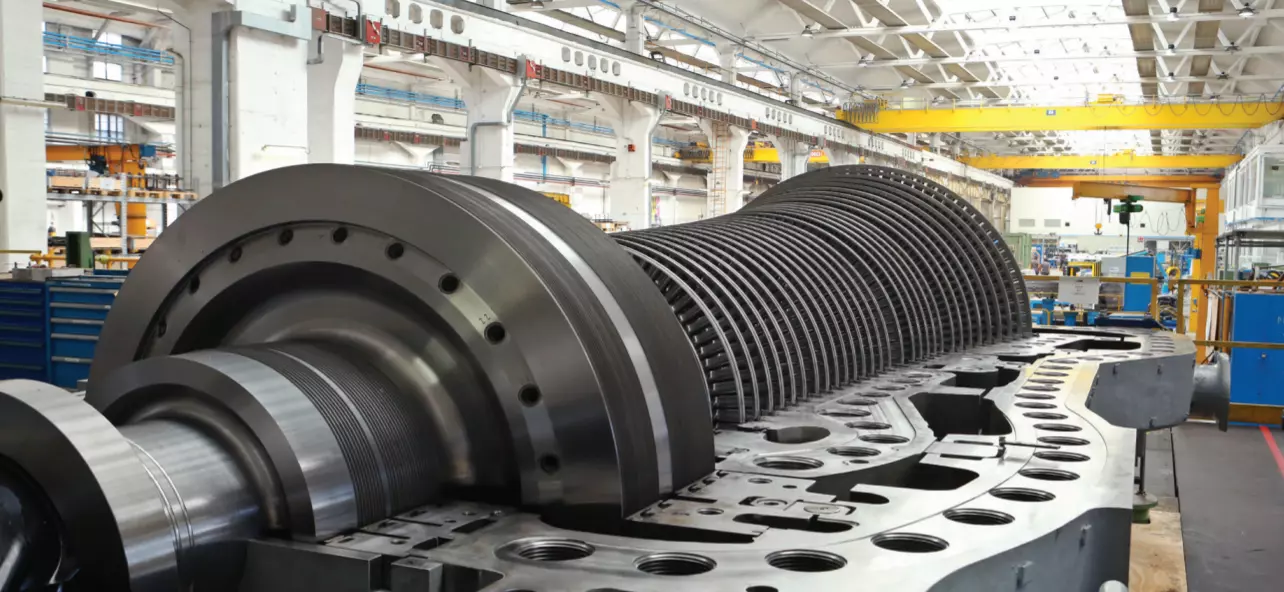
Back pressure steam turbine represents a fundamental element in power plants utilizing the Rankine cycle for electricity generation. This section provides an overview of the role of back pressure turbines in power systems, outlining their significance in converting thermal energy from steam into mechanical work.
- Thermodynamics of Back Pressure Steam Turbines:
A solid comprehension of thermodynamic principles underpinning the operation of back pressure steam turbines is crucial. This section delves into key concepts such as enthalpy, entropy, and the Rankine cycle, elucidating the interplay of pressure and temperature on turbine performance and efficiency.
- Design Considerations:
Designing efficient back pressure steam turbines requires a meticulous balance between aerodynamics, materials science, and mechanical engineering. This section explores the intricacies of blade profiles, steam path geometry, and rotor dynamics, emphasizing the critical role of these design elements in achieving optimal turbine efficiency.
- Operational Aspects:
Efficient operation of back pressure steam turbines demands careful consideration of various operational parameters. Topics covered include control mechanisms, governing systems, and the impact of variable steam conditions on turbine performance. Additionally, the role of extraction points and their influence on overall plant efficiency is discussed.
- Performance Optimization Strategies:
Optimizing the performance of back pressure steam turbines is essential for maximizing power plant efficiency. This section covers advanced strategies, including modifications to steam path geometry, the implementation of advanced control systems, and the integration of variable-speed drives. The impact of these strategies on overall efficiency and flexibility in responding to varying loads is examined.
- Advanced Technologies and Innovations:
The evolving landscape of power generation introduces new technologies and innovations in back pressure steam turbines. Concepts such as advanced materials, innovative blade designs, and the incorporation of digital control systems are explored. The potential impact of these advancements on turbine efficiency and reliability is discussed.
- Case Studies and Practical Applications:
Real-world case studies of successful back pressure steam turbine installations are presented, offering insights into challenges faced, solutions implemented, and lessons learned. These case studies serve as practical references for engineers and operators seeking to optimize the performance of existing installations or plan new projects.
- Future Trends and Challenges:
The text concludes with a discussion on emerging trends, challenges, and potential future developments in back pressure steam turbine technology. Topics such as the integration of advanced sensors for condition monitoring, the impact of Industry 4.0 on turbine operations, and the role of back pressure turbines in decentralized energy systems are explored.
In summary, this text provides a comprehensive exploration of back pressure steam turbines, offering a blend of theoretical foundations, practical insights, and a forward-looking perspective. Aimed at engineers, researchers, and students, this resource aims to deepen understanding of the complex dynamics involved in harnessing steam power through back pressure turbines for efficient electricity generation.
This comprehensive text delves into the intricacies of back pressure steam turbines, crucial components in power generation systems where the Rankine cycle is employed. Focusing on design principles, operational considerations, and strategies for optimizing performance, the following exposition serves as a valuable resource for engineers, researchers, and enthusiasts seeking an in-depth understanding of the nuanced dynamics associated with back pressure steam turbines.
- Introduction:
Back pressure steam turbines represent a fundamental element in power plants utilizing the Rankine cycle for electricity generation. This section provides an overview of the role of back pressure turbines in power systems, outlining their significance in converting thermal energy from steam into mechanical work.
- Thermodynamics of Back Pressure Steam Turbines:
A solid comprehension of thermodynamic principles underpinning the operation of back pressure steam turbines is crucial. This section delves into key concepts such as enthalpy, entropy, and the Rankine cycle, elucidating the interplay of pressure and temperature on turbine performance and efficiency.
- Design Considerations:
Designing efficient back pressure steam turbines requires a meticulous balance between aerodynamics, materials science, and mechanical engineering. This section explores the intricacies of blade profiles, steam path geometry, and rotor dynamics, emphasizing the critical role of these design elements in achieving optimal turbine efficiency.
- Operational Aspects:
Efficient operation of back pressure steam turbines demands careful consideration of various operational parameters. Topics covered include control mechanisms, governing systems, and the impact of variable steam conditions on turbine performance. Additionally, the role of extraction points and their influence on overall plant efficiency is discussed.
- Performance Optimization Strategies:
Optimizing the performance of back pressure steam turbines is essential for maximizing power plant efficiency. This section covers advanced strategies, including modifications to steam path geometry, the implementation of advanced control systems, and the integration of variable-speed drives. The impact of these strategies on overall efficiency and flexibility in responding to varying loads is examined.
