel-burning power plants. This aligns with the global push for decarbonization and the transition to greener energy solutions.
Additionally, with ongoing advancements in technology, the efficiency of steam engines in cogeneration applications continues to improve. Modern steam engines benefit from cutting-edge materials, enhanced combustion technologies, and sophisticated monitoring and control systems, all of which contribute to higher performance and lower emissions. These innovations further enhance the cost-effectiveness of steam engine-based cogeneration systems, making them even more appealing to industries looking for sustainable and efficient energy solutions.
The appeal of steam engines for cogeneration extends beyond just the industrial realm; they are also an excellent option for combined heating and power needs in district energy systems or commercial buildings. For example, universities, hospitals, and large residential complexes can integrate steam engine-based cogeneration systems to meet both their electricity and heating demands in a highly efficient manner. These systems help reduce dependency on external utility services while promoting sustainability, which is becoming an increasingly important factor for businesses and institutions looking to meet environmental goals and reduce operating costs.
In certain cases, steam engines in cogeneration systems can also contribute to local job creation, particularly when operated in rural or underserved areas. Maintaining and running a steam engine-based system requires skilled labor, from operators to engineers, which can offer new employment opportunities in areas where job prospects might otherwise be limited. Furthermore, the construction and maintenance of such systems can stimulate the local economy, especially when it involves sourcing materials and services locally.
Steam engines, though often viewed as outdated in the age of digital and electric technologies, provide a unique and highly efficient energy solution, especially when used in cogeneration systems. Their ability to simultaneously produce both power and useful thermal energy significantly boosts efficiency, reduces energy costs, and supports sustainability goals. Whether used in large industrial facilities, small-scale operations, or even for district heating and combined power generation, steam engines remain a vital option for industries and organizations seeking a reliable, cost-effective, and environmentally responsible energy source. As technology continues to evolve, the role of steam engines in cogeneration systems will likely expand, offering even more opportunities for energy optimization, cost savings, and environmental benefits.
Steam Engine Energy Recovery Systems
Steam engine energy recovery systems are designed to harness and reuse waste heat or energy that would otherwise be lost in industrial processes. These systems capitalize on the inherent properties of steam engines to capture thermal energy from exhaust gases, hot surfaces, or other sources of waste heat, converting it into usable energy. The goal is to improve overall system efficiency, reduce energy consumption, and minimize environmental impact by making the most out of every unit of energy input.
One of the most common ways that steam engines are used in energy recovery systems is through waste heat recovery. In these systems, the exhaust gases or steam from the engine, which are typically at high temperatures, are captured and redirected for use in other parts of the operation. For example, the hot exhaust gases may be passed through a heat exchanger to preheat water or air used in the process, reducing the need for additional energy input. This is especially valuable in industrial facilities where energy demands are high, and even small improvements in energy efficiency can result in significant cost savings.
In more advanced systems, a steam engine’s waste heat can be used to generate additional power, turning the heat into steam that drives additional turbines or engines. This is known as “cascade” or “multi-stage” energy recovery, where different stages of heat are captured and reused in progressively lower-temperature cycles. By doing so, the system extracts as much energy as possible from the exhaust and reduces the total fuel required to meet energy needs. This kind of system is commonly used in large-scale industrial settings like power plants or refineries, where efficiency is paramount, and the energy recovery potential is high.
Another form of energy recovery in steam engine systems involves the use of economizers and feedwater heaters. These devices capture waste heat from the exhaust gases and use it to preheat the feedwater entering the boiler. This reduces the amount of fuel needed to bring the water up to the necessary temperature, improving the overall efficiency of the steam engine system. Economizers and feedwater heaters are particularly beneficial in steam systems that operate under steady-state conditions, such as in continuous processes like chemical production, cement manufacturing, or paper milling.
Steam engines can also be paired with thermal storage systems as part of an energy recovery solution. These systems store excess heat generated during periods of high activity or low demand, and the stored heat can later be released when needed, such as during peak power demands. Thermal storage helps stabilize energy supply and can contribute to smoother, more reliable operations, especially in industries with fluctuating energy needs. For instance, in a factory that uses steam engines for heating, the stored thermal energy can be released when there’s a sudden increase in production or when energy demands exceed normal levels.
In some cases, steam engines are integrated into district heating systems, where the energy recovery system not only benefits the immediate facility but also provides excess heat to neighboring buildings or industries. This can be particularly useful in urban settings, where multiple buildings may benefit from a shared steam distribution network. By using steam engines to recover waste heat, these systems can lower the overall energy demand of the district, reduce heating costs for individual consumers, and lower carbon emissions across the community.
The integration of energy recovery systems in steam engines is also aligned with the push for sustainability and carbon reduction. By optimizing the use of thermal energy and reducing the consumption of primary fuels, these systems contribute to a circular economy where energy waste is minimized, and resources are used more efficiently. Furthermore, many industries and power plants are under increasing pressure to meet stringent environmental regulations, and energy recovery systems can help them do so by reducing emissions and lowering their carbon footprint.
The efficiency gains from energy recovery systems can result in lower operating costs, both through direct savings on fuel consumption and through reduced maintenance needs. By minimizing the amount of fuel required to generate the same amount of energy, facilities can save money on raw materials, and the reduced strain on engines and other components can extend the operational life of the system. This creates a win-win situation, where both economic and environmental benefits are realized.
As energy prices continue to rise and industries are increasingly held accountable for their environmental impact, energy recovery systems in steam engines will play a critical role in achieving energy efficiency goals. These systems can be retrofitted to existing steam engine setups or incorporated into new designs, making them a flexible solution for industries looking to maximize their energy utilization. The ability to recover energy, reduce fuel consumption, and lower emissions makes steam engine energy recovery systems a powerful tool for businesses looking to improve both their bottom line and their sustainability practices.
In addition to improving efficiency, energy recovery systems in steam engines help enhance the overall performance of industrial processes. By reducing energy losses, these systems ensure that the energy generated by the steam engine is utilized to its fullest potential. This is particularly beneficial in industries where high temperatures or large amounts of steam are required for processing, such as in food production, chemical processing, or metalworking. In these environments, minimizing waste heat and ensuring that as much energy as possible is retained and reused can be the difference between a profitable operation and one that struggles with high energy costs.
The adoption of energy recovery systems also helps industries meet increasingly stringent regulatory standards regarding energy consumption and emissions. As governments around the world implement policies to reduce carbon emissions and encourage the use of more sustainable energy practices, businesses that implement efficient energy recovery solutions may be able to benefit from tax incentives, grants, or other forms of financial assistance. In some cases, there may be specific environmental credits awarded to companies that integrate energy recovery technologies into their operations, further reducing their operational costs.
As industries increasingly focus on energy resilience and reducing their reliance on external energy sources, steam engine-based energy recovery systems are gaining attention as a reliable, cost-effective solution. These systems can be deployed in a wide range of applications, from small-scale operations to large industrial complexes. Their ability to capture and reuse waste heat reduces the need for additional fuel sources, which can be particularly valuable in areas with volatile energy prices or unreliable power grids. By relying on recovered thermal energy, businesses can insulate themselves from price fluctuations in traditional energy markets and avoid costly energy disruptions.
Another benefit of steam engine energy recovery systems is their contribution to long-term sustainability goals. The process of recovering and reusing waste heat is inherently environmentally friendly, as it helps reduce the amount of fuel needed for energy generation and cuts down on greenhouse gas emissions. This is a critical factor for industries aiming to meet global sustainability targets and reduce their environmental footprint. By embracing energy recovery, companies not only lower their operating costs but also contribute to the global effort to combat climate change.
Moreover, energy recovery in steam engine systems offers the potential for innovations in hybrid energy solutions. For example, steam engines can be paired with renewable energy sources, such as solar or biomass, to create a hybrid system that balances the intermittent nature of renewable energy with the reliability of steam power. In such setups, the steam engine can provide a consistent base load of energy, while the renewable energy source contributes to the system when available. This hybrid approach can help stabilize energy output, reduce reliance on fossil fuels, and increase overall system efficiency.
In the future, advancements in energy recovery technology could make these systems even more effective. Innovations in materials science, heat transfer techniques, and system design could lead to higher performance levels and greater energy savings. For example, newer, more efficient heat exchangers or better insulation materials could reduce thermal losses even further, while digital control systems could improve the accuracy with which energy recovery is optimized.
The growing focus on energy efficiency and sustainability is driving the demand for systems that can make the most of every unit of energy consumed. As such, steam engine-based energy recovery systems will likely continue to play an important role in the industrial sector, helping companies meet both their operational and environmental goals. With the right technology and implementation, these systems can contribute to significant cost savings, operational improvements, and reductions in carbon emissions, positioning them as a key part of the future of industrial energy management.
High-Pressure Steam Engines for Heavy-Duty Applications
High-pressure steam engines are critical for heavy-duty applications where large amounts of power and efficiency are required. These steam engines operate at significantly higher pressures than traditional low-pressure systems, allowing them to generate more energy from the same amount of fuel. By utilizing high-pressure steam, these engines can drive larger machinery, power industrial processes, and provide the high energy output needed for demanding operations, making them an essential component in sectors such as manufacturing, mining, chemical processing, and power generation.
The key advantage of high-pressure steam engines is their ability to produce greater mechanical power while maintaining a compact and efficient system. By increasing the pressure of the steam, the engine is able to convert more of the thermal energy into mechanical energy, enhancing the overall efficiency of the system. The higher pressure also allows the steam engine to deliver consistent power over longer periods of time, making it ideal for continuous operations in industries that require a reliable, non-intermittent power source.
In heavy-duty applications, high-pressure steam engines are commonly used to drive large mechanical systems such as compressors, pumps, turbines, and crushers. These machines require significant amounts of energy to operate effectively, and high-pressure steam engines are able to meet these demands by providing a robust and stable energy source. For example, in the mining industry, high-pressure steam engines can power large rock crushers or hoists that are essential for extracting and processing materials. Similarly, in large-scale manufacturing plants, these engines can drive equipment used for molding, shaping, and assembly processes that demand continuous, high-intensity power.
One of the significant benefits of high-pressure steam engines is their versatility. They can run on a variety of fuels, including coal, oil, natural gas, and biomass, which is important for heavy-duty applications where fuel availability and cost can vary. The ability to use alternative and renewable fuel sources, such as biomass or waste materials, makes high-pressure steam engines particularly attractive in industries that are looking to reduce their environmental footprint or meet sustainability targets.
High-pressure steam engines are also commonly found in power plants, particularly those that use steam turbines for electricity generation. In these settings, steam is produced at high pressures and temperatures, and it drives a turbine connected to a generator. The advantage of using high-pressure steam in power generation is that it allows for more efficient conversion of thermal energy into electricity, leading to higher output and better fuel utilization. This makes high-pressure steam engines ideal for large-scale power plants, where maximizing efficiency and minimizing fuel consumption are critical for profitability and environmental impact.
In chemical processing plants, high-pressure steam engines play an essential role in driving the reactors and other heavy equipment needed for various chemical reactions and separation processes. The high energy output is necessary to maintain the required reaction conditions, such as pressure and temperature, and to operate large distillation columns, evaporators, and other machinery. For these industries, the ability to maintain stable and high-energy outputs over extended periods is crucial to ensuring continuous production and meeting the high demands of modern chemical manufacturing.
Another area where high-pressure steam engines excel is in transportation, particularly in large ships and trains. Steam-powered locomotives, though largely replaced by diesel and electric engines, were once the backbone of industrial transportation and continue to serve as a model for high-pressure steam engine applications in heavy-duty transport. Modern high-pressure steam turbines are still used in marine vessels, where they generate electricity and drive the ship’s propulsion systems. The efficiency of high-pressure steam in marine engines allows for longer voyages and greater fuel economy, making them well-suited for long-distance travel.
Maintenance and durability are key considerations when utilizing high-pressure steam engines for heavy-duty applications. These engines are designed to withstand the stresses of operating at high pressures and temperatures, requiring advanced materials and robust construction to prevent wear and tear. Regular inspection and maintenance are necessary to ensure that the steam engine operates at peak performance and does not suffer from issues such as corrosion, scale buildup, or pressure drops, which can significantly reduce efficiency and damage components.
Despite their complexity, high-pressure steam engines provide a number of economic benefits in heavy-duty applications. Their high efficiency means that businesses can reduce their fuel consumption and operating costs, as more of the energy in the fuel is converted into useful power. Additionally, the long lifespan and reliability of high-pressure steam engines make them a cost-effective choice over the long term, particularly in industries that rely on continuous operation.
The integration of high-pressure steam engines into heavy-duty applications can also be a step toward achieving sustainability goals. With the ability to operate on renewable fuels and capture waste heat for energy recovery, these engines can help reduce overall greenhouse gas emissions and energy consumption. In some cases, waste heat from industrial processes or power generation can be captured and reused, further improving efficiency and minimizing environmental impact.
In conclusion, high-pressure steam engines are indispensable in heavy-duty applications due to their ability to produce large amounts of power efficiently. Whether in manufacturing, mining, chemical processing, power generation, or transportation, these engines provide the necessary energy to drive complex machinery and power demanding industrial processes. By maximizing energy conversion, utilizing renewable fuels, and providing reliable, continuous power, high-pressure steam engines remain a critical technology in heavy-duty applications, offering both economic and environmental benefits.
High-pressure steam engines continue to be integral to many industries, particularly as the demand for both high efficiency and sustainability grows. One of the ongoing trends in their application is the integration with advanced control systems that optimize their performance in real time. These systems monitor variables like steam pressure, temperature, and load demand, automatically adjusting engine output for maximum efficiency. This integration not only improves operational performance but also reduces the risk of overuse or unnecessary energy waste, which can significantly lower operational costs.
As industries move towards digitization and the adoption of Industry 4.0 technologies, high-pressure steam engines are also benefiting from advancements in sensor technology and predictive maintenance. Sensors placed throughout the engine can detect early signs of wear or malfunction, such as temperature fluctuations or pressure drops. This enables operators to address potential issues before they lead to costly breakdowns, improving the overall reliability and uptime of the engine. Predictive maintenance systems, driven by big data and machine learning algorithms, can forecast when components are likely to fail, allowing for more efficient scheduling of repairs and replacements.
Additionally, high-pressure steam engines are increasingly being paired with energy storage solutions in heavy-duty applications. For example, excess steam or energy that is generated during periods of low demand can be stored in thermal storage systems. These systems store the heat for later use, releasing it back into the system when energy demands increase. This approach reduces fuel consumption during peak periods and can improve the overall efficiency of a steam-powered system. In industries such as petrochemical manufacturing or steel production, where energy usage fluctuates based on production cycles, energy storage offers a cost-effective solution to managing these variations.
For industries looking to further reduce their environmental impact, high-pressure steam engines can be integrated into hybrid systems with renewable energy sources. In these hybrid setups, steam engines may work alongside solar, wind, or geothermal energy systems to provide a continuous and reliable energy supply. For instance, steam engines powered by biomass or waste-to-energy systems can complement renewable energy sources, creating a flexible energy grid that relies less on fossil fuels. These hybrid solutions can be especially beneficial in remote locations where access to conventional power grids is limited or unreliable.
Furthermore, high-pressure steam engines play a crucial role in the decarbonization of industries by facilitating the use of cleaner fuels. For example, industrial operations that traditionally rely on coal or oil can transition to cleaner, more sustainable energy sources such as hydrogen or biofuels. High-pressure steam engines can be adapted to operate on these alternative fuels, helping industries reduce their carbon footprint without sacrificing performance. This adaptability makes high-pressure steam engines a key enabler of the global transition to a low-carbon economy.
In the power generation sector, particularly in combined heat and power (CHP) or cogeneration systems, high-pressure steam engines can achieve even greater efficiency by simultaneously generating electricity and providing thermal energy for industrial processes. In these systems, the waste heat from electricity generation is captured and used for heating purposes, such as providing hot water for industrial processes or district heating systems. This form of energy recovery maximizes the overall energy output, reducing fuel consumption and lowering operational costs.
As high-pressure steam engines become more efficient and adaptable, they also contribute to enhancing energy security. By diversifying energy sources and enabling industries to generate their own power, these engines help reduce dependence on external energy suppliers. This is particularly important in regions where energy supply can be unreliable or subject to fluctuations in price. The ability to generate both power and thermal energy locally can improve energy resilience and provide industries with more control over their energy costs.
With global energy demands continuing to rise and environmental concerns growing more urgent, high-pressure steam engines are well-positioned to remain a crucial part of the industrial landscape. Their ability to provide reliable, high-efficiency energy, coupled with ongoing advancements in technology and sustainability practices, ensures that they will continue to be a go-to solution for heavy-duty applications for years to come.
In summary, high-pressure steam engines offer a versatile, efficient, and cost-effective solution for heavy-duty industrial applications, enabling industries to meet demanding power needs while enhancing energy efficiency, reducing operational costs, and supporting sustainability initiatives. The integration of advanced technologies, renewable fuels, energy storage, and hybrid systems further enhances their value, making them a key asset in the transition to a more sustainable, resilient energy future. Whether used in manufacturing, power generation, or other heavy-duty sectors, high-pressure steam engines will remain vital for industries seeking to optimize their energy usage and environmental performance.
Low-Pressure Steam Engines for Industrial Use
Low-pressure steam engines are commonly used in industrial applications where the demands for power generation or mechanical work are relatively lower compared to high-pressure systems. These engines operate at lower steam pressures, typically around 1 to 5 bars (14.5 to 72.5 psi), and while they do not generate as much power as high-pressure steam engines, they offer specific advantages, especially in smaller-scale operations or processes that do not require immense power output. The main benefits of low-pressure steam engines include their lower operational costs, simpler design, and versatility in a variety of industries.
One of the key advantages of low-pressure steam engines is their ability to operate efficiently with relatively low steam input. This makes them particularly suited for applications in industries where energy demand is moderate or where steam is needed for purposes such as heating, drying, or light mechanical work. These engines are often found in smaller industrial processes, such as food processing, textiles, and small-scale power generation. In these sectors, the energy needs may not justify the use of a large, high-pressure system, making a low-pressure steam engine a more cost-effective and practical solution.
Low-pressure steam engines also tend to have a simpler design compared to their high-pressure counterparts. They are generally less expensive to build, operate, and maintain. This makes them an attractive option for businesses looking for a reliable, low-cost energy solution for less demanding applications. Furthermore, the relatively lower pressure means that low-pressure steam engines operate with less stress on the materials and components, leading to longer lifespans and reduced wear and tear. This also translates into lower maintenance and repair costs over time.
These engines are especially beneficial in industries that rely on steam for heating, drying, or sterilization processes. For example, in the food industry, low-pressure steam is commonly used for pasteurization, drying, and cooking, as steam provides a consistent, gentle heat source that helps preserve the quality of food products. In textile manufacturing, steam is used to set dyes, remove wrinkles, and carry out other finishing processes. Low-pressure steam engines are capable of providing the necessary steam for these operations while keeping energy costs manageable.
In smaller-scale power generation, low-pressure steam engines can be used for cogeneration or combined heat and power (CHP) applications. In these systems, the steam engine produces both electricity and thermal energy, which can be used for heating or industrial processes. The relatively low pressure of the steam allows for efficient generation of both forms of energy in industries where power demand is moderate. For example, low-pressure steam engines can be used in district heating systems, where steam generated from a central plant is distributed to nearby buildings for heating purposes.
Another important aspect of low-pressure steam engines is their adaptability to various types of fuel sources. While many low-pressure systems are fueled by traditional energy sources like natural gas, oil, or coal, there is also growing interest in using renewable fuels such as biomass or waste materials. Biomass, including wood chips, agricultural waste, and even municipal waste, can be used as fuel for low-pressure steam engines, making them an environmentally friendly option for industries looking to reduce their carbon footprint. This fuel flexibility makes low-pressure steam engines an appealing choice in applications where energy costs and sustainability are important considerations.
Low-pressure steam engines are also easier to integrate into existing systems compared to higher-pressure engines. Their lower steam requirements make them ideal for retrofit applications, where businesses can add steam-powered solutions to their existing infrastructure without major overhauls or expensive upgrades. This ease of integration is particularly useful for industries that want to improve energy efficiency or reduce their reliance on external power sources but do not need the full capacity of a high-pressure steam system.
In some cases, low-pressure steam engines are used in backup or emergency power applications. Since they operate at lower pressures, they are less complex and can be brought online quickly in case of a power failure. While they may not provide the same level of power output as high-pressure steam engines, their ability to generate steam and electricity in a relatively short amount of time makes them valuable for maintaining operations in the event of grid disruptions or other emergencies.
The environmental benefits of low-pressure steam engines are also noteworthy. Since they often use renewable fuels and operate more efficiently in smaller applications, they contribute to the reduction of greenhouse gas emissions and overall energy consumption. By replacing or complementing traditional fossil fuel-powered systems, low-pressure steam engines can help industries meet sustainability targets and lower their environmental impact.
In conclusion, low-pressure steam engines are an essential component in industries that require moderate steam or mechanical power. They are characterized by their simplicity, low operational costs, and ability to provide energy for heating, drying, and small-scale power generation. Their use in cogeneration, renewable fuel applications, and energy recovery systems make them an increasingly important option for businesses looking to improve efficiency while keeping energy costs under control. Whether in food processing, textiles, or district heating, low-pressure steam engines remain a reliable, versatile, and environmentally friendly choice for a variety of industrial applications.
Low-pressure steam engines continue to see widespread adoption due to their flexibility and ability to serve a variety of industrial needs. Their simplicity and lower operational pressures make them particularly effective for applications where high power output is not required, yet there is still a need for a steady, reliable energy source.
In addition to traditional industrial applications, low-pressure steam engines are gaining traction in emerging industries, especially in sustainability-focused operations. Many companies are seeking ways to reduce energy consumption and carbon emissions, and low-pressure steam engines can be integrated with waste heat recovery systems to increase overall energy efficiency. By capturing and reusing waste heat from industrial processes, these engines can maximize their utility without the need for additional fuel inputs. This can significantly reduce operating costs and make industrial processes more environmentally friendly.
