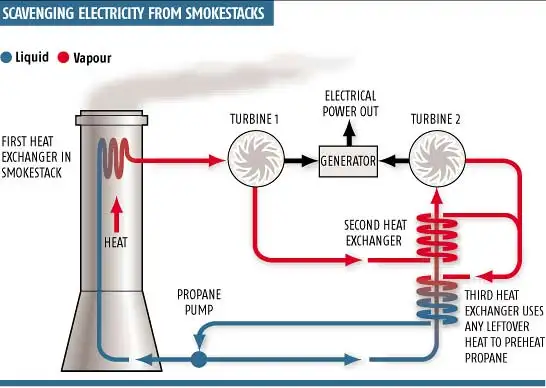
Small Scale Power Plant for Gas Production Facilities: Power plants utilizing steam turbines play a crucial role in global electricity generation. These plants operate on the principle of thermodynamic cycles, primarily the Rankine cycle, where water is heated to produce steam that drives a turbine connected to a generator. The process begins with a heat source, which can vary depending on the type of power plant. The most common sources include fossil fuels such as coal, natural gas, and oil, as well as nuclear energy, biomass, geothermal heat, and solar thermal energy. Regardless of the heat source, the goal is to convert thermal energy into mechanical energy and ultimately into electrical energy.
In a typical steam power plant, water is first fed into a boiler or steam generator, where it is heated under high pressure to produce superheated steam. This steam is then directed through a series of high-pressure turbines, where it expands and causes the turbine blades to rotate. The rotational energy is transferred to a generator via a shaft, converting mechanical energy into electrical power. After passing through the high-pressure turbine, the steam moves into intermediate- and low-pressure turbines, further expanding and transferring additional energy before being exhausted into a condenser.
The condenser plays a vital role in maintaining efficiency by cooling and condensing the steam back into liquid form. This condensed water, known as condensate, is then pumped back into the boiler through feedwater heaters that recover residual heat from extracted steam. This regenerative heating process improves overall efficiency by reducing the amount of fuel needed to reheat the water to its boiling point. The cycle continues, maintaining a continuous and efficient process of electricity generation.
Different types of steam power plants exist based on fuel source and operational characteristics. Fossil fuel power plants, including coal-fired and gas-fired plants, rely on burning hydrocarbons to generate heat. Coal-fired plants, though historically dominant, are facing increasing scrutiny due to environmental concerns related to carbon emissions and air pollution. Advanced technologies, such as supercritical and ultra-supercritical steam cycles, have been developed to enhance efficiency and reduce emissions. Natural gas power plants, particularly those using combined cycle technology, integrate steam turbines with gas turbines to maximize energy extraction, significantly increasing efficiency.
Nuclear power plants operate similarly but use nuclear fission to generate heat. In a pressurized water reactor (PWR), the reactor heats water in a primary loop, which then transfers heat to a secondary loop that generates steam to drive the turbine. Boiling water reactors (BWRs) produce steam directly within the reactor vessel. Nuclear plants provide a stable and continuous power supply, making them a key source of baseload electricity. However, they require strict safety measures and long-term solutions for radioactive waste disposal.
Renewable energy sources such as biomass, geothermal, and solar thermal also employ steam turbines. Biomass plants burn organic materials like wood, agricultural waste, or municipal solid waste to generate steam. Geothermal power plants utilize heat from beneath the Earth’s surface, extracting steam or hot water from underground reservoirs. Flash steam, dry steam, and binary cycle geothermal plants use different methods to harness this heat efficiently. Solar thermal power plants, such as concentrated solar power (CSP) systems, use mirrors to focus sunlight onto a receiver that heats a working fluid, producing steam to drive a turbine.
Efficiency improvements in steam power plants focus on advanced turbine designs, higher steam temperatures and pressures, and integrated heat recovery systems. Technologies such as cogeneration, also known as combined heat and power (CHP), increase efficiency by utilizing waste heat for industrial processes or district heating. Additionally, carbon capture and storage (CCS) is being explored to mitigate emissions from fossil fuel-based plants.
Steam turbine power plants continue to be a backbone of global electricity production due to their reliability and scalability. However, the transition towards cleaner and more sustainable energy sources is shaping the future of steam power technology. Research and development efforts are aimed at improving efficiency, reducing emissions, and integrating renewable energy with conventional steam turbine systems. The continued evolution of steam power plants will play a crucial role in balancing energy demand, environmental responsibility, and economic viability.
Small Scale Power Plant
Further advancements in steam power plant technology have been driven by the need for higher efficiency, reduced environmental impact, and increased reliability. One of the most significant developments has been the transition from subcritical steam cycles to supercritical and ultra-supercritical cycles. In subcritical plants, steam is generated at pressures below the critical point of water, meaning a distinct phase change from water to steam occurs in the boiler. However, supercritical plants operate at pressures above the critical point, eliminating the phase transition and allowing for more efficient thermal energy conversion. Ultra-supercritical plants push these limits further, achieving even higher steam temperatures and pressures, which significantly improve efficiency and reduce fuel consumption.
In addition to improvements in steam parameters, turbine technology itself has evolved. Modern turbines are designed with advanced aerodynamics, optimized blade profiles, and high-strength materials capable of withstanding extreme temperatures and pressures. Many large-scale power plants use multi-stage turbines, where high-pressure, intermediate-pressure, and low-pressure stages work together to extract the maximum amount of energy from the steam. Efficiency enhancements also come from reducing steam leakage, improving blade cooling techniques, and using coatings that prevent corrosion and wear.
Heat recovery is another crucial aspect of efficiency improvement in steam power plants. The use of regenerative feedwater heating allows for better utilization of extracted steam, reducing the amount of additional heat energy required in the boiler. Condenser efficiency is also vital, as the faster and more effectively steam is condensed back into water, the better the vacuum conditions in the turbine, which enhances power output. In combined cycle power plants, waste heat from a gas turbine is used to generate additional steam for a steam turbine, dramatically improving overall efficiency.
The environmental impact of steam power plants is a major concern, particularly for fossil fuel-based systems. Carbon dioxide (CO₂) emissions from coal and natural gas plants contribute to climate change, leading to increased focus on carbon capture and storage (CCS) technologies. These systems aim to capture CO₂ before it is released into the atmosphere and store it underground or utilize it in industrial applications. Other emission control technologies include flue gas desulfurization (FGD) for removing sulfur dioxide (SO₂), selective catalytic reduction (SCR) for nitrogen oxides (NOx), and particulate matter filters to reduce air pollution.
Water usage is another important factor in steam power plants. Traditional once-through cooling systems withdraw large amounts of water from natural sources, raising concerns about thermal pollution and water scarcity. As a result, many plants now use closed-loop cooling systems with cooling towers or air-cooled condensers to minimize water consumption. In regions with limited water resources, dry cooling technology is being adopted, though it can result in slightly lower plant efficiency due to higher condenser temperatures.
The role of digitalization and automation in modern steam power plants is growing, with advanced monitoring and control systems enhancing performance and reliability. Predictive maintenance using artificial intelligence (AI) and machine learning algorithms helps detect potential failures before they occur, reducing downtime and maintenance costs. Sensors and data analytics provide real-time insights into turbine performance, boiler efficiency, and emissions control, allowing for continuous optimization.
In addition to large centralized power plants, smaller-scale steam turbine systems are being used for industrial applications and distributed energy generation. Combined heat and power (CHP) plants, often found in industrial facilities, universities, and district heating systems, provide both electricity and useful heat, significantly increasing overall energy utilization. Some biomass and waste-to-energy plants use steam turbines to generate power while simultaneously addressing waste disposal and reducing landfill use.
The integration of renewable energy with steam turbine technology is another emerging trend. Hybrid systems that combine solar thermal energy with traditional steam power plants can improve efficiency and reduce fuel consumption. Some geothermal power plants utilize steam turbines to harness Earth’s natural heat, providing a renewable and stable source of electricity. In the long term, advancements in hydrogen combustion and zero-carbon fuels could allow steam power plants to operate sustainably while maintaining their reliability and large-scale energy production capabilities.
As global energy demand continues to grow, steam power plants will remain a key component of the energy mix. While renewable energy sources such as wind and solar are expanding rapidly, steam turbine plants provide a crucial source of baseload and dispatchable power. Future developments will likely focus on improving efficiency, reducing emissions, and integrating cleaner energy sources to ensure that steam power generation remains both viable and sustainable in a rapidly changing energy landscape.
Further improvements in steam turbine technology are focusing on efficiency gains through material advancements, operational optimizations, and alternative fuel sources. One of the key challenges in steam power plants is the thermal efficiency limitation imposed by the Carnot cycle. Since efficiency is directly related to the temperature difference between the heat source and the heat sink, researchers are exploring ways to increase maximum operating temperatures while maintaining the structural integrity of turbine components.
Advanced materials, such as nickel-based superalloys and ceramic matrix composites, are being developed to withstand extreme temperatures and pressures, allowing turbines to operate at higher efficiencies. These materials offer better resistance to thermal stress, oxidation, and corrosion, reducing maintenance needs and extending component lifespan. Additionally, advancements in coatings, such as thermal barrier coatings (TBCs), are helping to protect turbine blades from high-temperature degradation, further improving operational longevity.
Operational optimizations are also playing a crucial role in enhancing steam turbine performance. Digital twin technology, where a virtual model of a steam power plant is continuously updated with real-time data, is being used to optimize performance and predict potential failures. This approach allows operators to test different scenarios in a simulated environment, leading to better decision-making and efficiency improvements. Artificial intelligence (AI) and machine learning are being integrated into plant management systems to analyze vast amounts of operational data and identify opportunities for optimization.
One promising area of research is the development of closed-loop supercritical carbon dioxide (sCO₂) cycles as an alternative to traditional steam-based Rankine cycles. sCO₂ operates at higher efficiencies due to its superior thermodynamic properties, allowing for compact turbine designs and reduced heat losses. These systems have the potential to significantly improve efficiency while reducing water consumption, making them particularly attractive for arid regions where water availability is limited.
Another critical area of innovation is the transition toward alternative fuels in steam power plants. While coal remains a dominant fuel source in some regions, there is a growing push toward cleaner alternatives such as biomass, hydrogen, and ammonia. Biomass co-firing, where biomass is blended with coal, reduces net CO₂ emissions while utilizing existing infrastructure. Hydrogen, when produced using renewable energy sources, presents a zero-carbon alternative for steam generation, though challenges remain in terms of production costs and storage. Ammonia, which can be used as a hydrogen carrier, is also being explored as a potential fuel for steam power plants due to its high energy density and ease of transportation.
Nuclear steam power is another area of ongoing innovation. Small modular reactors (SMRs) are being developed as a more flexible and scalable alternative to traditional large nuclear power plants. These reactors use steam turbines for power generation but feature enhanced safety mechanisms, simplified designs, and reduced construction times. SMRs are expected to play a significant role in the future energy landscape, providing reliable, low-carbon electricity with improved adaptability to grid demands.
Hybrid power systems that integrate steam turbines with renewable energy sources are also being explored to enhance grid stability and reduce reliance on fossil fuels. Solar thermal plants with molten salt storage can generate steam for turbines even when sunlight is unavailable, providing a continuous power supply. Geothermal power plants utilizing steam turbines can serve as a baseload energy source, complementing intermittent renewables like wind and solar.
Waste heat recovery systems are further improving the efficiency of industrial processes and power generation. By capturing excess heat from industrial operations or power plants and using it to generate steam, these systems maximize energy utilization and reduce environmental impact. Combined cycle plants, which pair gas turbines with steam turbines, are an example of how waste heat can be effectively repurposed to increase overall efficiency.
As the global energy transition continues, steam turbine power plants will need to adapt to new regulatory requirements, carbon reduction targets, and evolving market demands. The future of steam power lies in greater efficiency, integration with cleaner energy sources, and the adoption of advanced digital technologies. With ongoing research and development, steam turbines will continue to be a vital part of the world’s energy infrastructure, balancing the need for reliability, sustainability, and economic feasibility.
The future of steam power plants is increasingly shaped by the need for sustainability, flexibility, and technological advancements. As global energy systems evolve, steam turbine technology must adapt to integrate with renewable energy, reduce emissions, and enhance efficiency through innovative engineering solutions. The role of steam turbines in power generation is being redefined, particularly as the world shifts toward decarbonization and grid modernization.
One of the most significant trends is the integration of steam turbines with renewable energy sources to create hybrid power systems. Solar thermal power plants, also known as concentrated solar power (CSP) plants, use mirrors to focus sunlight onto a receiver, which heats a working fluid to generate steam. Unlike photovoltaic solar panels that directly convert sunlight into electricity, CSP plants utilize steam turbines to produce power in a manner similar to conventional fossil-fuel plants. The advantage of CSP technology is its ability to incorporate thermal energy storage, such as molten salt systems, which allow for electricity generation even after sunset. This helps address the intermittency issues associated with solar energy and provides a stable, dispatchable power source.
Another promising area of development is geothermal power generation. Geothermal plants rely on heat from the Earth’s core to produce steam, which drives turbines to generate electricity. Unlike fossil fuel-based plants, geothermal energy is a renewable and consistent source of power with minimal carbon emissions. Enhanced geothermal systems (EGS) are being developed to expand the reach of geothermal energy by artificially creating reservoirs in deep rock formations, significantly increasing the potential for steam-based renewable electricity.
The role of steam turbines is also being explored in the hydrogen economy. Hydrogen combustion in steam power plants could provide a zero-emission alternative to natural gas and coal. Hydrogen-fueled boilers and combined-cycle plants are being tested as a means of decarbonizing traditional steam-based power generation. However, large-scale adoption will depend on advancements in hydrogen production, storage, and transportation infrastructure. Ammonia, as a hydrogen carrier, is being investigated as an alternative fuel for steam power plants, offering a potentially viable pathway for carbon-free electricity generation.
In parallel with fuel source diversification, advancements in turbine efficiency and design are pushing the limits of what steam power plants can achieve. Supercritical and ultra-supercritical steam cycles have already improved efficiency by increasing steam temperature and pressure, but new research is focusing on advanced cycles such as supercritical carbon dioxide (sCO₂) power cycles. Unlike traditional steam turbines, sCO₂ turbines operate at higher densities and require smaller components, allowing for more compact and efficient power plant designs. These systems have the potential to revolutionize steam-based power generation by reducing material costs, enhancing thermal efficiency, and minimizing water consumption.
The digital transformation of power plants is another key factor in the evolution of steam turbine technology. Modern plants are increasingly adopting advanced monitoring systems, artificial intelligence (AI), and automation to optimize performance, reduce downtime, and improve predictive maintenance. Digital twins—real-time virtual replicas of physical steam turbines—are being used to simulate operations, predict failures, and test optimization strategies before implementing them in actual power plants. This technology enhances reliability, reduces maintenance costs, and extends the lifespan of critical components.
The role of steam power plants in industrial applications is also expanding beyond traditional electricity generation. Industrial steam turbines are widely used in cogeneration (combined heat and power, or CHP) systems, which provide both electricity and process heat for manufacturing, chemical production, and district heating. These systems significantly increase overall energy efficiency by utilizing waste heat that would otherwise be lost in conventional power plants. Many industries, including pulp and paper, food processing, and petrochemicals, rely on steam turbines to meet their energy demands efficiently.
Despite these advancements, the transition toward a low-carbon future presents challenges for existing steam power plants, particularly those dependent on fossil fuels. Governments and regulatory bodies worldwide are implementing stricter emissions standards, prompting the need for carbon capture and storage (CCS) technologies. CCS systems capture CO₂ emissions from power plants and store them underground or use them for industrial applications, such as enhanced oil recovery or synthetic fuel production. While CCS offers a potential solution for reducing the carbon footprint of steam power plants, high costs and energy penalties associated with the process remain significant hurdles.
Additionally, the global shift toward decentralized and distributed energy generation is changing the way steam turbines fit into the energy landscape. Traditionally, large central power stations with steam turbines supplied electricity to vast regions, but modern energy grids are moving toward a mix of smaller, localized generation sources. This trend is driven by the expansion of renewable energy, microgrids, and energy storage technologies. To remain competitive, steam power plants must adapt to provide flexible and responsive generation, potentially operating in hybrid configurations with renewables and battery storage.
Ultimately, the future of steam power generation will be defined by a balance between innovation, environmental responsibility, and economic feasibility. While the share of fossil fuel-based steam power is expected to decline, steam turbine technology will continue to play a critical role in providing reliable baseload power, supporting industrial processes, and integrating with new energy systems. Whether through advanced steam cycles, alternative fuels, digital optimization, or hybrid renewable systems, steam turbines are set to remain a cornerstone of global energy infrastructure in the coming decades.
Small-scale power plants for biodiesel production plants play a crucial role in ensuring energy self-sufficiency and sustainability. These power plants are designed to meet the energy demands of biodiesel production facilities, which require electricity and heat for various processes such as oil extraction, transesterification, and refining. By integrating small-scale power plants with biodiesel production, manufacturers can reduce operational costs, minimize reliance on external energy sources, and enhance the overall efficiency of their production systems.