- Advanced Technologies and Innovations:
The evolving landscape of power generation introduces new technologies and innovations in back pressure steam turbines. Concepts such as advanced materials, innovative blade designs, and the incorporation of digital control systems are explored. The potential impact of these advancements on turbine efficiency and reliability is discussed.
- Case Studies and Practical Applications:
Real-world case studies of successful back pressure steam turbine installations are presented, offering insights into challenges faced, solutions implemented, and lessons learned. These case studies serve as practical references for engineers and operators seeking to optimize the performance of existing installations or plan new projects.
- Future Trends and Challenges:
The text concludes with a discussion on emerging trends, challenges, and potential future developments in back pressure steam turbine technology. Topics such as the integration of advanced sensors for condition monitoring, the impact of Industry 4.0 on turbine operations, and the role of back pressure turbines in decentralized energy systems are explored.
In summary, this text provides a comprehensive exploration of back pressure steam turbines, offering a blend of theoretical foundations, practical insights, and a forward-looking perspective. Aimed at engineers, researchers, and students, this resource aims to deepen understanding of the complex dynamics involved in harnessing steam power through back pressure turbines for efficient electricity generation.
The Back-Pressure Steam Turbine: A Balancing Act of Power and Process
While its condensing cousin steals the spotlight in electricity generation, the back-pressure steam turbine (BPST) hums quietly in the background, playing a crucial role in powering industrial processes. Unlike the condensing turbine, whose primary objective is electricity production, the BPST dances to a different tune, prioritizing mechanical power extraction while cleverly utilizing the leftover thermal energy for industrial needs. Let’s peel back the layers of this fascinating machine and witness its intricate balance of power and process.
1. Steam’s Tailor-Made Journey: Adapting to Process Demands
Unlike the standardized high-pressure steam fed to condensing turbines, the BPST receives steam customized for specific industrial needs. The boiler, its fiery forge, tailors the steam’s pressure and temperature to suit the downstream process requirements. Whether it’s driving pumps in a petrochemical plant or powering compressors in a paper mill, the BPST receives steam tuned to the specific energy demands of the application.
2. Power Unveiled: A Waltz of Blades and Rotational Might
Just like its condensing counterpart, the BPST’s heart lies in the intricate ballet of steam and blades. Arranged in stages within the turbine casing, the blades pirouette with the incoming steam, extracting its kinetic energy through meticulously designed geometries. However, unlike the condensing turbine, the BPST’s blade design and stage configuration prioritize maximizing shaft power output while maintaining a specific exhaust pressure. This tailored approach ensures the extracted energy seamlessly integrates into the downstream process, driving equipment and powering industrial needs.
3. The Exhaust’s Encore: Thermal Energy’s Second Act
But the BPST’s brilliance goes beyond mere shaft power. Recognizing the remaining thermal potential in the exhaust steam, the turbine strategically maintains a higher-than-condensing pressure at the outlet. This residual heat becomes the star of the second act, providing valuable thermal energy for industrial applications. From preheating boiler feedwater to supplying process steam for heating or distillation, the BPST’s exhaust finds a new purpose, maximizing overall energy utilization.
4. Efficiency’s Double Play: Balancing Power and Process
The BPST’s true mastery lies in its delicate balancing act. It optimizes mechanical power extraction for the immediate process needs while simultaneously preserving precious thermal energy for further utilization. This intricate dance between immediate power and future thermal benefit distinguishes the BPST from its condensing counterpart, making it the unsung hero of industrial energy efficiency.
5. A Spectrum of Applications: Where the BPST Shines
The BPST’s versatility finds application across a vast spectrum of industries. From the behemoths of oil and gas processing to the intricate operations of paper and textile mills, the BPST seamlessly integrates into diverse production chains. Its ability to tailor power output and provide valuable thermal energy makes it a crucial cog in the machinery of modern industrial processes.
Beyond the Technical Spotlight: Challenges and Advancements
Despite its efficiency prowess, the BPST faces its own challenges. Optimizing blade design and stage configurations for specific processes requires careful engineering considerations. Additionally, ensuring stable operation while handling exhaust steam at varying pressures necessitates advanced control systems. However, continuous advancements in materials science, thermodynamics modeling, and control technology are constantly pushing the boundaries of BPST efficiency and operational flexibility.
The back-pressure steam turbine might not bask in the same limelight as its electricity-generating cousin, but its intricate dance of power and process plays a vital role in powering the wheels of modern industry. Its ability to seamlessly integrate into diverse applications, while maximizing energy utilization, makes it a true testament to engineering ingenuity and a champion of industrial efficiency. So, the next time you encounter a humming factory or a bustling refinery, remember the silent maestro pulling the strings – the back-pressure steam turbine, a master of balance in the grand symphony of industrial progress.
Back Pressure Steam Turbine Characteristics
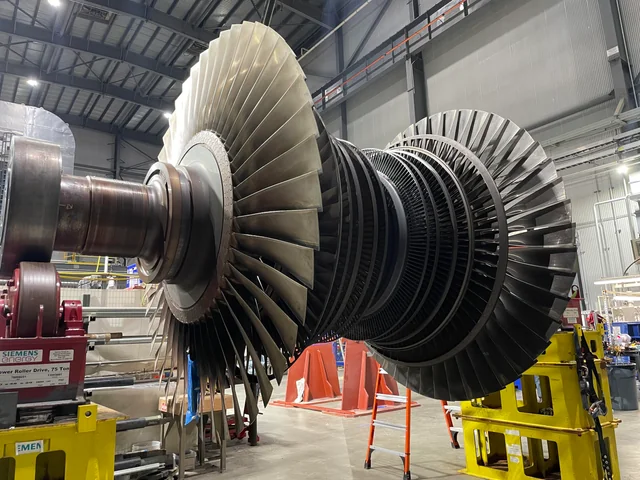
Governor Valve:
The governor valve is a crucial component in the control system of steam turbines, designed to regulate the flow of steam entering the turbine. Its primary function is to maintain a constant rotational speed of the turbine, ensuring a stable and predictable output of mechanical and electrical power.
In a back pressure steam turbine system, the governor valve responds to changes in load or demand for electricity. If there is an increase in demand, the governor valve opens to allow more steam into the turbine, facilitating higher power output. Conversely, if the demand decreases, the governor valve adjusts to reduce steam flow, maintaining a consistent turbine speed.
The governor valve operates based on a feedback loop that includes speed sensors, controllers, and actuators. As the turbine speed deviates from the setpoint, the governor valve adjusts to bring the speed back to the desired level. This dynamic control mechanism is essential for grid stability, ensuring that the turbine responds promptly to variations in electrical demand.
Advanced governor systems utilize digital controls and sophisticated algorithms to optimize turbine performance under varying conditions. The effectiveness of the governor valve directly impacts the overall efficiency and reliability of the back pressure steam turbine, making it a critical element in power generation systems.
Thermodynamics:
Thermodynamics is a branch of physics that deals with the principles governing the relationships between heat, work, temperature, and energy. In the context of back pressure steam turbines, a deep understanding of thermodynamics is essential for analyzing and optimizing the performance of these energy conversion systems.