Moreover, as industries embrace the circular economy, where resources are reused and waste is minimized, low-pressure steam engines can be used in closed-loop systems. For instance, in food or chemical production, steam generated by the engine can be used for various stages of the production process, and any residual heat or steam can be recaptured and recycled back into the system. This reduces the amount of energy that needs to be sourced externally and minimizes waste.
The growth of decentralized energy systems is another area where low-pressure steam engines can make a significant impact. In areas where grid power is unreliable or not available, industries can use low-pressure steam engines as part of off-grid energy solutions. These systems can be powered by locally sourced fuels, such as agricultural residues, waste products, or even biogas, making them a sustainable and cost-effective solution for off-grid communities or isolated industrial plants. For instance, rural processing plants, small-scale manufacturers, and even remote mining operations can benefit from localized steam power, which provides a more self-sufficient energy solution.
One of the key innovations in low-pressure steam engine technology is the development of more efficient heat exchangers and thermal management systems. These advancements enable better steam utilization, ensuring that even at lower pressures, steam engines can operate close to their peak efficiency. Modern materials and coatings also play a role in enhancing the lifespan of steam engines, as they help prevent issues like corrosion and scale buildup, which are more common in low-pressure systems. By improving the materials used in construction and maintenance of the engines, these innovations increase reliability and reduce the frequency of repairs or replacements.
Another area where low-pressure steam engines are gaining popularity is in industrial decarbonization efforts. By replacing or supplementing fossil fuel-based energy systems with low-pressure steam engines powered by biomass, waste-to-energy technologies, or even green hydrogen, industries can reduce their dependence on non-renewable energy sources. These alternative fuels, when paired with steam engines, not only provide an environmentally friendly power source but also help reduce greenhouse gas emissions in line with global climate targets. This is especially important for industries in regions with stringent emissions regulations or those seeking to improve their environmental credentials.
In industries where heat recovery and thermal energy management are critical—such as in pharmaceuticals, textiles, or paper manufacturing—low-pressure steam engines can play a key role in optimizing energy use. These engines can recover heat from cooling systems, air handling units, or waste streams, which can then be utilized in various production processes. The steam generated can be directed to critical processes such as drying, sterilization, or even for power generation, making the entire system more energy-efficient.
The economic advantages of low-pressure steam engines are also significant. Their ability to operate with a variety of fuels and at lower pressures means that initial capital costs are typically lower than those of high-pressure systems, making them an attractive option for small to medium-sized businesses or industries that do not require the massive power output of a high-pressure engine. Additionally, their relatively simple maintenance requirements and durability ensure that ongoing costs are manageable, further enhancing their cost-effectiveness.
Furthermore, as digital technologies such as sensors, IoT, and data analytics continue to transform industries, low-pressure steam engines are benefiting from improved monitoring and control capabilities. Digital systems can provide real-time insights into the performance of the engine, allowing operators to track parameters such as steam pressure, temperature, and energy output. These systems can also predict maintenance needs and optimize energy use, resulting in reduced downtime and improved overall efficiency.
In conclusion, low-pressure steam engines remain a versatile and efficient solution for a wide range of industrial applications. Their affordability, simplicity, and flexibility in fuel use make them a preferred choice for industries seeking reliable energy solutions. Whether used for heating, drying, small-scale power generation, or cogeneration, low-pressure steam engines contribute to energy efficiency, cost savings, and environmental sustainability. As advancements in technology continue to improve their performance, low-pressure steam engines will remain an essential part of the industrial landscape, helping businesses optimize energy use and reduce their environmental impact.
Applications of Steam Engines in Mining
Steam engines have been a cornerstone in the development of the mining industry, and even in the modern era, they continue to play significant roles in various mining operations. Although the use of steam engines has largely been replaced by more modern technologies in many areas, they remain a valuable option in some niche applications, particularly for remote or off-grid mining operations, as well as for their role in powering equipment and processes.
Here are the key applications of steam engines in mining:
1. Powering Mining Machinery
In the early days of the mining industry, steam engines were used to power machinery like pumps, drills, hoists, and conveyors. Even today, some mining operations in remote areas or those with limited access to electricity still rely on steam engines to power their equipment. Steam engines are particularly valuable in locations where electrical grids are unavailable or unreliable, allowing mining companies to run critical operations without being dependent on external power sources.
For example, steam engines can power water pumps to remove excess water from mines, especially in areas where groundwater infiltration is significant. These engines can also be used to operate hoists for lifting heavy loads from deep mines, ensuring that mining operations continue smoothly even in isolated areas.
2. Steam-Powered Pumps for Water Removal
One of the most common applications of steam engines in mining is water pumping. In underground mining operations, managing water levels is crucial to maintaining a safe working environment. Steam engines, specifically steam-driven pumps, were traditionally used to pump out water from deep mine shafts. These pumps were essential for dewatering flooded tunnels, ensuring the miners could continue their work without the risk of drowning or equipment damage due to excessive water accumulation.
Even in modern mining setups, steam-powered pumps can be used in certain situations where electricity or diesel-powered alternatives are not suitable. In these cases, steam engines serve as a backup or supplementary power source.
3. Ventilation Systems
In underground mining, proper ventilation is critical to ensuring air quality and safety. Steam engines can be used to power ventilation fans that circulate fresh air through the tunnels, ensuring that dangerous gases like methane are ventilated out and fresh oxygen is supplied to miners. Although modern electric fans are more common today, steam engines can still be useful in older mines or remote operations where electrical power is not available.
These engines were historically used to drive large ventilation fans that could move significant volumes of air through the mine, helping to keep miners safe from asphyxiation or exposure to harmful fumes.
4. Powering Crushing and Grinding Equipment
In mining, materials such as ore, coal, and minerals often need to be crushed or ground before they can be further processed. Steam engines were once used to power crushers, mills, and grinders. These machines break down raw materials into smaller, more manageable sizes for further refining or extraction.
While electric or diesel-powered machinery has largely taken over this role, steam engines can still serve in niche applications or in legacy systems where newer technology has not been integrated yet. In some remote mining locations, steam engines remain a viable option for driving these essential processes.
5. Cogeneration and Energy Recovery Systems
In some mining operations, especially those with large-scale processing facilities, steam engines are used in cogeneration systems. In these systems, waste heat generated during mining or processing is used to produce steam, which is then channeled into steam engines to generate power. This process can be highly efficient, as it recycles waste heat that would otherwise be lost, reducing the overall energy consumption of the mining operation.
Steam engines used in cogeneration systems can provide electricity for the mining operations themselves or for other industrial processes nearby. This setup is particularly useful for mining operations in regions where electricity is scarce or expensive, making it more economical for mines to generate their own energy.
6. Heating and Drying in Mineral Processing
Some mining operations, especially in the extraction of minerals like gold, copper, or salt, require heating or drying processes to extract valuable elements from ores or concentrate materials. Steam engines are employed in these processes to provide the necessary heat for drying or smelting.
For instance, steam can be used to heat ovens or drying systems in mineral processing plants, facilitating the removal of moisture or volatile elements from ores. In the past, steam engines were used to drive the furnaces in smelting processes, where ores were heated to high temperatures to separate valuable metals from waste materials.
7. Off-Grid Mining Operations
In certain mining operations that are located in remote regions or on isolated islands, access to a stable electrical grid may not be feasible. Steam engines, particularly those powered by locally available fuel sources like coal, biomass, or even waste materials, provide an efficient off-grid power generation solution. This makes steam engines an attractive option for mining companies that need to operate in remote or developing regions.
These steam engines are capable of generating the necessary power to run various equipment, including pumps, ventilation systems, processing machinery, and lighting, without relying on an external power supply.
8. Historical and Heritage Mining
In some heritage and historical mining sites, steam engines are still in operation as part of museum exhibits or for demonstrating historical mining methods. These sites often use original steam engines that were once critical to the mining industry. Visitors can learn how steam-powered engines were used to perform tasks that are now done by more modern means, such as pumping water or hauling ore from deep shafts.
Additionally, steam engines continue to be used in some regions where older mining technologies are still in use for smaller-scale, artisanal mining operations. The simplicity and low cost of steam engines make them an attractive option for small-scale miners who cannot afford the high investment in modern equipment.
9. Historical Power in Mine Hauling Systems
In the past, steam engines were commonly used to power cableways or trams that transported mined ore from the shaft to the surface or to processing facilities. This system, known as a mine hoist, relied on steam engines to pull ore buckets up from deep within the earth. While most modern mines have replaced these with electric or diesel-driven systems, steam engines are still a part of some older mines or museums dedicated to mining heritage.
Conclusion:
While modern mining operations predominantly use electric and diesel-powered machinery, steam engines continue to play an important role in certain niche applications and in legacy mining systems. Their ability to provide reliable power in remote locations, for pumping, ventilation, heating, and even cogeneration, ensures that they remain an integral part of the mining landscape in specific cases. Whether it’s for water removal, ore processing, or providing backup power, steam engines continue to contribute to the mining industry’s energy needs, particularly in off-grid areas or for historical applications.
In addition to the more traditional uses, steam engines have also found a place in specific mining scenarios where their low cost, reliability, and versatility offer unique advantages. For instance, in some mining regions where environmental regulations are strict or where the local infrastructure is underdeveloped, steam engines powered by biomass or other renewable sources are being explored as a cleaner alternative to diesel or coal-powered equipment. This is especially true in remote mining communities that are trying to reduce their carbon footprints while maintaining consistent operational efficiency.
The resurgence of interest in sustainable mining practices has also led to new applications for steam engines in energy recovery systems. As mining operations generate large amounts of heat, steam engines can be used to harness this waste heat for electricity generation. By using waste heat from smelting, crushing, or other high-temperature processes, mining companies can turn what would otherwise be wasted energy into usable power. This not only improves energy efficiency but can also significantly lower operational costs in the long run.
In some cases, steam engines are still used in older or smaller mining operations where the infrastructure is not suited for modern energy systems. For these types of operations, a steam engine offers an affordable and reliable solution that can be maintained with a relatively low level of technical expertise. The availability of steam engines in second-hand markets also makes them a cost-effective option for these smaller-scale operations, allowing mining businesses to keep running even with limited capital investment.
On the larger scale, while steam engines are not typically used for the heavy-duty work of contemporary mining—such as hauling massive amounts of ore or driving high-efficiency generators—smaller steam-powered systems can still have a place in supporting roles. For example, in gold mining, steam engines can be used in cyanide leaching processes, where a steady supply of heat is needed to improve the extraction of gold from ore. The ability to generate steam in a controlled and efficient manner allows for the consistent application of heat during leaching, which is essential for optimizing recovery rates.
Steam engines are also part of the growing trend of preserving historical mining heritage. Many mining museums and heritage sites around the world continue to showcase steam engines as a representation of the technological advancements that helped shape modern mining operations. Visitors can experience firsthand how steam engines were used to power the equipment that allowed miners to reach deeper levels of the earth or to process raw materials with greater efficiency. These sites serve as a reminder of the historical significance of steam technology in the evolution of the mining industry and provide educational value to the public.
As mining companies continue to explore new ways to reduce their environmental impact, the potential for steam engines to play a role in sustainable, off-grid, and small-scale mining operations remains strong. Their efficiency in specific applications like pumping, heating, and cogeneration, combined with the availability of renewable fuels, makes steam engines a viable solution for industries looking to reduce reliance on non-renewable energy sources. Even though modern technologies have mostly supplanted steam engines in large-scale mining operations, their role in specific applications, particularly in remote or heritage sites, ensures that steam technology remains relevant in the mining industry today.
Steam Engine Automation and Control Systems
Steam engine automation and control systems have evolved significantly over the years, making steam engines more efficient, reliable, and easier to operate. While steam engines were once manually operated and required constant oversight, modern automation and control technologies have been integrated to improve their performance, safety, and energy efficiency. These advances help streamline operations, reduce labor costs, and minimize the potential for human error, which is particularly important in industrial settings where steam engines are used for continuous power generation or process control.
One of the key components of steam engine automation is the integration of sensors and controllers that continuously monitor and adjust various parameters such as steam pressure, temperature, and flow rates. These sensors provide real-time data to control systems, which can make adjustments to optimize engine performance. For example, if the steam pressure drops below a set point, the control system can automatically adjust the fuel supply or combustion rate to bring the pressure back to the desired level. This ensures that the steam engine operates at its peak efficiency without manual intervention.
Modern steam engine control systems often employ programmable logic controllers (PLCs), which are digital computers used to automate industrial processes. These PLCs can be programmed to manage multiple variables and respond to changes in real-time, allowing for highly precise control of the steam engine’s operation. They can control the opening and closing of valves, adjust fuel flow rates, manage feedwater systems, and coordinate with other pieces of equipment in a steam generation system, such as boilers, turbines, and heat exchangers.
Another important advancement is the use of advanced control algorithms, such as model predictive control (MPC), which uses mathematical models to predict the behavior of the steam engine and optimize its performance based on future conditions. This allows the system to make proactive adjustments rather than just reactive ones, leading to improved efficiency and reduced wear on components. MPC can be particularly useful in complex systems where the steam engine interacts with other processes, such as in cogeneration plants or in systems where steam is used for both power generation and industrial heating.
In addition to improving operational efficiency, automation in steam engines also enhances safety. Steam systems operate under high pressure and temperature, making them inherently dangerous if not properly controlled. Automation can help prevent dangerous conditions by ensuring that parameters stay within safe ranges. For example, if a pressure relief valve fails, the control system can detect rising pressure and shut down the engine before it becomes a safety hazard. Similarly, automated monitoring systems can detect overheating or other abnormal conditions and trigger alarms or safety shutdowns to prevent damage to equipment and avoid accidents.
The use of sensors and automation also plays a significant role in predictive maintenance. By continuously monitoring the health of steam engine components, automation systems can detect early signs of wear, corrosion, or other issues before they lead to costly breakdowns. For example, vibration sensors can monitor the condition of steam engine bearings, and temperature sensors can detect overheating in critical areas. This data can be analyzed to predict when maintenance is required, reducing downtime and extending the life of the engine.
Another notable development in steam engine automation is the integration with digital control systems that allow for remote monitoring and operation. With the use of internet-connected devices and cloud-based software, operators can monitor the performance of steam engines from virtually anywhere, providing flexibility and improved operational oversight. Remote control capabilities can be particularly valuable in large industrial complexes or in off-grid locations where it may be difficult to have on-site personnel available at all times. Remote systems can send alerts, adjust settings, and even shut down the engine remotely if necessary, ensuring continuous monitoring without the need for constant human presence.
In addition to traditional steam engines, automated control systems are also being integrated into steam turbines and other related equipment. In power plants, for instance, automated systems control not only the steam engine but also the entire steam cycle, including the boiler, turbine, condenser, and cooling systems. This comprehensive control ensures that all parts of the system work in harmony to maximize energy output while minimizing fuel consumption and emissions.
Finally, the role of advanced software in steam engine automation cannot be understated. Through the use of sophisticated software tools, operators can perform simulations, optimize system performance, and create predictive models that improve decision-making. These software systems can also be used for troubleshooting, providing insights into potential problems before they become serious issues, and allowing operators to take corrective action proactively.
Overall, the integration of automation and control systems into steam engines has made these systems more efficient, safe, and cost-effective. With the continued advancements in sensor technology, machine learning, and digital control, steam engines are expected to become even more intelligent and autonomous, further enhancing their application across a wide range of industries.
The ongoing advancements in steam engine automation and control systems have the potential to revolutionize industries that rely on steam power for a variety of applications. As these systems become increasingly sophisticated, they offer new ways to improve operational efficiency, reduce environmental impacts, and optimize resource management in industries such as power generation, manufacturing, and even renewable energy sectors.
One of the most promising trends is the integration of artificial intelligence (AI) and machine learning into steam engine control systems. AI algorithms can analyze vast amounts of data in real time, learning from past performance and adjusting operational parameters to optimize energy usage and reduce fuel consumption. This can lead to significant cost savings for industries that rely on steam for power generation. For example, AI can predict fluctuations in steam demand and adjust the steam engine’s performance accordingly, ensuring that the system operates at optimal efficiency without wasting energy.
Moreover, AI can enhance predictive maintenance efforts by analyzing sensor data over time to detect patterns that may indicate impending failures. For instance, if a particular component is beginning to wear out or exhibit unusual behavior, the system can flag it for early maintenance, preventing unexpected breakdowns and extending the lifespan of the engine. By leveraging AI in this manner, companies can shift from reactive to proactive maintenance strategies, reducing both downtime and repair costs.
In addition to AI, the integration of the Internet of Things (IoT) in steam engine systems has paved the way for even more intelligent operations. With IoT-enabled devices, steam engines can be continuously monitored across a network of interconnected systems, allowing for real-time adjustments based on external factors such as weather conditions, fuel quality, or energy demand. For example, if a steam engine is part of a cogeneration system, IoT sensors could track the temperature of the steam and adjust boiler operations to optimize power generation and heat recovery. These intelligent systems can help minimize waste, enhance productivity, and align steam engine performance with broader business objectives.
Another area where automation is making a significant impact is in the energy efficiency of steam engines. As industries seek to reduce their carbon footprints and meet sustainability goals, the ability to optimize steam production and consumption becomes more critical. By automating fuel management systems, for example, the combustion process can be continuously adjusted to achieve the most efficient fuel-to-steam ratio, minimizing emissions and maximizing energy output. Automated systems can also monitor exhaust gases and make real-time adjustments to reduce harmful emissions, helping industries comply with environmental regulations.
Moreover, steam engine automation systems are increasingly integrated into broader energy management platforms that manage the entire energy lifecycle in industrial settings. These platforms allow for centralized control of energy sources, helping companies better manage their consumption patterns, reduce waste, and make data-driven decisions regarding energy procurement, storage, and usage. In large manufacturing plants or power generation facilities, these systems can provide detailed insights into the performance of each energy-consuming unit, enabling managers to identify inefficiencies and take corrective actions.
As industries shift towards more sustainable practices, the role of steam engines in renewable energy applications has gained attention. Steam engines, particularly in the form of combined heat and power (CHP) systems, are an integral part of cogeneration processes where both electricity and heat are produced from a single fuel source. With the automation of these systems, the efficiency of such cogeneration setups has increased, making them more competitive with traditional, less efficient energy production methods. By optimizing the steam engine’s output and recovery systems through advanced control strategies, these installations can provide substantial savings while contributing to a lower carbon footprint.
In some renewable energy projects, steam engines are integrated into solar thermal power plants, where concentrated solar power (CSP) is used to generate heat, which is then used to produce steam. This steam drives a turbine or engine, generating electricity. Automated steam engine systems are critical for adjusting the amount of heat used to create steam, based on solar conditions and electricity demand. The ability to manage these variables efficiently through automation ensures that solar thermal power plants can operate at peak efficiency, providing a consistent and reliable energy supply.
Furthermore, steam engines are being increasingly utilized in decentralized energy systems, where small-scale, modular steam engines provide on-site power generation. In such systems, automation ensures that steam engines operate autonomously, adjusting to varying loads and conditions without the need for manual intervention. This is particularly valuable in remote areas, off-grid locations, or in industries that require an independent and reliable energy source.
The ongoing push for digitalization in industrial processes is also affecting how steam engines are controlled and operated. With the advent of digital twins—virtual models of physical systems—engineers and operators can simulate and monitor the performance of steam engines in real time. This digital representation provides valuable insights into how the engine behaves under different conditions, and helps to identify potential issues before they manifest in the physical system. The digital twin can be connected to the control system, allowing for dynamic adjustments that optimize performance.
As the demand for more integrated, energy-efficient, and sustainable industrial processes grows, steam engines, when combined with advanced automation and control systems, offer significant potential to meet these challenges. From reducing energy consumption to improving safety and maintenance, the automation of steam engine operations not only enhances the overall performance but also contributes to the broader goals of energy efficiency and sustainability. Moving forward, steam engine technology will continue to evolve, with automation and smart technologies at the forefront of making them even more adaptable and efficient in modern industrial applications.
Steam Engine for Thermal Power Plants
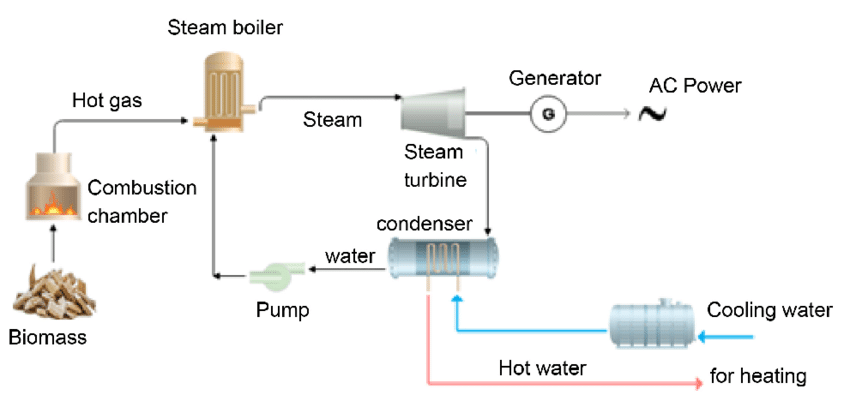
Steam engines have played a pivotal role in the development and operation of thermal power plants, though they have largely been replaced by steam turbines in most large-scale facilities due to their greater efficiency and capacity. However, understanding the role of steam engines in these power plants, especially in historical and smaller-scale applications, can provide insights into the fundamental principles of steam power generation and its evolution over time.
Role of Steam Engines in Thermal Power Plants
In early thermal power plants, steam engines were the primary technology used to convert thermal energy into mechanical energy, which was then used to generate electricity. These engines worked by heating water to create steam, which would then be directed into the steam engine’s cylinders. The steam’s pressure would push a piston, which was connected to a mechanical system to drive a generator and produce electricity. Over time, as the demand for electricity grew, the limitations of steam engines became apparent, particularly in terms of their efficiency and scale.