Small-scale power plants used in biodiesel facilities typically utilize renewable energy sources such as biomass, biogas, and biodiesel itself as fuels. Biomass gasification systems can convert agricultural residues, wood chips, or other organic waste into syngas, which can then be used to generate electricity. Biogas, produced through anaerobic digestion of organic waste, can be used in gas engines or microturbines to produce both heat and power. Additionally, biodiesel-powered generators provide a direct application of the fuel being produced, creating a closed-loop energy system that enhances sustainability.
The selection of power generation technology for a small-scale biodiesel plant depends on factors such as feedstock availability, energy demand, and economic feasibility. Internal combustion engines, steam turbines, and microturbines are commonly employed for power generation. Internal combustion engines, especially diesel engines modified to run on biodiesel, offer a flexible and cost-effective solution. Steam turbines, when integrated with biomass boilers, provide a continuous and efficient power supply, particularly for larger small-scale operations. Microturbines, though relatively expensive, offer high efficiency and low emissions, making them a suitable option for facilities prioritizing environmental performance.
The integration of cogeneration, or combined heat and power (CHP) systems, in small-scale biodiesel plants enhances overall energy efficiency. CHP systems utilize waste heat from power generation for heating processes such as transesterification, distillation, and drying. This significantly reduces energy waste and improves the economic viability of the biodiesel plant. The use of CHP also contributes to lower greenhouse gas emissions by maximizing fuel utilization and reducing the need for fossil fuel-based energy sources.
Economic considerations play a significant role in the deployment of small-scale power plants for biodiesel production. Capital investment, fuel costs, maintenance, and operational efficiency must be carefully assessed to ensure financial viability. Government incentives, feed-in tariffs, and renewable energy policies can influence the feasibility of implementing these systems. In regions where electricity costs are high or grid reliability is poor, small-scale power plants offer a compelling solution for energy security and cost savings.
Environmental benefits are another critical aspect of integrating small-scale power plants with biodiesel production. By utilizing renewable fuels and reducing dependence on fossil fuels, these systems contribute to lower carbon emissions and improved sustainability. The use of locally available biomass or waste-derived biogas reduces transportation-related emissions and promotes circular economy principles by valorizing waste materials.
Advancements in power generation technology, automation, and energy management systems further enhance the performance of small-scale power plants for biodiesel facilities. Smart grid integration, real-time monitoring, and predictive maintenance technologies improve reliability and efficiency. The adoption of modular and scalable designs allows biodiesel producers to expand their power generation capacity as their production scales up.
Overall, small-scale power plants serve as a key enabler of sustainable and cost-effective biodiesel production. By leveraging renewable energy sources, employing efficient power generation technologies, and integrating CHP systems, biodiesel plants can achieve energy independence and environmental benefits. The continued development and adoption of innovative energy solutions will further strengthen the role of small-scale power plants in the transition to a cleaner and more resilient energy future.
Small-scale power plants for biogas plants are another crucial component of sustainable energy production. Biogas plants generate energy from organic waste materials through anaerobic digestion, producing methane-rich biogas that can be utilized for power generation. These small-scale power plants help maximize the efficiency of biogas utilization while providing reliable electricity and heat for industrial, agricultural, and residential applications.
Biogas-fueled power plants typically employ gas engines, microturbines, or fuel cells to convert biogas into usable energy. Internal combustion engines are the most commonly used technology, offering a proven and cost-effective method for biogas power generation. Microturbines provide a compact and efficient alternative, especially in locations where space is limited. Fuel cells, although still in development for widespread use, offer high efficiency and minimal emissions, making them an attractive option for future applications.
The integration of CHP systems in small-scale biogas power plants further enhances energy efficiency by capturing and utilizing waste heat. This makes biogas plants more self-sufficient and economically viable, as they can generate power for internal operations while also supplying excess electricity to local grids or nearby consumers. The use of biogas for power generation significantly reduces reliance on fossil fuels and contributes to carbon neutrality by utilizing waste-derived methane that would otherwise be released into the atmosphere.
Economic and environmental benefits drive the adoption of small-scale power plants in biogas facilities. The ability to generate power on-site lowers energy costs, while the use of organic waste feedstocks reduces landfill disposal and greenhouse gas emissions. Government incentives, carbon credit programs, and renewable energy subsidies further support the deployment of biogas-based power plants, making them an attractive investment for businesses and communities seeking sustainable energy solutions.
Advancements in biogas upgrading and purification technologies continue to improve the efficiency and scalability of small-scale biogas power plants. Technologies such as membrane separation, water scrubbing, and pressure swing adsorption enhance biogas quality by removing impurities, allowing for cleaner and more efficient combustion in power generation systems. As energy storage and smart grid integration evolve, biogas power plants will play an increasingly important role in decentralized and renewable energy networks.
In summary, small-scale power plants for biogas production offer an effective means of utilizing organic waste for sustainable energy generation. By employing efficient conversion technologies, integrating CHP systems, and leveraging supportive policies, biogas facilities can achieve energy independence and contribute to a low-carbon economy. As advancements in biogas technology and energy management continue, small-scale biogas power plants will become an essential part of the global transition toward renewable energy solutions.
Small-scale power plants for geothermal plants offer a promising solution for harnessing geothermal energy in locations with moderate heat resources. These plants utilize geothermal heat to generate electricity on a smaller scale, making them suitable for decentralized energy production, remote communities, and industrial applications. Unlike large geothermal power plants that require extensive infrastructure, small-scale geothermal power plants can be deployed with relatively lower investment costs while still providing a sustainable and continuous energy source.
Geothermal power generation technologies for small-scale applications include binary cycle power plants, organic Rankine cycle (ORC) systems, and Kalina cycle systems. Binary cycle power plants use low-temperature geothermal fluids to heat a secondary working fluid with a lower boiling point, such as isobutane or pentane, which then drives a turbine to generate electricity. ORC systems are a widely used variation of this technology, offering high efficiency and compatibility with low-to-moderate temperature geothermal resources. Kalina cycle systems, which use a mixture of water and ammonia as the working fluid, provide further efficiency gains by optimizing heat exchange processes.
The integration of small-scale geothermal power plants with district heating, greenhouse agriculture, and industrial processes enhances overall energy utilization and economic viability. These plants can provide both electricity and thermal energy, reducing dependency on fossil fuels and supporting local industries. Government incentives, feed-in tariffs, and advances in drilling and exploration technologies continue to drive the adoption of small-scale geothermal power plants, making them a viable and sustainable solution for clean energy generation in various regions worldwide.
Another crucial factor in the design and operation of small-scale power plants for biodiesel production is energy storage and distribution. Since biodiesel plants may experience fluctuating energy demands depending on production cycles, incorporating an energy storage system can help balance supply and demand. Battery storage, flywheels, or thermal storage can be used to store excess energy generated during low-demand periods and release it when production requires additional power. This approach enhances reliability, reduces energy waste, and ensures a consistent power supply even during maintenance or unexpected power fluctuations.
Grid integration is another consideration for small-scale power plants. Some biodiesel facilities may be connected to the local electrical grid, allowing them to sell excess electricity back to the grid under feed-in tariff programs or net metering schemes. This can provide an additional revenue stream while also contributing to local renewable energy supplies. However, grid integration requires compliance with regulations and standards, including voltage stability, synchronization, and safety protocols. In cases where grid connectivity is unreliable or unavailable, off-grid power solutions using biodiesel-fueled generators, solar hybrid systems, or microgrids can ensure uninterrupted plant operation.
Automation and digital monitoring play an increasingly important role in optimizing power plant efficiency. Modern small-scale power plants benefit from smart control systems that monitor energy consumption, adjust power output based on real-time demand, and optimize fuel usage. Internet of Things (IoT) technology and cloud-based analytics enable remote monitoring, predictive maintenance, and fault detection, reducing downtime and improving overall performance. Automated control systems can also integrate multiple power sources, such as biodiesel generators, solar PV, and biomass gasifiers, to ensure seamless operation with minimal human intervention.
Sustainability and circular economy principles further enhance the value of integrating power plants with biodiesel production. Beyond using biodiesel as a fuel source, facilities can explore additional waste-to-energy opportunities, such as utilizing spent biomass residues, waste oils, or glycerol byproducts for heat and power generation. The use of modular and scalable power plant designs allows facilities to expand capacity as production increases without significant infrastructure changes. Moreover, adopting life-cycle assessment (LCA) methodologies helps biodiesel producers evaluate the environmental footprint of their energy systems and identify areas for improvement.
Research and innovation continue to drive advancements in small-scale power generation for biodiesel plants. Emerging technologies such as hydrogen fuel cells, advanced biofuels, and hybrid renewable energy systems hold promise for further improving efficiency and reducing emissions. Policymakers and industry stakeholders are also exploring financing mechanisms, such as green bonds and carbon credits, to support the development of decentralized renewable energy solutions tailored for small biodiesel producers.
Ultimately, the integration of small-scale power plants with biodiesel production represents a strategic move toward energy independence, cost reduction, and environmental responsibility. By leveraging renewable energy sources, optimizing energy use, and embracing technological innovations, biodiesel facilities can achieve long-term sustainability while contributing to the broader transition toward a cleaner energy future.
Another important aspect of small-scale power plants for biodiesel production is the role of hybrid energy systems in enhancing efficiency and resilience. While biodiesel generators serve as a reliable energy source, integrating renewable energy technologies such as solar photovoltaics (PV), wind turbines, and biomass gasifiers can create a more sustainable and cost-effective energy mix. Hybrid systems reduce reliance on a single energy source, optimizing fuel consumption and lowering operational costs. For instance, solar panels can provide electricity during the daytime, reducing the load on biodiesel-powered generators and extending their lifespan by minimizing wear and tear. Similarly, wind turbines can contribute power when wind conditions are favorable, ensuring continuous energy generation without excessive fuel usage.
Microgrid technology further supports the development of resilient energy systems for biodiesel plants. A microgrid is a localized power network that can operate independently or in conjunction with the main electrical grid. It enables biodiesel producers to manage their energy production, storage, and distribution efficiently. In remote areas or regions with unreliable grid access, microgrids offer a decentralized solution, ensuring continuous operations without interruptions caused by external power failures. Advanced microgrid controllers can balance multiple energy sources, prioritize renewable inputs, and optimize power flow to critical plant processes.
The economic feasibility of small-scale power plants for biodiesel production is heavily influenced by fuel supply chain logistics. The cost and availability of feedstocks, transportation infrastructure, and local energy market conditions all impact the viability of an on-site power generation system. In regions where biodiesel feedstocks are abundant and affordable, on-site power generation using biodiesel-fueled generators can be a cost-effective solution. However, in areas where biodiesel production costs are high, hybrid energy systems that incorporate solar or wind power can help offset expenses and improve overall financial sustainability.
Additionally, the policy landscape plays a vital role in supporting or hindering the adoption of small-scale power plants in biodiesel production. Governments and regulatory bodies often provide incentives, tax benefits, and grants for renewable energy projects, making investment in on-site power generation more attractive. Policies promoting decentralized energy production, such as net metering, power purchase agreements (PPAs), and feed-in tariffs, can further enhance the financial viability of biodiesel plant power systems. Compliance with environmental regulations, emissions standards, and safety requirements must also be factored into the planning and implementation of power plants.
Technological advancements in energy storage solutions continue to improve the efficiency and reliability of small-scale power plants. Battery storage systems allow biodiesel plants to store excess electricity generated during periods of low demand and deploy it when energy needs peak. Lithium-ion batteries, flow batteries, and emerging solid-state battery technologies provide flexible storage options that can complement hybrid power systems. Thermal energy storage, where excess heat from power generation is stored and later used for biodiesel processing, offers another avenue for maximizing energy utilization.
Community-scale biodiesel projects can also benefit from shared power generation infrastructure. In rural or cooperative farming communities where multiple small biodiesel producers operate, a centralized small-scale power plant can supply energy to multiple facilities. This approach enhances economies of scale, reduces investment costs per facility, and fosters energy-sharing models that strengthen local resilience. Shared power systems can also integrate additional renewable energy sources, such as small hydro or geothermal, depending on the local resource availability.
Looking to the future, ongoing research in bioenergy and small-scale power generation is expected to yield even more efficient and sustainable solutions. Innovations in biodiesel refining processes, enzyme-based catalysts, and waste-to-energy conversion technologies will contribute to more integrated and self-sufficient production systems. Digitalization and artificial intelligence (AI) will further optimize power plant performance, predicting energy demand patterns, automating system maintenance, and enhancing decision-making for biodiesel producers.
In conclusion, small-scale power plants are a vital component of sustainable biodiesel production, offering energy security, cost savings, and environmental benefits. By leveraging hybrid energy systems, microgrids, advanced storage solutions, and smart technologies, biodiesel facilities can enhance efficiency and resilience. As renewable energy adoption continues to grow, small biodiesel producers will increasingly benefit from innovations that improve sustainability, reduce operational costs, and contribute to a cleaner, decentralized energy future.
Another significant factor in the development of small-scale power plants for biodiesel production is the role of decentralized energy networks in supporting local economies and enhancing energy security. Decentralized energy systems allow biodiesel producers to generate power independently, reducing reliance on national grids, which can be unreliable or expensive in some regions. This is particularly beneficial for rural and remote areas where access to consistent electricity is limited. By producing their own energy, biodiesel facilities not only achieve operational stability but also contribute to local energy resilience, enabling nearby businesses and households to benefit from surplus power through community microgrids or local distribution networks.
One of the key advantages of integrating small-scale power plants with biodiesel production is the potential for energy cost savings. Electricity prices fluctuate based on demand, fuel supply, and geopolitical factors, making energy expenditures a major concern for industrial operations. On-site power generation using biodiesel, biomass, or hybrid renewable systems provides cost predictability, shielding producers from market volatility. Additionally, government incentives, such as tax credits for renewable energy projects, carbon trading schemes, and subsidies for decentralized energy production, further enhance the economic viability of biodiesel-powered generation systems.
From an environmental perspective, the integration of energy-efficient technologies into small-scale power plants significantly reduces emissions and waste. Carbon capture and utilization (CCU) technologies are being explored to capture CO₂ from biodiesel combustion processes, converting it into useful byproducts such as biochar or synthetic fuels. Additionally, the implementation of low-emission combustion systems, exhaust treatment mechanisms, and energy recovery processes helps biodiesel plants meet stringent environmental regulations while minimizing their carbon footprint.
Advanced automation and smart grid technologies are also transforming the efficiency and effectiveness of small-scale biodiesel power plants. Artificial intelligence (AI) and machine learning (ML) algorithms can analyze energy consumption patterns, optimize fuel use, and predict maintenance needs, reducing downtime and operational costs. Smart meters and digital monitoring platforms provide real-time data on energy production, allowing biodiesel producers to make informed decisions on load management, storage, and distribution. Furthermore, blockchain technology is emerging as a potential tool for enabling transparent energy transactions, allowing biodiesel plants to participate in peer-to-peer energy trading networks.
The global push for energy diversification and climate change mitigation is driving innovations in next-generation biofuels and power generation methods. Research in algal biodiesel, waste-derived biofuels, and synthetic fuel production is expanding the range of viable feedstocks for small-scale biodiesel plants. When coupled with next-generation power technologies such as hydrogen fuel cells, supercritical CO₂ turbines, and thermoelectric generators, biodiesel production facilities can become even more energy-efficient and environmentally friendly.
Collaboration between policymakers, industry stakeholders, and research institutions is essential to further advance small-scale biodiesel power plants. Supportive regulatory frameworks, investment in research and development, and knowledge-sharing initiatives will accelerate the adoption of cleaner and more efficient energy systems. International partnerships and technology transfer programs can help developing countries implement sustainable energy solutions tailored to their unique challenges and resources.
In summary, small-scale power plants are crucial enablers of sustainable and cost-effective biodiesel production. By leveraging decentralized energy systems, hybrid renewable technologies, and advanced automation, biodiesel facilities can enhance energy security, improve economic resilience, and minimize environmental impact. Continued innovation in bioenergy and power generation will further strengthen the role of biodiesel plants in the global transition toward cleaner, more sustainable energy solutions.