The first law of thermodynamics, also known as the law of energy conservation, states that energy cannot be created or destroyed but can only change forms. In a back pressure steam turbine, this law is fundamental as it governs the conversion of thermal energy from high-pressure steam into mechanical work and subsequently into electrical power.
The second law of thermodynamics introduces the concept of entropy, which measures the degree of disorder or randomness in a system. In steam turbines, maintaining low entropy during the expansion of steam is crucial for maximizing the efficiency of the energy conversion process.
The isentropic process, where the entropy remains constant, is often used as an idealized model for steam expansion in turbines. However, real-world processes involve irreversibilities, and understanding these thermodynamic inefficiencies is crucial for practical turbine design and operation.
Thermodynamics also plays a role in the selection of working fluids, the design of heat exchangers, and the overall efficiency analysis of power cycles. Engineers use thermodynamic principles to optimize the performance of back pressure steam turbines, ensuring that they operate within specified parameters for maximum energy conversion efficiency and reliability.
Isentropic Efficiency:
Isentropic efficiency is a key parameter in the performance assessment of steam turbines, providing a measure of how efficiently a turbine converts the enthalpy of steam into mechanical work under idealized, reversible conditions.
Defined as the ratio of actual work output to the maximum work output possible in an isentropic (constant entropy) process, isentropic efficiency is expressed mathematically as the ratio of the change in enthalpy (h) to the isentropic change in enthalpy (h_s):
Isentropic Efficiency(isentropic)=ℎ1−ℎ2ℎ1−ℎ2Isentropic Efficiency(ηisentropic)=h1−h2sh1−h2
where:
- h1 is the enthalpy of the steam at the turbine inlet,
- h2 is the actual enthalpy of the steam at the turbine exit, and
- h2s is the enthalpy of the steam at the turbine exit under isentropic conditions.
In practical terms, isentropic efficiency provides insights into how well a steam turbine is performing compared to an idealized, frictionless, and reversible process. Actual processes involve inherent irreversibilities, such as friction, heat losses, and turbulence, which reduce the efficiency from the ideal isentropic case.
Engineers use isentropic efficiency as a critical parameter in the design and analysis of steam turbines, aiming to improve overall efficiency and energy conversion performance. Monitoring and optimizing isentropic efficiency help ensure that the turbine operates at its highest potential while considering real-world thermodynamic limitations.
Condenser:
A condenser is a vital component in steam power plants, including those utilizing back pressure steam turbines, designed to enhance the efficiency of the overall thermodynamic cycle by converting steam back into water.
After steam passes through the turbine blades, it enters the condenser, where it is exposed to a cooling medium, often water. The heat from the steam is transferred to the cooling medium, causing the steam to undergo a phase change from vapor to liquid. This condensed water is then pumped back into the steam cycle, completing the water-steam-water cycle.
The primary purpose of the condenser is to maintain a low-pressure environment at the turbine exhaust. This low pressure allows the steam to expand more fully in the turbine, extracting as much energy as possible. The efficiency of the condensation process is crucial for overall power plant efficiency.
Various types of condensers exist, such as surface condensers and direct-contact condensers, each with its advantages and disadvantages. Surface condensers use a series of tubes to facilitate heat exchange between steam and a cooling medium, while direct-contact condensers allow steam to come into direct contact with the cooling water.
The performance of the condenser significantly influences the efficiency of the steam cycle, making it a critical element in the design and operation of power plants using back pressure steam turbines.
Inlet Temperature:
In the context of back pressure steam turbines, the inlet temperature refers to the temperature of the steam entering the turbine. This parameter is a crucial factor influencing the efficiency and overall performance of the turbine.
The efficiency of a steam turbine is closely tied to the temperature difference between the steam at the inlet and the condenser pressure. Higher inlet temperatures generally lead to higher thermal efficiency, as the available temperature drop across the turbine is increased.
However, there are practical limits to the inlet temperature, primarily dictated by the materials used in turbine components. Higher temperatures can lead to increased stress and wear on turbine blades and other components. Advanced materials and cooling techniques are employed to push these temperature limits and improve overall efficiency.
The relationship between steam temperature and turbine efficiency is a key consideration in the design and operation of back pressure steam turbines. Engineers carefully balance the benefits of higher temperatures for increased efficiency with the challenges associated with material limitations and the potential for decreased turbine lifespan. Understanding and optimizing the inlet temperature contribute to achieving the desired performance and efficiency in power generation systems.
Blade Erosion:
Blade erosion is a significant concern in the operation of steam turbines, including back pressure steam turbines, and refers to the gradual wear and damage experienced by turbine blades over time. This erosion is primarily attributed to the impact of steam containing entrained solid particles, such as small water droplets or particulate matter.
As steam passes through the turbine stages, especially at high velocities, it can carry tiny solid particles. These particles impinge on the turbine blades, causing erosion and gradual material loss. Blade erosion is a complex phenomenon influenced by factors like steam quality, steam velocity, and the characteristics of the entrained particles.
The erosion process can result in changes to the blade’s surface geometry, leading to efficiency losses and potentially compromising the structural integrity of the turbine components. Engineers employ various strategies to mitigate blade erosion, including optimizing steam quality, employing advanced blade materials with enhanced erosion resistance, and implementing effective steam filtering systems.
Regular maintenance, inspections, and monitoring of erosion rates are essential to ensure the continued reliable and efficient operation of back pressure steam turbines. Understanding and addressing blade erosion contribute to extending the operational life and maintaining the performance of steam turbines in power generation and industrial applications.
Cogeneration Efficiency:
Cogeneration efficiency is a key metric in evaluating the performance of cogeneration or combined heat and power (CHP) systems, where both electrical and thermal energy are simultaneously produced from a single energy source, often involving back pressure steam turbines.
The efficiency of cogeneration is calculated by considering the total useful energy output (both electricity and thermal energy) compared to the energy input from the primary fuel source. The formula for cogeneration efficiency is:
Cogeneration Efficiency=Total Useful Energy OutputTotal Energy InputCogeneration Efficiency=Total Energy InputTotal Useful Energy Output
In the context of a back pressure steam turbine, this includes accounting for the electrical power generated and the thermal energy extracted from the steam for heating or industrial processes.
High cogeneration efficiency is desirable as it indicates effective utilization of the available energy, reducing waste and improving overall system sustainability. Cogeneration systems offer significant advantages in energy efficiency compared to separate production of electricity and thermal energy. Optimizing the cogeneration efficiency involves balancing the electrical and thermal loads, selecting appropriate equipment, and maximizing the use of both energy outputs to meet the demands of the end-users. This approach contributes to a more efficient and sustainable energy utilization strategy.
Steam Header:
A steam header is a vital component in steam power plants and industrial facilities utilizing back pressure steam turbines. It serves as a manifold that collects and distributes steam from multiple sources to various parts of the plant or industrial processes.
Steam produced in different sections of the plant, such as boilers or heat recovery steam generators, is directed to the steam header. The steam header acts as a distribution point, ensuring a steady and controlled supply of steam to different users or processes. It plays a critical role in balancing steam distribution and maintaining uniform pressure across the system.