Transition to Steam Turbines
While steam engines were effective for smaller applications and served as the foundation of early power generation, their relatively low efficiency and difficulty scaling up for larger power plants made them less suitable for meeting the growing energy needs of industrialized societies. Steam turbines, developed in the late 19th century, emerged as a more efficient alternative.
Steam turbines operate on the same basic principle as steam engines (converting steam’s energy into mechanical work), but they use a rotating blade system rather than pistons to capture the steam’s energy. This allows steam turbines to operate at much higher efficiencies and handle larger steam flows, making them ideal for use in modern thermal power plants. Today, virtually all large-scale thermal power plants use steam turbines instead of steam engines to generate electricity.
However, the principles of steam generation and the importance of maintaining high-pressure and high-temperature steam remain central to the operation of both steam engines and steam turbines.
The Steam Cycle in Thermal Power Plants
In thermal power plants, whether using steam engines or steam turbines, the process of generating electricity follows the basic Rankine cycle, which involves the following steps:
- Boiler (Steam Generation): Heat energy is applied to water in a boiler, causing the water to vaporize into steam. This steam is then fed into the engine or turbine at high pressure and temperature.
- Expansion (Mechanical Work): In the case of a steam engine, the high-pressure steam would be directed into cylinders, where it pushes pistons to produce mechanical energy. In a steam turbine, the steam passes through rotating blades that convert the steam’s energy into mechanical energy, turning a generator.
- Condensation: After expanding through the engine or turbine, the steam is cooled and condensed back into water in a condenser. The water is then pumped back into the boiler to begin the cycle again.
- Energy Conversion: The mechanical energy generated by the steam engine or turbine is then converted into electrical energy by a generator.
Advantages of Steam Engines in Small-Scale or Historic Applications
While steam turbines are more efficient for large-scale power generation, steam engines can still be useful in certain smaller or specialized applications within thermal power plants. For example:
- Off-Grid or Remote Power Plants: In areas where electricity access is limited or where large-scale infrastructure is not feasible, small steam engines may still be used for power generation in thermal plants. These engines are typically fueled by local resources, such as biomass, coal, or waste heat, providing a cost-effective solution for remote communities or industrial sites.
- Heritage and Historical Power Plants: Some older thermal power plants, particularly those in heritage sites or museums, may still have functioning steam engines as part of their educational or historical exhibits. These engines demonstrate the evolution of steam technology and provide a practical understanding of how thermal power generation operated in the past.
- Hybrid Power Systems: Steam engines can be part of hybrid systems in smaller-scale, specialized applications, such as combined heat and power (CHP) systems. These systems use the waste heat from steam generation to provide heating or other forms of energy, improving overall efficiency.
Modern-Day Use of Steam in Thermal Power Plants
Although steam engines are largely obsolete in modern thermal power plants, the principle of generating steam to drive turbines remains central to thermal power generation. In contemporary power plants, the steam is generated by burning fossil fuels, nuclear reactions, or through concentrated solar power (CSP), which heat water to generate steam. The steam then drives a steam turbine that turns a generator to produce electricity.
In recent years, there has been an increasing focus on making thermal power plants more efficient and environmentally friendly. Innovations in boiler design, turbine technology, and steam cycle optimization (such as supercritical and ultra-supercritical steam cycles) have led to significant improvements in the efficiency of modern plants. Supercritical and ultra-supercritical cycles operate at higher pressures and temperatures, increasing the efficiency of steam turbines by allowing them to extract more energy from the same amount of steam.
Challenges in Steam Engine Use in Power Plants
Despite their early importance, steam engines face several challenges when considered for use in modern thermal power plants:
- Low Efficiency: Steam engines are less efficient than modern steam turbines, especially at the scale needed for large power plants. Steam turbines can convert more thermal energy into mechanical energy, resulting in higher electrical output and lower fuel consumption.
- Scaling Issues: Steam engines are typically less capable of scaling up to handle the large amounts of steam required in modern power plants. They are best suited for smaller-scale applications, which limits their use in the expansive power generation plants that meet the needs of modern cities and industries.
- Maintenance Complexity: Steam engines require more frequent maintenance and have higher operational costs compared to steam turbines. They involve more mechanical components, which are subject to wear and tear, and their operation requires more manual intervention.
Conclusion
While steam engines have been mostly replaced by more efficient steam turbines in large-scale thermal power plants, their historical role in the development of power generation cannot be understated. Steam engines were foundational in early thermal power plants, and their principles still inform the design of modern steam cycles. Today, steam engines continue to find niche applications in small-scale, off-grid, or heritage contexts, but for most industrial and power generation needs, steam turbines have become the dominant technology.
The continuing focus on optimizing steam cycles, improving energy efficiency, and exploring sustainable energy sources will ensure that steam-based power generation remains a vital part of the global energy mix. Despite the shift from engines to turbines, steam technology, in all its forms, continues to evolve and contribute to the world’s energy landscape.
Future Trends in Steam Engine Use for Thermal Power Plants
Although steam engines have been largely phased out of large-scale applications, there are still several emerging trends that may influence the role of steam engines or steam-based technology in the future of thermal power plants. These trends are centered around increased efficiency, sustainability, and the integration of advanced technologies to meet the evolving energy demands of the world.
1. Sustainable and Green Steam Technology
The global push for sustainability and the reduction of carbon emissions has led to significant research in cleaner and more efficient thermal power generation technologies. Steam engines, when integrated into systems like biomass or waste-to-energy plants, could offer environmentally friendly alternatives by utilizing renewable fuels and waste heat. While steam turbines dominate large-scale applications, steam engines in smaller setups might contribute to these green energy goals, particularly in decentralized energy systems.
Biomass Power Generation: Biomass is a renewable resource that can be used to generate steam for power generation. Steam engines could be used in smaller biomass power plants or combined heat and power (CHP) systems, providing energy for local communities while reducing dependence on fossil fuels. This aligns with sustainability goals and helps reduce emissions associated with traditional energy production.
Waste Heat Recovery: Industrial processes and manufacturing plants often generate significant amounts of waste heat, which can be harnessed using steam engines. By recovering and reusing this waste heat, steam engines can convert otherwise lost thermal energy into usable power, contributing to overall energy efficiency and reducing the need for additional fuel inputs.
2. Combined Heat and Power (CHP) Systems
Combined Heat and Power systems use a single energy source to generate both electricity and useful heat, making them highly efficient. Steam engines, due to their simplicity and smaller size, could be used in CHP setups for industrial facilities or even residential areas, particularly in places where space and efficiency are key considerations.
In smaller industrial settings or areas that require both heating and electricity, steam engines could operate in CHP units to take advantage of waste heat and provide both power and thermal energy simultaneously. This reduces overall energy consumption and maximizes the output from available fuel, making steam engines valuable in niche applications.
3. Hybrid Systems and Microgrids
The development of microgrids—small-scale, localized energy networks that can operate independently or in conjunction with the main power grid—has created new opportunities for steam engine use in power generation. In off-grid or remote areas, steam engines powered by biomass, coal, or waste could provide a reliable and efficient energy source. They could be part of hybrid systems that combine various renewable and traditional energy sources, like solar, wind, and steam-powered engines, to ensure energy reliability.
Automation and advanced control systems would make it easier to integrate steam engines into such hybrid systems, where they could be automatically adjusted based on energy demand, fuel availability, and environmental conditions. These systems could offer a sustainable, reliable solution for off-grid power generation in communities that are not connected to the main electricity grid.
4. Decentralized Energy Systems
The shift toward decentralized energy systems, where power generation is distributed across smaller, localized plants rather than relying on centralized power stations, could lead to a resurgence in the use of steam engines in specific applications. Smaller thermal plants powered by steam engines could be built closer to industrial users, reducing transmission losses and providing on-demand power for manufacturing and other industrial activities.
Steam engines, particularly in modular setups, could offer a reliable energy solution for industries where large-scale power plants are not feasible. Modular steam systems could be installed in a variety of settings, including manufacturing plants, chemical processing facilities, and even large residential developments. By providing localized energy, these systems reduce transmission infrastructure costs while ensuring that industries have access to reliable power sources.
5. Advanced Materials and Technology for Steam Engines
Advancements in materials science could enhance the performance and efficiency of steam engines in thermal power generation. Innovations in heat-resistant materials, such as high-strength alloys and ceramics, could allow steam engines to operate at higher temperatures and pressures, similar to modern steam turbines. This would increase the efficiency of the steam engines, making them more competitive in both large and small-scale applications.
Additionally, additive manufacturing (3D printing) technology could make it easier to produce complex steam engine components more efficiently, reducing production costs and enhancing customizability. This could lead to more versatile steam engines that are better suited to a variety of industrial applications, particularly in settings where space or specialized conditions limit the use of larger steam turbines.
6. Digitalization and Smart Control Systems
The integration of digital technologies into thermal power plants, including steam engines, is transforming the way these systems are monitored and operated. Advanced sensors, real-time monitoring, and artificial intelligence (AI) can improve the operational efficiency and predictive maintenance of steam engines. These technologies allow operators to continuously monitor the condition of the engine, ensuring it operates at peak performance while minimizing fuel use and maintenance costs.
Digital Twins: A digital twin is a virtual replica of a physical system, like a steam engine, which can be used for real-time monitoring, simulation, and predictive analysis. Operators can use digital twins to simulate various operating conditions and assess how different parameters (e.g., temperature, pressure, or steam flow) affect engine performance. This allows for better optimization of the steam engine’s operation and a reduction in unplanned downtime.
AI and Machine Learning: AI can be used to optimize the steam engine’s operation by learning from historical data and adjusting operating parameters for maximum efficiency. Machine learning models can predict when maintenance is required, minimizing downtime and extending the engine’s lifespan.
Conclusion
While steam engines may no longer dominate large-scale power generation, they continue to play a significant role in specific thermal power applications, particularly in small-scale, off-grid, and hybrid energy systems. Their ability to operate on a variety of fuels, including biomass, waste heat, and even coal, makes them versatile and adaptable to modern energy needs. With ongoing advancements in materials science, automation, and control systems, steam engines may find a renewed place in the energy landscape, especially in decentralized energy systems and applications requiring combined heat and power.
As industries and communities increasingly prioritize energy efficiency and sustainability, the future of steam engines will depend on their ability to integrate with emerging technologies like AI, renewable energy sources, and smart control systems. Whether they are used in historical power plants, small-scale CHP systems, or hybrid renewable energy setups, steam engines will continue to be a part of the evolving energy mix in the future.
Advanced Materials in Steam Engine Manufacturing
The performance, efficiency, and longevity of steam engines, especially in modern and industrial applications, heavily depend on the materials used in their construction. Advanced materials have made significant contributions to improving steam engine technology, allowing for more efficient operation, higher thermal and mechanical stress resistance, and longer service life. These materials are crucial in both steam engine components like boilers, cylinders, and pistons, and in the supporting infrastructure of modern thermal power plants.
The following are some of the key advanced materials used in steam engine manufacturing and their roles in improving engine performance:
1. High-Temperature Alloys
Steam engines, particularly those in thermal power plants, are subjected to extremely high temperatures and pressures. High-temperature alloys are designed to withstand these harsh conditions without degrading. These alloys are used in critical components such as steam boilers, turbine blades, and other engine parts that come into direct contact with hot steam.
- Nickel Alloys: Nickel-based alloys, such as Inconel, are used in steam engines due to their high resistance to oxidation and corrosion at elevated temperatures. These alloys maintain their strength and integrity in high-pressure steam environments, making them ideal for use in steam turbines and high-temperature engine components.
- Chromium Alloys: Chromium alloys, often used in conjunction with nickel, are highly resistant to wear, corrosion, and oxidation. These properties are vital in preventing the erosion of components that are exposed to steam, making them suitable for parts such as steam pipes, valves, and boiler tubes.
- Titanium Alloys: While not as commonly used in traditional steam engines, titanium alloys are gaining interest for specific applications where weight, strength, and resistance to corrosion are critical. Titanium can also withstand extreme temperatures and provide high tensile strength, making it suitable for high-performance steam engines and modern power generation systems.
2. Superalloys
Superalloys are a group of high-performance materials that can operate under extreme environmental conditions, such as high temperatures and pressure, without losing their mechanical properties. Superalloys are particularly useful for critical components like turbine blades and heat exchangers, where prolonged exposure to heat and stress occurs.
- Cobalt-based Superalloys: These alloys are known for their excellent resistance to thermal fatigue, oxidation, and creep (deformation under stress). They are often used in the manufacturing of components that need to withstand extreme heat and pressure, making them suitable for steam engine turbines and other high-stress parts.
- Iron-based Superalloys: These are used in steam engines that require exceptional strength and fatigue resistance at high temperatures. Iron-based superalloys are commonly found in heavy-duty industrial applications, providing superior strength for engine cylinders and piston components.
3. Ceramics and Ceramic Composites
Ceramics are non-metallic materials known for their high thermal resistance, hardness, and wear resistance. Their use in steam engines is becoming more prominent due to their ability to withstand high temperatures without deteriorating. Ceramic composites combine the advantages of ceramics and metals, providing additional mechanical strength while maintaining high resistance to thermal expansion.
- Ceramic Coatings: Ceramic coatings are often applied to components like turbine blades, pistons, and boiler tubes to protect them from heat-induced damage. These coatings enhance the thermal efficiency of the engine by providing a barrier that reduces heat loss and minimizes the wear and tear on the underlying metal components.
- Silicon Carbide (SiC): This ceramic material is used for high-performance steam engine components due to its excellent wear resistance and ability to handle high temperatures. Silicon carbide is often employed in valve seats, pumps, and seals, where it can endure high friction and temperature variations without degradation.
- Alumina Ceramics: Alumina is another type of ceramic that offers good wear resistance and can be used for components exposed to both high heat and mechanical stress, such as in engine valves and combustion chambers.
4. Composite Materials
Composite materials, which combine two or more distinct materials to achieve desirable properties, are increasingly used in steam engine manufacturing to reduce weight, improve efficiency, and enhance component durability.
- Carbon Fiber Composites: Carbon fiber composites are lightweight yet incredibly strong and durable. These materials are used in specific engine components like fan blades, exhaust systems, and other areas where reducing weight without sacrificing strength is essential. Carbon fiber can also improve the efficiency of steam engines by lowering the inertia of rotating components.
- Fiberglass Reinforced Plastics (FRP): These materials are used for non-structural components in steam engine systems, such as insulation covers, piping, and protective coatings. FRP is resistant to corrosion and provides excellent thermal insulation, which helps to retain heat and improve the engine’s overall efficiency.
- Metal Matrix Composites (MMCs): These composites are composed of a metal matrix (such as aluminum or steel) combined with reinforcing materials like ceramics. MMCs are used in steam engine parts that require enhanced wear resistance, improved thermal conductivity, and high strength. They are particularly useful in components like pistons and cylinder heads.
5. Graphene and Advanced Carbon Materials
Graphene, a one-atom-thick layer of carbon, has become a material of significant interest due to its extraordinary mechanical, electrical, and thermal properties. In steam engine technology, graphene and advanced carbon materials are being explored for their potential to improve heat transfer, reduce friction, and enhance overall engine efficiency.
- Graphene-based Lubricants: Graphene can be used in lubricants to reduce friction in steam engine components. The addition of graphene improves the efficiency of moving parts, such as pistons and turbines, by providing smoother operation and reducing wear.
- Carbon Nanotubes (CNTs): CNTs are highly conductive and possess remarkable strength and thermal stability. Their use in engine components could lead to lighter, stronger parts that can better withstand the thermal and mechanical stresses typical in steam engine systems.
6. Stainless Steel and High-Strength Steels
Stainless steel and high-strength steels remain essential materials in the construction of steam engines, particularly in components exposed to high temperatures and pressure. These steels offer a good balance of strength, durability, and resistance to corrosion and are used in parts such as:
- Boiler Tubes: Stainless steel is commonly used in boiler tubes and pressure vessels due to its ability to withstand corrosion, thermal fatigue, and high temperatures, all of which are prevalent in steam generation processes.
- Cylinders and Pistons: High-strength steel alloys are used in steam engine cylinders and pistons to provide the necessary durability and strength for handling the mechanical forces generated during operation.
- Steam Valves and Fittings: The excellent corrosion resistance of stainless steel makes it ideal for valves, fittings, and other parts that come into direct contact with steam and hot water.
7. Advanced Insulation Materials
In steam engine systems, minimizing heat loss is essential for maximizing energy efficiency. Advanced insulation materials help retain heat within the system, improving overall thermal efficiency and reducing fuel consumption. Modern insulation materials used in steam engine systems include:
- Aerogels: Aerogels are lightweight materials with very low thermal conductivity, making them ideal for insulating high-temperature components. They are used to insulate steam pipes, boilers, and other engine components where heat retention is crucial for efficiency.
- Mineral Wool and Ceramic Fiber: These materials are used for insulating steam engine components and preventing heat loss. They are durable and can withstand extreme temperatures, making them suitable for high-heat environments like steam boilers.
Conclusion
The continuous advancement of materials science has had a profound impact on steam engine technology, allowing for greater efficiency, longer operational lifetimes, and improved performance. The use of high-temperature alloys, ceramics, composites, and other advanced materials has allowed modern steam engines and their components to operate under increasingly demanding conditions. As the need for energy efficiency and sustainability grows, further developments in materials will continue to push the boundaries of steam engine design, ensuring that they remain a viable part of both modern power generation and specialized industrial applications.
The evolution of steam engine technology is closely tied to advancements in materials science. As the demands for higher efficiency, longer operational life, and greater sustainability grow, the role of advanced materials becomes even more crucial. Materials such as high-temperature alloys, ceramics, composites, and other advanced substances allow steam engines to perform under the harshest conditions, withstanding the extreme temperatures, pressures, and mechanical stresses common in modern applications. This progress ensures that steam engines continue to be relevant in both large-scale and niche power generation setups, as well as industrial systems that require robust, reliable machinery.
Nickel-based and chromium alloys, for instance, offer significant improvements in corrosion and oxidation resistance, essential for the longevity and effectiveness of steam engines. These alloys are critical in components such as turbines, boilers, and high-pressure pipes, where they help maintain structural integrity in environments with constant high temperatures. Similarly, cobalt-based superalloys and iron-based variants offer enhanced resistance to fatigue and thermal degradation, making them ideal for critical parts that experience constant stress, such as engine pistons and blades.
Meanwhile, ceramics and ceramic composites provide an important solution for high-performance steam engine components. Their inherent properties, such as resistance to high temperatures and wear, make them suitable for parts like turbine blades and combustion chambers. The use of ceramic coatings also helps protect metal parts from excessive heat, improving the overall efficiency of the engine by reducing heat loss.
The introduction of composite materials, such as carbon fiber reinforced plastics and metal matrix composites, has led to significant reductions in the weight of steam engine components, enhancing efficiency without compromising strength. These materials are especially valuable in reducing inertia in moving parts, which results in smoother operation and less energy required to keep components moving.
The ongoing research into graphene and other advanced carbon materials opens up exciting new possibilities for steam engine technology. Graphene’s extraordinary properties, such as its high strength and thermal conductivity, are being explored for use in engine lubricants, where they can significantly reduce friction and wear. This could lead to improvements in the performance of steam engines, especially in terms of energy conservation and mechanical longevity.
In the context of insulation, advanced materials such as aerogels and ceramic fibers have drastically improved heat retention in steam systems. These materials ensure that heat loss is minimized, improving the overall thermal efficiency of steam engines. Such developments contribute to better fuel economy and reduced environmental impact, key considerations as industries shift toward greener and more sustainable energy solutions.
As the technology continues to evolve, the integration of these advanced materials into steam engine design and manufacturing promises to enhance both the performance and sustainability of steam-powered systems. With an increasing focus on reducing environmental footprints, the ongoing development of materials that enhance efficiency, reduce waste, and extend the life of components will play a significant role in shaping the future of steam engines, particularly in power generation and industrial applications.
Moreover, the combination of materials advancements with automation, digital monitoring, and AI-driven optimization will help ensure that steam engines remain competitive in an energy landscape that is increasingly driven by the demand for efficiency and environmental consciousness. The future of steam engines lies in their ability to adapt to new materials and technologies, making them more versatile and suitable for a wide array of modern applications while maintaining their historical significance in energy production.
Steam Engine for Home Use: Efficient Energy Solutions
Steam engines, once predominantly used in large-scale industrial applications, are being re-evaluated for home use due to their potential to provide efficient, sustainable, and reliable energy solutions. As concerns about energy costs, sustainability, and self-sufficiency grow, steam engines could offer an alternative to conventional home heating, power generation, and other energy needs. By utilizing a small-scale, home-based steam engine, homeowners could harness energy from renewable resources or waste heat, reducing reliance on grid electricity while promoting greener, more efficient energy use.
One of the primary advantages of using steam engines for home applications is the ability to generate both electricity and heat through combined heat and power (CHP) systems. A small steam engine could be integrated into a home’s energy infrastructure to produce electricity, and the waste heat generated from the steam engine could be used for space heating, water heating, or even cooking, depending on the system’s design.
A major challenge for implementing steam engines in residential settings is the size, complexity, and cost of traditional steam engines. However, advancements in materials, design, and miniaturization have paved the way for the development of smaller, more efficient steam engines that are more suited for home use. These smaller engines could run on a variety of fuels, such as wood, biomass, natural gas, or even solar energy in combination with steam storage systems, allowing homeowners to choose a fuel source that aligns with their sustainability goals and local resources.
Biomass, in particular, holds great promise for small-scale steam engines due to its renewable nature and widespread availability. Wood pellets, agricultural residues, or even household waste can be used to generate steam, which drives a steam engine to generate power. The biomass could be burned in a small boiler, with the steam produced driving the engine’s piston or turbine, which in turn generates electricity. This setup could be an attractive solution for rural homes or areas where access to the power grid is limited or unreliable.