Small Scale Power Plant for Biogas Plants
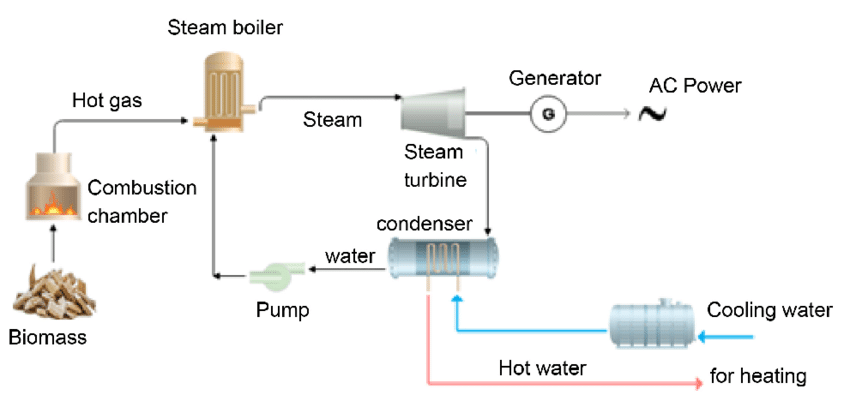
Another critical aspect of small-scale power plants for biogas production is the optimization of biogas utilization to maximize energy output and system efficiency. Since biogas primarily consists of methane and carbon dioxide, upgrading its quality through purification processes enhances its usability in power generation. Technologies such as pressure swing adsorption (PSA), water scrubbing, and membrane separation help remove impurities, ensuring a higher methane concentration for combustion. This results in improved engine performance, reduced emissions, and greater overall efficiency in electricity and heat production.
The integration of energy storage solutions with small-scale biogas power plants further enhances operational stability. Since biogas production can fluctuate due to variations in feedstock availability and digestion rates, incorporating battery storage or thermal energy storage systems allows for better management of energy supply and demand. Excess electricity generated during peak production periods can be stored and used during periods of lower biogas output, ensuring continuous power availability. This is particularly beneficial for off-grid or remote installations that rely solely on biogas for energy needs.
Hybrid power systems that combine biogas with other renewable energy sources, such as solar or wind, further improve efficiency and resilience. A hybrid system allows for more stable energy production by utilizing solar or wind energy when biogas production is low, thereby reducing dependence on fossil fuel-based backup power. Advanced microgrid solutions, incorporating smart control systems, enable seamless integration of multiple energy sources, optimizing power distribution based on real-time energy demand and resource availability.
Economic feasibility remains a key factor in the successful deployment of small-scale biogas power plants. Capital investment in digester infrastructure, purification systems, and power generation equipment must be balanced with potential cost savings from on-site energy generation and revenue from excess electricity sales. Governments and international organizations often provide financial incentives, subsidies, or low-interest loans to encourage the adoption of biogas technology, making small-scale power plants more accessible to agricultural enterprises, municipalities, and industrial facilities.
Environmental sustainability is a driving force behind the growth of small-scale biogas power plants. By capturing methane emissions from organic waste decomposition, biogas systems prevent a potent greenhouse gas from being released into the atmosphere. The digestate byproduct from anaerobic digestion can also be used as a nutrient-rich organic fertilizer, closing the loop in sustainable waste management. Moreover, by replacing conventional fossil fuels with renewable biogas, these power plants contribute to reducing overall carbon emissions, supporting climate change mitigation efforts.
Technological advancements in automation and digital monitoring further enhance the efficiency of biogas power plants. IoT-based sensors and cloud-based data analytics provide real-time insights into digester performance, gas composition, and power generation metrics. Automated control systems adjust operational parameters dynamically, ensuring optimal energy output while minimizing maintenance requirements. Predictive maintenance algorithms help identify potential issues before they lead to system failures, reducing downtime and operational costs.
Looking ahead, the role of small-scale biogas power plants will continue to expand as demand for decentralized, renewable energy solutions grows. Innovations in gasification, microbial fuel cells, and bioelectrochemical systems are expected to further improve energy conversion efficiency and broaden the applicability of biogas technology. The integration of biogas with green hydrogen production and carbon capture technologies presents new opportunities for advancing sustainable energy systems.
In conclusion, small-scale biogas power plants are an essential component of the transition toward a cleaner and more resilient energy future. By leveraging purification technologies, hybrid energy systems, economic incentives, and digital innovations, biogas facilities can optimize their energy production, reduce environmental impact, and enhance long-term sustainability. As research and development in biogas technology progress, small-scale power plants will play an increasingly vital role in achieving energy independence and reducing carbon emissions on a global scale.
Another key factor in the advancement of small-scale biogas power plants is the diversification of feedstocks used for biogas production. Traditional biogas plants primarily rely on agricultural residues, manure, and food waste; however, emerging technologies are enabling the use of alternative organic materials such as algae, industrial wastewater sludge, and lignocellulosic biomass. Expanding the range of feedstocks not only enhances biogas yield but also improves waste management efficiency, turning previously underutilized resources into valuable energy sources.
Feedstock pre-treatment techniques are also playing an increasingly important role in optimizing biogas production. Methods such as hydrothermal processing, enzymatic hydrolysis, and mechanical disintegration help break down complex organic compounds, increasing the availability of digestible material for microbial activity. This leads to higher methane yields and more efficient energy conversion, making small-scale biogas plants more productive and economically viable.
The role of community-scale biogas power plants is also gaining attention, particularly in rural and agricultural regions where decentralized energy solutions are crucial. Cooperative biogas projects allow multiple farms, food processing facilities, and households to contribute organic waste to a shared digester, collectively generating electricity and heat. This collaborative model helps distribute costs, maximize feedstock availability, and provide a stable energy supply for the participating community. In some cases, excess biogas can be upgraded to biomethane and injected into local gas grids or used as a renewable vehicle fuel.
Another promising development is the use of bioelectrochemical systems, such as microbial fuel cells and microbial electrolysis cells, to enhance biogas production efficiency. These systems leverage the metabolic activities of bacteria to generate additional electrical power or produce hydrogen gas alongside methane. Although still in the research and pilot phase, these technologies have the potential to further improve the energy balance of biogas plants, making them even more efficient and sustainable.
The application of artificial intelligence (AI) and machine learning in small-scale biogas power plants is revolutionizing plant operations. AI-driven models can analyze large datasets on feedstock composition, digestion rates, and environmental conditions to optimize biogas production in real time. These predictive analytics tools help biogas plant operators make data-driven decisions on feedstock blending, retention times, and maintenance schedules, leading to increased efficiency and reduced operational costs.
Grid integration strategies for small-scale biogas power plants are also evolving. Many countries are implementing policies that enable biogas power plants to participate in decentralized energy markets, allowing them to sell excess electricity to the grid or engage in demand response programs. This provides additional revenue streams for biogas plant operators while contributing to overall grid stability. The use of smart inverters and virtual power plant (VPP) technology further enhances the ability of small-scale biogas plants to integrate seamlessly with existing energy infrastructure.
As the transition to renewable energy continues, there is growing interest in coupling small-scale biogas power plants with hydrogen production systems. Excess biogas can be used to generate green hydrogen through steam reforming or electrolysis processes, creating a valuable energy carrier for fuel cells, industrial applications, and transport. This synergy between biogas and hydrogen aligns with global efforts to develop low-carbon energy solutions and establish sustainable fuel supply chains.
In summary, the continued evolution of small-scale biogas power plants is being driven by advancements in feedstock utilization, pre-treatment technologies, AI-based optimization, and grid integration. The expansion of community-scale biogas projects, the development of bioelectrochemical systems, and the emerging role of biogas in hydrogen production all contribute to the increasing importance of biogas as a key component of the renewable energy transition. As innovation progresses, small-scale biogas power plants will become more efficient, economically viable, and environmentally beneficial, playing a crucial role in sustainable energy systems worldwide.
The future of small-scale biogas power plants is closely linked to advancements in energy storage and utilization, as well as the development of circular economy models that maximize resource efficiency. One of the primary challenges of biogas power generation is managing fluctuations in biogas production, which can be influenced by feedstock variability, seasonal changes, and digestion efficiency. To address this, hybrid energy storage systems, including batteries and thermal energy storage, are being integrated into small-scale biogas plants to ensure a steady and reliable energy supply. By storing excess electricity generated during peak biogas production periods, these systems enable continuous power availability, improving plant efficiency and resilience.
Additionally, innovations in power-to-gas (P2G) technology are enhancing the potential of small-scale biogas plants. P2G systems allow surplus renewable electricity—such as excess solar or wind energy—to be used for electrolysis, producing hydrogen that can be combined with biogenic CO₂ from biogas upgrading to generate synthetic methane. This approach not only maximizes the use of biogas plant byproducts but also creates a renewable gas that can be injected into natural gas networks or used as a clean fuel for transportation and industrial applications.
Another emerging trend is the development of modular and scalable biogas power plants that can be easily adapted to different operational scales. Containerized biogas systems, for example, offer a plug-and-play solution for small-scale energy projects, enabling rapid deployment in rural areas, farms, and off-grid communities. These prefabricated units come equipped with digesters, gas engines, and power management systems, allowing for easy installation and scalability based on energy demand.
The role of small-scale biogas power plants in sustainable agriculture is also expanding. Many farms are adopting integrated biogas systems that utilize livestock manure, crop residues, and organic waste to produce energy for irrigation, grain drying, and on-farm processing activities. These systems not only reduce dependency on fossil fuel-based energy but also help manage agricultural waste more effectively, reducing methane emissions and improving soil health through the use of digestate as an organic fertilizer.
Policy and regulatory support play a crucial role in the long-term success of small-scale biogas power plants. Governments around the world are introducing incentives such as feed-in tariffs, carbon credits, and investment grants to encourage the adoption of biogas technology. In some regions, decentralized energy policies are facilitating the development of community-owned biogas projects, enabling local stakeholders to participate in renewable energy production and benefit from shared economic and environmental advantages.
Looking ahead, the digitalization of biogas power plants is expected to further optimize their efficiency and operational performance. Advanced sensor networks, real-time monitoring platforms, and automated control systems are allowing operators to track key performance indicators, detect inefficiencies, and implement corrective measures remotely. Blockchain technology is also being explored for transparent tracking of biogas production, carbon savings, and energy transactions, increasing trust and efficiency in decentralized energy markets.
In conclusion, small-scale biogas power plants are poised to play an increasingly significant role in the global transition to sustainable energy. Through the integration of energy storage solutions, modular designs, digital technologies, and policy-driven incentives, these plants are becoming more efficient, flexible, and accessible. As advancements in power-to-gas technology, hybrid renewable energy systems, and circular economy practices continue to emerge, small-scale biogas power plants will contribute not only to energy security but also to environmental protection and economic development. Their growing importance in rural electrification, sustainable agriculture, and decentralized energy networks underscores their potential as a key component of a resilient, low-carbon energy future.
Small-scale geothermal power plants are often used in locations where there is moderate geothermal activity, or for situations where larger-scale plants are not viable due to space, budget, or infrastructure constraints. These plants can produce electricity using the heat from the Earth, typically from hot water or steam reservoirs. Here’s a breakdown of the key features and considerations for small-scale geothermal power plants:
Types of Small-Scale Geothermal Power Plants:
- Binary Cycle Power Plants:
- These are often used in areas with lower temperature geothermal resources (below 400°F or 200°C). The hot water or steam from the geothermal reservoir is passed through a heat exchanger where it heats a secondary fluid with a lower boiling point (like isobutane or pentane).
- The secondary fluid evaporates and drives a turbine, generating electricity. Afterward, the secondary fluid is condensed and recycled.
- These systems are more compact and can operate at lower temperatures compared to flash or dry steam plants.
- Flash Steam Power Plants:
- Suitable for higher-temperature geothermal resources (above 400°F or 200°C).
- The geothermal fluid is extracted, and its pressure is reduced (flashed), causing part of the fluid to vaporize. The steam is then used to drive a turbine for electricity generation, while the remaining water is reinjected into the ground.
- Flash plants are typically larger, but there are smaller-scale versions with reduced turbine capacities.
- Dry Steam Power Plants:
- These are used when there is a direct source of steam from the geothermal reservoir, which can be sent directly to the turbine without needing to convert water into steam.
- These systems are less common for small-scale applications due to the specific and high-quality geothermal resources needed.
Key Advantages of Small-Scale Geothermal Power Plants:
- Sustainability:
- Geothermal energy is a renewable resource, and small-scale plants help reduce dependence on fossil fuels. They provide a clean energy solution with minimal carbon emissions.
- Reduced Environmental Impact:
- The environmental footprint of small geothermal plants is relatively low compared to larger facilities or other forms of power generation. The land use is smaller, and the impacts on local ecosystems are typically minimal.
- Low Operating Costs:
- Geothermal plants generally have low operating and maintenance costs once built, as geothermal energy is a free resource. Small plants benefit from a reduced initial capital investment and have lower overhead compared to large-scale facilities.
- Energy Security:
- Small-scale geothermal plants can provide localized, stable, and independent energy production, reducing reliance on external energy sources.
Challenges of Small-Scale Geothermal Power Plants:
- Initial Capital Investment:
- While operating costs are low, the initial costs of drilling and establishing a geothermal plant can be significant. The drilling phase, in particular, can be expensive, as it requires deep wells to access geothermal resources.
- Resource Availability:
- Small-scale geothermal power plants depend heavily on the availability of geothermal resources in a given area. Without adequate geothermal potential, these plants may not be feasible or efficient.
- Site Selection:
- Identifying suitable sites for geothermal development is crucial. Not all regions have access to the geothermal heat needed for power generation, and poor site selection can result in reduced efficiency or the inability to operate a plant.
- Grid Connection and Energy Storage:
- Depending on the location and size of the plant, integrating small geothermal plants into the power grid can present challenges. In some cases, energy storage systems might be needed to manage the intermittent nature of the grid demand.
Technology and Innovations:
- Enhanced Geothermal Systems (EGS): A newer approach that involves stimulating geothermal reservoirs by creating fractures in hot dry rock to allow water to flow through, thus creating a more accessible heat source. Small-scale plants using EGS are a potential breakthrough, especially for areas with no natural geothermal resources.
- Modular Power Plants: There are also innovations in modular systems that can be scaled up or down depending on the resource potential and demand, making it easier to adapt to various market sizes.
Applications:
- Remote Locations: Small geothermal power plants are especially useful in remote or off-grid locations where connecting to a national grid is not feasible.
- Industrial or Agricultural Use: Geothermal power can be used directly for heating or in cogeneration (combined heat and power systems) to serve industrial processes, aquaculture, or agricultural needs.
Conclusion:
Small-scale geothermal power plants offer a promising solution for sustainable energy production, particularly in areas with access to geothermal resources. While they face challenges like high upfront costs and resource location issues, they provide a clean, reliable, and cost-effective power source with low environmental impact. Advances in technology and modular plant designs are making these systems increasingly viable for small and remote communities.
Small-scale Geothermal Power Plants
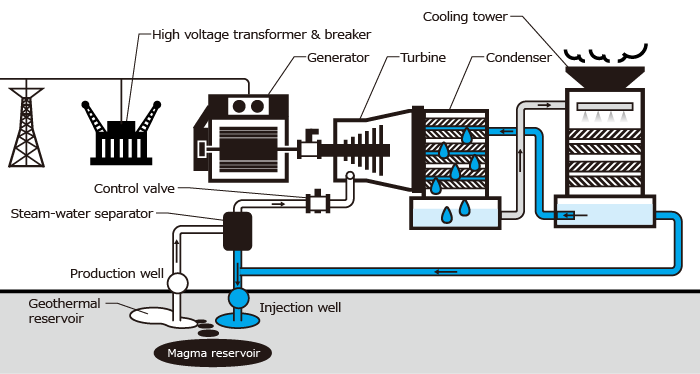
Small-scale geothermal power plants are typically used in areas where the geothermal resource is moderate or where space, budget, or infrastructure limits larger installations. They work by harnessing the heat from beneath the Earth’s surface to generate electricity, with several technologies available for conversion.
The primary advantages of small-scale geothermal plants are sustainability and low environmental impact. Geothermal energy is renewable and generates minimal carbon emissions. These plants also have reduced land use and ecosystem disruption, making them an attractive option for environmentally conscious power generation. Once constructed, the operating costs for geothermal plants are relatively low, as the geothermal resource itself is free. The low overhead costs and efficiency of geothermal energy also help make these systems economically viable.
Another significant advantage is energy security. Small-scale plants provide localized, stable power that is independent of external sources, reducing reliance on imported or grid-based electricity. For remote areas or regions that are difficult to connect to the main grid, small-scale geothermal plants can be particularly useful. Additionally, geothermal systems can be integrated into other systems for heating purposes, serving industrial, agricultural, or residential needs.
However, there are some challenges to consider. The initial capital investment required to build these plants can be high, especially when it comes to drilling wells to access the geothermal resource. Site selection is crucial because the success of the plant depends on having access to enough geothermal heat to make power generation feasible. Not all regions have viable geothermal resources, and poor site selection can lead to a loss of efficiency or even render a project unworkable.
There are also technical hurdles in connecting small-scale geothermal plants to the power grid. While some plants may function independently, others may need to address the challenge of intermittency and storage to align with grid demand. To manage this, some plants incorporate energy storage systems, but this can add another layer of complexity and cost.
Technological advancements are making small-scale geothermal plants more efficient and adaptable. The development of enhanced geothermal systems (EGS) has opened up the potential for creating geothermal reservoirs in areas that don’t have natural geothermal resources. EGS involves stimulating hot dry rock formations to create fractures through which water can flow, improving heat extraction. Furthermore, the modular nature of some geothermal systems means that power plants can be scaled up or down based on the specific geothermal resource and demand of the location.