The design of a steam header involves considerations of pressure, temperature, and flow rate, ensuring that steam is delivered efficiently and safely to the intended destinations. Valves and control systems may be integrated into the steam header to regulate steam flow and pressure, contributing to the overall stability and reliability of the steam supply.
Proper maintenance and monitoring of the steam header are essential to prevent issues such as steam leakage, uneven distribution, or pressure fluctuations. The effective operation of the steam header optimizes the performance of back pressure steam turbines and supports various industrial processes requiring a reliable source of steam.
Steam Quality:
Steam quality is a crucial parameter in the operation of steam turbines, including back pressure steam turbines. It refers to the dryness fraction or the percentage of vapor in a steam-water mixture. Steam quality is a key factor influencing the efficiency and reliability of the energy conversion process in steam turbines.
In an ideal scenario, steam would be perfectly dry, meaning it contains 100% vapor with no liquid water. However, in real-world conditions, steam often contains some water droplets or moisture. The presence of moisture can impact the performance of steam turbines, leading to issues such as erosion of turbine blades and reduced efficiency.
Steam quality is typically expressed as a percentage, with higher percentages indicating drier steam. Engineers carefully control and monitor steam quality in power plants by employing separators, moisture removal systems, and ensuring proper steam generation processes. Effective steam quality management helps minimize the potential for erosion and ensures that the energy in the steam is efficiently converted into mechanical work by the turbine.
Understanding and maintaining optimal steam quality are essential for the reliable and efficient operation of back pressure steam turbines, contributing to the longevity and performance of the turbine components.
Reheat Steam Turbine:
A reheat steam turbine is a specialized type of steam turbine commonly used in power plants to enhance the overall efficiency of the thermodynamic cycle. Unlike simple back pressure turbines, reheat turbines incorporate a reheat process, which involves reheating the steam before it undergoes additional expansion in the turbine.
In a reheat steam turbine system, high-pressure steam from the boiler is first expanded through the initial stages of the turbine, producing mechanical work. Instead of allowing the steam to continue its expansion through the remaining turbine stages, it is extracted and sent back to the boiler for reheating. The reheated steam is then directed into the subsequent stages of the turbine for further expansion and work production.
The inclusion of the reheat process allows the steam to expand over a larger temperature range, increasing the overall efficiency of the turbine. This is particularly beneficial in power plants where high thermal efficiency is a priority. Reheat turbines are commonly used in large-scale power generation facilities and are often associated with combined cycle power plants that integrate both gas and steam turbines.
The design and operation of reheat steam turbines require careful consideration of temperature and pressure conditions to optimize efficiency and power output while ensuring the reliability of turbine components.
Combined Cycle Power Plant:
A combined cycle power plant is a sophisticated energy generation facility that integrates both gas turbines and steam turbines to maximize overall efficiency in electricity production. This type of power plant is commonly employed to extract the most energy possible from fuel sources, making it an efficient and environmentally friendly option.
In a combined cycle power plant, the process begins with a gas turbine that burns fuel, typically natural gas. The gas turbine produces mechanical power by driving a generator, generating electricity. The hot exhaust gases from the gas turbine, which still contain significant thermal energy, are then directed to a heat recovery steam generator (HRSG).
The HRSG captures the residual heat from the gas turbine exhaust and uses it to produce high-pressure steam. This steam is then directed into a steam turbine, such as a back pressure turbine, where it undergoes additional expansion, generating additional mechanical power to drive a second generator.
The combination of the gas turbine and steam turbine in a single power plant creates a synergistic effect, significantly improving overall efficiency compared to separate power generation systems. Combined cycle power plants are known for their high efficiency, reduced environmental impact, and versatility in various applications, ranging from large-scale utility plants to industrial facilities.
Back Pressure Valve:
A back pressure valve is a crucial component in steam systems, particularly those utilizing back pressure steam turbines. Its primary function is to control and maintain the pressure of steam within the desired range at the turbine outlet, influencing both power generation and the extraction of thermal energy for other processes.
In a back pressure steam turbine system, steam is allowed to expand through the turbine, producing mechanical work and generating electricity. The back pressure valve, located at the turbine exhaust, regulates the pressure of the steam before it is released to the condenser or other downstream processes.
The operation of the back pressure valve is essential for controlling the overall system pressure, which directly impacts the efficiency and performance of the steam turbine. By adjusting the opening of the valve, operators can control the pressure at the turbine outlet and, consequently, the power output of the turbine. Properly configured back pressure valves contribute to stable turbine operation and optimal energy conversion.
Additionally, back pressure valves play a role in managing the extraction of low-pressure steam for heating or industrial applications. This dual functionality enhances the versatility of back pressure steam turbines in applications where both electricity generation and thermal energy utilization are essential. Engineers carefully design and maintain back pressure valves to ensure efficient and reliable operation in steam power systems.
Steam Turbine Rotor:
The steam turbine rotor is a central and critical component in the operation of steam turbines, including back pressure steam turbines. It is the rotating element that receives the mechanical energy from the expanding steam and transfers it to the generator for the production of electrical power.
In a back pressure steam turbine, high-pressure steam is admitted into the turbine, causing the rotor to spin. The rotor is typically a shaft with multiple blades or buckets mounted on it. As the steam expands through the turbine blades, the rotor experiences a torque that results in rotational motion.
The design of the steam turbine rotor is a complex engineering task, considering factors such as material strength, aerodynamics, and dynamic balancing. The rotational speed of the rotor is a critical parameter, and it must be carefully controlled to maintain stable and efficient turbine operation.
To achieve the highest efficiency and reliability, modern steam turbine rotors are often designed with advanced materials and technologies. Additionally, precise manufacturing and assembly processes, along with continuous monitoring, are employed to ensure the integrity and performance of the steam turbine rotor throughout its operational life. The efficiency and overall success of the back pressure steam turbine system heavily depend on the design and maintenance of this essential rotating component.
Extraction Steam:
Extraction steam in the context of steam turbines refers to the controlled withdrawal of steam at intermediate stages of the turbine for specific industrial processes or heating applications. This process enhances the versatility and efficiency of back pressure steam turbines, allowing them to serve multiple purposes beyond electricity generation.
In a back pressure steam turbine system, high-pressure steam is admitted into the turbine to produce mechanical work and generate electricity. However, before the steam completes its expansion through all the turbine stages, a portion of it can be extracted for various purposes. This extracted steam, known as extraction steam, is redirected to other parts of the industrial process or utilized for district heating, enhancing the overall energy efficiency of the system.
Extraction steam is commonly used in industries where both electricity and thermal energy are required. The extraction process allows for the simultaneous production of electricity and the utilization of steam for heating applications or industrial processes. Careful control of extraction steam is essential to balance the energy requirements of different processes and maintain optimal efficiency in the overall system. Engineers design and optimize extraction systems based on specific operational needs, contributing to the flexibility and efficiency of back pressure steam turbines in various applications.