Another growing trend in steam engine technology for home use is the integration of solar thermal systems with steam engines. Solar collectors can heat water or other fluids to generate steam, which is then used to power a steam engine. This hybrid system takes advantage of the sun’s energy, reducing dependence on traditional fossil fuels. The benefit of such a system is that homeowners could produce energy during sunny days and store excess steam or energy in a thermal battery for later use. This approach promotes energy independence while significantly lowering energy bills.
For homeowners with a focus on sustainability, steam engines powered by waste heat recovery systems could also be a viable solution. Many homes, especially those that rely on industrial or manufacturing processes for heating, produce waste heat that can be harnessed to generate steam. This heat could be used to power a steam engine that produces both electricity and useful heat, further enhancing energy efficiency.
A key benefit of using a steam engine for home energy generation is the potential for energy independence. A well-designed home steam engine system could reduce reliance on external energy sources, whether it be electricity from the grid or heating fuel. Over time, such a system could lead to significant savings, especially as energy prices continue to rise. Furthermore, steam engines are durable, reliable, and well-suited for off-grid applications, making them an ideal choice for homeowners looking to adopt a more self-sufficient energy model.
Modern steam engines designed for home use are also more automated than their historical counterparts. With the integration of advanced control systems, digital sensors, and automation technology, steam engines can operate more efficiently and require less manual intervention. These systems can monitor fuel levels, steam pressure, and temperature to ensure the engine operates optimally. Homeowners can even monitor and control their steam-powered systems remotely via mobile apps or other smart home technologies, making the system easier to manage and maintain.
Maintenance of home steam engines is typically straightforward, though regular servicing is necessary to keep the engine in top condition. Modern materials and technologies ensure that steam engines are more resistant to corrosion and wear than older models. Ensuring that the boiler, piston components, and other parts remain in good working order will maximize the engine’s lifespan and performance.
Overall, the use of steam engines in home energy systems holds the promise of more sustainable, efficient, and reliable energy production. By providing both heat and power, steam engines can address two critical home energy needs at once. Whether fueled by renewable biomass, solar thermal systems, or waste heat recovery, steam engines can contribute to reducing a home’s carbon footprint, decreasing reliance on external energy sources, and increasing energy independence. As technology continues to advance, it is likely that we will see even more compact, cost-effective, and efficient steam engines that make sense for residential energy solutions.
As the demand for energy-efficient, sustainable solutions continues to rise, the adoption of steam engines for home use offers a promising path toward energy independence and reduced environmental impact. While traditional steam engines may seem outdated, modern advancements have made it possible to create compact, efficient, and relatively affordable systems suitable for residential applications.
One of the most significant challenges in integrating steam engines into home energy systems is ensuring their adaptability to a wide range of residential needs. Fortunately, miniaturization and advancements in engine design have enabled the development of smaller engines capable of generating sufficient power for everyday household needs. These small-scale steam engines can be used to power homes, provide heat for water or space heating, and even support appliances in off-grid or remote locations where access to the electricity grid is limited.
The ability to use multiple renewable fuel sources is another compelling feature of steam engines for home use. While traditional fossil fuels such as coal or oil have historically been used to generate steam, modern systems allow for the use of cleaner, renewable fuels such as biomass, wood pellets, and agricultural waste. These fuels can be sourced locally, reducing transportation costs and further contributing to energy security. Furthermore, these alternative fuel options can help reduce carbon emissions, making the home energy system more environmentally friendly.
For homeowners who prioritize sustainability, integrating a steam engine system with existing renewable energy technologies such as solar or wind power can create a more robust and self-sufficient energy solution. Solar thermal energy systems, for instance, can be paired with steam engines to harness the sun’s heat, generating steam to power the engine. This system could reduce the need for external energy sources, especially in regions with ample sunlight. Wind and solar energy can be used to charge batteries that then power an electric boiler or pump to create steam, which could then drive the steam engine.
Waste heat recovery systems also provide another route for utilizing steam engines in home applications. Many industrial, manufacturing, or even household systems produce excess heat that goes unused. Capturing this waste heat and converting it into steam allows homeowners to recover energy that would otherwise be lost, improving overall system efficiency. For instance, a steam engine could be paired with a home’s furnace or even an industrial HVAC system to convert heat into usable power.
Furthermore, the flexibility of steam engines makes them highly suitable for hybrid applications. For example, a home could use a small steam engine powered by solar thermal systems during the day, with the ability to switch to biomass or another fuel source at night. This kind of energy management system ensures that energy production continues seamlessly and without interruptions, providing a reliable source of power even when solar resources are unavailable.
One important consideration for homeowners interested in steam engine systems is the initial investment cost. While modern steam engines for home use are more affordable than their industrial counterparts, they still represent a significant upfront investment. However, these systems offer long-term savings through reduced energy bills and the potential to earn credits or incentives from renewable energy programs. Over time, the savings generated by reduced reliance on grid electricity, heating, and fuel costs can more than offset the initial cost of installation.
Additionally, the maintenance requirements for home steam engine systems are relatively low. Steam engines are known for their reliability and durability, particularly when built with modern materials that resist corrosion and wear. Routine maintenance, such as monitoring fuel levels, checking steam pressure, and ensuring the boiler and pistons remain clean and operational, will ensure optimal performance and longevity of the system.
Finally, as with any emerging technology, advancements in automation and smart home integration are making steam engines easier to operate and manage. Remote monitoring, control systems, and AI-driven optimizations can ensure that the engine operates at peak efficiency. Homeowners can easily monitor steam production, fuel consumption, and energy output through smartphone apps or home automation platforms, providing convenience and control over their energy use.
In conclusion, steam engines for home use represent a promising solution for homeowners looking to adopt more efficient, self-sufficient, and environmentally-friendly energy systems. With their ability to provide both electricity and heat, as well as their adaptability to various renewable energy sources, these systems offer a unique opportunity to reduce energy costs and decrease reliance on fossil fuels. Whether integrated with solar, biomass, or waste heat recovery systems, modern steam engines are well-suited for a variety of home applications, making them an attractive option for the future of residential energy. As technology continues to advance and the demand for sustainable energy solutions grows, steam engines could play a pivotal role in the homes of tomorrow.
Understanding the Mechanics of Steam Engine Design
Understanding the mechanics of steam engine design involves exploring several key principles that govern how steam engines work and the factors that contribute to their efficiency, reliability, and performance. At its core, a steam engine operates by converting heat energy from steam into mechanical energy, which can then be used to perform useful work, such as driving machinery, generating electricity, or powering vehicles. The fundamental components of a steam engine are designed to work together to maximize this conversion process, each serving a specific purpose in the overall operation.
1. Boiler System The boiler is the heart of a steam engine. Its primary function is to heat water to produce steam. The boiler works by burning a fuel (such as coal, wood, or gas) to generate heat, which is transferred to the water inside the boiler. As the water heats up, it turns into steam, which is stored under high pressure. The pressure of the steam is crucial because it directly influences the efficiency of the engine. Higher pressure steam typically results in more power output, as the steam’s force is used more effectively to move pistons or turbines.
2. Steam Generation and Pressure When water turns into steam, it expands rapidly, creating a high-pressure system within the boiler. The pressure is essential because it determines how much force the steam can exert on the engine components, such as pistons or turbines. The high-pressure steam is directed into the engine’s working parts, where its energy is harnessed.
The pressure is often regulated by a valve system to prevent over-pressurization, which could damage the engine or boiler. In modern steam engines, this is typically done through safety valves that release excess pressure to ensure safe operation.
3. Steam Engine Cylinder and Piston The cylinder and piston are the primary components that convert the steam’s energy into mechanical motion. In a traditional steam engine, the high-pressure steam enters a cylinder, where it pushes against a piston. The piston is connected to a mechanical linkage, such as a crankshaft, which transforms the back-and-forth motion of the piston into rotational motion.
The cylinder design is critical because it must accommodate the steam’s pressure while maintaining efficiency. Materials used for the cylinder must be able to withstand the high pressures and temperatures generated during steam expansion. Modern steam engines use specialized alloys and coatings to ensure durability and efficiency.
The piston within the cylinder moves back and forth, driven by the pressure of the steam. This reciprocating motion is what drives the mechanical work of the engine. Depending on the engine’s design, the piston may move in a single-cylinder engine or may be part of a more complex multi-cylinder engine that increases overall power output.
4. Steam Valves and Timing Mechanisms Valves are crucial in directing the steam into and out of the cylinder. The timing of when steam enters and exits the cylinder affects the efficiency and power of the engine. The steam valve controls the flow of steam into the cylinder, while exhaust valves allow the spent steam to exit the system after it has performed work.
Valve timing is a delicate balancing act. If steam is allowed to enter too early or too late, it can cause inefficiencies, loss of power, and even damage to the engine components. Advanced steam engines use valve mechanisms, such as a “slider valve” or “pop-off valve,” which help regulate the intake and exhaust of steam based on the position of the piston.
5. Connecting Rods and Crankshafts The piston’s reciprocating motion must be converted into rotary motion to power machinery or generate electricity. This is accomplished through a system of connecting rods and crankshafts. The connecting rod links the piston to the crankshaft, and as the piston moves, the connecting rod transmits the motion to rotate the crankshaft.
The crankshaft then transmits this rotational force to other mechanical parts, such as gears, wheels, or generators, depending on the intended application of the engine. This system enables the steam engine to power a wide range of devices, from early locomotives and ships to modern power generation turbines.
6. Condenser and Heat Rejection After the steam has performed its work in the engine, it must be cooled and condensed back into water so it can be reused in the boiler. This is typically done in a condenser, which is an essential component of a closed-loop system. The condenser cools the steam, causing it to condense back into water, which can then be pumped back into the boiler for reheating.
Condensers can be either air-cooled or water-cooled, depending on the engine’s design. In large-scale power plants, water-cooled condensers are common, using natural bodies of water or cooling towers. In smaller or mobile steam engines, air-cooled systems may be more practical, relying on fans or other methods to dissipate heat.
7. Efficiency and Heat Loss Efficiency is a critical factor in steam engine design. The more efficiently the engine converts the heat energy from the steam into useful mechanical energy, the more cost-effective and environmentally friendly it becomes. One of the primary areas for improving efficiency is reducing heat loss, which occurs at every stage of the steam cycle—particularly during steam generation, steam expansion, and heat rejection in the condenser.
Designers focus on insulating boilers and piping to minimize heat loss, using advanced materials that retain heat longer and more effectively. Additionally, modern steam engines often incorporate regenerative systems that recover heat from exhaust gases or waste steam, which can be reused to preheat water entering the boiler, further increasing overall efficiency.
8. Engine Layout and Integration The physical layout of a steam engine is an important aspect of its design. Steam engines must be built with considerations for balance, weight distribution, and ease of maintenance. In stationary applications like power plants, space is often less of a concern, so larger engines with extensive heat recovery systems are used. In contrast, mobile steam engines, such as those used in locomotives or ships, require careful consideration of weight, size, and space constraints.
Steam engines also integrate with other mechanical systems, such as pumps, compressors, and turbines. The overall design must account for how these components interact to ensure smooth and reliable operation. In modern steam power generation systems, this often includes digital control systems and automation to manage the flow of steam and monitor key performance metrics.
9. Modern Innovations Modern steam engine design continues to evolve with advancements in materials science, thermodynamics, and automation. For example, new high-temperature alloys, ceramics, and composites allow steam engines to operate at higher pressures and temperatures, which can lead to more efficient power generation. Additionally, computerized control systems have made it possible to optimize steam engine performance and improve maintenance schedules.
In addition to enhancing the performance of steam engines in traditional applications, these innovations are also enabling new applications, such as combining steam engines with renewable energy sources (e.g., solar-thermal or biomass) to create hybrid systems that offer both sustainability and energy efficiency.
By understanding the mechanics behind steam engine design, engineers can continue to refine and innovate in this area, ensuring that steam engines remain a viable energy solution for both modern industry and specialized applications, such as home energy systems, renewable energy generation, and industrial cogeneration.
The design and functionality of a steam engine are rooted in a combination of thermodynamic principles, mechanical components, and careful attention to detail in order to optimize efficiency. As engineers continue to innovate in steam engine technology, they focus on enhancing the overall system’s performance, reducing fuel consumption, and increasing the engine’s lifespan.
One significant challenge in steam engine design is the conversion of heat into mechanical energy. In a perfect system, all of the heat generated by the combustion process would be converted into work. However, in reality, some energy is always lost due to inefficiencies, friction, and heat dissipation. Engineers strive to reduce these losses through improvements in insulation, sealing mechanisms, and component design. For example, using more heat-resistant materials in high-stress areas such as the cylinder and piston can minimize heat loss and improve thermal efficiency. Similarly, improved lubrication systems help reduce friction between moving parts, ensuring smoother operation and less energy wasted.
The pressure and temperature at which steam is produced also directly influence the performance of a steam engine. High-pressure steam allows for more powerful engine performance, but it also requires careful management. If steam pressure is not properly controlled, it can lead to catastrophic failure. Therefore, modern steam engines incorporate advanced pressure regulators and safety valves that allow for fine control over the steam’s pressure, ensuring that the engine operates within safe limits while still delivering optimal performance.
In addition to steam generation, condensation is another important aspect of steam engine design. Once the steam has passed through the engine and performed its work, it needs to be cooled and turned back into water. A properly designed condenser system is essential in this process. Condensers work by reducing the temperature of the exhaust steam, converting it back into liquid water that can then be reused in the boiler. The efficiency of this process impacts the overall performance of the engine, as the faster the steam can be condensed and reused, the more energy-efficient the engine becomes. Modern steam engines often incorporate heat exchangers or air-cooled condensers to speed up this process and recover energy from exhaust gases.
As steam engines are often used in large-scale applications such as power plants, ships, and factories, their design must also account for the systems that support their operation, including boilers, fuel storage, and maintenance systems. These components must be robust and capable of handling the stresses and strains placed on them during continuous operation. Materials used in these systems must withstand extreme temperatures and pressures, and they must be regularly maintained to ensure that wear and tear do not compromise the engine’s performance or safety.
With the rise of environmental consciousness, steam engines are also being adapted to work with renewable energy sources. For instance, solar-thermal steam engines use solar collectors to heat a fluid, which then generates steam. This technology can be used to drive a steam engine in off-grid or remote areas, providing electricity and heat without relying on fossil fuels. Similarly, waste heat recovery systems are being designed to capture and convert heat produced by industrial processes into usable steam, offering a sustainable way to improve energy efficiency in factories and plants.
Another exciting area of development is the integration of automation and digital controls into steam engines. Modern steam engines are increasingly being equipped with sensors, monitoring devices, and automated control systems that allow for more precise management of the engine’s performance. These systems can track variables such as steam pressure, temperature, fuel consumption, and engine speed, and they adjust the operation of the engine accordingly. Not only does this improve efficiency, but it also reduces the risk of human error and helps with predictive maintenance, identifying potential problems before they lead to failures.
The increasing interest in sustainable energy solutions also brings about opportunities for combining steam engine technology with other green energy technologies, such as combined heat and power (CHP) systems, which allow steam engines to produce both electricity and useful heat. This hybrid approach is more efficient than traditional systems because it uses the waste heat from the steam engine to heat water or provide space heating, reducing energy waste.
The steam engine’s ability to function using a variety of fuels—such as biomass, wood, coal, or even agricultural waste—also makes it a versatile energy solution. While fossil fuels are still commonly used in large-scale steam engines, biomass and other renewable fuels can reduce the carbon footprint of steam-powered systems. As fuel technology continues to improve, and as the world moves toward more sustainable energy sources, steam engines that run on biomass or waste-derived fuels may become an increasingly attractive option for both industrial and residential energy solutions.
In the future, steam engine technology is likely to continue evolving, incorporating more sophisticated materials, advanced thermodynamic cycles, and smarter control systems to enhance performance. With the increasing focus on clean energy, steam engines have the potential to play a significant role in various sectors, including power generation, transportation, and industrial processes, offering a reliable and environmentally friendly alternative to traditional energy systems. Whether through improvements in fuel efficiency, integration with renewable energy sources, or advancements in automation, steam engine technology is poised to remain a key player in the global energy landscape for many years to come.
Steam Engine Design for Power and Efficiency
Designing a steam engine for both power and efficiency requires a careful balance between multiple factors: the engine’s ability to generate sufficient mechanical work while minimizing energy losses and maximizing thermal efficiency. Achieving this balance involves optimizing the key components and systems of the steam engine to ensure smooth operation, durability, and effective energy conversion.
At the heart of steam engine design is the boiler system, which generates the steam necessary to drive the engine’s mechanical components. For power and efficiency, the boiler must be designed to maximize heat transfer while minimizing heat loss. This means using advanced materials that have high thermal conductivity and durability under the extreme temperatures and pressures within the system. Insulation plays a critical role in reducing heat loss to the environment, which increases the overall energy efficiency of the system. Boilers designed for efficiency often incorporate multi-pass systems, where the combustion gases pass through multiple sections of heat exchangers, transferring as much heat as possible before exiting the system.
Pressure and temperature control are vital in steam engine design. High-pressure steam tends to produce more power, but it also requires more robust materials to withstand the increased stress. Modern steam engines often use superheaters to further increase steam temperature, which enhances the steam’s energy content. Superheated steam can drive turbines or pistons with greater force, improving the engine’s power output. However, maintaining steam pressure within safe limits is essential, and sophisticated pressure regulation systems, such as automatic control valves and safety valves, are critical to avoid dangerous over-pressurization.
Once the steam is produced, it is directed into the cylinder where it exerts force on the piston. The movement of the piston is typically converted into rotary motion via a crankshaft, which can drive machinery or generate electricity. For power and efficiency, the cylinder and piston must be designed to handle the high-pressure steam while maintaining low friction. The use of advanced materials, such as heat-resistant alloys or ceramics, ensures that these components remain durable even under the stress of continuous operation.
Steam valve systems are equally important in controlling the flow of steam into and out of the cylinder. Precision in the timing of steam admission and exhaust is essential to optimize engine performance. Too early or too late an admission of steam can result in wasted energy, while inefficient exhaust can reduce the engine’s overall output. Advanced valve timing mechanisms, such as the Watt governor or automatic valve timing systems, help to optimize the intake and exhaust of steam throughout the engine cycle, ensuring efficient operation. These systems regulate the timing of steam release based on the position of the piston, further enhancing efficiency.
Another factor in achieving efficiency is the condensation and heat recovery process. After the steam has done its work, it must be cooled and converted back into water so that it can be reused in the boiler. Condensers play a vital role in this phase. The design of the condenser should ensure that it efficiently cools the steam using a heat exchanger system, either air-cooled or water-cooled, depending on the application. Efficient condensation systems prevent heat loss and allow the engine to recover and reuse heat, significantly boosting overall system efficiency.
To further optimize efficiency, steam engines in modern applications often integrate regenerative systems. These systems recover waste heat from exhaust steam or flue gases and use it to preheat the incoming water entering the boiler. This helps reduce the amount of fuel needed to reach the desired temperature for steam generation, thereby improving fuel economy and overall thermal efficiency.
Automation and digital control systems are increasingly being incorporated into steam engine designs to optimize performance. These systems allow for real-time monitoring of steam pressure, temperature, fuel consumption, and engine speed, adjusting the engine’s operation to ensure maximum efficiency at all times. For example, if the engine detects a dip in steam pressure, it can automatically adjust fuel input or steam valve settings to maintain optimal performance. These smart systems also contribute to predictive maintenance by identifying wear and tear on critical components before they fail, reducing downtime and maintenance costs.
Reducing friction is another crucial aspect of steam engine design. Frictional losses in the engine’s moving parts, such as pistons, cylinders, and crankshafts, can lead to significant reductions in power output and efficiency. Engineers use high-quality lubricants, precision machining, and low-friction materials to minimize these losses, ensuring that the engine operates smoothly and efficiently.
Finally, for power generation applications, steam engines must be designed to integrate seamlessly with the systems they support, such as electrical generators or mechanical systems. The steam engine’s output must be matched to the energy demand of the generator or machinery it is driving. This requires careful attention to the engine’s operating speed, torque, and load capacity to ensure that the engine delivers consistent and reliable power.
In conclusion, optimizing steam engine design for both power and efficiency involves a multi-faceted approach that combines careful selection of materials, advanced thermodynamics, precise component design, and the integration of automation and control systems. By maximizing heat transfer in the boiler, managing steam pressure and temperature, and ensuring that every phase of the engine’s cycle is fine-tuned for efficiency, engineers can design steam engines that deliver reliable power while minimizing fuel consumption and environmental impact. With modern advances in materials science, automation, and energy recovery systems, steam engines continue to evolve, offering both power and efficiency for a wide range of applications, from industrial power generation to small-scale, renewable energy systems.
Optimizing steam engine design for both power and efficiency also involves considering the overall system integration. This means not just the engine itself, but also how it interfaces with external systems like boilers, turbines, and heat recovery systems. The performance of these systems is interdependent, so attention to every detail is essential to achieving an optimal balance of power output and fuel efficiency.
One of the key aspects is the relationship between the steam engine’s thermal efficiency and the fuel it uses. The type of fuel can impact both the steam generation process and the efficiency of the entire system. While traditional coal or gas may still be common in larger installations, the rising demand for more sustainable energy sources has led to the development of biomass-fired steam engines and waste-to-energy systems. These alternative fuels may require different boiler designs or modifications to ensure that the engine can run efficiently while minimizing the environmental impact.
The fuel-to-steam conversion process is another area where efficiency gains can be made. Engineers are always looking for ways to maximize the efficiency of this process, ensuring that as much energy as possible is extracted from the fuel while minimizing waste products such as ash, smoke, or greenhouse gases. Techniques like fluidized bed combustion or circulating fluidized bed systems are examples of advanced technologies that enhance combustion efficiency and allow steam engines to run cleaner.
In addition, advancements in steam engine component designs continue to play a critical role in improving overall engine efficiency. For example, modern turbines in power plants have become more efficient due to improvements in their design and material science. Super-alloys capable of withstanding high temperatures and pressures ensure that turbines can extract the maximum possible energy from high-temperature steam. These turbines, in combination with optimized heat exchangers, allow for more efficient use of steam, making the engine more reliable while increasing its overall performance.