Despite the challenges, small-scale geothermal plants are gaining traction, particularly for communities looking to reduce their dependence on fossil fuels and stabilize their energy supply. Whether used for local grid integration, off-grid power, or industrial heating, these plants are proving to be an effective and sustainable energy solution in the right conditions.
Building on the previous points, small-scale geothermal power plants continue to gain attention due to their growing potential to address energy needs while reducing environmental impacts.
Expanding Applications and Potential
Small-scale geothermal power plants can be particularly beneficial in various settings. For instance, in remote or isolated locations where access to the national grid is limited or unavailable, geothermal energy offers an effective way to generate reliable power. This can be vital for rural communities, islands, or mining operations that require a steady energy supply but may struggle to connect to large-scale energy infrastructure. In these scenarios, small-scale geothermal plants can provide long-term, sustainable power.
Moreover, the versatility of geothermal energy extends beyond just electricity generation. Cogeneration systems, where both electricity and useful heat are produced, are increasingly popular. These plants can serve industrial processes that require both power and heat, such as in food processing, paper mills, and district heating systems. For agricultural sectors, geothermal heat can be used for greenhouse heating, aquaculture, or drying crops. In regions with both geothermal resources and strong agricultural or industrial sectors, the ability to provide multiple forms of energy (electricity and heat) can make geothermal plants an even more attractive solution.
Technological Developments in Geothermal Energy
One area of development is the growing interest in modular geothermal systems. These are smaller, more flexible plants that can be scaled up or down depending on the resource available and the power demand. Instead of large, centralized plants, modular units can be deployed to meet specific community needs, with potential for expansion as demand grows. These smaller systems are more adaptable to various geographies and may reduce the need for large, upfront investments. The modular approach can also help mitigate risks by allowing developers to test and optimize smaller-scale operations before committing to larger-scale geothermal projects.
Another emerging technology is the enhanced geothermal systems (EGS) approach. EGS techniques allow for the stimulation of geothermal resources in areas that might not have sufficient natural geothermal activity. By creating artificial reservoirs in hot dry rock, water can be introduced to create steam for power generation. Although EGS is still in the early stages of commercialization, it holds tremendous promise in unlocking geothermal resources in regions that were previously considered unsuitable. For smaller-scale applications, this could enable a broader geographic range of geothermal power plants, especially in areas where traditional geothermal resources are not present.
Additionally, direct use geothermal energy (as opposed to generating electricity) is gaining popularity in some areas. In this system, geothermal energy is used directly for heating purposes in homes, buildings, or even for spa-like applications in tourism areas. Small-scale geothermal plants that focus on direct use rather than electricity generation can offer an alternative that reduces the need for complex power plant infrastructures, while still utilizing the Earth’s heat efficiently. This approach can also be combined with district heating systems, where geothermal heat is used to warm multiple buildings or facilities within a specific geographic area.
Challenges and Future Directions
Despite the potential, there are ongoing challenges that need to be addressed for small-scale geothermal power plants to thrive. One such challenge is the geothermal resource management. While geothermal energy is renewable, it is important to manage the resources carefully to avoid depleting them. This requires efficient monitoring and reinjection of used geothermal fluids into the reservoir. Failure to properly manage resources can lead to a decline in plant efficiency over time. Small-scale geothermal plants may face more difficulty in maintaining an adequate supply of geothermal fluid compared to large-scale operations, as smaller reservoirs may be more sensitive to overuse.
In addition, while the modular nature of smaller plants can offer flexibility, initial capital costs remain a barrier. Although the operating costs of geothermal plants are generally low, drilling and resource exploration can be costly, especially in regions where the resource depth is significant. Financing mechanisms and government support for renewable energy projects, such as grants, subsidies, or tax incentives, can help offset some of these initial costs, but the economic viability of small-scale geothermal plants depends largely on the availability of these financial resources.
Finally, the integration of small-scale geothermal plants into national or local grids remains an important consideration. In regions where geothermal energy is part of the broader energy mix, small plants may need to interact with the grid and potentially balance energy fluctuations. Geothermal energy itself can be quite stable, but depending on the plant’s size and resource availability, it may need to be complemented by other sources of renewable energy or battery storage systems to ensure a consistent supply.
Conclusion
In summary, small-scale geothermal power plants offer significant opportunities for renewable energy generation, especially in areas with geothermal potential. They have a minimal environmental footprint, provide reliable power, and can be versatile in their applications—serving both electricity and heat needs. Technological innovations such as modular systems and enhanced geothermal systems are expanding the possibilities for these plants to be more efficient and adaptable. However, challenges like initial costs, resource management, and grid integration still need to be addressed for these plants to achieve their full potential. With continued development and investment in geothermal technologies, small-scale geothermal power plants could play an increasingly important role in sustainable energy generation worldwide.
As we continue exploring the development and potential of small-scale geothermal power plants, there are a few additional considerations and strategies that can help overcome the challenges and maximize the benefits of these systems.
1. Policy and Regulatory Support
Government policies and incentives play a critical role in the development and success of small-scale geothermal power plants. Regulatory frameworks that encourage renewable energy development, offer financial incentives (such as tax credits or subsidies), and streamline permitting processes can significantly reduce the initial investment burden and accelerate project timelines.
- Feed-in Tariffs (FiTs) and Power Purchase Agreements (PPAs): Governments can establish financial mechanisms like FiTs, which guarantee a fixed price for the electricity produced from renewable sources like geothermal, giving developers a predictable return on investment. Similarly, PPAs between the plant operators and utilities or other large consumers can ensure a stable revenue stream.
- Research and Development Support: Continued support for R&D initiatives can foster innovation in geothermal technologies, including drilling techniques, resource management, and system efficiency. Governments and private entities can work together to fund demonstration projects and pilot programs that explore new applications of small-scale geothermal systems.
- Geothermal Resource Mapping and Data Sharing: One of the major barriers to geothermal plant development is the upfront cost and risk associated with exploration and resource assessment. Governments can aid this process by investing in detailed geothermal resource mapping and sharing data with developers, which reduces uncertainty and encourages investment.
2. Improved Drilling Techniques and Cost Reductions
A significant portion of the cost of building a geothermal power plant comes from drilling wells to access geothermal reservoirs. As drilling technology improves, these costs are expected to decrease, making geothermal energy more competitive.
- Directional Drilling: New techniques, such as horizontal or directional drilling, can help access geothermal resources more efficiently, especially in locations where deep drilling is required. These methods allow operators to reach geothermal resources without having to drill as many wells, lowering costs and reducing surface impact.
- Deep Drilling Innovations: Advances in high-temperature and high-pressure drilling technologies could open up new geothermal fields and make the resource accessible even in regions that were previously considered economically unfeasible. More efficient drilling also allows for deeper reservoirs to be tapped, which could significantly extend the life of geothermal plants.
3. Hybrid and Integrated Systems
Small-scale geothermal plants do not have to operate in isolation. One promising strategy for overcoming intermittency or optimizing energy output is integrating geothermal with other renewable energy sources or storage solutions.
- Hybrid Systems: Small-scale geothermal power plants can be combined with solar or wind energy systems to create hybrid power plants. These systems can leverage the strengths of both geothermal (which provides a steady, base-load supply of power) and solar or wind (which offer peak generation during specific times). By pairing these resources, the overall system can provide more reliable, 24-hour power.
- Energy Storage: While geothermal energy is relatively constant, there may be instances when demand exceeds the plant’s generation capacity or when grid instability occurs. By integrating energy storage systems, such as batteries or thermal storage, small-scale geothermal plants can store excess energy during periods of low demand and discharge it when needed. This makes geothermal systems more flexible and better integrated into the broader energy market.
4. Microgrids and Decentralized Energy Systems
Microgrids, which are localized energy networks that can operate independently of the central grid, are becoming increasingly popular in remote or off-grid areas. Small-scale geothermal plants can be an ideal component of such systems. A microgrid with a geothermal energy source can provide stable, reliable power to a community, even if the larger grid is unavailable or unreliable. These decentralized systems are particularly useful for areas prone to natural disasters or in remote regions that are difficult to connect to centralized infrastructure.
- Resilient Energy Systems: In disaster-prone areas, microgrids powered by geothermal energy can provide greater resilience, ensuring that essential services like hospitals, water treatment plants, and communication systems continue to function even during grid outages. Geothermal microgrids can also support critical industries that require uninterrupted power supply.
5. Environmental and Social Considerations
While geothermal energy is widely regarded as one of the cleanest energy sources, its development still needs to consider environmental and social impacts.
- Resource Management: Sustainable management of geothermal resources is essential to prevent depletion and ensure long-term viability. Effective reinjection practices (where the cooled water is reintroduced into the geothermal reservoir) can help maintain pressure in the system and extend the life of the resource. Developing effective monitoring systems for resource management can prevent overuse and optimize plant efficiency.
- Local Community Engagement: The social impact of geothermal development should not be overlooked. In many cases, local communities can benefit directly from geothermal power plants through job creation, infrastructure development, and increased access to energy. However, there may also be concerns about the environmental impact, including land use and the risk of induced seismicity (small earthquakes caused by fluid injection or extraction). Engaging local communities early in the planning process and addressing their concerns through transparency and collaboration can help build support for the project and ensure that it meets both energy and social needs.
- Ecosystem Impact: While geothermal plants generally have a lower environmental impact compared to fossil fuel-based power generation, it’s important to consider the specific context of each site. The use of chemicals in geothermal fluids, for example, needs to be managed carefully to prevent contamination of local water supplies. In addition, the construction and operation of geothermal plants should minimize disruption to wildlife habitats and ecosystems.
6. Global Expansion and Future Outlook
The potential for small-scale geothermal power plants extends far beyond traditional geothermal hotspots. As technology improves and costs decrease, geothermal energy could become more accessible in regions that were once considered non-viable for geothermal development. Enhanced geothermal systems, modular technologies, and hybrid solutions are all expected to play a significant role in making geothermal power a key player in the global renewable energy landscape.
Additionally, international collaboration on research, technology transfer, and financing could help bring geothermal energy to countries with untapped geothermal potential. Small-scale geothermal plants offer a path toward greater energy independence and sustainability, particularly for developing nations that have limited access to large-scale energy infrastructure.
Conclusion
Small-scale geothermal power plants are emerging as a promising solution for sustainable and resilient energy production. By leveraging geothermal energy’s potential for both electricity generation and direct use applications, these systems offer a clean, renewable alternative to fossil fuels. The combination of technological advancements, supportive policies, and innovative system designs will be key to overcoming the challenges of cost, resource management, and grid integration. With continued development and collaboration, small-scale geothermal energy could play a central role in achieving a sustainable and decentralized global energy future.
Small Scale Power Plant for Water Pumping Stations
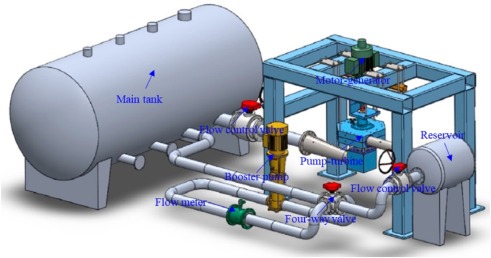
Small-scale power plants for water pumping stations represent an innovative solution for areas where traditional energy infrastructure may be limited or where there is a need for a sustainable and reliable power supply for water management systems. These plants typically provide energy to pump water for agricultural irrigation, municipal water supply, and other essential water management functions.
Key Considerations for Small-Scale Power Plants in Water Pumping Stations
- Power Demand and Load Requirements: Water pumping stations require a consistent supply of energy to operate pumps, often driven by electric motors. The power demand depends on factors such as:
- The flow rate of water needed for irrigation or municipal supply.
- The vertical lift (height the water needs to be lifted).
- The distance over which the water needs to be pumped.
- Renewable Energy Integration: Many small-scale power plants for pumping stations are designed with renewable energy sources in mind. These systems can be particularly useful in rural, remote, or off-grid locations where traditional grid power is not available or too costly to install.Some of the most common renewable energy sources used in small-scale power plants for water pumping include:
- Solar-Powered Water Pumps: Solar energy is widely used for small-scale water pumping systems. Photovoltaic (PV) panels are used to generate electricity that powers pumps. Solar pumps can be used for irrigation, livestock watering, and community water supply in rural areas, especially where the availability of sunlight is reliable. Solar-powered systems can be designed to operate in remote locations without the need for a connection to the electrical grid.The power generated by solar panels is often stored in batteries for use when sunlight is unavailable, such as during the night or on cloudy days. However, battery storage increases the initial cost of the system.
- Wind-Powered Water Pumps: In areas with consistent wind patterns, wind turbines can be used to power water pumps. Wind-powered systems work by converting kinetic energy from the wind into electrical power, which is then used to drive pumps. These systems can be particularly useful in coastal or elevated areas where wind speeds are higher. Wind pumps are often used for irrigation or to provide drinking water in off-grid locations.
- Hydropower for Small-Scale Pumping: In areas where there is a consistent flow of water (e.g., rivers or streams), small hydropower plants can be an excellent option for powering water pumps. By harnessing the kinetic energy of flowing water, small hydroelectric systems can generate continuous power for pumping stations without the need for external fuel sources. Micro-hydropower systems, which generate power from small water flows, can be scaled to meet the needs of local pumping stations.
- Energy Storage Solutions: When using intermittent renewable sources like solar or wind, energy storage is critical to ensuring the reliability of the power supply. Battery storage or pumped storage systems are common solutions.
- Battery Storage: Excess energy generated during peak sunlight or wind hours can be stored in batteries and used when power demand exceeds generation or when renewable energy generation is unavailable.
- Pumped Hydro Storage: For small hydropower systems, a form of energy storage called pumped hydro can be implemented. In this system, excess power is used to pump water to an elevated reservoir, which can then be released to generate electricity when needed, particularly during peak demand.
- System Size and Scalability: Small-scale water pumping systems must be tailored to the specific needs of the station. Factors such as the volume of water to be pumped, the geographical area covered, and the operational hours will determine the size of the power plant. Modular and scalable systems allow for adjustments to the size and capacity of the power plant based on growing demand.For example, in agricultural settings, a small solar-powered pump system might be sufficient for a small farm’s irrigation needs, but as the farm expands, additional solar panels or wind turbines could be added to meet the higher demand.
- Maintenance and Reliability: Small-scale power plants for water pumping stations, especially those powered by renewable energy, tend to have lower maintenance needs compared to traditional diesel-powered generators. Renewable energy systems like solar and wind generally require minimal servicing after installation, with maintenance focused mainly on cleaning panels or inspecting wind turbines. However, the durability of components like pumps, batteries, and turbines needs to be monitored closely to avoid downtime.Regular inspections and preventative maintenance are crucial to ensure the continuous, reliable operation of these systems. For example, maintaining proper water filtration can prevent pumps from becoming clogged or damaged.
Advantages of Small-Scale Power Plants for Water Pumping
- Sustainability and Low Operating Costs: Once installed, renewable energy-based small-scale power plants have very low operating costs, as they rely on free resources (sun, wind, or flowing water) and require minimal fuel. This can be especially beneficial for remote areas where the cost of fuel delivery or grid access is prohibitive.
- Reduced Carbon Emissions: By using renewable energy sources, small-scale power plants can significantly reduce the carbon footprint of water pumping stations compared to diesel or other fossil-fuel-powered generators. This contributes to both local and global sustainability goals.
- Energy Independence: Small-scale renewable energy systems offer energy independence, particularly in rural and remote areas where access to the electrical grid may be unreliable or non-existent. This ensures that critical water supply systems can operate without reliance on external sources of energy.
- Scalability and Flexibility: Small-scale renewable energy systems can be easily scaled or modified to meet changing demands, making them versatile solutions for a variety of water pumping needs. Whether it’s an irrigation system for a small farm or a community water supply, these systems can be adapted as needed.
- Resilience to Fuel Price Fluctuations: Traditional water pumping systems that rely on fuel-powered generators are susceptible to price fluctuations in fuel markets. By using renewable energy, small-scale power plants mitigate this risk and provide a more stable long-term cost structure.
Challenges to Consider
- Upfront Capital Investment: Although the operating costs of renewable energy-powered systems are low, the initial capital investment required for solar panels, wind turbines, or small hydroelectric installations can be significant. This upfront cost can be a barrier for communities or operators with limited access to financing.
- Intermittency of Renewable Sources: The biggest challenge for small-scale renewable energy systems, such as solar and wind, is their intermittency. Solar energy depends on sunlight, and wind energy depends on wind patterns, both of which can be variable. Energy storage is critical to ensure that power is available when the renewable resource is not generating electricity. This adds to the overall system cost and complexity.
- Geographic Limitations: The effectiveness of renewable energy systems is often highly dependent on location. For example, solar energy is most effective in regions with consistent sunlight, while wind power is best suited for areas with strong wind patterns. Similarly, hydropower is only viable where there is a consistent flow of water.