Condensate Pump:
A condensate pump is a critical component in steam power plants, especially those employing back pressure steam turbines. Its primary function is to move the condensed water, known as condensate, from the condenser back into the steam cycle, completing the water-steam-water cycle and ensuring efficient reuse of water within the system.
After steam passes through the turbine and undergoes condensation in the condenser, the resulting condensate is collected at the bottom of the condenser. The condensate pump then draws this water and pumps it back to the boiler or heat exchanger, where it is heated to become steam once again.
Efficient condensate removal is vital for maintaining a continuous and reliable steam cycle. The condensate pump helps prevent water accumulation in the condenser, ensuring a low-pressure environment for the incoming steam in subsequent cycles. Additionally, proper condensate return enhances the overall thermal efficiency of the power plant by reducing the need for additional makeup water.
Condensate pumps come in various designs, including centrifugal pumps and positive displacement pumps, and their selection is based on factors such as system pressure, flow requirements, and the distance the condensate needs to be pumped. Regular maintenance and monitoring of condensate pumps are essential to ensure their continued reliability and optimal performance in steam power systems.
Steam Accumulator:
A steam accumulator is a storage device in steam power systems designed to store excess steam during periods of low demand and release it when demand increases. This serves as a means of smoothing out fluctuations in steam supply and demand, contributing to the stability and efficiency of back pressure steam turbines.
During times of low demand or when the steam production exceeds the immediate requirements, the excess steam is directed into the steam accumulator. The accumulator stores this surplus steam at a high pressure. When the demand for steam rises, the stored steam can be released to supplement the current steam production, ensuring a continuous and stable supply.
Steam accumulators are particularly beneficial in situations where there are rapid and significant variations in steam demand, such as in industrial processes with intermittent heating requirements. By acting as a buffer, the steam accumulator helps prevent abrupt pressure changes in the steam distribution system and allows the back pressure steam turbine to operate more efficiently.
The design and sizing of steam accumulators involve considerations of pressure, temperature, and storage capacity. Properly implemented steam accumulators contribute to energy efficiency, reduced stress on the steam generation system, and improved responsiveness to varying steam demands.
Turbine Efficiency Curve:
The turbine efficiency curve is a graphical representation of how the efficiency of a steam turbine varies across different operating conditions. It provides a valuable tool for engineers and operators to analyze and optimize the performance of back pressure steam turbines under varying loads and steam conditions.
The x-axis of the curve typically represents the turbine’s operating range, often in terms of steam flow rate or power output. The y-axis represents the efficiency of the turbine at each corresponding point on the curve. The curve illustrates how the turbine efficiency changes as operating conditions, such as steam pressure or temperature, vary.
A well-designed turbine efficiency curve allows operators to identify the optimal operating point for the given conditions, maximizing the turbine’s efficiency and overall performance. It also aids in understanding the impact of load changes on efficiency and helps in making informed decisions about turbine operation.
Factors influencing the shape of the efficiency curve include the design of the turbine, the quality of steam, and the control mechanisms in place. Regular monitoring and analysis of the turbine efficiency curve enable operators to identify potential issues, implement improvements, and ensure that the back pressure steam turbine operates within its optimal efficiency range for prolonged reliability and cost-effective energy generation.
Critical Speed:
Critical speed, in the context of steam turbines, refers to the rotational speed at which a turbine rotor or shaft experiences resonance and significant vibrations. It is a crucial parameter in the design and operation of back pressure steam turbines to prevent mechanical failures and ensure the long-term reliability of the system.
When a rotor rotates at or near its critical speed, the natural frequencies of the rotor coincide with the excitation frequencies caused by imbalances or external forces. This resonance can lead to excessive vibrations, which may result in fatigue and failure of turbine components, such as blades or shafts.
Engineers carefully analyze the critical speed of a steam turbine during the design phase. Factors such as the rotor’s mass distribution, stiffness, and damping characteristics influence the critical speed. Balancing techniques, including precision manufacturing and assembly, are employed to avoid resonant conditions during operation.
Regular monitoring and analysis of turbine vibrations are essential to detect any deviations from normal behavior that might indicate approaching critical speeds. Maintaining safe operating speeds and addressing any resonance issues contribute to the longevity and reliability of back pressure steam turbines in power generation applications.
Rotor Balancing:
Rotor balancing is a critical process in the design and maintenance of back pressure steam turbines, ensuring that the rotating components of the turbine operate smoothly and reliably. Balancing aims to minimize vibrations and reduce the potential for mechanical failures caused by uneven distribution of mass.
During operation, a rotating rotor can develop imbalances due to variations in the distribution of mass or changes in the operating conditions. These imbalances can lead to increased vibrations, which, if left unaddressed, may result in fatigue and premature wear of turbine components.
Rotor balancing involves the careful adjustment of the mass distribution to eliminate or minimize vibrations. This process may be carried out during the manufacturing of the turbine components or as part of routine maintenance. Balancing techniques include adding or removing mass strategically, using counterweights or trim balancing.
Modern rotor balancing often employs precision instruments and computerized systems to measure and analyze vibrations. Dynamic balancing, where adjustments are made while the rotor is in operation, allows for precise fine-tuning.
Effective rotor balancing is crucial for the overall performance and longevity of back pressure steam turbines. It contributes to smoother operation, reduced wear on components, and enhanced reliability, ensuring that the turbine operates within specified tolerances and delivers optimal efficiency in power generation.
Inlet Flow Path:
The inlet flow path in a back pressure steam turbine refers to the route steam takes as it enters the turbine from the steam source, such as a boiler or heat recovery system. This pathway is a critical element in the turbine’s design, influencing the efficiency and overall performance of the system.
The inlet flow path typically includes components like nozzles, diaphragms, and other control mechanisms that guide and control the flow of steam into the turbine. Each component plays a specific role in preparing the steam for expansion, ensuring uniform distribution, and optimizing the conditions for energy extraction.
Nozzles in the inlet flow path accelerate the steam, converting its pressure into velocity. This high-velocity steam is then directed onto the turbine blades, where it expands, producing mechanical work. Diaphragms help control the expansion process, allowing for multiple stages of the turbine to extract energy efficiently.
The design of the inlet flow path is crucial for achieving optimal energy conversion and minimizing losses. Engineers carefully consider factors such as steam pressure, temperature, and velocity in designing these components. Computational fluid dynamics and advanced modeling techniques aid in optimizing the geometry and performance of the inlet flow path.
Efficient inlet flow path design contributes to the overall effectiveness of back pressure steam turbines, ensuring that steam is utilized to its maximum potential for electricity generation and other industrial applications.
Main Key Features of a Back Pressure Steam Turbine
A back pressure steam turbine is a type of steam turbine used in power generation and industrial applications. Unlike condensing steam turbines, which exhaust steam at a lower pressure and temperature, back pressure turbines release steam at a higher pressure. This higher exhaust pressure enables the utilization of steam for various processes, such as heating or industrial applications, after it passes through the turbine.