To further enhance steam engine efficiency, advanced monitoring and diagnostic systems are being employed to provide real-time data on the performance of the system. With the advent of Internet of Things (IoT) technologies and smart sensors, steam engines can now be equipped with monitoring tools that track every critical parameter, from steam pressure and temperature to wear and tear on individual components. This data is used to adjust engine performance in real-time, improving operational efficiency, reducing energy consumption, and identifying maintenance issues before they lead to failure.
In modern applications, steam engines are often part of cogeneration systems, where they are paired with other energy-producing devices, such as gas turbines or solar collectors, to improve overall system efficiency. In these systems, the steam engine produces not only mechanical work or electricity but also useful heat, which can be used for industrial processes, space heating, or hot water production. By making use of what would otherwise be waste heat, cogeneration systems reduce fuel consumption and improve overall efficiency.
Furthermore, the size and scale of steam engines are crucial considerations in design. While small steam engines are typically used in applications like home heating or backup power systems, large-scale steam engines are employed in industrial settings, such as power plants and factories. These engines need to be carefully designed to match the scale of the application, ensuring that they can handle high loads while maintaining efficiency. Modern designs often include features such as multi-stage turbines or combined cycle systems to maximize power output while minimizing fuel use.
Another important aspect of modern steam engine design is environmental sustainability. With increasing awareness of climate change and environmental issues, reducing the carbon footprint of steam engines has become a significant design goal. Modern steam engines are now often designed to incorporate clean-burning technologies, advanced filtration systems to reduce particulate emissions, and efficient waste heat recovery systems to lower overall emissions. Additionally, the development of carbon capture technologies is being explored for use in steam engine applications, especially in industrial settings, to mitigate the release of CO2 and other greenhouse gases into the atmosphere.
The maintenance and reliability of steam engines are also crucial factors in ensuring they remain efficient over their operational lifespan. Regular maintenance is necessary to ensure that components such as pistons, valves, and cylinders remain in optimal condition, and that wear and tear don’t lead to inefficiencies or failures. Modern steam engine designs incorporate components that are easier to inspect, maintain, and replace, reducing the downtime and maintenance costs associated with these systems. Additionally, many steam engines now feature self-monitoring systems that alert operators when specific components are starting to fail or when operational parameters fall outside the expected range, allowing for quicker corrective action.
Finally, the future of steam engine technology is likely to see continued innovation as engineers work to improve fuel efficiency, reduce emissions, and develop hybrid systems that combine steam with other renewable technologies. We are already seeing examples of steam engines that integrate with solar-thermal systems or geothermal energy sources, which could further reduce dependence on fossil fuels and lead to more sustainable energy production. Steam engines may also play an increasingly important role in decentralized power generation, where they provide reliable energy to remote areas or off-grid locations.
In conclusion, designing a steam engine for power and efficiency requires a comprehensive approach that balances several factors: heat generation, steam management, material selection, system integration, and environmental impact. With ongoing advances in technology, such as high-performance materials, automation, and clean energy solutions, steam engines continue to evolve to meet the growing demand for efficient and sustainable energy systems. The future of steam engine design holds promise for even more efficient, reliable, and eco-friendly power generation, making them a critical component of the global energy landscape for years to come.
How Steam Engines Work: A Detailed Breakdown
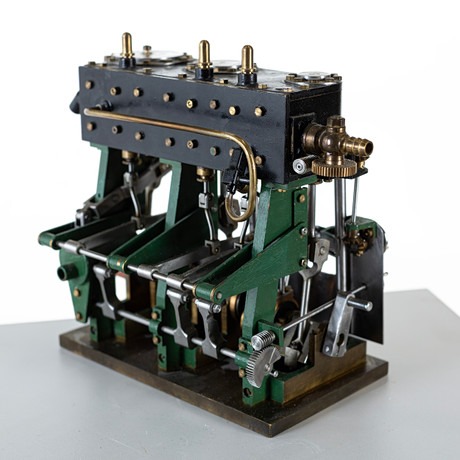
Steam engines have been a fundamental part of industrial development, harnessing the power of steam to perform mechanical work. To understand how they work, it’s important to break down their core components and processes, from steam generation to energy conversion and waste heat recovery.
1. Steam Generation (Boiler)
The heart of any steam engine is the boiler, where water is heated to create steam. The process starts with burning a fuel (commonly coal, oil, or gas) in a furnace, which heats water contained in a closed system of pipes or a large pressure vessel. The fuel’s heat causes the water to turn into steam. The temperature and pressure of the steam depend on the fuel source, the boiler design, and how much heat is being applied. In some advanced designs, such as superheaters, steam temperature is increased even further to maximize the energy it can deliver.
The boiler is carefully designed to handle the pressures created by the steam. The system must ensure that it can safely operate at high pressure to produce powerful steam, but it also requires built-in safety valves to release steam if the pressure becomes too high to avoid catastrophic failure.
2. Steam Expansion (Cylinder and Piston)
Once the steam is generated, it is directed into the cylinder, where it is used to move the piston. The cylinder is a chamber that is usually fitted with a tightly sealed piston that moves back and forth inside the cylinder. When steam enters the cylinder, it pushes the piston. This force is created by the pressure difference between the steam and the atmosphere. As the steam expands, it drives the piston, which is connected to a crankshaft that turns the linear motion of the piston into rotary motion. This rotary motion can then be used to drive mechanical systems, such as wheels, generators, or other machinery.
3. Exhaust and Condensation
After the steam has expanded and performed its work by pushing the piston, it is exhausted from the cylinder and must be cooled and condensed back into water. This process occurs in the condenser. In older steam engines, this was simply achieved by allowing the steam to cool in the open air, but modern steam engines typically use water-cooled or air-cooled condensers. These condensers help reduce the temperature of the exhaust steam, turning it back into liquid water. The liquid water is then returned to the boiler for reuse in a closed-loop system.
This process of cooling and condensing steam is essential for efficiency. If the steam is not adequately condensed, the system will experience energy loss, and the engine’s overall performance will suffer.
4. The Role of Valves
Steam engines are equipped with valves to control the flow of steam into and out of the cylinder. The precise timing of steam admission and exhaust is crucial for maximizing the engine’s efficiency and performance. As the piston moves, valves open and close to allow steam in and exhaust steam out. In many traditional engines, slide valves were used, but in modern steam engines, rotary valves and automated control systems are used to ensure more precise control over steam flow.
The valve timing is coordinated with the motion of the piston to ensure that steam is introduced at the right moment during the piston’s cycle, and the exhaust occurs at the appropriate point in the stroke. Proper valve timing is vital for maintaining engine efficiency and preventing steam wastage.
5. Heat Recovery and Efficiency
One of the challenges with traditional steam engines is the significant energy lost through exhaust steam and hot gases. To enhance efficiency, heat recovery systems are often employed. These systems recover waste heat from exhaust gases or steam and reuse it to preheat the water entering the boiler or to heat other parts of the system. By capturing waste heat, these systems reduce fuel consumption and improve the overall thermal efficiency of the engine.
Regenerative heating systems work by using a heat exchanger to transfer heat from exhaust gases to the incoming water supply. By doing so, the temperature of the water entering the boiler is raised, which reduces the amount of fuel needed to generate the necessary steam.
6. Power Transmission
As the piston moves, it is connected to a crankshaft, which converts the linear motion of the piston into rotational motion. The crankshaft’s rotation is the key to converting the mechanical energy from the steam into usable work. This rotary motion can then be transmitted to various mechanical systems, such as gears, flywheels, or generators.
In large power plants, steam engines are often coupled with electric generators. The steam engine drives the generator’s turbine or alternator, which converts mechanical energy into electrical energy. This electricity can then be supplied to the grid or used locally to power various devices and machinery.
7. Control and Automation
Modern steam engines incorporate control systems that monitor various operational parameters, such as steam pressure, temperature, and fuel consumption. These systems can adjust the steam flow, fuel input, and other critical variables to optimize performance. Automation is increasingly integrated into steam engines to provide real-time performance feedback and make adjustments based on external conditions.
For example, if the steam pressure drops below a certain threshold, an automated control system may adjust the fuel input or open additional valves to restore performance. This ensures that the engine operates efficiently without overburdening components, reducing wear and increasing the overall lifespan of the engine.
8. Safety Systems
Because steam engines operate at high temperatures and pressures, they are equipped with several safety systems to prevent dangerous failures. Safety valves are one of the most important components, as they allow steam to escape if pressure levels exceed safe limits. In addition, modern steam engines may incorporate sensors and safety cutoffs that monitor the engine’s performance, alerting operators to potential issues before they cause harm.
9. Types of Steam Engines
There are different types of steam engines, including reciprocating steam engines, turbine engines, and locomotive engines. Each of these types varies in terms of how the steam is harnessed and converted into mechanical energy. Reciprocating engines, for example, rely on pistons to produce work, while turbine engines use steam to rotate a turbine. The choice of engine depends on the application, with some designs being more suitable for stationary power generation and others for transportation.
10. Modern Adaptations and Sustainability
Today’s steam engines are adapted for a variety of green energy applications. For example, solar-thermal steam engines use solar energy to heat water, creating steam without the need for conventional fossil fuels. Similarly, biomass-fired steam engines use organic materials such as wood chips or agricultural waste to produce steam, offering a more sustainable alternative to traditional coal-fired systems.
These modern adaptations allow steam engines to contribute to the growing demand for renewable energy while maintaining their long-established efficiency and versatility.
In conclusion, steam engines work by utilizing the thermal energy from steam to perform mechanical work. The process involves steam generation, expansion in the cylinder, exhaust and condensation, and the conversion of mechanical energy via pistons and crankshafts. With ongoing innovations in automation, heat recovery, and clean energy solutions, steam engines continue to evolve, maintaining their relevance as a reliable and efficient source of power for a wide range of industries.
Steam engines operate on the principle of converting thermal energy into mechanical energy through the use of steam. The heart of the engine is the boiler, where fuel is burned to generate heat, which then turns water into steam. This high-pressure steam is directed into a cylinder, where it expands and pushes a piston, creating motion. This motion is then converted into rotary power through a crankshaft, allowing the engine to perform useful work. After the steam has done its work, it is exhausted from the cylinder, typically entering a condenser where it is cooled and returned to its liquid state, ready to be reheated in the boiler.
Over the years, engineers have worked to improve steam engine efficiency, introducing features like superheating the steam, recovering waste heat, and optimizing the timing of the valve systems that control the flow of steam into and out of the cylinder. Modern steam engines can be equipped with sensors and control systems that monitor steam pressure, temperature, and fuel consumption, allowing for real-time adjustments to maximize efficiency.
One of the most important developments in steam engine technology has been the ability to use various types of fuel, from coal and oil to biomass and even solar energy. This flexibility allows steam engines to be applied in a wide range of industries, from power generation and manufacturing to transportation and marine applications. The ability to operate on renewable energy sources, such as biomass or solar thermal energy, has made steam engines a viable option for reducing dependence on fossil fuels.
The basic operating cycle of a steam engine involves several key processes: first, the boiler heats water to generate steam, which is then sent to the engine’s cylinder. In the cylinder, the steam expands and pushes the piston, which is connected to a crankshaft that transforms the piston’s linear motion into rotational motion. After the steam has done its work, it is exhausted from the cylinder, and the cycle repeats.
However, the efficiency of the engine depends not only on the design of the boiler and cylinder but also on the effectiveness of the valve system, the quality of the materials used, and the ability to capture and recycle waste heat. Advances in materials science have led to the development of components that can withstand higher temperatures and pressures, improving the overall efficiency and power output of the engine.
In recent years, steam engines have become an integral part of cogeneration systems, where they work alongside other energy technologies to provide both electricity and useful heat. This synergy maximizes the energy output of the system while minimizing fuel consumption and emissions, making steam engines a key component in sustainable energy systems.
Despite their historical importance, steam engines continue to evolve. Today, they are often found in modern applications such as combined cycle power plants, solar-thermal energy systems, and waste-to-energy technologies. As technology advances, steam engines may play an increasingly significant role in achieving global sustainability goals, particularly in industries where high heat and power are necessary.
In essence, steam engines remain a vital part of the global energy landscape, offering a flexible, efficient, and increasingly eco-friendly solution for a wide variety of energy needs. Whether in large-scale power generation, industrial processes, or even home use, the core principles of the steam engine continue to provide a reliable and sustainable means of producing mechanical power from heat.
Steam Engines for Industrial Applications
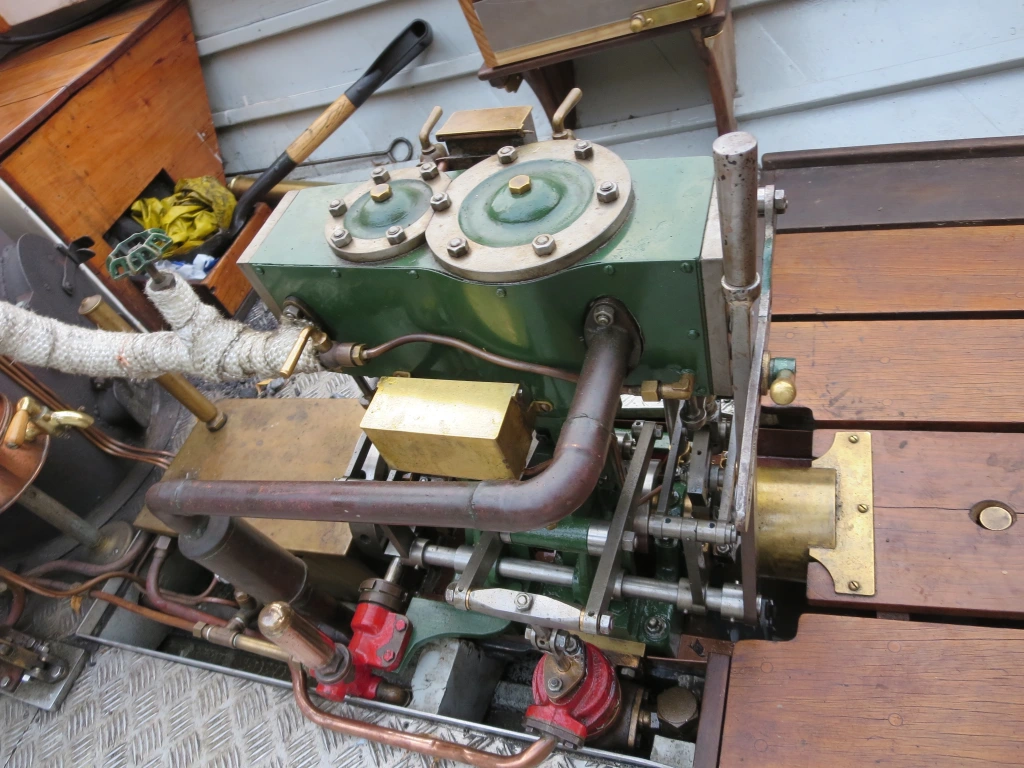
Steam engines have been a crucial component in industrial applications for centuries, powering everything from factories to locomotives and ships. Despite the development of newer technologies like electric motors and gas turbines, steam engines still play an important role in many industrial sectors due to their reliability, versatility, and ability to use a variety of fuel sources. Here’s how steam engines continue to be used in various industries today.
In power generation, steam engines are often used in large-scale thermal power plants, where they generate electricity. These power plants use boilers to produce steam by heating water using various fuel sources, such as coal, oil, natural gas, or even renewable sources like biomass or waste materials. The steam is directed into a steam turbine, which converts the thermal energy into mechanical energy to generate electricity. This process is a crucial part of many industrial and residential energy grids, providing reliable power for cities and industries.
Manufacturing industries also rely on steam engines for a range of applications. Steam engines can drive large machinery, such as pumps, compressors, and conveyor belts, by converting the energy from steam into mechanical motion. For instance, steam-driven pumps are often used in industries like chemical manufacturing, where the transfer of fluids at high pressures is required. Additionally, steam engines are commonly found in textile mills and sawmills, where they provide the necessary mechanical power for spinning, weaving, or cutting materials.
The food processing industry benefits from steam engines as well. In processes like cooking, sterilizing, and drying, steam is used for its high heat content. Steam engines are often incorporated into systems that use steam boilers to provide a controlled source of heat for processes such as pasteurization, canning, or drying products. The ability to maintain consistent temperatures with steam makes it ideal for ensuring the quality and safety of food products.
In the chemical industry, steam engines are used to power various reaction vessels, distillation columns, and mixing equipment. Heat is often required to facilitate chemical reactions, and steam provides an effective way to achieve the necessary temperature control. Additionally, steam can be used in processes that require the transfer of large amounts of heat, such as in the production of chemicals like fertilizers, pharmaceuticals, and plastics.
Steam engines are also valuable in mining applications, especially in operations that require pumping, crushing, and grinding. The steam engines drive equipment that extracts minerals or transports material from one location to another. In some mining operations, steam engines are still employed to power equipment such as draglines, shovels, and hoists that are used in the extraction and handling of materials.
Another industrial application is in shipbuilding and maritime transport. Steam engines, particularly steam turbines, were once the dominant form of propulsion for ocean-going ships, including cargo vessels and passenger liners. While marine diesel engines have largely replaced steam in modern vessels, steam engines are still used in certain applications, particularly in older ships, research vessels, or specialized craft. Steam turbines are also used in nuclear-powered vessels, where steam is generated by the heat from nuclear reactions.
In pulp and paper production, steam engines are used to power paper mills and pulping machinery. Steam is essential for the high temperatures needed in the pulping process, which breaks down wood fibers into usable pulp. The energy produced by steam engines is also used in drying and pressing paper, ensuring that the paper produced is consistent and of high quality.
In the oil and gas industry, steam engines are used in oil refineries and petrochemical plants. Steam is often required for heating crude oil to facilitate its separation into various components. Steam engines can also drive machinery used in the distillation and cracking processes, converting oil into useful products like gasoline, diesel, and other petrochemicals.
Furthermore, steam engines are a crucial part of district heating systems, where they are used to generate heat for urban areas or industrial complexes. In these systems, steam is produced in a central plant and then distributed through a network of pipes to provide heat for buildings, factories, and other facilities. These systems are energy-efficient, especially when coupled with renewable heat sources like geothermal or solar thermal energy.
In research and development, steam engines continue to be used in experimental setups, particularly for testing the efficiency of steam turbines, boilers, and other related systems. They are often part of academic research projects aimed at improving steam engine technology, energy efficiency, and the environmental impact of steam-powered systems.
Despite the development of alternative power generation technologies, steam engines remain a vital part of industrial infrastructure. Their adaptability, efficiency, and ability to use a variety of fuel sources make them an enduring solution for many industries. Advances in steam engine technology, including innovations in turbine design, materials science, and automation, continue to improve their performance and efficiency, ensuring that they will remain a critical energy source in industrial applications for years to come.
Steam engines have proven to be a highly adaptable technology that continues to support a wide range of industries by providing reliable power for various applications. The versatility of steam engines allows them to operate in diverse environments, and their ability to utilize different fuel sources, such as coal, natural gas, oil, and renewable biomass, enhances their appeal in industries that require reliable and consistent energy output.
In addition to traditional power generation and industrial applications, modern steam engines are also increasingly being integrated into combined heat and power (CHP) systems, also known as cogeneration systems. These systems combine electricity generation with the simultaneous production of useful heat. The waste heat from the steam engine, which would typically be lost in a conventional system, is instead captured and used for industrial processes, district heating, or hot water generation. This significantly improves the overall efficiency of the system, making steam engines a more sustainable option in industries focused on reducing energy consumption and emissions.
The maintenance and upkeep of steam engines are also critical in industrial settings. Regular maintenance is essential for ensuring safe operation and maximizing the lifespan of the engine. This includes checking for leakages, ensuring valve systems operate correctly, monitoring boiler pressures, and cleaning heat exchangers to ensure they are functioning optimally. By implementing rigorous maintenance schedules, industries can reduce the risk of breakdowns and enhance the overall performance of their steam-powered systems.
Automation and digital control systems have transformed the way modern steam engines are operated in industrial settings. These systems allow operators to remotely monitor and control steam pressure, flow rates, and temperature levels, optimizing the engine’s performance while reducing the risk of human error. Automation also enables real-time diagnostic tools to alert operators to potential issues before they become critical, helping to prevent unplanned downtime and costly repairs.
The role of sustainability in industrial applications is becoming increasingly important, and steam engines are being adapted to meet the growing demand for environmentally friendly technologies. For example, the integration of carbon capture technologies with steam-powered systems can significantly reduce the carbon footprint of industries that rely on steam for their operations. These technologies capture carbon dioxide emissions before they are released into the atmosphere, helping industries comply with stringent environmental regulations while continuing to use steam as a viable energy source.
Steam engines also continue to play an important role in remote or off-grid applications, where access to electricity from conventional power grids may be limited or non-existent. In such cases, steam engines powered by biomass or waste materials can provide a reliable and cost-effective source of energy for isolated communities, mining operations, or agricultural processes. By using locally sourced materials, these steam engines can provide a sustainable and autonomous energy solution that is crucial for maintaining industrial activities in areas with limited access to external power sources.
While the global trend is shifting towards renewable energy sources such as solar, wind, and hydroelectric power, steam engines still offer a robust alternative in areas where these technologies are not viable due to location or energy demands. Waste-to-energy technologies, which rely on steam engines to convert municipal waste or biomass into power, are gaining traction as an eco-friendly solution to waste disposal while simultaneously generating power. This form of energy generation can be particularly useful in urban areas where landfills are reaching capacity, and waste management becomes an increasing challenge.
As technology continues to evolve, there are also innovations in materials and engineering that are enhancing the efficiency and performance of steam engines. The use of high-performance alloys and ceramics in steam engine components such as boilers, turbines, and valves helps withstand higher pressures and temperatures, which in turn improves the overall energy conversion efficiency. These advancements in materials also contribute to extended equipment lifespans, reduced maintenance costs, and enhanced safety.