- Infrastructure and Installation: Setting up small-scale power plants in remote areas requires careful consideration of infrastructure, including transportation of equipment, maintenance logistics, and the availability of skilled labor for installation and repair. In areas with limited access to infrastructure, this can be a logistical challenge.
Future Outlook
Small-scale power plants for water pumping stations, especially those utilizing renewable energy, have a bright future. As technology advances and the cost of renewable energy equipment continues to decrease, these systems will become even more accessible. Innovations in energy storage, battery technologies, and hybrid systems will also help improve the reliability and efficiency of small-scale water pumping systems.
As water scarcity and climate change continue to challenge water resources globally, small-scale, sustainable energy solutions for water management will play an increasingly important role. Integrating renewable energy with water pumping infrastructure can help ensure that water management systems remain operational in the face of both environmental and economic challenges.
In the coming years, we can expect to see greater adoption of small-scale renewable energy solutions, especially in underserved or off-grid communities. These systems offer an environmentally friendly, cost-effective, and reliable means of providing water, a vital resource, to those who need it most.
1. Power Demand and Load Requirements (Expanded)
Understanding the power demand and load requirements for water pumping stations is essential to designing an efficient small-scale power plant. Water pumping stations typically serve several purposes, such as irrigation, drinking water supply, industrial processes, and wastewater management, each with different power needs. The design of the small-scale power plant must align with the specific operational requirements of the pumping station, ensuring it can deliver consistent and adequate energy to meet demand. Here are some key aspects to consider:
Factors Affecting Power Demand
- Flow Rate of Water: The volume of water that needs to be pumped is a crucial factor in determining power requirements. For instance, an irrigation system for a small farm may require less power than a municipal water supply system that needs to supply an entire community or city. Flow rate (measured in liters per second or gallons per minute) directly correlates with pump capacity and thus energy consumption.
- Lift Height (Head): The vertical distance water needs to be lifted, referred to as the “head,” significantly impacts the power requirement. Higher lift heights require more energy to push water to the desired level. This is particularly relevant for stations that need to pump water to higher elevations for irrigation, agricultural fields, or storage reservoirs.
- Distance of Water Transport: The horizontal distance over which water must be pumped also affects the total energy required. Longer distances mean more energy is needed to overcome friction losses in pipes or channels. The larger the pipe diameter and the smoother the material, the lower the resistance and power losses. However, as distances increase, more energy is needed to ensure the water reaches its destination efficiently.
- Pump Efficiency: The efficiency of the pump itself plays a significant role in determining the power demand. High-efficiency pumps convert a larger percentage of the input energy into usable water-moving energy, reducing the total energy required for the same output. Conversely, older or poorly maintained pumps may require more power to operate, increasing operating costs.
Peak Demand vs. Base Load
Pumping stations typically experience fluctuating power needs. There are two key categories of power demand to consider:
- Base Load: This refers to the minimum continuous power needed to keep the system running, such as maintaining a constant supply of water in a municipal system or keeping a water reservoir at a certain level. For small-scale power plants, the base load is generally more consistent, requiring a stable energy supply.
- Peak Demand: This occurs during periods of increased water demand, such as during peak irrigation seasons or when there is a sudden surge in water requirements (e.g., firefighting or agricultural harvest periods). Small-scale power plants designed for water pumping stations need to be capable of handling peak loads, which may require additional storage or supplementary energy sources (such as battery storage or backup generators).
Operating Hours and Time of Use
Understanding when the water pumping station will need power is another critical factor in designing the system. For instance, a solar-powered water pumping system would rely on sunlight, so it would be most effective during daylight hours. Conversely, systems powered by wind or hydroelectricity could be more constant but still may need backup power sources for times of low production.
- Daytime vs. Nighttime: In systems powered by solar energy, the pump’s operation will be limited to daylight hours unless battery storage is used. This means energy storage systems need to be designed to store enough power during peak sunlight hours to supply energy during nighttime or cloudy periods.
- Seasonal Variation: In areas with seasonal fluctuations in energy demand (e.g., higher water demand during the dry season for irrigation), small-scale power plants must account for these variations. The system might need to be designed with greater capacity during peak seasons or rely on energy storage solutions to meet seasonal demand.
Energy Efficiency Considerations
Maximizing energy efficiency is critical when designing small-scale power plants for water pumping stations. A well-optimized system can minimize both the initial capital investment and long-term operating costs. Several strategies can be used to increase efficiency:
- Variable Frequency Drives (VFDs): VFDs can be installed to adjust the speed of the pump motor in response to changes in demand. Instead of running the pump at full speed continuously, a VFD ensures the pump operates at the optimal speed required for the desired flow, improving energy efficiency and reducing wear on equipment.
- Pump and Motor Sizing: Properly sizing pumps and motors is essential for avoiding energy waste. Oversized pumps consume more energy than necessary, while undersized pumps may struggle to meet the required flow rates and could be inefficient. Conducting an energy audit and careful system design can ensure that the pump and motor are matched to the flow and lift requirements of the system.
- Energy Recovery: In some cases, it is possible to recover energy from the system to improve efficiency. For instance, in certain pumping stations, a turbine or generator can be installed on the return side of the pump system to capture some of the energy and feed it back into the grid or into storage.
Integrated Control Systems
To optimize the energy usage in small-scale power plants, an intelligent control system can be implemented to monitor power demand and adjust the operation of the plant accordingly. These systems can:
- Track the real-time flow and pressure data of the pumping station.
- Automatically adjust the speed of pumps to match the required load.
- Integrate with weather forecasts to predict energy generation from solar, wind, or hydro sources, enabling the system to adjust to varying conditions.
- Coordinate with backup power sources (e.g., battery storage, diesel generators) to ensure reliable power supply during periods of low renewable energy generation.
Conclusion
Understanding the power demand and load requirements for water pumping stations is crucial in designing a small-scale power plant that meets the specific needs of the station while ensuring efficiency, reliability, and sustainability. Key factors such as flow rate, lift height, energy storage, pump efficiency, and variability in demand must be considered in the design phase. By accurately estimating the power needs, integrating energy-efficient technologies, and accounting for peak demands and seasonal variations, operators can ensure that small-scale power plants provide consistent and reliable energy for water pumping stations. Additionally, incorporating renewable energy sources and energy storage solutions can help create a sustainable, cost-effective system, especially for off-grid or rural locations.
2. Renewable Energy Integration (Expanded)
Renewable energy integration is an essential component of small-scale power plants designed for water pumping stations. By using renewable sources of energy like solar, wind, and small hydro, water pumping systems can reduce their dependency on conventional fossil fuels, lower operational costs, and improve sustainability. The integration of renewable energy can also enhance the reliability of these systems, particularly in remote or off-grid areas.
Solar-Powered Water Pumps
Solar energy is one of the most widely used and effective renewable energy sources for small-scale water pumping systems, especially in regions with abundant sunlight. Solar-powered water pumps use photovoltaic (PV) panels to convert sunlight into electricity, which is then used to drive the pump.
- System Components:
- Solar Panels: Solar panels convert sunlight into electricity. The number of panels needed depends on the power demand of the pumping station and the amount of sunlight available.
- Pump Controller and Inverter: A pump controller regulates the flow of electricity to the pump, and an inverter (if needed) converts the DC electricity generated by the solar panels into AC electricity suitable for the pump motor.
- Energy Storage: To ensure the system operates during cloudy days or at night, battery storage is often included. Batteries store excess power generated during peak sunlight hours and provide energy when the sunlight is not available.
- Applications:
- Irrigation: Solar-powered pumps are commonly used in agricultural irrigation systems, especially in remote areas where access to the electrical grid is limited or non-existent.
- Livestock Watering: Solar-powered water pumps are also used for providing drinking water to livestock in rural or arid regions.
- Community Water Supply: Solar pumps are used to supply drinking water to rural communities, offering a sustainable solution in regions with scarce access to clean water and electricity.
- Advantages:
- Low Operating Costs: Once installed, solar-powered pumps have minimal operating costs, as sunlight is free, and there is little maintenance required.
- Scalability: Solar systems can be scaled to meet specific needs, from small systems for individual farms to larger systems for community-wide water supply.
- Environmentally Friendly: Solar energy reduces greenhouse gas emissions compared to fossil fuel-based energy sources, making it a clean and sustainable solution for water pumping.
- Challenges:
- Intermittency: Solar energy is only available during daylight hours and is weather-dependent. Therefore, energy storage or backup systems are crucial to ensure continuous operation.
- Upfront Costs: While the long-term savings are substantial, the initial capital investment for solar panels, batteries, and installation can be high.
Wind-Powered Water Pumps
Wind energy is another renewable source commonly used to power small-scale water pumps, particularly in areas with consistent wind patterns. Wind turbines convert the kinetic energy from the wind into mechanical energy, which is then used to drive a pump.
- System Components:
- Wind Turbine: A wind turbine generates electricity from wind energy. The size and capacity of the turbine depend on the average wind speeds and the power requirements of the pumping station.
- Pump Mechanism: In some systems, the wind turbine is directly coupled to the pump, eliminating the need for electrical conversion. In others, a generator is used to produce electricity that powers the pump motor.
- Energy Storage: Like solar-powered systems, wind-powered systems may include batteries or other forms of storage to ensure reliable operation during times of low wind.
- Applications:
- Remote Water Supply: Wind-powered pumps are often used for small water supply systems in remote areas with reliable wind resources.
- Agricultural Irrigation: Wind-powered pumps can be integrated into irrigation systems, providing a consistent and renewable energy source for farms.
- Livestock and Wildlife Watering: Wind pumps can serve rural livestock operations or be used in wildlife conservation projects where water is needed in off-grid locations.
- Advantages:
- Reliable in Windy Areas: In regions where wind speeds are consistent and predictable, wind-powered pumps provide a reliable energy source for water pumping.
- Low Operating Costs: Like solar systems, wind-powered pumps have low operating costs once installed, as wind is free, and turbines require minimal maintenance.
- Off-Grid Capability: Wind energy is particularly useful in off-grid locations where connection to the electricity grid is not feasible or cost-effective.
- Challenges:
- Wind Variability: Wind speed can be highly variable, and periods of low wind may require supplemental energy sources or energy storage systems to maintain consistent operation.
- Installation Complexity: Installing wind turbines in remote areas can be complex and expensive, especially when dealing with transportation and local infrastructure challenges.
Small-Scale Hydropower for Water Pumping
In locations where a consistent water source is available, small-scale hydropower can provide an excellent solution for powering water pumps. A micro-hydropower system uses the kinetic energy of flowing water (from rivers, streams, or even irrigation canals) to generate electricity.
- System Components:
- Turbine: A turbine converts the kinetic energy of flowing water into mechanical energy. The water flow rate and head height determine the size and type of turbine required.
- Generator: A generator converts mechanical energy into electrical power, which is used to power the pump.
- Energy Storage or Backup: Small-scale hydropower systems can be combined with battery storage or a grid connection to ensure continuous power, especially if the flow rate fluctuates.
- Applications:
- Irrigation Systems: Small hydropower systems are well-suited for irrigation systems, particularly in areas where natural water sources are abundant and reliable.
- Community Water Supply: Small hydro systems can provide water for communities with limited access to grid electricity, offering a sustainable and continuous power source.
- Advantages:
- Constant Power Generation: Unlike solar or wind, hydropower systems can provide continuous power as long as water is flowing, making it a reliable source of energy for water pumping stations.
- High Efficiency: Micro-hydro systems can be very efficient, especially when the water flow is consistent and the system is well-designed.
- Low Operating Costs: Once installed, hydropower systems have very low operating and maintenance costs.
- Challenges:
- Geographical Limitations: Hydropower is only feasible in areas with sufficient water flow, such as rivers or streams, and the system must be properly sized to match the available water resources.
- Environmental Impact: The construction of hydropower systems may have environmental implications, including disruptions to local ecosystems, fish migration, and water quality. Proper environmental assessments and sustainable design practices are necessary to minimize these impacts.
Hybrid Systems
One of the most effective ways to ensure a reliable and consistent power supply for water pumping stations is to integrate multiple renewable energy sources into a hybrid system. Hybrid systems combine different technologies, such as solar, wind, and small hydropower, to take advantage of each source’s strengths and mitigate their individual limitations.
- Solar-Wind Hybrid Systems: By combining solar and wind energy, a hybrid system can provide a more stable energy supply. For example, wind energy may be available during the night when solar power is not, and solar energy may be available during low-wind periods.
- Solar-Hydro Hybrid Systems: In regions with reliable water sources, solar and hydropower systems can be combined to ensure consistent energy availability. Solar power can supplement the hydropower system during times of low water flow or low light.
- Energy Storage: Hybrid systems often integrate energy storage solutions, such as batteries, to store excess power for use during periods of low generation. This improves the overall reliability and resilience of the system.
Advantages of Hybrid Systems:
- Reduced Intermittency: By combining different renewable energy sources, hybrid systems reduce the impact of intermittency (e.g., solar only works when the sun shines).
- Increased Reliability: Hybrid systems increase the reliability of energy supply, especially in regions with variable or seasonal renewable resources.
- Scalability: Hybrid systems are flexible and can be scaled to meet specific energy requirements, allowing for optimal use of available resources.
Challenges:
- Complexity in Design and Maintenance: Hybrid systems require careful integration and maintenance of different components, which can be more complex than standalone systems.
- Higher Initial Costs: The upfront capital investment for hybrid systems is typically higher due to the integration of multiple technologies and energy storage solutions.
Conclusion
Integrating renewable energy into small-scale power plants for water pumping stations offers several benefits, including sustainability, lower operating costs, and energy independence. Solar, wind, and small hydropower systems can provide reliable and continuous power, though their effectiveness depends on local conditions such as climate, geography, and resource availability. Hybrid systems that combine multiple renewable sources are particularly well-suited for ensuring reliability and meeting varying energy demands. Despite the initial investment and the need for storage solutions to address intermittency, the long-term benefits of renewable energy integration make it an attractive option for water pumping stations, particularly in remote or off-grid locations.
3. System Design and Sizing (Expanded)
Designing and sizing a small-scale power plant for water pumping stations requires a detailed assessment of both the water pumping system’s operational needs and the available renewable energy resources. The goal is to match the energy generation capacity of the power plant with the energy consumption requirements of the pumping station to ensure optimal performance. Proper system sizing is crucial to balance capital costs, energy efficiency, and long-term reliability. Here’s a breakdown of the key design considerations and steps for sizing the system:
1. Understanding Energy Requirements
Before designing the power system, it’s essential to gather data on the water pumping station’s energy needs. This includes the power consumption of pumps, the required flow rates, the lift heights, and the operating conditions of the pumping station. The key steps in determining the energy requirements include:
- Flow Rate (Q): This refers to the volume of water to be pumped per unit of time. It is typically measured in liters per second (L/s), cubic meters per hour (m³/h), or gallons per minute (GPM). Estimating the required flow rate is crucial for sizing the pump and power generation system.
- Total Dynamic Head (TDH): The TDH is the total height the water must be lifted by the pump, factoring in the vertical lift (static head) and the frictional losses in pipes and fittings. TDH is typically measured in meters or feet and determines the energy required to overcome resistance during pumping.
- Pump Power Consumption: The power needed by the pump can be calculated using the following formula:P=ρ×g×Q×HηP = \frac{\rho \times g \times Q \times H}{\eta}P=ηρ×g×Q×HWhere:
- PPP is the power required (in watts or kilowatts),
- ρ\rhoρ is the density of water (approximately 1000 kg/m³),
- ggg is the acceleration due to gravity (9.81 m/s²),
- QQQ is the flow rate (in m³/s),
- HHH is the total head (in meters),
- η\etaη is the efficiency of the pump and motor (a value between 0.6 and 0.9, depending on the system’s efficiency).
- Pump Efficiency: The efficiency of the pump system (including the motor and mechanical transmission) will impact the total power required. Higher efficiency means less power is needed for the same output, reducing energy consumption and operational costs.
2. Determining Power Generation Requirements
The power generation system (solar, wind, hydro, or a hybrid combination) must be designed to meet the energy demand of the pumping station. To determine the necessary power generation capacity, the following factors must be considered:
- Renewable Resource Availability: The availability of renewable energy resources varies by location and season. For example:
- Solar Energy: The amount of sunlight in the region, measured in peak sun hours per day, affects the sizing of solar panels. This information can be obtained from solar resource maps or local meteorological data.
- Wind Energy: Wind speed data is used to assess whether wind turbines are a viable option. Wind turbines are typically rated for certain wind speeds, and the average wind speed over time will influence the energy output.
- Hydropower: For hydropower, the available flow rate and head height are critical to determine the potential energy output. Small-scale hydropower systems are typically designed for locations where water flow is consistent, such as streams or canals.