In a back pressure turbine, steam is admitted at a high pressure, and it expands through the turbine blades, causing the rotor to turn and generate mechanical energy. The steam is then exhausted at an elevated pressure, allowing it to be used for heating purposes in industrial processes or district heating systems. This design is particularly suitable for applications where both electricity generation and thermal energy are required, making it a common choice in cogeneration or combined heat and power (CHP) systems.
The efficiency of a back pressure steam turbine is influenced by factors such as steam inlet pressure, turbine design, and the selection of operating parameters. Balancing the steam flow rate and optimizing the exhaust pressure are critical for achieving the desired performance and energy conversion efficiency in back pressure turbines.
Steam Turbine Efficiency:
Steam turbine efficiency is a crucial parameter that measures how effectively a steam turbine converts the thermal energy of steam into mechanical work. It is expressed as the ratio of the actual mechanical power output to the energy content of the steam entering the turbine. Higher efficiency indicates better utilization of the steam’s energy and, consequently, improved performance in power generation or industrial processes.
Efficiency in steam turbines is influenced by various factors, including turbine design, operating conditions, steam quality, and maintenance practices. Modern turbines are designed to achieve high efficiency levels, typically ranging from 40% to 50%, depending on the specific application and technology used.
Efficiency improvements can be achieved through advancements in turbine design, materials, and control systems. Proper maintenance, regular inspections, and optimization of operating parameters also contribute to maintaining or enhancing steam turbine efficiency over its operational life. Monitoring and improving efficiency are essential aspects of sustainable and economical energy generation, making it a key consideration in the design and operation of steam turbine systems.
Turbine Exhaust Pressure:
Turbine exhaust pressure refers to the pressure at which steam exits the steam turbine after performing mechanical work. In the context of back pressure steam turbines, this parameter is of particular importance because it determines the energy available for subsequent processes or applications.
In a back pressure turbine, the exhaust pressure is intentionally maintained at a higher level compared to condensing turbines. This higher exhaust pressure allows the steam to be used for various industrial processes or heating applications after leaving the turbine. The selection of the exhaust pressure is a critical design consideration as it directly influences the potential for extracting additional useful work from the steam.
Optimizing the turbine exhaust pressure involves a trade-off between maximizing electricity generation and utilizing the steam for other applications. Engineers carefully select the exhaust pressure based on the specific requirements of the overall system, aiming to achieve a balance that maximizes the overall efficiency and economic viability of the power generation or cogeneration plant. Control systems, such as governor mechanisms, play a crucial role in maintaining the desired turbine exhaust pressure under varying operating conditions.
Power Generation:
Power generation refers to the process of converting various forms of energy into electrical power. Steam turbines, including back pressure steam turbines, play a significant role in power generation by converting the thermal energy of steam into mechanical energy, which is then used to generate electricity.
In the context of back pressure steam turbines, power generation begins with the introduction of high-pressure steam into the turbine. The steam expands through the turbine blades, causing the rotor to turn and generate mechanical work. This rotational energy is then used to drive an electrical generator, converting the mechanical energy into electrical power.
The efficiency and performance of the back pressure steam turbine directly impact the amount of electricity generated. Engineers and operators carefully design and control the system to optimize power output while considering factors such as steam conditions, turbine design, and overall plant efficiency.
Power generation using back pressure steam turbines is prevalent in various industries and power plants, especially in applications where simultaneous electricity generation and thermal energy utilization are required, making it a versatile solution in combined heat and power (CHP) or cogeneration systems.
Energy Conversion:
Energy conversion refers to the process of transforming one form of energy into another. In the case of back pressure steam turbines, the primary focus is on converting the thermal energy stored in high-pressure steam into mechanical work and, ultimately, electrical energy.
The energy conversion process in a back pressure steam turbine involves several stages. First, high-pressure steam is introduced into the turbine, where it expands through the turbine blades. This expansion causes the turbine rotor to rotate, converting the thermal energy of steam into mechanical energy.
The mechanical energy generated by the turbine is then utilized for various purposes, with the most common application being the rotation of an electrical generator. The generator, coupled with the turbine, converts the mechanical energy into electrical power. This process demonstrates the fundamental principle of energy conversion – changing the form of energy from steam’s high-pressure and high-temperature state to electricity.
Efficiency in energy conversion is a key consideration in the design and operation of back pressure steam turbines, ensuring that a significant portion of the thermal energy is effectively transformed into useful electrical power for industrial, commercial, or residential use.
Condensing Steam Turbine:
A condensing steam turbine is a type of steam turbine used in power generation where steam is allowed to expand and do mechanical work, and then it is condensed back into water. Unlike back pressure steam turbines, condensing turbines operate with lower exhaust pressures, often below atmospheric pressure.
In a condensing steam turbine, high-pressure steam is introduced into the turbine, where it expands through the blades, generating mechanical work. After passing through the turbine, the low-pressure steam is condensed back into water using a condenser. The condensation of steam allows the turbine to operate at lower pressures, improving the efficiency of the energy conversion process.
Condensing turbines are commonly used in power plants where the objective is to maximize electricity generation and overall efficiency. The lower exhaust pressure results in a larger pressure drop across the turbine, extracting more work from the steam. This design is suitable for large-scale power generation facilities that focus on optimizing electricity output without the need for simultaneous thermal energy utilization.
Steam Inlet Pressure:
Steam inlet pressure is a critical parameter in the operation of steam turbines, including back pressure steam turbines. It refers to the pressure at which high-temperature and high-pressure steam enters the turbine for the purpose of energy conversion.
In the context of back pressure turbines, maintaining an optimal steam inlet pressure is essential for achieving efficient and reliable performance. The inlet pressure affects the energy available for conversion and, consequently, the power output of the turbine. Engineers carefully design systems to ensure that steam entering the turbine is at the appropriate pressure to maximize the efficiency of the energy conversion process.
The selection of steam inlet pressure is influenced by factors such as the desired power output, turbine design, and the overall configuration of the power generation or industrial process. Controlling and regulating steam inlet pressure is typically managed by control systems and valves to adapt to varying operating conditions and demands, ensuring the turbine operates within specified parameters for optimal performance.
Turbine Blades:
Turbine blades are crucial components in steam turbines responsible for extracting mechanical energy from the expanding steam. In back pressure steam turbines, these blades play a key role in the conversion of thermal energy into rotational motion.
The design and material composition of turbine blades are critical factors influencing the efficiency and performance of the steam turbine. Blades are shaped to efficiently guide the steam flow, optimizing the extraction of energy as steam expands through the turbine. Materials with high strength and resistance to corrosion and erosion are chosen to withstand the demanding conditions of high-speed steam flows.
There are various types of turbine blades, including impulse and reaction blades, each designed to handle specific pressure and velocity profiles of steam. The arrangement and geometry of these blades contribute to the overall efficiency of the turbine in converting steam energy into mechanical work. Advances in blade technology, including aerodynamics and materials science, continue to improve the overall performance and longevity of steam turbines in power generation and industrial applications.