In heavy industries, such as steel manufacturing, cement production, and chemical processing, steam engines continue to be an indispensable source of power for operating furnaces, kilns, and large industrial reactors. The high temperatures required for many of these processes are ideally suited to steam engines, which provide a consistent and controllable heat source.
Finally, as the industrial landscape continues to prioritize sustainability, the integration of steam engines with emerging technologies such as solar thermal energy or geothermal energy is gaining interest. These hybrid systems combine traditional steam engine designs with renewable energy sources, creating efficient, low-emission solutions for industries aiming to reduce their carbon footprint while maintaining high energy output.
In conclusion, steam engines are far from obsolete in the modern industrial world. Their enduring relevance is due to their versatility, efficiency, and the ongoing innovations that continue to improve their performance and sustainability. Whether in power generation, manufacturing, or renewable energy applications, steam engines remain a key player in the global effort to meet energy needs while advancing industrial capabilities. Their capacity to use diverse fuel sources and their integration with newer technologies ensures they will continue to be an essential part of many industries well into the future.
Affordable Steam Engine Solutions for Small Businesses
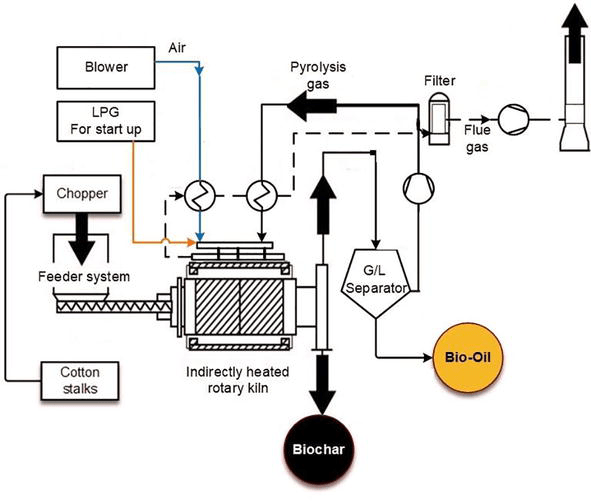
Affordable steam engine solutions for small businesses are increasingly becoming a viable option for those looking to reduce energy costs, improve operational efficiency, and even move toward more sustainable energy sources. While larger industrial operations have traditionally relied on steam engines, there is growing interest from small businesses in adopting steam-powered systems to drive machinery, generate heat, and even produce electricity. Here are some ways small businesses can take advantage of affordable steam engine solutions:
- Energy Efficiency for Small-Scale Operations: For small businesses, energy costs can be one of the largest expenses, and steam engines can help reduce this burden. By installing small, efficient steam engines or boilers, businesses can generate heat and power directly from local, renewable sources such as biomass, wood chips, or agricultural waste. These locally sourced materials can help reduce dependence on expensive fossil fuels and ensure a more reliable and affordable energy supply. Additionally, steam engines can power smaller machines, such as pumps, fans, or conveyor systems, which are commonly used in manufacturing and agricultural operations.
- Combined Heat and Power (CHP) Systems: Many small businesses, particularly those in food processing, textiles, or even small-scale manufacturing, can benefit from combined heat and power (CHP) systems. These systems use steam engines to generate both electricity and useful heat simultaneously. For example, a small brewery could use a steam engine to power the brewing equipment while using the waste heat for brewing and cleaning processes. Similarly, cogeneration systems can provide a cost-effective solution for small businesses in areas where the local power grid is unreliable or where there are opportunities to reduce heating costs.
- Custom Steam Engine Designs for Small Business Needs: Custom-designed steam engine solutions can be tailored to meet the specific needs of small businesses. Whether it’s a small-scale steam turbine for power generation or a compact steam boiler for heating purposes, these systems can be designed to match the capacity and energy requirements of the business. By choosing the right size and configuration, businesses can optimize energy efficiency while keeping installation and operational costs low.
- Renewable Energy Integration: Small businesses can benefit from integrating renewable energy sources such as solar thermal or biomass with steam engine systems. Solar thermal systems can generate heat for steam production, which can then be used for heating, power generation, or industrial processes. Biomass steam engines, for example, can run on agricultural residues, wood chips, or even organic waste, making them an environmentally friendly option for businesses in agricultural, forestry, or waste management sectors.
- Financing and Incentives for Small Businesses: Many governments and organizations offer financing programs, grants, or incentives for businesses that invest in energy-efficient systems, including steam engines. These programs can significantly reduce the upfront cost of installation, making steam-powered systems more affordable for small businesses. In addition, by switching to steam engines that use renewable energy or waste heat recovery, businesses can often access tax credits or rebates that further lower the initial investment.
- Low-Cost and Low-Maintenance Options: For small businesses that may not have extensive budgets for energy systems, there are steam engine solutions that require minimal investment while still offering long-term benefits. Smaller, modular steam boilers or simple steam-powered systems can provide a cost-effective starting point for businesses without requiring significant capital investment. These systems often come with lower maintenance costs due to simpler designs and fewer moving parts compared to larger, more complex steam engines.
- Small-Scale Power Generation: Small businesses that operate in off-grid locations or remote areas can benefit from affordable steam engines for off-grid power generation. For example, businesses in rural areas, such as those in agriculture, mining, or even remote hospitality, may not have reliable access to the electrical grid. In such cases, small steam engines powered by biomass or even waste materials can provide a sustainable and independent power solution.
- Low Emission Steam Engines: In light of increasing environmental concerns, businesses are also focusing on sustainability. Low-emission steam engines designed to minimize the environmental impact are available and can be particularly appealing for small businesses seeking to improve their carbon footprint. Modern steam engines can be designed with more advanced combustion technology that reduces particulate matter and carbon dioxide emissions, making them an environmentally responsible choice for businesses aiming to meet sustainability goals.
- Training and Support for Small Business Owners: Many suppliers of steam engine systems for small businesses offer training and technical support to ensure smooth installation and operation. This can help small business owners who may not have extensive experience with steam engine technology to easily understand the system’s operation and perform basic maintenance tasks. Having access to support can minimize downtime and prevent costly repairs.
- Scalable and Flexible Solutions: As a small business grows, its energy needs may change. The advantage of choosing steam engines is their scalability. A small business can start with a relatively simple steam-powered system and later upgrade to a larger, more efficient system as energy demands increase. This scalable flexibility allows businesses to expand without the need for completely overhauling their energy infrastructure.
In conclusion, affordable steam engine solutions for small businesses are not only about reducing energy costs but also about providing an eco-friendly, reliable, and versatile energy source. By taking advantage of smaller, customized steam engine systems and integrating them with renewable energy solutions, small businesses can benefit from increased efficiency and energy independence. Moreover, with incentives and financing options available, these systems are becoming more accessible, making them a viable and cost-effective choice for many small business owners looking to improve their energy efficiency and sustainability.
Steam engines for small businesses can also contribute significantly to reducing operating costs over the long term. By switching to steam-powered systems, businesses can lower their dependence on costly electricity, especially in areas where energy prices are high or where grid reliability is poor. Steam engines can be used to provide power for a variety of small-scale applications such as heating, powering machinery, or generating electricity, which helps businesses become more self-sufficient.
Another benefit is the potential for steam engines to make use of locally available and often low-cost fuels. For example, biomass, agricultural waste, or even organic waste materials can be used to fuel steam engines, reducing reliance on fossil fuels and helping small businesses reduce their environmental impact. By choosing renewable fuels, businesses can also improve their sustainability profile, which is increasingly important for attracting environmentally conscious customers and meeting regulatory requirements.
In addition to fuel flexibility, steam engines can also help small businesses by increasing overall process efficiency. For instance, in industries such as food processing, paper production, or small-scale manufacturing, steam engines can provide a reliable and cost-effective source of heat for cooking, drying, or curing processes. By integrating steam engines into their operations, businesses can improve productivity while reducing energy consumption, ultimately lowering operating costs.
Small businesses can also benefit from the modularity and customizability of steam engine systems. Modular steam engine designs allow businesses to start with smaller systems that meet their immediate needs, with the option to expand as their operations grow. This flexibility makes it possible for businesses to invest in a system that fits their current requirements while keeping future scalability in mind.
While steam engine technology is often seen as antiquated, modern advances in materials science and engineering have made steam engines more efficient, compact, and affordable. For small businesses, this means that they can now access high-quality, durable steam engine solutions without having to invest in outdated or inefficient equipment. Enhanced boiler designs, improved turbine efficiency, and more advanced control systems allow small businesses to maximize performance and reduce downtime, further boosting their cost-effectiveness.
One of the critical aspects of implementing steam engines in small businesses is the training and technical knowledge required to operate and maintain the systems effectively. Fortunately, many manufacturers of steam engine systems offer comprehensive training and ongoing support to help small businesses maximize the lifespan and efficiency of their systems. This support can include everything from installation assistance to troubleshooting, ensuring that small business owners are equipped to handle the day-to-day management of their steam engines.
For businesses that are new to steam power or have limited technical experience, some companies provide fully managed services, including installation, maintenance, and monitoring. This option can take the burden off business owners, allowing them to focus on running their operations while ensuring that the steam engine systems are functioning optimally.
As businesses strive to keep costs down and remain competitive, steam engines offer an affordable and reliable alternative to traditional power generation methods. By utilizing steam to produce energy or heat, small businesses can create an independent and efficient energy system tailored to their specific needs. Moreover, the long lifespan and low operational costs associated with steam engines can make them a valuable investment for small businesses seeking to reduce energy costs and improve operational efficiency over time.
In addition, many small businesses can benefit from the ability to combine steam engines with other renewable energy systems, such as solar or wind power. This hybrid approach not only enhances energy security but also positions businesses as environmentally responsible, which can lead to favorable market recognition and potentially attract new customers.
While the initial cost of implementing steam engine systems may seem high for some small businesses, the long-term benefits often outweigh the upfront investment. Over time, businesses can recoup their initial expenditure through reduced energy costs, improved process efficiency, and a more sustainable energy infrastructure. Additionally, as renewable energy becomes more prominent, there may be incentives, rebates, or subsidies available to offset the cost of installing renewable energy-based steam systems.
Ultimately, affordable steam engine solutions for small businesses are becoming more accessible and practical than ever before. With advances in technology, flexible design options, and financial incentives, steam engines are no longer just a relic of the past but a viable and sustainable energy solution for modern small businesses. By incorporating steam power into their operations, small businesses can lower costs, improve efficiency, and take an active role in reducing their environmental impact, all while increasing their energy independence and competitiveness in their respective industries.
Steam Engine Performance Metrics and Specifications
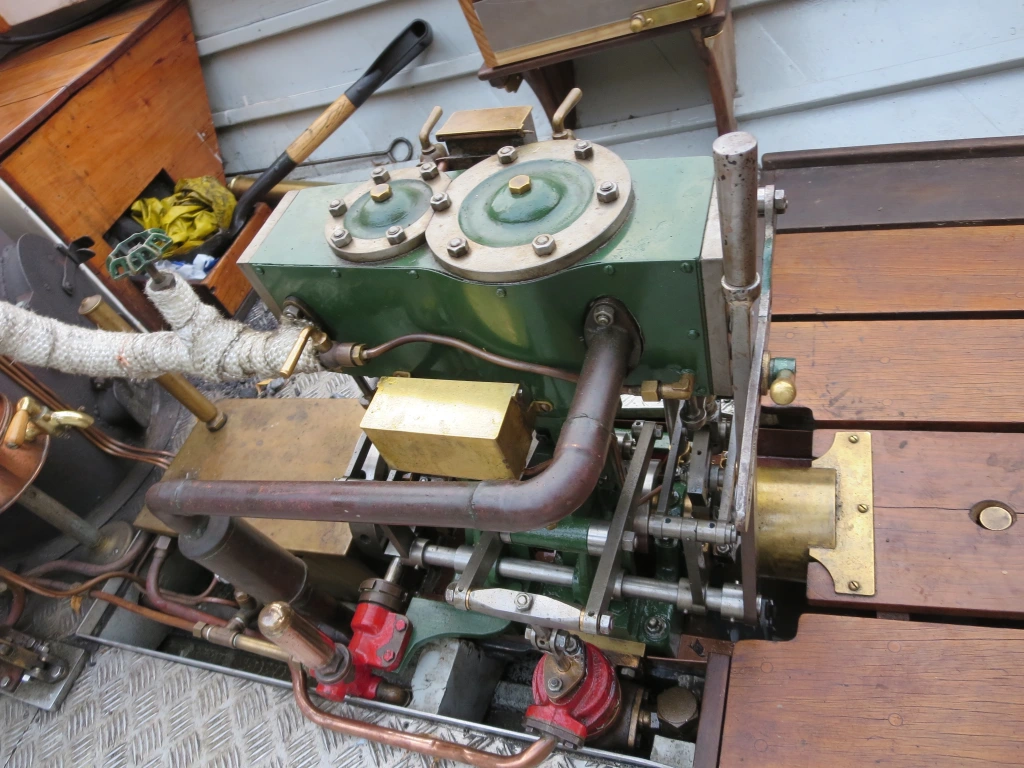
Steam engine performance metrics and specifications are critical for assessing the efficiency, reliability, and operational suitability of a steam engine in various applications. These metrics allow engineers, operators, and businesses to optimize steam engine performance, compare different systems, and ensure the engine meets the required standards for specific industrial tasks. Here are the key performance metrics and specifications typically used in evaluating steam engines:
1. Thermal Efficiency
Thermal efficiency measures the percentage of energy from fuel that is successfully converted into usable work or power by the steam engine. This metric is a critical indicator of how efficiently the engine converts the thermal energy from steam into mechanical energy. Higher thermal efficiency means less fuel consumption for the same amount of output.
- Formula:
Thermal Efficiency=Useful Work OutputEnergy Input from Fuel×100%\text{Thermal Efficiency} = \frac{\text{Useful Work Output}}{\text{Energy Input from Fuel}} \times 100\%Thermal Efficiency=Energy Input from FuelUseful Work Output×100%
2. Mechanical Efficiency
Mechanical efficiency refers to the ratio of the useful mechanical work produced by the engine compared to the total energy input from the steam. This efficiency is a measure of how well the steam engine converts steam energy into mechanical power while accounting for losses such as friction, heat loss, and other mechanical inefficiencies.
- Formula:
Mechanical Efficiency=Mechanical Work OutputTotal Energy Input×100%\text{Mechanical Efficiency} = \frac{\text{Mechanical Work Output}}{\text{Total Energy Input}} \times 100\%Mechanical Efficiency=Total Energy InputMechanical Work Output×100%
3. Power Output
Power output is a key metric that indicates the total amount of work a steam engine can produce over time. It is typically measured in horsepower (HP) or kilowatts (kW). The power output depends on the engine’s size, design, steam pressure, and steam flow rate.
- Formula:
Power Output (kW)=Steam Flow Rate (kg/s)×Enthalpy Difference (kJ/kg)1000\text{Power Output (kW)} = \frac{\text{Steam Flow Rate (kg/s)} \times \text{Enthalpy Difference (kJ/kg)}}{1000}Power Output (kW)=1000Steam Flow Rate (kg/s)×Enthalpy Difference (kJ/kg)
4. Steam Pressure
Steam pressure is one of the most important specifications, as it determines the energy content of the steam. Higher steam pressure increases the engine’s power output and efficiency but also requires more robust components, such as boilers and turbines, to handle the increased pressure. Steam pressure is usually measured in bars or pascals (Pa).
- Range:
Steam pressure typically ranges from 1 bar for low-pressure systems to 100 bars or more for high-pressure industrial engines.
5. Steam Temperature
Steam temperature, along with steam pressure, dictates the energy available in the steam. High temperatures increase the overall energy content and efficiency of the steam. For example, superheated steam, which is steam heated above its boiling point, can improve the efficiency of steam engines by reducing the risk of condensation within the engine components.
- Units:
Steam temperature is measured in degrees Celsius (°C) or degrees Fahrenheit (°F).
6. Specific Steam Consumption (SSC)
Specific Steam Consumption (SSC) is a measure of the amount of steam required to produce one unit of power (usually in horsepower or kilowatts). It is used to assess the efficiency of the engine in terms of steam usage.
- Formula:
SSC=Steam Flow Rate (kg/h)Power Output (kW)\text{SSC} = \frac{\text{Steam Flow Rate (kg/h)}}{\text{Power Output (kW)}}SSC=Power Output (kW)Steam Flow Rate (kg/h) Lower SSC values indicate better efficiency, as less steam is required to generate the same amount of power.
7. Boiler Efficiency
Boiler efficiency measures how effectively the boiler converts fuel into steam, which is a key factor in overall steam engine efficiency. Higher boiler efficiency results in less fuel consumption for steam production.
- Formula:
Boiler Efficiency=Heat Used to Generate SteamHeat Supplied by Fuel×100%\text{Boiler Efficiency} = \frac{\text{Heat Used to Generate Steam}}{\text{Heat Supplied by Fuel}} \times 100\%Boiler Efficiency=Heat Supplied by FuelHeat Used to Generate Steam×100%
8. Exhaust Temperature
Exhaust temperature is the temperature of the steam or gases leaving the engine after performing work. Lower exhaust temperatures indicate that the engine is effectively converting steam energy into mechanical energy rather than wasting heat.
- Units:
Exhaust temperature is typically measured in Celsius (°C) or Fahrenheit (°F).
9. Cylinder Efficiency
In engines with cylinders, cylinder efficiency is a measure of how efficiently the steam expands and does work inside the cylinder. Steam engines often use multiple expansion stages to improve cylinder efficiency, as steam can expand in stages to extract more mechanical energy from a given amount of steam.
10. Startup Time
Startup time is the time it takes for the steam engine to reach operational speed and start generating power. A faster startup time is desirable for systems that require rapid response, such as in emergency power generation or certain manufacturing processes.
- Units:
Startup time is typically measured in minutes.
11. Load Capacity
Load capacity refers to the maximum amount of load or power that a steam engine can handle without being overloaded. This is crucial in applications where the steam engine will be powering machinery or equipment, as it determines how much work the engine can handle before it reaches its operational limits.
- Units:
Load capacity is measured in horsepower (HP) or kilowatts (kW).
12. Durability and Maintenance Requirements
The durability of a steam engine is a measure of its ability to operate reliably over time with minimal downtime and maintenance. Engines designed for industrial applications typically have longer lifespans and require regular maintenance to maintain optimal performance. This includes checks for wear and tear, lubrication, and the condition of steam-related components such as the boiler, valves, and turbines.
13. Vibration and Noise Levels
Vibration and noise levels are important for the comfort and safety of the operating environment. Excessive vibration can cause mechanical stress, reduce component lifespans, and even lead to failures. Steam engines are often equipped with vibration dampeners or are designed to minimize noise in industrial settings.
- Units:
Vibration is typically measured in mm/s or inches per second (ips), while noise levels are measured in decibels (dB).
14. Fuel Type and Fuel Efficiency
The type of fuel used in a steam engine, whether coal, oil, biomass, or natural gas, significantly affects its efficiency and operational costs. Each fuel type has different energy densities and environmental impacts. The fuel efficiency metric indicates how much power is produced per unit of fuel consumed, with higher efficiency resulting in less fuel usage and lower operational costs.
15. Emissions (CO2, NOx, SOx)
In the context of modern steam engines, emissions are an important specification, particularly for industries aiming to meet environmental regulations. Monitoring CO2, nitrogen oxides (NOx), and sulfur oxides (SOx) is crucial for assessing the environmental impact of the steam engine.
Conclusion
The performance of a steam engine is determined by a combination of factors including thermal efficiency, mechanical efficiency, power output, and fuel consumption. These specifications allow businesses and engineers to select the right steam engine for their particular needs, whether it’s for power generation, industrial applications, or heating. Regular monitoring of these metrics is essential for optimizing performance, ensuring sustainability, and reducing operational costs in steam engine systems.
When evaluating steam engines, understanding how they perform under different conditions is crucial. Factors like the type of steam (saturated or superheated), load variation, and operational environment all influence the overall efficiency and effectiveness of the engine. The actual performance often varies with these parameters, which is why constant monitoring is necessary to ensure that the engine is running at its optimal level.
In industries where steam engines are used for continuous operations, such as power plants or large manufacturing setups, performance metrics like uptime and reliability become key considerations. Ensuring that steam engines maintain consistent power output without unexpected failures helps in maintaining production schedules and minimizing costly downtime. Engine components such as bearings, pistons, and steam valves need to be regularly checked to prevent wear and tear, which can compromise performance.
Additionally, automation and control systems play an important role in maintaining engine performance by adjusting parameters like steam pressure, flow rate, and temperature. This allows for real-time adjustments that can improve efficiency and prevent overheating or overloading of components. Advanced control systems with sensors can automatically adjust steam generation to match the load demand, ensuring that energy is used efficiently and reducing excess fuel consumption.
In terms of long-term operational efficiency, maintaining an engine’s heat balance is critical. Heat recovery systems that capture waste heat can improve the overall thermal efficiency of a steam engine by utilizing exhaust gases or other heat sources to preheat water or steam. This process reduces the energy input required to generate steam, ultimately lowering fuel consumption and increasing the engine’s performance.
For modern steam engines, incorporating advanced materials into the manufacturing process has become a key focus area. High-strength, corrosion-resistant materials are used to improve the durability and longevity of steam engine components, such as the boiler, condenser, and turbine blades. These materials help in resisting wear from high-pressure steam and reduce the need for frequent maintenance or component replacement. Innovations in materials science, such as composite alloys and ceramic coatings, also enable steam engines to operate at higher temperatures and pressures, further improving efficiency.
Another emerging trend is the use of hybrid systems that combine steam engines with renewable energy sources such as solar, wind, or biomass. These hybrid systems can allow industries to shift towards cleaner energy solutions without completely abandoning the proven reliability and power output of steam engines. Such setups also make use of energy storage systems that can store excess power generated during peak periods, which can be released during times of high demand, further enhancing overall operational efficiency.