- Energy Storage: To ensure continuous operation of the pumping station, particularly during periods when renewable resources are unavailable (e.g., at night for solar systems or during low wind periods), an energy storage system such as batteries is required. Battery storage helps to store excess energy generated during peak renewable resource availability and discharge it when energy demand exceeds generation capacity.
3. Sizing the Power Generation System
After understanding the energy requirements and resource availability, the next step is to size the power generation system to meet those needs. Here’s how to approach sizing for various renewable energy sources:
- Solar Power System Sizing:
- Step 1: Estimate the daily energy demand of the pump system (in kilowatt-hours per day, kWh/day). This can be calculated by multiplying the power consumption of the pump (in kilowatts) by the number of hours it operates per day.
- Step 2: Assess the average solar resource in the location (typically expressed as average peak sun hours per day). This is a measure of the amount of sunlight available for generating power.
- Step 3: Size the solar panel system by dividing the daily energy demand by the average solar resource. This gives the number of panels required, considering panel efficiency and potential energy losses.
- Wind Power System Sizing:
- Step 1: Calculate the power requirement for the pumping station, similar to solar sizing.
- Step 2: Assess the average wind speed and the capacity of the wind turbine(s) to convert that wind energy into electricity. Wind turbine manufacturers provide power curves based on wind speed.
- Step 3: Choose the appropriate size and number of turbines to meet the energy demand, considering factors like turbine size, efficiency, and the site’s average wind speed.
- Hydropower System Sizing:
- Step 1: Assess the available flow rate and head height at the chosen location.
- Step 2: Calculate the potential energy available using the formula for hydropower power: P=ρ×g×Q×H×η1000P = \frac{\rho \times g \times Q \times H \times \eta}{1000}P=1000ρ×g×Q×H×η Where the variables are the same as mentioned earlier, and the result is in kilowatts (kW).
- Step 3: Choose an appropriately sized turbine and generator to match the available flow and head.
- Hybrid System Sizing: Hybrid systems combine renewable energy sources to maximize reliability and energy availability. In such cases:
- Step 1: Calculate the energy demand of the pumping station.
- Step 2: Assess the available solar, wind, and/or hydro resources and estimate their contribution to the total energy demand.
- Step 3: Size each energy source in proportion to its availability and capacity to meet the load, while factoring in energy storage requirements to ensure continuous operation during off-peak generation times.
4. Incorporating Backup and Energy Storage
Backup power is critical to ensure the pumping station operates continuously, even when renewable resources are unavailable. Energy storage solutions like batteries or pumped hydro storage can help bridge the gap between power generation and demand.
- Battery Storage: The storage capacity of batteries must be sized to meet the load during periods when renewable generation is insufficient. The sizing can be based on the daily energy demand of the pump system and the number of hours the system is expected to run without generation (e.g., overnight for solar-powered systems). For example:Battery Capacity (kWh)=Daily Energy Demand (kWh)×Autonomy Period (hours)\text{Battery Capacity (kWh)} = \text{Daily Energy Demand (kWh)} \times \text{Autonomy Period (hours)}Battery Capacity (kWh)=Daily Energy Demand (kWh)×Autonomy Period (hours)Battery systems should also include some margin for efficiency losses and aging effects.
- Pumped Hydro Storage: For areas with access to sufficient water resources, a small-scale pumped hydro system can serve as energy storage. Water is pumped to a higher elevation during periods of low energy demand and released to generate electricity when needed.
5. Consideration of System Efficiency
When designing a small-scale power plant, it’s essential to account for system losses, including:
- Conversion Losses: Losses in converting energy from one form to another (e.g., from mechanical energy to electrical energy in turbines, or from DC to AC in inverters).
- Transmission Losses: Losses due to resistance in electrical wiring and cables.
- Storage Losses: Energy losses during storage and retrieval from batteries or other storage systems.
It’s important to optimize system components to minimize these losses and maximize overall efficiency. Proper sizing, regular maintenance, and efficient technology selection can reduce energy waste and improve the system’s long-term reliability and cost-effectiveness.
Conclusion
System design and sizing for small-scale power plants in water pumping stations require a thorough analysis of both the energy needs of the pumping station and the renewable resources available at the site. By carefully considering the flow rate, head height, pump efficiency, and renewable resource availability, the system can be optimized to provide the required power while minimizing costs and environmental impact. Properly sized renewable energy generation systems, combined with energy storage and backup solutions, will ensure reliable, sustainable operation, especially in off-grid or remote locations.
4. Small Scale Power Plant for Rural Communities
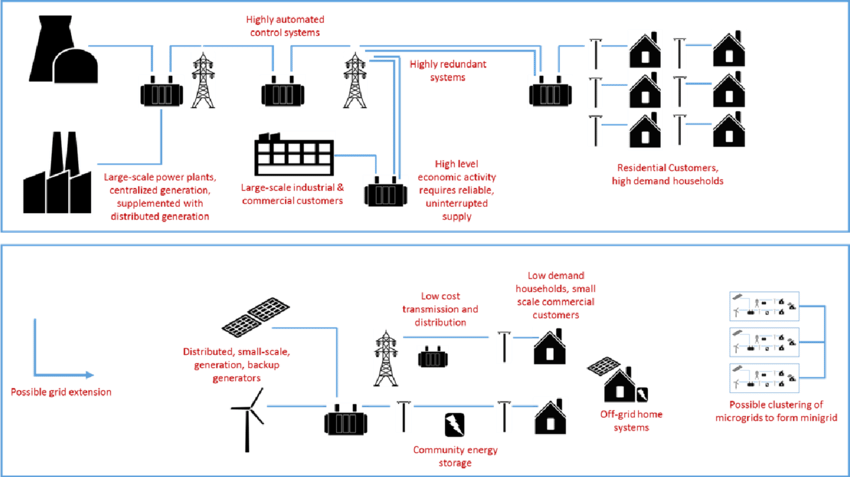
Small-scale power plants for rural communities are designed to provide reliable, affordable, and sustainable electricity where grid access is limited or non-existent. These systems often rely on renewable energy sources, which are more cost-effective and environmentally friendly than fossil fuels. Such power plants can play a crucial role in enhancing the quality of life for rural populations by supporting agriculture, education, healthcare, and overall economic development. The design and implementation of these systems require careful planning and consideration of the local resources, population size, and energy demand.
1. Energy Demand Assessment for Rural Communities
Understanding the energy demand of rural communities is the first step in designing a small-scale power plant. Energy demand in rural areas can vary widely depending on the local economy, population size, lifestyle, and the number of electrified homes and businesses.
Key considerations include:
- Residential Needs: The power required for lighting, heating, and appliances like refrigerators, televisions, and mobile phone chargers.
- Agricultural Needs: Energy for irrigation pumps, crop drying, food processing, and livestock management.
- Community Infrastructure: Energy for schools, hospitals, small businesses, and other community services.
- Industrial Needs: If there are small-scale local industries (e.g., milling, water pumping, or local manufacturing), their energy needs must also be considered.
By accurately assessing energy consumption patterns and peak demand times, the power plant can be sized appropriately. This includes factoring in energy-saving measures such as energy-efficient appliances, and demand-side management techniques.
2. Renewable Energy Sources for Rural Power Plants
Renewable energy systems offer a sustainable and low-cost way to generate electricity for rural communities. The choice of energy source depends on the local resources available, climate conditions, and the energy needs of the community. Common renewable energy sources used for rural power plants include:
- Solar Power: Solar energy is one of the most widely used renewable resources for small-scale power plants, particularly in areas with abundant sunlight. Solar photovoltaic (PV) panels can be installed on rooftops or in fields to generate electricity. Solar power systems are scalable, allowing for incremental expansion as the demand for electricity grows.Advantages:
- Low Operating Costs: Once installed, solar PV systems have low maintenance and operating costs.
- Modular: Systems can be sized and expanded as needed.
- Off-Grid Capabilities: Solar power systems can be used in off-grid areas, where connecting to the national grid is not feasible.
- Intermittency: Solar energy is only available during the day and is dependent on weather conditions.
- Energy Storage: To ensure a reliable power supply during nighttime or cloudy periods, energy storage solutions like batteries or hybrid systems (solar plus backup generators) are necessary.
- Wind Power: Wind turbines can be used to generate electricity in areas with consistent wind patterns. Small-scale wind turbines are particularly useful in rural areas where wind resources are abundant. Like solar systems, wind power systems can be installed in rural settings where grid electricity is not available.Advantages:
- Renewable and Abundant: Wind power is a clean, renewable resource that can provide consistent energy in regions with reliable wind.
- Low Operating Costs: Wind turbines generally have low operational and maintenance costs after installation.
- Wind Variability: Wind speeds can vary seasonally or daily, affecting the reliability of power generation.
- Initial Costs: Wind turbine installations can have a higher upfront cost compared to solar systems, especially if large-scale turbines are needed.
- Micro-Hydropower: For communities located near rivers or streams, small-scale hydropower can be an excellent option. Micro-hydropower systems harness the kinetic energy of flowing water to generate electricity. They are particularly suitable for communities near reliable water sources.Advantages:
- Continuous Power: Unlike solar or wind, hydropower can generate power 24/7 as long as water is flowing.
- High Efficiency: Micro-hydro systems tend to be very efficient compared to other renewable energy systems.
- Location Dependent: Hydropower requires access to flowing water, which may not be available in all rural areas.
- Environmental Impact: If not designed carefully, hydropower systems can disrupt local ecosystems, particularly aquatic life.
- Biomass Energy: Biomass systems convert organic materials (e.g., agricultural waste, wood, or animal dung) into heat or electricity. In rural areas, biomass can be a valuable resource, especially in farming communities where organic waste is abundant.Advantages:
- Waste-to-Energy: Biomass systems can use locally available organic materials, reducing waste and providing a reliable energy source.
- Economic Benefits: Biomass can stimulate local economies by creating jobs in the collection, processing, and conversion of biomass materials.
- Fuel Supply: Continuous fuel supply is necessary for biomass plants, and supply chains must be reliable.
- Emissions: While biomass is renewable, combustion can produce particulate matter and other pollutants, requiring efficient filtration and emission control.
3. Hybrid Power Systems
Hybrid systems that combine multiple renewable energy sources (e.g., solar-wind or solar-biomass) are often the most effective solution for rural communities. These systems take advantage of the different strengths of each energy source to provide more reliable and consistent electricity generation.
For instance:
- A solar-wind hybrid system can take advantage of solar power during the day and wind power during the night or during periods of low solar generation.
- A solar-biomass hybrid system can provide electricity when sunlight is abundant, while biomass can serve as a backup during periods of cloud cover or nighttime.
Hybrid systems can be complemented with energy storage (e.g., batteries) to store excess energy generated during peak production times for use during periods of low generation.
4. System Sizing for Rural Communities
Once the renewable energy resources and system types have been chosen, the next step is to size the power plant appropriately to meet the needs of the rural community. This includes calculating:
- Total Energy Demand: Estimate the total energy needs of the community, including residential, commercial, and industrial demands.
- Renewable Energy Potential: Assess the renewable energy resources available in the area (e.g., solar radiation, average wind speeds, or water flow rates) and estimate the potential generation capacity.
- Energy Storage Requirements: Determine the capacity needed for energy storage to ensure continuous power supply when renewable energy generation is low.
Sizing the system will also involve considering potential growth in energy demand, seasonal variations in resource availability, and maintaining flexibility in the system design to allow for future expansion.
5. Grid Connection vs. Off-Grid Systems
- Off-Grid Systems: In areas where connecting to the national grid is not feasible or cost-effective, off-grid renewable energy systems can provide electricity to rural communities. These systems typically rely on solar, wind, or micro-hydro power, often with battery storage to ensure continuous operation. Off-grid systems are especially important in remote areas where infrastructure development is limited.Advantages:
- Energy Independence: Off-grid systems provide energy independence, allowing communities to rely on local resources rather than external supply chains.
- Reduced Infrastructure Costs: Building an off-grid power system can be cheaper than extending the national grid to remote locations.
- Initial Investment: Off-grid systems may require higher upfront costs due to energy storage systems and renewable energy equipment.
- Maintenance: Remote areas may face challenges with maintaining and repairing off-grid systems.
- Grid-Connected Systems: In some rural areas, small-scale power plants can be connected to the national grid, either to support local energy needs or to export surplus power. This is particularly feasible if there is an existing grid infrastructure that can be expanded or upgraded.Advantages:
- Backup Power: Grid-connected systems can act as a backup during times of low renewable energy generation, allowing the community to draw power from the grid.
- Economic Viability: Surplus energy generated by the power plant can be sold back to the grid, providing additional revenue for the community.
- Infrastructure Costs: Expanding or connecting to the grid requires significant investment in infrastructure, which may not be feasible in remote areas.
- Dependence on External Supply: Grid-connected systems rely on external supply sources, which may still be unreliable in some rural regions.
6. Community Engagement and Sustainability
For a small-scale power plant to be successful in rural communities, it’s important to engage local stakeholders throughout the design, implementation, and operation stages. Community involvement ensures that the power plant meets the specific needs of the population, while local knowledge can help overcome challenges such as fuel supply (for biomass systems) or maintenance.
Key considerations include:
- Capacity Building: Training local technicians and operators to manage, maintain, and repair the power plant ensures the long-term sustainability of the system.
- Financial Models: Developing financing mechanisms that are affordable for rural communities is essential. This could involve government subsidies, microfinance, or pay-as-you-go systems that allow residents to pay for electricity based on usage.
Additionally, environmental sustainability should be a priority when designing renewable energy systems. This includes minimizing the impact of installations on local ecosystems, ensuring efficient use of resources, and considering the long-term resilience of the system in the face of changing weather patterns.
Conclusion
Small-scale power plants for rural communities provide an essential service by delivering reliable, sustainable electricity to areas that are otherwise underserved by traditional grid infrastructure. By leveraging renewable energy sources like solar, wind, hydropower, and biomass, these power plants can meet the energy needs of rural populations while promoting environmental sustainability. Properly designed and sized systems, along with community engagement and capacity building, are critical to ensuring the success and long-term sustainability of rural power plants. Whether grid-connected or off-grid, renewable energy solutions offer significant potential to empower rural communities, improve livelihoods, and contribute to broader economic development.
1. Energy Demand Assessment for Rural Communities
The first step in designing a small-scale power plant for a rural community is to understand its energy demand. This assessment helps in determining the required size and type of the power plant, as well as ensuring that the plant can meet the needs of all sectors within the community, including households, agriculture, local businesses, and essential infrastructure.
1.1. Residential Energy Needs
In rural areas, electricity consumption typically begins with the basic needs of households, including lighting, heating, and powering appliances. The residential energy demand is usually the largest share of a rural community’s total electricity usage.
Common residential appliances and systems that need to be considered:
- Lighting: Most rural households require lighting, especially if they are in off-grid areas.
- Cooking: In some rural areas, electric stoves or cooking appliances may be common.
- Refrigeration: For food preservation, including refrigerators or freezers.
- Communication Devices: Charging stations for mobile phones, radios, and other electronics.
- Entertainment and Media: Powering televisions, radios, and small electronic devices.
To estimate residential demand, the following steps are typically used:
- Identify the average number of people per household.
- Determine the number of electrical devices per household (including the wattage of each).
- Estimate the number of hours each device is used per day.
- Multiply the wattage of devices by usage hours to get kWh per day per household.
For example:
- If each household uses 5 light bulbs (each 15 watts) for 5 hours a day, that’s 5×15×5=375 watt-hours/day=0.375 kWh/day5 \times 15 \times 5 = 375 \, \text{watt-hours/day} = 0.375 \, \text{kWh/day}5×15×5=375watt-hours/day=0.375kWh/day.
- If the community has 100 households, total residential electricity demand for lighting would be 100×0.375 kWh/day=37.5 kWh/day100 \times 0.375 \, \text{kWh/day} = 37.5 \, \text{kWh/day}100×0.375kWh/day=37.5kWh/day.
1.2. Agricultural Energy Needs
Agriculture is often the primary industry in rural communities, and energy is required for several agricultural activities. These needs can include:
- Irrigation: Powering water pumps for irrigation systems, especially in dry or semi-arid regions.
- Livestock Management: Providing power for feed mills, water pumps for animals, and heating for livestock during colder months.
- Crop Processing: Energy for processing crops (e.g., milling, grinding, or drying grains).
- Cold Storage: Refrigeration for storing perishable products like fruits and vegetables.
To assess agricultural energy demand:
- Estimate the number of irrigation systems and the power required for each system (e.g., pump power based on the flow rate and total head).
- Determine energy usage for crop processing (e.g., how much energy is consumed per hour of operation of agricultural machinery).
Example for irrigation:
- A 5 HP pump running for 8 hours a day would consume around 5 HP×0.7457 kW×8 hours=29.83 kWh/day5 \, \text{HP} \times 0.7457 \, \text{kW} \times 8 \, \text{hours} = 29.83 \, \text{kWh/day}5HP×0.7457kW×8hours=29.83kWh/day.