Thermal Power Plant:
A thermal power plant is a facility that generates electricity by converting heat energy into electrical power. Back pressure steam turbines often play a central role in the operation of thermal power plants, where the primary source of heat is typically the combustion of fossil fuels like coal, natural gas, or biomass.
In a thermal power plant, fuel is burned to produce high-temperature and high-pressure steam. This steam is then directed into a steam turbine, such as a back pressure turbine. As the steam expands through the turbine blades, it causes the rotor to turn, generating mechanical energy. The mechanical energy is subsequently used to drive an electrical generator, converting the thermal energy into electricity.
Thermal power plants are essential components of the global energy infrastructure, providing a reliable and large-scale source of electricity. The integration of back pressure steam turbines in such plants allows for the simultaneous production of electrical power and the utilization of steam for industrial processes or district heating, contributing to the overall efficiency and sustainability of the power generation system.
Steam Flow Rate:
Steam flow rate is a critical parameter in the operation of steam turbines, representing the quantity of steam that passes through the turbine per unit of time. In the context of back pressure steam turbines, the steam flow rate is a key factor influencing the power output and overall efficiency of the turbine.
The control and optimization of steam flow rate involve considerations of system design, turbine capacity, and the specific requirements of the power generation or industrial process. Maintaining a balance between steam flow rate and turbine capacity is essential for achieving optimal performance. Too high or too low a steam flow rate can impact the efficiency and reliability of the turbine.
Engineers and operators carefully design and control steam flow rates based on factors such as the desired power output, turbine design specifications, and the need to meet varying operational conditions. Accurate measurement and control of steam flow are essential for ensuring the stability and efficiency of back pressure steam turbines in diverse applications.
Power Output:
Power output in the context of back pressure steam turbines refers to the amount of mechanical or electrical power generated by the turbine as a result of converting the thermal energy of steam. It is a crucial performance metric that directly impacts the overall efficiency and effectiveness of the power generation or industrial process.
The power output is influenced by factors such as steam conditions (pressure and temperature), turbine design, steam flow rate, and the rotational speed of the turbine. Engineers aim to optimize these factors to achieve the desired level of power output while maintaining system reliability and efficiency.
Efficient power output is a key consideration in the design and operation of back pressure steam turbines, especially in applications where simultaneous electricity generation and thermal energy utilization are essential. It involves a careful balance between maximizing the conversion of steam energy into useful work and meeting the specific requirements of the intended use, whether it be electricity generation, industrial processes, or combined heat and power applications.
Steam Velocity:
Steam velocity is the speed at which steam travels through the steam turbine blades during the expansion process. In back pressure steam turbines, managing and optimizing steam velocity is crucial for efficient energy conversion and turbine performance.
The velocity of steam is influenced by factors such as steam pressure, temperature, and the design of the turbine blades. Engineers carefully design the blades to control steam flow, ensuring that the velocity is within optimal ranges for efficient energy extraction. Proper control of steam velocity helps prevent issues such as erosion or excessive wear on turbine components.
Maintaining an appropriate steam velocity is essential for maximizing the energy transfer from steam to the turbine blades. This ensures that a significant portion of the thermal energy is effectively converted into mechanical work, contributing to the overall efficiency of the back pressure steam turbine in power generation or industrial applications. Control systems are employed to manage steam velocity under different operating conditions, ensuring stable and reliable turbine performance.
Steam Expansion:
Steam expansion is a fundamental process in steam turbines, including back pressure steam turbines, where high-pressure steam undergoes controlled expansion to produce mechanical work. This expansion process is a key aspect of converting the thermal energy stored in steam into useful energy, typically in the form of rotation that drives a generator for electricity generation.
In a back pressure steam turbine, steam expansion occurs as the high-pressure steam is admitted into the turbine. As steam flows through the turbine blades, it expands, and its pressure and temperature decrease. This expansion leads to the rotation of the turbine rotor, generating mechanical energy.
The efficiency and performance of a back pressure steam turbine depend on the careful design and control of the steam expansion process. Engineers optimize factors such as blade design, steam inlet conditions, and turbine geometry to ensure efficient energy extraction during steam expansion. The goal is to harness as much energy as possible from the expanding steam while maintaining stable and reliable turbine operation.
Steam Pressure Control:
Steam pressure control is a critical aspect of operating back pressure steam turbines efficiently. It involves managing and regulating the pressure of steam entering the turbine to ensure optimal performance and safety.
Control systems are employed to maintain a consistent and appropriate steam pressure based on the operational requirements of the system. These systems often include valves and sensors that monitor and adjust steam flow to achieve the desired pressure levels. Proper control of steam pressure is essential for preventing issues such as turbine instability, excessive wear on components, or inefficient energy conversion.
In back pressure turbines, precise steam pressure control is particularly important because it directly affects the turbine’s power output and overall efficiency. Engineers design control mechanisms to respond to variations in demand and operating conditions, ensuring the turbine operates within specified pressure ranges for optimal performance in power generation or industrial applications.
Generator Drive:
A generator drive refers to the mechanism by which the mechanical energy produced by a steam turbine, such as a back pressure steam turbine, is used to drive an electrical generator. The generator converts this mechanical energy into electrical power.
In the context of back pressure steam turbines, the rotating shaft connected to the turbine rotor is coupled to the generator. As the turbine blades extract energy from expanding steam, the rotor turns, causing the generator to rotate. This rotation induces the generation of electrical power through electromagnetic processes within the generator.
Efficient generator drive systems are crucial for maximizing the conversion of mechanical energy into electrical power. The design and synchronization of the turbine and generator, along with control systems, play a significant role in achieving stable and reliable power generation. The output from the generator can be further controlled and managed to meet the demands of the electrical grid or specific industrial applications.
Turbine Governor:
A turbine governor is a control system used to regulate the speed and output of a steam turbine, including back pressure steam turbines. Its primary function is to maintain a consistent and stable rotational speed of the turbine, which, in turn, ensures the desired electrical power output.
The governor achieves this by adjusting the flow of steam entering the turbine based on changes in load or demand. If there is an increase in electrical demand, the governor opens the steam control valves to allow more steam into the turbine, increasing the power output. Conversely, if the demand decreases, the governor reduces steam flow to maintain a steady speed and output.
Turbine governors are crucial for grid stability and the reliable operation of power plants. They help prevent fluctuations in electrical frequency, ensuring a consistent and synchronized supply of electricity to the grid. Modern turbine governors use advanced control algorithms and feedback systems to respond quickly and accurately to changes in load, contributing to the overall stability and efficiency of the power generation system.
Turbine Performance:
Turbine performance in the context of back pressure steam turbines encompasses various factors that influence the efficiency, reliability, and overall effectiveness of the turbine in converting steam energy into mechanical work and electricity.
Key aspects of turbine performance include steam inlet conditions, steam flow rate, expansion efficiency, and the control of turbine parameters such as speed and pressure. Engineers focus on designing turbines to operate within optimal ranges for these factors, balancing the need for electricity generation with other potential uses of the steam, such as industrial processes or district heating.