Furthermore, data analytics and predictive maintenance technologies are becoming increasingly important in optimizing steam engine performance. By collecting data on engine conditions and performance metrics, companies can predict when maintenance will be needed and identify potential problems before they cause breakdowns. This approach helps reduce the risk of unplanned outages and ensures that the engine operates at peak efficiency over its lifespan. Technologies like condition monitoring sensors and real-time performance tracking are now commonly integrated into modern steam engine systems.
Despite the advancements in steam engine technology, the system’s overall cost of ownership remains a significant factor in decision-making. Beyond the initial capital expenditure for the engine and installation, businesses need to consider long-term operating costs such as fuel, maintenance, spare parts, and labor. While steam engines can be more expensive upfront compared to other energy generation systems, the long-term savings in energy costs and maintenance might make them a more cost-effective solution, particularly in industries with high energy demands.
It is also worth noting that, for many industries, steam engines are not standalone systems but are part of larger, integrated energy solutions. In these setups, steam engines may be used in conjunction with other forms of energy generation, such as gas turbines, and can be integrated into cogeneration or combined heat and power (CHP) systems, where both electricity and useful heat are produced simultaneously. This approach maximizes energy efficiency and can provide substantial savings on heating and electricity costs.
In terms of environmental impact, steam engines are often viewed with skepticism due to the emissions produced from burning fossil fuels. However, advancements in carbon capture technologies and the use of biofuels, such as wood pellets or agricultural waste, have significantly reduced the environmental footprint of steam engines. The shift toward green steam generation is expected to continue, with companies adopting cleaner fuel alternatives and reducing CO2 emissions.
In conclusion, steam engine performance is influenced by a wide range of factors, from mechanical design to operational conditions and maintenance strategies. Performance metrics like thermal and mechanical efficiency, steam pressure, power output, and fuel consumption offer valuable insights into how well an engine is operating. By continuously monitoring these metrics and implementing technologies like automation, advanced materials, and data analytics, industries can maximize the efficiency and longevity of their steam engines, while minimizing their environmental impact and operational costs.
Steam Engine Troubleshooting and Solutions
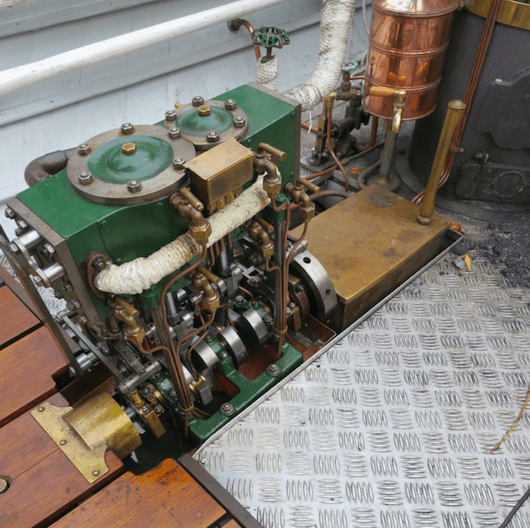
Steam engine troubleshooting and solutions are essential for maintaining the efficiency and reliability of these systems. Regular maintenance, combined with a deep understanding of the potential issues and remedies, can help avoid costly downtime and ensure that steam engines continue to perform at optimal levels. Below are common problems associated with steam engines, potential causes, and solutions for each:
1. Low Power Output
Low power output is a common issue that can stem from various causes, affecting engine efficiency and performance.
- Possible Causes:
- Insufficient steam pressure or temperature
- Low fuel quality or improper combustion
- Steam leaks in pipes or joints
- Inadequate maintenance of the turbine or piston components
- Solutions:
- Check Steam Pressure: Ensure that the boiler is generating the required steam pressure. If pressure is too low, inspect the boiler system for issues like inefficient combustion, fouling, or leaks.
- Increase Fuel Quality: If the fuel is subpar, switch to a higher-quality fuel or adjust combustion settings for better efficiency.
- Seal Steam Leaks: Identify and fix any steam leaks in pipes, valves, or joints that may be reducing steam efficiency.
- Inspect Engine Components: Regularly maintain turbine blades, pistons, and bearings to prevent mechanical inefficiencies. Check for any wear and tear that may cause a decrease in output.
2. Overheating of the Engine
Excessive heat is detrimental to the engine’s performance and longevity, leading to damage and even failure.
- Possible Causes:
- Faulty cooling system or inadequate cooling water flow
- High steam pressure causing the engine components to overheat
- Poor lubrication leading to friction and excessive heat generation
- Solutions:
- Check Cooling System: Ensure that the engine’s cooling system is working properly. Inspect for blockages, leaks, or low water levels that could impair cooling. Cleaning heat exchangers and radiators can improve heat dissipation.
- Adjust Steam Pressure: If the steam pressure is too high, reduce it to prevent excessive temperatures in the engine components.
- Improve Lubrication: Regularly check lubrication levels and apply the correct type of oil to ensure smooth moving parts and prevent overheating due to friction.
3. Excessive Vibration
Vibration in a steam engine can lead to component damage, noise, and decreased operational efficiency.
- Possible Causes:
- Misalignment of engine components, such as the crankshaft or turbine blades
- Worn or damaged bearings and supports
- Steam pressure fluctuations or improper loading
- Solutions:
- Align Components: Perform regular alignment checks for the crankshaft, flywheel, and turbine components. Misalignment can cause imbalanced loads and excessive vibrations.
- Replace Worn Bearings: Inspect bearings, supports, and other moving parts for wear and tear. Replace damaged components as needed to ensure smooth operation.
- Stabilize Pressure: Ensure that the steam pressure is stable and within the recommended range to avoid fluctuations that could lead to vibrations.
4. Steam Leaks
Leaks in the steam engine system can drastically reduce efficiency, waste energy, and pose a safety hazard.
- Possible Causes:
- Deterioration of seals, gaskets, and joints over time
- Incorrectly tightened or damaged piping
- Corrosion or buildup around connections
- Solutions:
- Inspect Seals and Gaskets: Regularly check seals and gaskets for wear and tear. Replace any damaged components to ensure airtight connections.
- Tighten or Replace Pipes: Inspect all pipe joints and connections for tightness. If necessary, replace damaged sections of piping to prevent steam loss.
- Corrosion Prevention: Apply protective coatings to components exposed to moisture and high temperatures to reduce corrosion and prolong the life of the system.
5. Water Hammer
Water hammer is a potentially destructive phenomenon that occurs when steam condenses rapidly, creating a shockwave that can damage components.
- Possible Causes:
- Water accumulation in the steam pipes
- Inadequate drainage or improper steam trap function
- Rapid changes in steam pressure
- Solutions:
- Ensure Proper Drainage: Regularly check the steam system for water accumulation and ensure that proper drainage and steam traps are functioning. Water should not be allowed to accumulate in the pipes.
- Install or Maintain Steam Traps: Steam traps help to remove condensate from the system. Make sure they are correctly installed and functioning to prevent water hammer.
- Control Pressure Changes: Avoid sudden pressure changes in the system, as they can contribute to water hammer. Maintain a steady steam flow to prevent rapid condensation.
6. Low Efficiency Due to Scale or Sludge Buildup
Over time, scale, sludge, and mineral deposits can build up in the boiler and steam lines, reducing efficiency and potentially causing damage.
- Possible Causes:
- Hard water or improper water treatment
- Insufficient boiler cleaning
- Poor heat transfer due to scale buildup
- Solutions:
- Implement Water Treatment: Use water softeners or other treatments to prevent scale buildup. Ensure that the water used in the boiler is of the appropriate quality.
- Regular Cleaning: Schedule routine cleaning of the boiler to remove scale and sludge. This includes chemical cleaning and descaling procedures.
- Monitor Water Quality: Regularly test and monitor the water quality for hardness and other contaminants that can contribute to scaling.
7. Faulty Boiler Operation
The boiler is a crucial part of the steam engine, and any malfunction can directly impact the engine’s performance.
- Possible Causes:
- Poor combustion due to improper fuel-air mixture
- Boiler tube leaks or corrosion
- Inefficient heat exchange due to fouling or blockage
- Solutions:
- Optimize Combustion: Adjust the fuel-air mixture for better combustion efficiency. This may involve tuning burners or replacing air filters to ensure optimal fuel combustion.
- Inspect for Leaks and Corrosion: Regularly inspect boiler tubes for leaks or signs of corrosion. Replace any damaged sections and apply anti-corrosion coatings where necessary.
- Clean Heat Exchange Surfaces: Clean the heat exchange surfaces of the boiler to ensure maximum heat transfer and avoid energy loss.
8. Control System Failures
Modern steam engines often feature computerized control systems that monitor and adjust various parameters such as steam pressure, temperature, and power output. A failure in these systems can lead to improper engine operation.
- Possible Causes:
- Faulty sensors or control circuits
- Software or calibration errors
- Lack of system updates or maintenance
- Solutions:
- Check Sensors and Wiring: Inspect all sensors and wiring for faults. Replace any malfunctioning components to restore proper control system functionality.
- Calibrate System: Ensure that the control system is correctly calibrated for the steam engine’s specifications. Perform regular software updates and maintenance to avoid errors.
- Manual Override: In case of a control system failure, ensure that manual control systems are available to manage the steam engine safely until the issue is resolved.
9. Excessive Noise
Excessive noise from a steam engine can be a sign of mechanical issues or improper operation.
- Possible Causes:
- Loose parts or fittings
- Worn bearings or misaligned components
- Pressure fluctuations or mechanical imbalances
- Solutions:
- Tighten Loose Parts: Regularly inspect and tighten any loose parts or fittings to prevent rattling or vibrations.
- Replace Worn Parts: Inspect bearings and components for wear, and replace any parts that are contributing to excess noise.
- Stabilize Steam Pressure: Ensure that steam pressure is stable and within the required limits to avoid loud operational sounds from pressure fluctuations.
Conclusion
Proper steam engine troubleshooting requires a methodical approach to identify the root causes of performance issues. Regular maintenance and the use of high-quality materials in steam systems can help mitigate common problems. Troubleshooting is not only about fixing immediate issues but also about optimizing long-term operation by improving system design, reducing wear, and ensuring efficiency. Keeping detailed logs, monitoring key performance metrics, and adhering to preventive maintenance schedules are essential to maintaining a steam engine’s reliability and performance over time.
To ensure that steam engines continue to perform at their best, it’s essential to adopt a proactive approach toward both maintenance and troubleshooting. Regular inspections and routine checks help identify potential issues before they escalate into significant problems. By addressing minor issues early, you can avoid costly repairs, extend the lifespan of the engine, and maintain optimal performance.
Preventative maintenance is key to preventing many of the common issues steam engines face. This includes regularly cleaning and replacing components that wear out over time, such as gaskets, seals, and bearings. Ensuring that steam pipes, valves, and boiler tubes are free from corrosion or scale buildup is also crucial for maintaining performance and avoiding blockages. Similarly, proper water treatment systems can prevent mineral deposits from forming inside the engine, which can hinder heat transfer and lower overall efficiency.
In addition to regular maintenance, employing advanced technologies such as condition monitoring sensors can provide real-time data on the engine’s performance, allowing operators to spot inefficiencies or early signs of wear before they become critical. These systems can alert technicians to potential issues such as pressure fluctuations, excessive temperatures, or abnormal vibrations, enabling timely intervention and avoiding unexpected downtime.
Another important aspect of troubleshooting is the ongoing training of personnel. Operators should be familiar with common steam engine issues and the solutions for each. Having a well-trained team ensures that any abnormalities or performance issues are identified and dealt with quickly. This reduces the risk of human error and increases the likelihood that the engine will be operated at peak efficiency. Furthermore, staff should be trained in emergency response procedures in case of a critical failure, ensuring that the steam engine can be shut down safely without causing harm to the system or personnel.
In cases where major repairs or component replacements are needed, it’s essential to use high-quality, compatible parts. Utilizing OEM (original equipment manufacturer) or certified components ensures that the replacement parts will perform to the same standards as the original ones, maintaining the engine’s efficiency and safety. Additionally, opting for upgraded or advanced components, such as more efficient turbines or heat exchangers, can significantly improve the engine’s overall performance and efficiency.
For large-scale operations, integrating steam engines into a broader energy management system can help optimize their performance. For example, using steam engines in cogeneration or combined heat and power (CHP) systems can increase overall efficiency by capturing waste heat and utilizing it for other processes. This not only makes better use of the energy produced but also reduces the environmental impact by minimizing energy waste.
While steam engines remain a reliable and powerful energy source for many industries, understanding their limitations and weaknesses is important for troubleshooting. For instance, steam engines are generally not as efficient as newer technologies, such as gas turbines, and they can be prone to wear from constant high-temperature, high-pressure environments. However, these challenges can be overcome through good maintenance practices, careful operation, and technological upgrades.
In modern industrial applications, the demand for energy efficiency and sustainability is higher than ever. Many companies are turning to steam engine retrofitting or hybrid solutions to enhance energy recovery and reduce fuel consumption. For example, integrating steam turbines with renewable energy sources like solar or biomass can help reduce dependence on fossil fuels and lower the carbon footprint of steam engine systems. This shift to cleaner energy sources is not only beneficial for the environment but also provides a competitive advantage for businesses aiming to meet sustainability goals.
Ultimately, steam engine troubleshooting and solutions are about more than just addressing immediate issues—they are about maintaining a system that is reliable, efficient, and cost-effective over the long term. By implementing comprehensive maintenance plans, using modern diagnostic tools, and investing in high-quality components, industries can ensure that their steam engines continue to operate smoothly and meet performance expectations. Whether you are dealing with minor inefficiencies or major breakdowns, a systematic and informed approach will help keep the steam engine in top shape, minimizing downtime and maximizing productivity.
Steam Engine Testing and Quality Assurance
Steam engine testing and quality assurance are critical aspects of ensuring that steam engines meet performance standards, operate efficiently, and have a long service life. Comprehensive testing processes and stringent quality assurance protocols are necessary for verifying that the steam engine performs reliably under the expected operational conditions, with safety being a top priority. Here’s an overview of the essential steps involved in steam engine testing and quality assurance:
1. Pre-Installation Testing
Before a steam engine is installed in a facility or integrated into an industrial system, several tests are carried out at the manufacturing facility to verify the performance and safety of its components. These include:
- Component Inspection: All individual components (boiler, turbine, pump, and condenser) undergo rigorous inspection to check for defects, material integrity, and conformance to specifications.
- Pressure Tests: High-pressure tests are conducted on the boiler and steam piping system to ensure that they can safely handle the required steam pressure without leaks or failures. This ensures the system is robust enough to withstand real-world operating conditions.
- Dimensional and Tolerance Checks: Manufacturers ensure that all parts are fabricated to precise dimensions, with clearances and tolerances that prevent mechanical failures or inefficiencies.
- Material Testing: Various materials used in steam engine construction, such as metals for boilers and turbines, undergo tests to verify their durability, resistance to heat and corrosion, and overall mechanical strength.
2. Factory Acceptance Testing (FAT)
Once the steam engine has been assembled, a factory acceptance test is conducted to verify that the engine meets design specifications and operational criteria before leaving the manufacturing facility. This test includes:
- Operational Testing: The engine is run at various loads to evaluate how it performs under different operational conditions. This involves testing for power output, fuel consumption, steam pressure, temperature, and efficiency.
- Safety Features Check: All safety features such as pressure relief valves, temperature sensors, and emergency shutdown mechanisms are thoroughly tested to ensure they function correctly.
- Noise and Vibration Testing: The engine is examined for unusual vibrations or excessive noise, which can indicate mechanical problems or inefficiencies. Vibration analysis helps to identify misalignments or imbalances in rotating components.
- System Integration Test: The engine is tested as part of a complete system, simulating its actual operating environment to verify how well it interacts with other components, such as the control systems, power generation systems, or ancillary equipment.
3. Installation and Commissioning Tests
Once the steam engine is installed at the operational site, additional tests are performed during the commissioning phase. These tests ensure that the engine is correctly installed and integrates well with the broader system. Tests conducted during this phase include:
- Startup Testing: This involves carefully starting the steam engine and monitoring its performance as it comes online. The steam pressure, temperature, and flow rates are carefully monitored to ensure they align with specified limits.
- Load Testing: The engine is tested under full load conditions to evaluate how it performs under maximum operational capacity. Load testing helps identify potential issues that might arise when the engine is subjected to continuous high-demand operation.
- Performance Verification: The engine’s performance is compared against manufacturer specifications and benchmarks to ensure that it meets expected efficiency levels and power output.
- Control System Integration Testing: The engine’s control system is thoroughly tested to verify that it responds correctly to commands and signals. The control systems are calibrated to optimize engine performance.
4. Ongoing Performance Monitoring
Once the steam engine is operational, continuous performance monitoring is essential to ensure the engine remains within optimal operating conditions. This can include:
- Condition Monitoring: Real-time sensors and monitoring systems track key performance indicators, such as steam pressure, temperature, vibration, and exhaust emissions. By constantly monitoring these parameters, operators can detect any deviations from expected performance and make adjustments or repairs as needed.
- Efficiency Audits: Regular performance audits assess the engine’s fuel efficiency, steam-to-electricity conversion rate, and overall energy usage. This allows for identifying areas of improvement or modifications to reduce fuel consumption and improve the engine’s output.
- Predictive Maintenance: Using historical data and condition monitoring, predictive analytics can forecast potential failures and maintenance needs, allowing for preventive maintenance to be performed before problems arise. This helps reduce downtime and extend the engine’s lifespan.
5. Quality Assurance Protocols
Quality assurance in steam engine manufacturing and operation focuses on ensuring that all components and systems meet or exceed predefined quality standards. It involves:
- Standardized Testing Procedures: Manufacturers follow internationally recognized testing standards, such as those outlined by the American Society of Mechanical Engineers (ASME) or ISO certifications, to ensure consistency and reliability in performance.
- Third-Party Inspections: Many steam engine manufacturers subject their products to third-party inspections and certifications. These external auditors evaluate the quality of materials, assembly processes, and testing procedures, providing an additional layer of confidence in the engine’s quality.
- Documentation and Certification: Complete records of all testing, inspections, and performance metrics are maintained, allowing for traceability of every steam engine component and test. This documentation serves as a quality assurance audit trail that proves compliance with safety and performance standards.
- Compliance with Environmental Standards: Steam engines are subject to environmental regulations, such as emissions standards. Quality assurance checks ensure that the engine adheres to relevant environmental requirements, including low emissions, noise control, and efficiency.
6. Post-Installation Testing and Maintenance
After installation and commissioning, the steam engine will undergo a series of post-installation checks to verify that it continues to operate at peak performance. These include:
- Performance Testing after Commissioning: A final round of testing is done after the engine has been running for a period, ensuring that all systems are fully integrated and functioning optimally.
- Routine Maintenance Checks: Scheduled maintenance ensures that the engine operates efficiently. This may include cleaning, lubrication, and part replacements based on the manufacturer’s recommended intervals.
- Troubleshooting and Problem-Solving: If performance issues arise, targeted troubleshooting tests are performed to identify the cause. Common tests include checking the boiler pressure, inspecting turbine operation, and analyzing vibration levels.
7. Long-Term Quality Assurance
To ensure that the steam engine continues to meet performance standards over its lifespan, long-term quality assurance practices are necessary. These include:
- Long-Term Performance Monitoring: Operators track the engine’s performance over time, adjusting operational parameters as necessary to optimize efficiency.
- Re-certification: Periodic re-certification and compliance checks may be required to meet updated regulations, ensuring that the steam engine remains compliant with industry standards.
- Upgrades and Retrofitting: As new technologies become available, steam engines may undergo retrofitting or upgrades to improve performance, enhance efficiency, or reduce emissions. These upgrades are tested and validated to ensure compatibility with existing systems.
Conclusion
Steam engine testing and quality assurance are fundamental to ensuring reliable and efficient operation, whether the engine is used for industrial power generation, marine transport, or smaller-scale applications. Through rigorous pre-installation testing, thorough commissioning checks, ongoing performance monitoring, and adherence to industry standards, steam engines can be kept in top operating condition throughout their service life. These practices not only ensure that the engines meet the required performance and safety standards but also contribute to extended operational lifespans, lower maintenance costs, and greater overall efficiency.
The process of steam engine testing and quality assurance extends beyond just initial installation and commissioning. It involves continuous monitoring, maintenance, and performance validation to ensure that the engine operates efficiently and reliably throughout its operational life. Beyond simply meeting safety standards and performance metrics, the goal is to optimize the engine’s output, reduce operational costs, and extend the lifespan of the system.
Long-term operational efficiency relies heavily on the continued performance of the steam engine’s components. Regular inspections allow for early identification of wear and tear, which can be mitigated through timely maintenance and part replacements. It is crucial that these inspections are conducted systematically, often based on a set schedule or in response to specific performance metrics being outside normal ranges. As steam engines age, certain components may require more frequent checks or may need to be upgraded to newer, more efficient alternatives.
Technological advancements play a key role in optimizing steam engine performance. Advanced diagnostic tools such as thermal imaging, ultrasonic testing, and vibration analysis can help identify issues that are not immediately visible to the naked eye, such as internal corrosion, material fatigue, or misalignments. These technologies help reduce downtime by allowing for proactive repairs and adjustments, ensuring that the engine continues to perform at its best.
Another aspect of ongoing quality assurance is the integration of modern software systems. Computerized control systems can continuously monitor and adjust parameters like steam pressure, temperature, and flow rates, optimizing performance without requiring manual intervention. Additionally, these systems can store historical data, allowing engineers to track trends over time and predict future issues. Predictive maintenance tools, powered by machine learning and artificial intelligence, can use this data to forecast when certain components are likely to fail, enabling the organization to take preventive actions before a failure occurs.
Operators should also stay updated with regulatory requirements. As environmental standards evolve, steam engines may need to be retrofitted or modified to comply with new emission limits or efficiency standards. In many cases, upgrades such as improved insulation, more efficient heat exchangers, or advanced filtration systems can make older engines more competitive and environmentally friendly.