- If there are 10 irrigation systems in the community, the total agricultural energy demand for irrigation would be 10×29.83 kWh/day=298.3 kWh/day10 \times 29.83 \, \text{kWh/day} = 298.3 \, \text{kWh/day}10×29.83kWh/day=298.3kWh/day.
1.3. Community Infrastructure Energy Needs
Essential infrastructure requires energy to ensure the functioning of services that are critical to the community. These include:
- Schools and Educational Facilities: Powering lights, projectors, computers, and other educational tools.
- Healthcare Centers: Powering lights, medical equipment (e.g., refrigeration for vaccines, oxygen machines, etc.), and general healthcare appliances.
- Public Buildings and Offices: Power for administrative functions, lighting, and small equipment.
Each building or facility will have specific energy requirements. For instance:
- A healthcare center may require power for essential equipment like refrigeration and lighting, while a school may focus more on powering classrooms and computers.
To estimate infrastructure demand:
- Identify key facilities in the community (e.g., schools, clinics).
- Determine the energy usage of each facility based on the number of devices and operating hours.
- Sum up energy usage across all critical infrastructure.
For example:
- If a health clinic uses 10 light bulbs (15W each) for 12 hours a day, plus a refrigerator consuming 300W for 24 hours a day:
- Lighting: 10×15×12 hours=1.8 kWh/day10 \times 15 \times 12 \, \text{hours} = 1.8 \, \text{kWh/day}10×15×12hours=1.8kWh/day
- Refrigerator: 0.3 kW×24 hours=7.2 kWh/day0.3 \, \text{kW} \times 24 \, \text{hours} = 7.2 \, \text{kWh/day}0.3kW×24hours=7.2kWh/day
- Total for the clinic: 1.8+7.2=9 kWh/day1.8 + 7.2 = 9 \, \text{kWh/day}1.8+7.2=9kWh/day
1.4. Local Business and Commercial Needs
Small businesses in rural communities also require electricity to operate. These businesses may include:
- Small retail shops: Lighting, refrigeration, and point-of-sale equipment.
- Agro-processing units: Energy-intensive processes like grinding, milling, or packaging.
- Local services: Barber shops, repair shops, or small offices that need lighting and equipment.
Estimates for commercial energy demand should be based on the number of businesses in the community, the types of businesses, and the operating hours. For example:
- A small shop might use around 3 kWh/day3 \, \text{kWh/day}3kWh/day for lighting, refrigeration, and equipment.
- A larger agro-processing facility might consume 50 kWh/day50 \, \text{kWh/day}50kWh/day depending on its operations.
1.5. Estimating Total Community Energy Demand
Once residential, agricultural, infrastructure, and commercial needs are assessed, it is time to aggregate them into a total energy demand for the community. This gives a comprehensive picture of the total daily and peak electricity consumption, which will be necessary for sizing the power generation system.
Total demand can be calculated as:Total Daily Energy Demand (kWh/day)=Residential Demand+Agricultural Demand+Infrastructure Demand+Commercial Demand\text{Total Daily Energy Demand (kWh/day)} = \text{Residential Demand} + \text{Agricultural Demand} + \text{Infrastructure Demand} + \text{Commercial Demand}Total Daily Energy Demand (kWh/day)=Residential Demand+Agricultural Demand+Infrastructure Demand+Commercial Demand
For example:
- Residential demand = 37.5 kWh/day
- Agricultural demand = 298.3 kWh/day
- Infrastructure demand = 9 kWh/day
- Commercial demand = 3 kWh/day
Total = 37.5+298.3+9+3=347.8 kWh/day37.5 + 298.3 + 9 + 3 = 347.8 \, \text{kWh/day}37.5+298.3+9+3=347.8kWh/day
1.6. Peak Demand and Seasonal Variations
The total daily demand is an important starting point, but it’s also critical to consider peak demand (i.e., the maximum energy usage in a day) and seasonal variations:
- Peak Demand: If the community experiences spikes in energy use (for example, when agricultural activities such as irrigation or processing are at their peak), the power system must be sized to accommodate these peaks.
- Seasonal Variations: Some regions may have seasonal variations in energy demand due to agricultural cycles (e.g., higher demand during planting and harvest periods) or weather conditions (e.g., colder months requiring more heating).
Assessing peak demand ensures the system can handle short bursts of higher energy consumption without overloading, while factoring in seasonal load variations ensures consistent and reliable operation year-round.
Conclusion
Accurate energy demand assessment is crucial in designing a small-scale power plant for rural communities. By understanding the needs of residential, agricultural, commercial, and infrastructure sectors, it is possible to estimate the required energy generation capacity. This, in turn, helps determine the type and size of the renewable energy system needed to provide a reliable and sustainable power supply to the community. Additionally, understanding seasonal and peak demand patterns ensures that the system is capable of handling fluctuations in usage while maintaining long-term operational efficiency.
2. Renewable Energy Sources for Rural Power Plants
Rural communities often rely on renewable energy sources for small-scale power plants, as these sources can provide sustainable, cost-effective, and reliable electricity. The choice of renewable energy depends on the local environmental conditions, available resources, and the community’s energy needs. The main renewable energy options for small-scale rural power plants include solar, wind, micro-hydropower, and biomass.
Each source has distinct advantages and challenges, and sometimes a hybrid system combining multiple sources is used to ensure a consistent and reliable power supply.
2.1. Solar Power
Solar energy is one of the most popular and versatile renewable energy sources for small-scale power plants in rural areas, especially in regions with high levels of sunlight. Solar photovoltaic (PV) panels convert sunlight directly into electricity, and the energy can be stored in batteries or fed directly into the grid.
Advantages of Solar Power:
- Abundant and Clean: Solar power is clean and renewable, and sunlight is abundant in many rural areas, especially in arid and semi-arid regions.
- Low Operating Costs: Once installed, solar PV systems have very low operating and maintenance costs, making them economically viable in the long run.
- Scalable and Flexible: Solar systems can be scaled up or down depending on the energy needs of the community. Panels can be installed on rooftops, or large solar farms can be built if more power is required.
- Off-Grid Feasibility: Solar PV systems can be easily adapted for off-grid applications, making them ideal for rural areas without grid access.
Challenges of Solar Power:
- Intermittency: Solar power generation is dependent on sunlight and is unavailable at night or on cloudy days, which can lead to variability in energy production.
- Energy Storage: To provide a continuous power supply during non-sunny hours, energy storage solutions such as batteries are often required. However, battery storage can add to the cost and maintenance requirements of the system.
- Land Use: Large-scale solar installations require significant land area, which may be a constraint in densely populated or agricultural regions.
Sizing a Solar Power System:
- The size of the solar power system will depend on the total daily energy demand of the community. For example, if the community requires 350 kWh/day and each solar panel generates 300 watts in full sunlight for 5 hours per day, the system will need approximately 350 kWh ÷ (0.3 kW × 5 hours) = 233.3 solar panels.
2.2. Wind Power
Wind energy is another key renewable resource for rural communities, particularly in areas where wind speeds are consistently high. Wind turbines convert the kinetic energy of wind into electricity, which can either be stored in batteries or fed into the local grid.
Advantages of Wind Power:
- Reliable and Continuous: In areas with consistent wind patterns, wind turbines can provide a steady supply of energy throughout the day and night.
- Large-Scale Potential: Wind farms can generate significant amounts of energy, making wind power a suitable option for larger rural communities or those with larger energy demands.
- Complementary to Solar: Wind power can complement solar energy systems because wind patterns often peak when sunlight is minimal (e.g., during the night or on cloudy days).
Challenges of Wind Power:
- Intermittency and Variability: Wind speed can fluctuate, and turbines only generate electricity when wind speeds are within a certain range. Low wind speeds will result in less energy production.
- Initial Costs: Wind turbines, especially larger ones, require a significant upfront investment, both in terms of equipment and installation.
- Land and Space Requirements: Wind turbines need significant land area to be effective, and sites with consistent winds are necessary. In some areas, the installation of wind turbines may be restricted due to land use concerns.
Sizing a Wind Power System:
- Wind turbine sizing is determined by the community’s energy demand, average wind speed, and the capacity of the turbines. For example, a 10 kW wind turbine typically produces about 30-40 kWh/day, depending on local wind conditions. If a community needs 350 kWh/day, it may require around 10-12 such turbines.
2.3. Micro-Hydropower
For rural communities located near rivers or streams, micro-hydropower systems can be an excellent renewable energy option. These systems use the kinetic energy of flowing water to generate electricity. Micro-hydro systems can be particularly beneficial in areas with consistent water flow throughout the year.
Advantages of Micro-Hydropower:
- Continuous Power Generation: Unlike solar and wind, hydropower can provide continuous, reliable electricity 24/7, as long as water is flowing.
- High Efficiency: Micro-hydro systems tend to be more efficient than other renewable energy sources and can generate power consistently at low operational costs.
- Low Environmental Impact: Micro-hydropower systems typically have a smaller environmental footprint compared to large-scale hydroelectric dams.
Challenges of Micro-Hydropower:
- Geographic Limitations: Micro-hydropower is only viable in locations with suitable water resources, such as flowing rivers or streams. In regions without adequate water sources, this option may not be feasible.
- Initial Investment: Micro-hydropower systems can be costly to install, especially if infrastructure (such as water diversion channels, turbines, and transmission lines) needs to be built.
- Environmental Concerns: Although generally environmentally friendly, improper design and construction of hydropower systems can disrupt local ecosystems, particularly aquatic life.
Sizing a Micro-Hydro System:
- The system size depends on the available water flow (measured in cubic meters per second) and the height (head) of the water drop. For example, a micro-hydropower system with a 10-meter head and 0.5 cubic meters per second of flow could generate around 5 kW of power continuously.
2.4. Biomass Energy
Biomass energy uses organic materials (such as wood, agricultural residues, or animal dung) to produce heat or electricity. In rural communities with access to agricultural waste, biomass can provide a valuable energy source.
Advantages of Biomass Energy:
- Local Fuel Source: Biomass can be sourced locally, reducing transportation costs and providing a solution to agricultural waste management.
- Waste-to-Energy: Biomass systems turn waste materials into usable energy, helping to reduce environmental pollution and create a sustainable energy supply.
- Reliable Generation: Biomass can provide continuous power generation, as long as fuel is available.
Challenges of Biomass Energy:
- Fuel Availability: Biomass systems require a consistent supply of organic material, which may not always be available. This can lead to challenges in maintaining a steady fuel supply.
- Emissions: While biomass is renewable, its combustion can release carbon dioxide and particulate matter. Efficient combustion technologies and emission control systems are needed to minimize environmental impact.
- Storage and Handling: Biomass materials need to be properly stored and processed, which may involve additional equipment and labor.
Sizing a Biomass System:
- The amount of biomass required will depend on the energy needs of the community and the efficiency of the biomass conversion system. For example, a 50 kW biomass power plant could require several tons of organic material per day, depending on the type of fuel and technology used.
2.5. Hybrid Systems
To overcome the intermittency of solar, wind, and hydropower systems, many rural communities opt for hybrid renewable energy systems that combine two or more sources. Common hybrid systems include combinations of:
- Solar and Wind: Solar power is abundant during the day, while wind power can be most effective during the night or on windy days.
- Solar and Biomass: Solar can meet daytime needs, while biomass can be used to generate power during cloudy days or at night.
- Solar and Micro-Hydro: Solar can provide power when sunlight is available, while micro-hydro can generate power continuously when water flow is sufficient.
Hybrid systems can help ensure a more reliable and consistent power supply while making the best use of available resources. They also provide redundancy, ensuring that if one resource is unavailable, the other can compensate for it.
Conclusion
Choosing the right renewable energy sources for a small-scale power plant in rural communities depends on local resources, climate, and the specific energy needs of the community. Solar, wind, micro-hydropower, and biomass are all viable options, each with its own advantages and challenges. In many cases, hybrid systems that combine multiple renewable sources offer the best solution for ensuring a reliable, sustainable energy supply. By carefully evaluating the available resources and understanding the energy demand, rural communities can design efficient and effective renewable energy systems to meet their needs.
3. Design and Sizing of Small-Scale Power Plants for Rural Communities
Once the energy demand of a rural community has been assessed and the renewable energy sources have been selected, the next step is to design and size the small-scale power plant. Proper design and sizing are crucial for ensuring the system meets the energy needs of the community efficiently, reliably, and economically. This process involves selecting the appropriate generation capacity, choosing the right technology, and considering storage and distribution components.
3.1. Sizing the Power Plant
The sizing of the power plant depends on several factors, including the total energy demand, the renewable energy sources chosen, and the capacity of each energy source. The goal is to ensure that the power plant can generate enough electricity to meet the community’s needs, including providing for peak demand and accounting for seasonal variations.
To size a small-scale power plant:
- Step 1: Estimate Total Energy Demand
As discussed in the previous section, the total daily energy demand for the community should be calculated by adding up the residential, agricultural, infrastructure, and commercial energy needs.Example:
If the total energy demand is 350 kWh/day, this figure represents the minimum generation capacity required. - Step 2: Assess Resource Availability
The availability of renewable resources (solar, wind, hydropower, or biomass) must be evaluated to determine how much energy each resource can reliably produce. This step includes assessing factors like:- Average daily sunlight hours for solar.
- Average wind speeds for wind power.
- Available water flow for micro-hydropower.
- Availability of biomass material for combustion.
- Step 3: Calculate Generation Capacity
Based on the resource availability, calculate how much energy can be generated from each renewable energy source. For instance, if a solar panel produces 0.3 kW per panel and the community needs 350 kWh/day, the total number of panels needed would be:350 kWh/day0.3 kW×5 hours/day=233.3 solar panels\frac{350 \, \text{kWh/day}}{0.3 \, \text{kW} \times 5 \, \text{hours/day}} = 233.3 \, \text{solar panels}0.3kW×5hours/day350kWh/day=233.3solar panelsSimilarly, calculate for other sources like wind or hydro. - Step 4: Account for Peak Demand
The system must also be sized to handle peak demand, which can be higher than average daily usage due to occasional spikes in electricity consumption. The power plant should be capable of meeting these peaks without overloading.Example:
If the peak demand is estimated to be 450 kWh/day, the system must be designed to handle this level of demand, which may require additional capacity or storage solutions to meet these peak periods. - Step 5: Consider Seasonal Variations
Some energy sources are subject to seasonal fluctuations. For instance, wind power may be stronger during the rainy season, and solar power may be less effective during the monsoon. The design should factor in these variations to ensure a consistent power supply throughout the year. A hybrid system that combines different sources can help smooth out these seasonal fluctuations.
3.2. Energy Storage and Backup Systems
Since many renewable energy sources are intermittent (e.g., solar and wind), energy storage is often necessary to ensure a stable power supply when generation is low. There are several storage options, with battery storage being the most common for small-scale systems.
Types of Energy Storage Systems:
- Batteries: Batteries are used to store excess energy generated during periods of high renewable output (e.g., sunny or windy days) for use during periods of low generation (e.g., night or calm days). The most common battery technologies include:
- Lead-Acid Batteries: Cost-effective and reliable, but less efficient and shorter-lasting compared to newer technologies.
- Lithium-Ion Batteries: Higher efficiency, longer lifespan, and more expensive, but increasingly popular due to their performance.
- Flow Batteries: Newer technology that offers longer lifespan and can be scaled more easily, though they are still relatively expensive.
- Grid Connection: In some cases, rural power plants can be connected to the national grid or a local mini-grid, allowing the community to buy power during times of insufficient local generation or sell excess power back to the grid during times of high generation. Grid connection can provide an additional layer of backup and increase system reliability.
- Backup Generators: In remote areas, a diesel or biodiesel generator can be used as a backup when renewable sources fail to meet demand. Although this introduces an additional cost and fuel dependency, it can provide a safety net for critical infrastructure and services during extended periods of low renewable generation.
3.3. Distribution System Design
Once the generation and storage components are in place, the next step is designing the distribution system that will deliver the electricity to the end users. The distribution system typically consists of:
- Transformers: To step up or step down the voltage for safe transmission and use.
- Transmission Lines: To transport electricity from the power plant to homes, businesses, and other facilities.
- Distribution Panels: To regulate and distribute electricity to individual households and buildings.
The design of the distribution system depends on the size of the community, the distance between homes and businesses, and the local topography. In remote areas, underground cables or above-ground poles may be necessary to minimize loss and improve reliability.
Considerations for Distribution System Design:
- Voltage Levels: Rural communities may require different voltage levels for different types of users (e.g., households may need 120/240 V, while businesses may need 380/415 V).
- Losses in Transmission: Power loss in the transmission lines can reduce the efficiency of the system, especially if the community is large and spread out. Proper sizing of cables and optimizing the layout of the distribution network are essential to minimize losses.
- Grid vs. Off-Grid: If the community is connected to the main grid, the distribution system will need to integrate with the national grid. If the community is off-grid, a standalone system will need to be designed to ensure reliable power supply.