Monitoring and assessing turbine performance involve the use of instrumentation and control systems that provide real-time data on operating conditions. Regular maintenance and adjustments based on performance data are essential to ensure the turbine operates efficiently and meets its design specifications over its operational life. Optimizing turbine performance contributes to the overall success of power generation systems, especially in applications where both electricity and thermal energy are valuable outputs.
Cogeneration:
Cogeneration, also known as combined heat and power (CHP), is a highly efficient energy generation strategy that involves the simultaneous production of electricity and useful thermal energy from a single energy source. Back pressure steam turbines are commonly employed in cogeneration systems to achieve this dual output.
In a cogeneration plant, high-pressure steam is directed into a back pressure steam turbine, generating mechanical work to drive an electrical generator. The electricity produced is then utilized for various purposes. Importantly, the steam, after passing through the turbine, retains a significant amount of thermal energy. This low-pressure steam is then used for heating or industrial processes, maximizing the overall efficiency of the energy conversion.
Cogeneration is advantageous in applications where there is a demand for both electricity and thermal energy, such as in industrial facilities, district heating systems, or commercial buildings. The integration of back pressure steam turbines in cogeneration enhances energy sustainability by efficiently utilizing the available energy and minimizing waste.
Steam Cycle:
The steam cycle refers to the continuous and cyclical process through which steam is generated, expanded in a steam turbine, and then condensed back into water to be reused. This cycle is fundamental to the operation of steam turbines, including back pressure steam turbines, in power generation and industrial applications.
The steam cycle typically begins with the combustion of a fuel source, such as coal, natural gas, or biomass, to produce high-temperature and high-pressure steam. This steam is then directed into the steam turbine, where it expands, producing mechanical work that is used to drive an electrical generator. After passing through the turbine, the low-pressure steam is condensed back into water in a condenser, and the cycle repeats.
Efficient operation of the steam cycle is essential for optimizing the performance of back pressure steam turbines. Engineers carefully design and control the various stages of the cycle to ensure maximum energy conversion and minimize losses. The steam cycle is a cornerstone of steam power generation, providing a reliable and widely used method for converting thermal energy into electricity.
Renewable Energy:
While not directly tied to the back pressure steam turbine itself, the concept of renewable energy is significant in the broader context of sustainable power generation. Renewable energy sources, such as wind, solar, hydro, and biomass, are gaining prominence in the quest for cleaner and more environmentally friendly electricity production.
Back pressure steam turbines can be part of renewable energy systems, particularly when biomass is used as a fuel source. Biomass, derived from organic materials, can be burned to produce steam that powers a back pressure steam turbine for electricity generation. The utilization of renewable resources in conjunction with steam turbines contributes to reducing greenhouse gas emissions and dependence on fossil fuels.
In the ongoing transition toward a more sustainable energy landscape, the integration of renewable energy sources with technologies like back pressure steam turbines showcases a commitment to environmentally responsible power generation. This approach aligns with global efforts to address climate change and promote a cleaner and more sustainable energy future.
EMS Power Machines
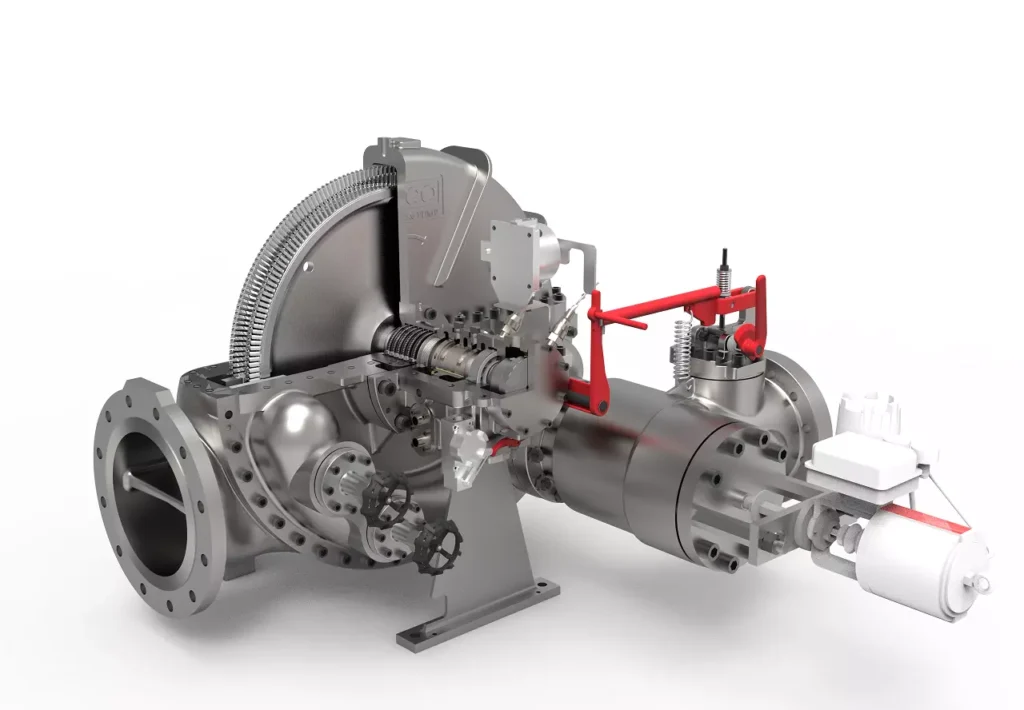
We design, manufacture and assembly Power Machines such as – diesel generators, electric motors, vibration motors, pumps, steam engines and steam turbines
EMS Power Machines is a global power engineering company, one of the five world leaders in the industry in terms of installed equipment. The companies included in the company have been operating in the energy market for more than 60 years.
EMS Power Machines manufactures steam turbines, gas turbines, hydroelectric turbines, generators, and other power equipment for thermal, nuclear, and hydroelectric power plants, as well as for various industries, transport, and marine energy.
EMS Power Machines is a major player in the global power industry, and its equipment is used in power plants all over the world. The company has a strong track record of innovation, and it is constantly developing new and improved technologies.
Here are some examples of Power Machines’ products and services:
- Steam turbines for thermal and nuclear power plants
- Gas turbines for combined cycle power plants and industrial applications
- Hydroelectric turbines for hydroelectric power plants
- Generators for all types of power plants
- Boilers for thermal power plants
- Condensers for thermal power plants
- Reheaters for thermal power plants
- Air preheaters for thermal power plants
- Feedwater pumps for thermal power plants
- Control systems for power plants
- Maintenance and repair services for power plants
EMS Power Machines is committed to providing its customers with high-quality products and services. The company has a strong reputation for reliability and innovation. Power Machines is a leading provider of power equipment and services, and it plays a vital role in the global power industry.
EMS Power Machines, which began in 1961 as a small factory of electric motors, has become a leading global supplier of electronic products for different segments. The search for excellence has resulted in the diversification of the business, adding to the electric motors products which provide from power generation to more efficient means of use.