Finally, the emphasis on sustainability has led many industries to explore alternative fuel sources and hybrid technologies in their steam engines. Incorporating biomass, solar energy, or waste heat recovery systems into steam engine operations can help reduce carbon footprints and lower energy costs, all while maintaining or improving the engine’s performance. These systems not only offer a cleaner energy alternative but also present opportunities for innovation in steam engine design and operation.
In summary, steam engine testing and quality assurance are critical for ensuring that engines remain safe, efficient, and reliable throughout their lifespan. Regular testing, monitoring, and maintenance ensure that the engine performs optimally, minimizes downtime, and meets both performance and environmental standards. By integrating advanced diagnostic tools, predictive maintenance, and modern software systems, companies can enhance operational efficiency, reduce costs, and extend the longevity of their steam engine systems. Ultimately, this proactive approach to steam engine management leads to more sustainable, reliable, and cost-effective operations in industries worldwide.
Steam Engine Innovations and Future Trends
The future of steam engine technology is shaped by a combination of historical legacy and cutting-edge innovations aimed at improving efficiency, reducing environmental impact, and expanding the range of applications. Despite being one of the oldest power generation technologies, steam engines continue to evolve. Innovations in materials, energy recovery, automation, and hybrid systems are all paving the way for a modern revival of steam power in a wide range of industries. Here’s a look at some of the key innovations and future trends shaping the steam engine industry:
1. Enhanced Efficiency Through Advanced Materials
One of the major drivers of innovation in steam engine technology is the development of new materials. Modern steam engines benefit from high-performance alloys and composites that can withstand higher temperatures and pressures without degrading. These materials improve thermal efficiency, reduce corrosion, and extend the lifespan of critical components such as boilers, turbines, and condensers. Advanced coatings and corrosion-resistant materials are helping to reduce maintenance costs and increase the operational life of steam engine systems, especially in harsh industrial environments.
2. Hybrid Steam Systems with Renewable Energy Integration
As industries look for ways to reduce their carbon footprints, there is growing interest in integrating steam engines with renewable energy sources such as solar, wind, and biomass. Hybrid steam systems, which combine traditional steam engines with renewable energy technologies, allow for more sustainable energy production. For instance, waste heat recovery from industrial processes or biomass combustion can feed steam engines, reducing the need for fossil fuels and improving overall efficiency. This integration not only reduces emissions but also optimizes fuel use by taking advantage of multiple energy sources in parallel.
3. Advanced Control Systems and Automation
The automation of steam engine systems is another key area of innovation. Modern steam engines now incorporate advanced control systems that allow for precise regulation of steam pressure, temperature, and flow rate. These systems are typically integrated into a broader energy management system, allowing for real-time monitoring and adjustment of engine performance based on demand. Automated systems can detect inefficiencies, monitor equipment health, and adjust operational parameters to optimize performance, all without manual intervention. This automation improves the safety, reliability, and efficiency of steam engines, and minimizes the chances of human error.
4. Waste Heat Recovery and Cogeneration
One of the most promising innovations in steam engine technology is the increased use of waste heat recovery and cogeneration. Waste heat recovery systems capture excess heat that would otherwise be lost to the environment and use it to generate additional power or pre-heat fluids. Cogeneration systems, which combine power generation with heat production, are increasingly being used in industries such as chemical manufacturing, steel production, and food processing. These systems can significantly improve overall energy efficiency by utilizing the same steam engine for both electricity generation and heating processes, making them an attractive option for energy-conscious industries.
5. Miniaturization and Portable Steam Engines
While steam engines are often associated with large industrial applications, there is increasing interest in the miniaturization of steam engine technology for smaller-scale applications. Small-scale, portable steam engines are becoming more viable for niche markets such as backup power generation, remote operations, and even home energy systems. Innovations in miniaturized turbines, lightweight materials, and compact boilers are making it possible to use steam power in a broader range of contexts. These portable systems can offer efficient, reliable power in areas where grid access is limited or non-existent.
6. Carbon Capture and Environmental Compliance
As part of the push towards greener technologies, steam engines are being adapted to meet modern environmental regulations. In many applications, particularly those in power generation, the steam engine’s role in contributing to CO2 emissions is a concern. However, emerging technologies in carbon capture and storage (CCS) are being integrated into steam-based systems to reduce their environmental impact. By capturing carbon dioxide emissions and storing them safely, these systems help mitigate the carbon footprint of steam engines and make them more compatible with global efforts to combat climate change. In addition, improvements in steam engine efficiency lead to lower fuel consumption and, consequently, reduced emissions.
7. Digital Twins and Predictive Maintenance
The use of digital twin technology is transforming the way steam engines are monitored, managed, and maintained. A digital twin is a virtual model of a physical system, allowing engineers to simulate, predict, and optimize performance without relying solely on physical testing. For steam engines, digital twins provide real-time data from sensors embedded in the engine to create a dynamic simulation of its operations. This data is analyzed to identify potential issues, optimize performance, and predict failures before they happen. Predictive maintenance, powered by machine learning algorithms, helps operators determine the best time for maintenance, preventing unnecessary downtime and improving the reliability of the engine.
8. Superheated Steam and Advanced Thermodynamics
The use of superheated steam is an ongoing innovation that enhances the thermodynamic efficiency of steam engines. Superheated steam, which is steam heated beyond its boiling point, has a higher energy content, allowing steam engines to achieve higher efficiency and better performance. Advances in thermodynamic cycles and turbine designs are optimizing the generation of superheated steam, enabling steam engines to operate more efficiently at higher pressures and temperatures. This innovation is particularly beneficial in power generation, where efficiency gains translate to significant cost savings and reduced fuel consumption.
9. Advanced Turbine Technologies
Innovations in turbine design and materials have also contributed significantly to the evolution of steam engines. Modern turbines are more efficient and capable of handling higher speeds and pressures. Newer turbine designs focus on minimizing mechanical losses and improving energy conversion efficiency. For example, multi-stage turbines, which use multiple stages to gradually expand steam, increase the energy extraction from the steam, leading to improved performance. Coupled with advanced bearing systems, new materials such as ceramics, and better cooling techniques, turbines are becoming more robust, reliable, and energy-efficient.
10. Artificial Intelligence and Machine Learning
The integration of artificial intelligence (AI) and machine learning (ML) technologies into steam engine systems is enhancing operational efficiency and enabling more sophisticated performance optimization. AI can be used to analyze large amounts of data from steam engine sensors to detect patterns and trends that might not be immediately visible to human operators. Machine learning algorithms can optimize engine operations based on real-time conditions, improving efficiency, reducing energy consumption, and anticipating maintenance needs. This form of intelligent automation is becoming a key part of the future of steam engine technology.
Conclusion
The future of steam engine technology is rich with innovation, driven by the need for greater efficiency, sustainability, and adaptability. As industries continue to prioritize energy efficiency and environmental responsibility, steam engines will evolve to meet these demands. From hybrid systems combining renewable energy with traditional steam power to advanced materials and automation technologies, the steam engine is poised to remain a vital part of the global energy landscape. Through innovations such as waste heat recovery, miniaturization, and the integration of digital technologies, steam engines are becoming more flexible, efficient, and environmentally friendly, opening new possibilities for their use in modern applications.
The advancements in steam engine technology are not just limited to efficiency and performance improvements; they also reflect broader shifts in global energy trends. For example, as more countries commit to reducing their carbon emissions and transitioning to greener energy sources, the demand for technologies that can complement renewable energy systems is growing. Steam engines, traditionally seen as fossil fuel-dependent machines, are evolving to play a more integrated role in sustainable energy systems. With their ability to use alternative fuels such as biomass and waste products, steam engines are becoming a crucial part of circular economies where waste is repurposed into energy.
As industries push toward greater decentralization of energy production, smaller-scale steam systems are becoming increasingly viable. These systems can be deployed in remote locations, providing reliable power and heating where grid infrastructure is inadequate or unavailable. The ability to produce energy locally not only reduces transmission losses but also enhances energy security and resilience, particularly in regions vulnerable to natural disasters or other disruptions in power supply. The growing interest in off-grid and microgrid solutions opens up new opportunities for steam engine applications in rural areas, remote facilities, and even for individual households looking for alternative energy solutions.
Furthermore, the rise of 3D printing and additive manufacturing technologies is poised to revolutionize steam engine production. These technologies allow for rapid prototyping and production of complex components that would have traditionally been difficult or costly to manufacture. With 3D printing, custom parts and components can be produced on-demand, reducing lead times, cutting costs, and enabling a higher degree of customization for specific industrial needs. This also reduces material waste, as components can be designed with minimal excess material, aligning with sustainability goals.
Steam engines are also being explored in new and innovative ways in niche markets. For instance, research into steam-powered vehicles is gaining traction, with several companies and engineers experimenting with the idea of steam-powered cars, trucks, and even aircraft. While these concepts face challenges in terms of weight, efficiency, and practical application compared to modern internal combustion engines, they present interesting possibilities for alternative propulsion systems. These efforts, though still in the early stages, demonstrate the potential for steam engines to find new life in sectors where alternative energy sources are highly desired.
In agriculture, steam-powered engines could offer solutions for small-scale farmers, particularly in developing regions. These engines can be used for powering irrigation systems, food processing machines, and even basic electricity generation. Their versatility and relatively low fuel requirements make them attractive options for rural communities that may not have reliable access to electricity. The integration of steam systems with other agricultural technologies, such as solar panels and wind turbines, could enable off-grid farms to become more self-sufficient and economically viable.
Another area where steam engine technology is evolving is in the field of energy storage. The potential for steam engines to be used in combination with thermal energy storage systems is an exciting development. In such systems, excess energy from renewable sources like solar or wind is converted into heat, which is stored in thermal storage units and later used to generate steam when needed. This can provide a continuous and stable power supply even when renewable energy production is intermittent, helping to balance supply and demand. By using steam engines in this way, renewable energy can be stored in a more efficient and cost-effective manner, supporting the ongoing shift toward clean energy systems.
With these ongoing innovations, the steam engine is shedding its old image as a relic of the past and transforming into a modern, adaptable, and sustainable power source. The continued research and development in materials, automation, hybrid systems, and applications in diverse industries ensure that steam engines will remain relevant and valuable in the energy landscape for many years to come. By embracing these innovations, industries can not only optimize the performance of their existing steam systems but also contribute to the development of a more sustainable, resilient, and energy-efficient future.
Sustainable Steam Engine Technologies
Sustainable steam engine technologies are emerging as a key component of the global push toward cleaner, more efficient, and renewable energy systems. These innovations are aimed at reducing the environmental impact of traditional steam engines while improving their efficiency, reliability, and versatility. Sustainable steam technologies focus on utilizing renewable resources, minimizing waste, and optimizing energy use to create a more sustainable energy future. Here are several key areas where sustainable steam engine technologies are making significant strides:
One of the most promising developments is the integration of biomass and waste-to-energy solutions into steam engines. Biomass fuels, such as wood chips, agricultural waste, and other organic materials, can be burned to produce steam, reducing reliance on fossil fuels and lowering greenhouse gas emissions. These renewable fuel sources not only provide a sustainable energy supply but also contribute to a circular economy by utilizing waste products that would otherwise be discarded. This approach reduces the environmental impact of steam engine operation while supporting sustainable farming and forestry practices.
Waste heat recovery is another important innovation driving sustainability in steam engine systems. Many industrial processes generate excess heat that is typically released into the environment without being used. However, with advancements in waste heat recovery technology, this excess heat can be captured and converted into useful steam. By utilizing waste heat, steam engines can operate more efficiently and reduce the overall energy consumption of industrial facilities. This process not only lowers operational costs but also reduces the need for additional energy inputs, making it a sustainable solution for energy-intensive industries.
Another significant area of innovation is the hybridization of steam engine systems with other renewable energy technologies, such as solar thermal and geothermal energy. In hybrid systems, steam engines are used in conjunction with renewable energy sources to provide reliable, consistent power generation. For example, solar thermal energy can be used to heat water or fluids to generate steam, which is then used to power steam engines. This reduces the reliance on fossil fuels, lowering emissions and enhancing the overall efficiency of the system. Hybridization with geothermal energy, which provides constant heat from the Earth’s core, can further optimize steam engine performance, providing a sustainable and continuous source of energy.
Additionally, carbon capture and carbon-neutral technologies are gaining attention in steam engine design. As industries face increasing pressure to reduce their carbon footprints, integrating carbon capture systems with steam engines can help mitigate their environmental impact. Carbon capture technology captures CO2 emissions from the combustion process and stores it safely, preventing it from entering the atmosphere. This makes steam engines a more viable option in industries where reducing greenhouse gas emissions is a top priority.
Advancements in thermal storage systems also contribute to the sustainability of steam engines. By coupling steam engines with advanced thermal storage systems, excess energy can be stored and used later, even when renewable sources like solar or wind are not available. This storage capability provides flexibility in energy production, ensuring that steam engines can operate efficiently and reliably while minimizing the need for additional fuel or energy input. Thermal storage systems are particularly useful in regions with fluctuating energy demands or in industries that require continuous power supply.
The use of advanced materials in steam engine components is further enhancing sustainability. Materials that can withstand higher temperatures and pressures allow steam engines to operate at greater efficiency levels while using less fuel. For example, the development of high-performance alloys and coatings has led to turbines and boilers that are more durable and resistant to wear and corrosion, extending their lifespan and reducing the need for frequent maintenance and replacements. These materials also contribute to the overall efficiency of the engine, reducing fuel consumption and emissions.
Digital monitoring and optimization technologies are also contributing to the sustainability of steam engines. By incorporating sensors, real-time data analysis, and advanced control systems, steam engines can be continuously monitored and adjusted to optimize their performance. These technologies help to reduce waste by ensuring that the engine operates at peak efficiency, while predictive maintenance tools enable early detection of potential issues, minimizing downtime and unnecessary repairs. Additionally, digital optimization allows for better integration of steam engines into larger energy systems, improving grid stability and reducing energy waste.
Finally, decentralized energy systems and off-grid solutions are becoming more popular as sustainable alternatives to traditional centralized power generation. Steam engines are increasingly being used in microgrid and off-grid applications, where they can provide power to remote communities, industrial operations, or even individual homes. By using locally available fuels, such as biomass or waste products, these small-scale steam systems can reduce dependence on centralized energy sources, lower transmission losses, and provide reliable power in areas with limited access to traditional grid infrastructure.
Sustainable steam engine technologies are transforming industries and applications across the globe. By focusing on renewable energy sources, waste heat recovery, advanced materials, and cutting-edge digital systems, steam engines are evolving into a more environmentally friendly and energy-efficient power solution. As these technologies continue to develop, steam engines will play an increasingly important role in helping industries meet their sustainability goals, reduce their carbon footprints, and contribute to a cleaner, more efficient energy future. The integration of these innovations into the steam engine landscape promises to offer cleaner, more reliable, and more affordable energy solutions for generations to come.
As we look further into the future of sustainable steam engine technologies, it’s clear that the development of green technologies and smart grid integration will continue to shape the landscape. One of the significant opportunities lies in combining steam engines with smart grids that can dynamically balance supply and demand. By integrating steam power into smart grids, energy distribution becomes more responsive and efficient, allowing for the smooth integration of intermittent renewable sources like solar and wind. This helps stabilize the grid, reducing the need for fossil-fuel-based backup power and making it easier to incorporate decentralized, local energy generation systems, including small-scale steam engines.
Moreover, distributed energy generation systems, powered by steam engines, could become increasingly commonplace in industrial and residential applications. For instance, industries could install compact, highly efficient steam engines onsite to meet their heating and power generation needs, while residential buildings in rural areas could utilize smaller steam systems powered by locally sourced biomass or waste materials. This localized approach reduces energy losses associated with long-distance transmission and increases resilience to power outages or disruptions in the central grid.
Energy efficiency will continue to be a focal point in steam engine development. One area of attention will be the use of heat exchangers to further improve thermal efficiency. Steam engines traditionally lose a significant amount of energy through heat dissipation, but new heat exchanger technologies, such as plate heat exchangers or multi-stage heat recovery systems, can improve the utilization of heat. By capturing more of this wasted energy and reusing it in the system, steam engines can operate more efficiently and with a reduced need for fuel, lowering their carbon emissions and operational costs.
Another important trend is the continued evolution of modular steam engine systems that offer flexibility in scaling and adapting to various applications. These modular systems can be used in everything from large power plants to remote villages, providing customizable solutions for a range of energy needs. These systems are designed for easier maintenance and upgrades, ensuring that as technology advances, existing infrastructure can be retrofitted to stay up-to-date with the latest innovations.
With the increasing focus on circular economy principles, steam engine technology can contribute to reducing waste and increasing the reuse of materials. For example, steam engines used in industrial applications can be designed for easier disassembly, with parts that can be recycled or refurbished for reuse. Additionally, steam engine systems can be designed with sustainability in mind, ensuring that each component has a minimal environmental impact throughout its life cycle—from production and use to end-of-life disposal or repurposing.
The transition to low-carbon steam technologies also opens up opportunities for partnerships and cross-industry collaboration. Collaborations between steam engine manufacturers, energy providers, and environmental organizations could help create more sustainable, energy-efficient solutions. For example, industries that traditionally rely on coal, natural gas, or other fossil fuels for steam generation could transition to hybrid or renewable steam solutions, supported by government incentives or carbon trading programs. By joining forces across sectors, companies can achieve shared sustainability goals while reducing the overall environmental footprint of their operations.
Finally, sustainable steam engine technologies are expected to become more affordable and accessible as manufacturing techniques improve and economies of scale come into play. As technologies mature and become more widely adopted, the cost of renewable steam engines and associated systems will likely decrease, making them more feasible for small and medium-sized businesses, as well as for developing countries looking to harness renewable energy sources. In turn, this could lead to broader adoption of steam engine systems, helping to democratize access to clean energy and promoting a more sustainable, equitable energy future.
The future of steam engines is bright, with advancements in sustainability, efficiency, and integration into modern energy systems driving new applications across various industries. As technology progresses, steam engines will continue to evolve to meet the demands of a cleaner, more sustainable world, offering a powerful, adaptable solution for both traditional and emerging energy needs. Through innovation, collaboration, and smart design, steam engines are positioned to play a key role in a future that values both technological progress and environmental stewardship.
EMS Power Machines
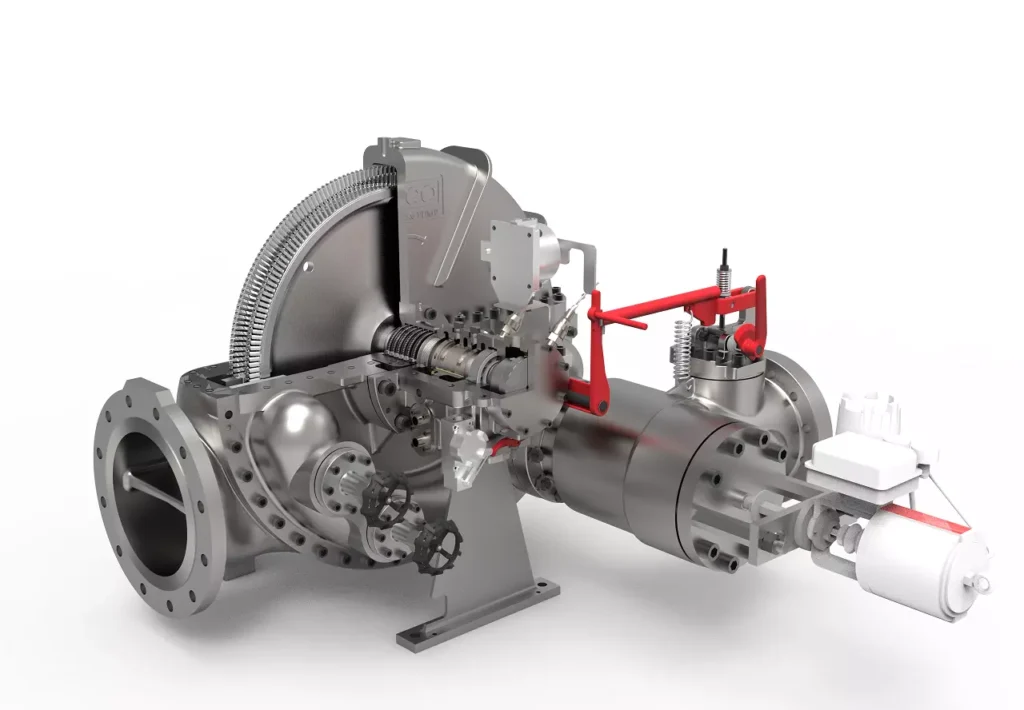
We design, manufacture and assembly Power Machines such as – diesel generators, electric motors, vibration motors, pumps, steam engines and steam turbines
EMS Power Machines is a global power engineering company, one of the five world leaders in the industry in terms of installed equipment. The companies included in the company have been operating in the energy market for more than 60 years.
EMS Power Machines manufactures steam turbines, gas turbines, hydroelectric turbines, generators, and other power equipment for thermal, nuclear, and hydroelectric power plants, as well as for various industries, transport, and marine energy.
EMS Power Machines is a major player in the global power industry, and its equipment is used in power plants all over the world. The company has a strong track record of innovation, and it is constantly developing new and improved technologies.
Here are some examples of Power Machines’ products and services:
- Steam turbines for thermal and nuclear power plants
- Gas turbines for combined cycle power plants and industrial applications
- Hydroelectric turbines for hydroelectric power plants
- Generators for all types of power plants
- Boilers for thermal power plants
- Condensers for thermal power plants
- Reheaters for thermal power plants
- Air preheaters for thermal power plants
- Feedwater pumps for thermal power plants
- Control systems for power plants
- Maintenance and repair services for power plants
EMS Power Machines is committed to providing its customers with high-quality products and services. The company has a strong reputation for reliability and innovation. Power Machines is a leading provider of power equipment and services, and it plays a vital role in the global power industry.
EMS Power Machines, which began in 1961 as a small factory of electric motors, has become a leading global supplier of electronic products for different segments. The search for excellence has resulted in the diversification of the business, adding to the electric motors products which provide from power generation to more efficient means of use.