3.4. Integration of Multiple Renewable Energy Sources (Hybrid Systems)
For communities that are geographically diverse or have variable energy needs, combining multiple renewable energy sources (solar, wind, hydro, and biomass) can be a highly effective solution. Hybrid systems help reduce reliance on a single resource, ensuring a more reliable and consistent energy supply. The design of a hybrid system requires careful planning to integrate the different sources efficiently, manage power flow, and optimize performance.
Key Considerations for Hybrid System Design:
- Resource Availability: The local resource availability for each energy source must be carefully assessed to determine the most suitable combination.
- Control Systems: Advanced control systems are often needed to manage the integration of multiple renewable sources, energy storage, and backup generators. These systems ensure that the most appropriate energy source is used at any given time and that excess energy is stored or fed into the grid.
- Cost Optimization: While hybrid systems can provide reliable energy, they may also incur higher upfront costs due to the complexity of the design and integration. However, they often reduce the risk of power shortages and lower operating costs over the long term.
3.5. Maintenance and Monitoring
After the power plant is designed, constructed, and commissioned, ongoing maintenance and monitoring are essential for ensuring optimal performance. Regular maintenance of renewable energy systems is necessary to:
- Ensure the longevity of the equipment: Solar panels, wind turbines, and batteries require regular checks to ensure they continue to operate efficiently.
- Address wear and tear: Components like turbines, inverters, and batteries may degrade over time and need replacement or repair.
- Monitor performance: Real-time monitoring systems can track power generation, storage levels, and system performance, alerting operators to any issues before they become major problems.
Maintenance may include cleaning solar panels, lubricating wind turbine components, checking batteries for wear, and inspecting wiring for damage. A well-maintained system will provide reliable energy to the community for years.
Conclusion
The design and sizing of a small-scale power plant for rural communities is a comprehensive process that involves understanding energy demand, selecting appropriate renewable energy sources, and ensuring the system is capable of reliably meeting community needs. Sizing the power plant correctly, accounting for resource availability, and considering energy storage, backup systems, and distribution network design are essential to create a sustainable and efficient energy solution. Additionally, integrating multiple renewable energy sources and regular maintenance ensure that the power plant operates efficiently over its lifespan.
4. Small-Scale Power Plant for Eco-Villages
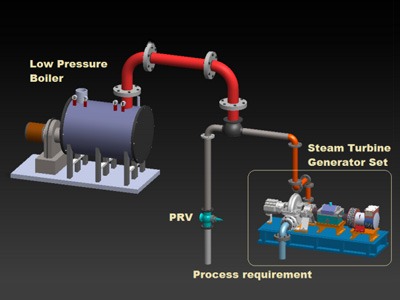
Eco-villages are sustainable, self-sufficient communities that aim to minimize their environmental impact while promoting eco-friendly living practices. The energy systems in these villages are designed to integrate renewable energy sources, promote resource conservation, and ensure long-term sustainability. Small-scale power plants are often central to eco-village energy solutions, providing clean, renewable electricity that aligns with the principles of environmental stewardship.
In designing a small-scale power plant for an eco-village, several factors must be considered, including the community’s energy needs, the available renewable energy resources, and the design of a system that minimizes waste and maximizes sustainability. The following sections detail the key considerations and approaches for designing power plants for eco-villages.
4.1. Key Principles for Eco-Village Energy Systems
The power systems in eco-villages are generally designed to meet specific sustainability goals. These include:
- Energy Independence: Eco-villages aim to generate as much of their own energy as possible through renewable sources, reducing dependency on external power grids and fossil fuels.
- Low Environmental Impact: The design of the power plant should minimize negative environmental effects, including carbon emissions, land degradation, and resource depletion.
- Resource Efficiency: Systems should use available resources in a way that maximizes their potential while reducing waste and improving the community’s overall ecological footprint.
- Resilience: Energy systems should be resilient to external shocks, such as extreme weather events or power supply interruptions, ensuring the eco-village can operate independently if needed.
4.2. Renewable Energy Options for Eco-Village Power Plants
Several renewable energy sources are suitable for eco-village power plants, depending on the geographic location, available resources, and the specific energy needs of the community. A combination of these resources may be used to create a hybrid system that ensures reliable and continuous energy supply.
4.2.1. Solar Power
Solar energy is one of the most common and widely adopted renewable energy sources for eco-villages, particularly in sunny regions. The efficiency of solar systems, their relatively low installation and maintenance costs, and their scalability make them a popular choice.
- Photovoltaic (PV) Panels: Solar panels are typically installed on rooftops, open fields, or community structures. PV systems convert sunlight into electricity, and they can be connected to the grid or be used in an off-grid system.
- Solar Thermal Systems: In addition to PV systems, eco-villages may also use solar thermal technology to provide hot water and space heating, reducing the overall energy demand from electric systems.
Advantages for Eco-Villages:
- Clean, renewable, and abundant energy source.
- Low maintenance and operational costs.
- Scalable systems that can grow with the village’s energy demands.
Challenges:
- Solar power is intermittent and depends on sunlight, which requires additional storage solutions (batteries or thermal storage) to ensure reliability during nighttime or cloudy days.
4.2.2. Wind Power
Wind power is another effective renewable energy source for eco-villages, especially in areas with favorable wind conditions. Small-scale wind turbines can provide reliable electricity for communities, particularly when combined with solar energy.
- Small Wind Turbines: These turbines can be installed on buildings, towers, or other structures, providing power generation without the need for large-scale infrastructure.
Advantages for Eco-Villages:
- Provides reliable and continuous power in areas with strong, consistent winds.
- Can complement solar power by generating energy when solar production is low (e.g., at night or during cloudy weather).
Challenges:
- Wind availability can be variable, so reliable site selection is essential.
- Wind turbines can be visually intrusive and require significant land space.
4.2.3. Micro-Hydropower
For eco-villages located near rivers, streams, or other water sources, micro-hydropower can provide a continuous and reliable source of energy. This type of system uses the kinetic energy of flowing water to generate electricity.
Advantages for Eco-Villages:
- Continuous and reliable energy generation, unaffected by day/night cycles or weather conditions.
- Can provide a constant energy supply for eco-villages near running water sources.
Challenges:
- Only viable in locations with consistent water flow.
- Requires careful design to minimize ecological impact on local waterways and aquatic life.
4.2.4. Biomass and Biogas
Biomass energy, derived from organic materials such as wood, agricultural residues, and food waste, can be used for power generation, heating, and cooking in eco-villages. Additionally, biogas systems can be installed to convert organic waste into methane, which can be used for cooking or generating electricity.
- Biomass Combustion: Organic materials are burned to produce heat or electricity, often used in combined heat and power (CHP) systems.
- Biogas Digesters: Organic waste is converted into biogas through anaerobic digestion, which can be used for cooking or electricity generation.
Advantages for Eco-Villages:
- Reduces waste and creates energy from available organic resources.
- Provides a reliable power source, especially when other renewable sources are unavailable.
Challenges:
- Requires a steady supply of organic material, which may not always be available.
- Can have environmental impacts if not managed properly, such as emissions from combustion or land use issues.
4.2.5. Hybrid Systems
To ensure a continuous and reliable energy supply, many eco-villages opt for hybrid systems that combine multiple renewable energy sources. For example, solar and wind can complement each other by generating electricity during different times of the day, while biomass or micro-hydro can provide power when other sources are unavailable.
Advantages:
- Reduces dependency on a single energy source, providing greater reliability and stability.
- Allows for optimization of each renewable source based on local availability.
Challenges:
- Requires more complex design and installation.
- Potentially higher upfront costs, but long-term reliability and energy security are improved.
4.3. Energy Storage and Management
Because renewable energy sources like solar and wind are intermittent, energy storage is critical for eco-villages to maintain a continuous and reliable power supply. Energy storage systems ensure that excess energy produced during times of high generation (e.g., sunny days or windy periods) can be stored and used when generation is low (e.g., at night or on calm days).
- Batteries: Lead-acid or lithium-ion batteries are commonly used for storing electricity. They allow the village to store excess energy and use it later when needed.
- Thermal Storage: Solar thermal systems can store heat energy for later use, especially in colder climates where heating is required.
- Flywheels: A less common option, flywheel energy storage stores excess energy by converting it into rotational energy, which can later be converted back into electricity.
Storage systems must be designed to meet the specific needs of the eco-village, ensuring sufficient capacity to handle fluctuations in energy supply and demand.
4.4. Energy Efficiency and Demand Side Management
In addition to generating renewable energy, eco-villages prioritize energy efficiency in their buildings and infrastructure. Energy-efficient homes, green building materials, passive solar heating, and energy-efficient appliances reduce the overall energy demand of the community.
Approaches to Energy Efficiency:
- Passive Design: Buildings can be designed to minimize energy use by incorporating natural lighting, good insulation, and ventilation systems.
- Energy-Efficient Appliances: Using energy-efficient lighting (e.g., LEDs), appliances, and heating/cooling systems reduces electricity consumption.
- Demand Side Management: Encouraging residents to use electricity during off-peak hours can reduce pressure on the power system and avoid overburdening the renewable energy sources during peak demand periods.
4.5. Grid Connection and Energy Trading
For eco-villages located in areas where grid connection is possible, the energy system may be integrated into the national or local grid. Excess energy generated by renewable systems can be sold back to the grid, providing additional income or offsetting the costs of the energy system.
- Net Metering: Net metering allows eco-villages to sell excess power back to the grid, providing financial incentives for energy production.
- Microgrids: In some cases, eco-villages may establish their own microgrid that can operate independently from the main grid. This allows them to maintain energy independence while still benefiting from grid connections when necessary.
4.6. Resilience and Adaptation
In eco-villages, energy systems must also be resilient to climate change and extreme weather events. The power plant design should take into account potential environmental disruptions, such as droughts, floods, or heatwaves, which may affect the availability of renewable resources.
- Redundancy: Backup systems such as batteries, hybrid setups, or backup generators can ensure that the community has access to power during emergencies.
- Distributed Systems: Distributing power generation across multiple renewable sources and locations within the eco-village can reduce the risk of a total system failure.
Conclusion
The design of small-scale power plants for eco-villages emphasizes sustainability, energy independence, and minimal environmental impact. By leveraging renewable energy sources such as solar, wind, micro-hydro, biomass, and hybrid systems, eco-villages can create resilient, self-sufficient energy solutions that align with their sustainable living goals. Energy storage, efficiency, and demand-side management further enhance the reliability of these systems, while integration with the grid or microgrids offers additional flexibility. The success of these systems depends on careful design, local resource availability, and a commitment to environmental stewardship, ensuring that the eco-village remains a sustainable, low-impact community.
5. Construction and Implementation of Small-Scale Power Plants for Eco-Villages
Once the design phase is complete, the next step is the construction and implementation of the small-scale power plant. This phase involves the actual building of the infrastructure, the installation of renewable energy systems, and the setup of associated components like storage, distribution, and backup systems. The goal is to ensure that the system is installed efficiently, cost-effectively, and with minimal disruption to the eco-village community and its environment.
5.1. Site Preparation and Infrastructure Development
Before the construction of the power plant begins, it’s essential to prepare the site where the renewable energy systems will be installed. This includes:
- Clearing and Grading: For solar panel arrays, wind turbines, or biomass facilities, the site needs to be cleared and leveled. In the case of hydropower systems, the area around the water source may require modifications.
- Access Roads: Building or improving roads to the site for the transport of materials and equipment.
- Utility Connections: If the eco-village is connected to the grid, installing transformers, substations, and transmission lines may be necessary.
- Water Management: For hydropower or biomass plants, water and waste management facilities need to be constructed or upgraded to handle the production process.
5.2. Renewable Energy System Installation
The installation process will depend on the type of renewable energy technologies chosen for the eco-village. Below is an overview of the installation steps for each type of system.
5.2.1. Solar Power Systems
- Panel Installation: Solar panels are mounted on rooftops or ground-mounted racking systems. For ground-mounted systems, the panels are often placed in a large open area with optimal sun exposure.
- Inverters: Inverters are installed to convert DC electricity from the solar panels into AC electricity for use in the eco-village.
- Battery Storage (if applicable): Battery banks are set up for storing excess energy generated during the day, so it can be used at night or on cloudy days. The batteries are connected to the inverters and the energy management system.
- Wiring and Distribution: Wiring is installed to connect the panels, inverters, batteries, and other components to the distribution system that will provide electricity to the village.
5.2.2. Wind Power Systems
- Turbine Installation: Wind turbines are installed at optimal heights on towers to capture wind energy. Depending on the size of the wind turbine, installation can require specialized cranes and equipment.
- Power Conversion Equipment: Like solar systems, wind turbines are connected to inverters and battery storage systems to manage the power conversion and storage.
- Grid Integration: If the wind turbine is part of a grid-connected system, it will be linked to the utility grid using transformers and transmission lines.
5.2.3. Micro-Hydropower Systems
- Turbine and Generator Installation: The turbine and generator are installed in a water flow channel, either by a stream or river. Proper water diversion is critical to ensure a steady flow of water through the turbine for energy generation.
- Penstock and Water Infrastructure: A penstock (pipe) is used to carry water from the source to the turbine. Other water infrastructure such as spillways, catch basins, and reservoirs may also be needed.
- Control Systems: Micro-hydropower systems need control systems to regulate the flow of water and adjust power generation to match demand.
5.2.4. Biomass and Biogas Systems
- Biomass Combustion: If the system involves biomass combustion, the facility will need to be equipped with boilers or furnaces that can burn organic material to produce heat and electricity.
- Biogas Digester Installation: For biogas systems, anaerobic digesters are set up to break down organic waste and produce methane gas. The gas is then stored in tanks and converted into electricity using biogas generators or burned for heating.
- Feedstock Management: Sufficient storage and processing systems for organic waste must be created to ensure that the biomass and biogas systems can operate efficiently. This includes composting areas, waste sorting facilities, and storage tanks for biogas.
5.3. Integration of Storage and Backup Systems
As renewable energy systems often produce intermittent power, energy storage systems such as batteries and thermal storage must be integrated to store excess energy. These systems allow the eco-village to use renewable energy during periods when production is low (e.g., at night or during cloudy days for solar power).
- Battery Banks: Batteries are connected to the power generation systems (solar, wind, hydro) to store excess energy and release it when demand exceeds supply.
- Thermal Storage: In systems using solar thermal or biomass for heating, thermal storage tanks may be used to store hot water or heat that can be used when temperatures drop.
- Grid Connection or Backup Generators: For off-grid eco-villages, backup generators or grid connections can serve as additional security in case renewable energy systems fail to meet demand.
5.4. Distribution and Power Management Systems
After the energy generation systems are installed, the distribution system needs to be set up to deliver the power to the eco-village homes, businesses, and infrastructure.
- Power Lines and Transformers: The electrical output from renewable systems is transmitted through power lines and transformers to adjust the voltage for use in homes and buildings.
- Smart Grids: Many eco-villages are adopting smart grid technology for real-time monitoring and control of energy use. Smart grids allow residents and energy managers to track energy consumption, control distribution, and optimize power flows.
- Energy Management Systems: These systems are responsible for regulating the flow of energy between the generation, storage, and distribution systems. They ensure that power is distributed efficiently, and storage systems are charged and discharged as needed to meet the community’s energy demand.
5.5. Environmental and Regulatory Compliance
The construction of renewable energy systems must meet local environmental and regulatory requirements. This includes:
- Environmental Impact Assessments (EIAs): Before construction begins, an EIA may be required to assess the potential environmental effects of the power plant, including impacts on local ecosystems, water resources, and wildlife.
- Permitting: Depending on the location, permits may be required for activities such as water use (for hydropower), air emissions (for biomass), and the construction of large infrastructure.
- Sustainability Standards: Eco-villages typically aim to meet specific sustainability standards, such as zero-carbon emissions or low-impact construction practices. Choosing eco-friendly materials and adhering to green building standards is crucial during the construction process.
5.6. Community Involvement and Education
In eco-villages, community involvement is often a critical component of the construction and implementation process. Residents should be actively involved in the decision-making process and educated on how the energy system works. This helps foster a sense of ownership and responsibility over the energy resources and encourages energy conservation behaviors.
- Workshops and Training: Community members can participate in workshops on renewable energy technologies, energy efficiency, and how to maintain the power systems.
- Collaborative Decision-Making: Engaging the community in discussions about energy system design, implementation, and operation ensures that the system meets local needs and aligns with the eco-village’s values.
5.7. Testing and Commissioning
Once the construction is completed, the entire system undergoes a testing and commissioning phase. During this phase, each component (renewable energy systems, storage, distribution) is tested to ensure it operates as expected. Any issues that arise during testing are addressed before the system is fully operational.
- System Checks: Verify that all components are functioning properly and connected.
- Load Testing: Ensure that the power plant can handle the expected load and meet peak demand.
- Safety Checks: Perform safety inspections on electrical systems, battery storage, and other equipment to ensure they meet safety standards.
Conclusion
The construction and implementation of a small-scale power plant for an eco-village involves careful planning, site preparation, and the installation of renewable energy systems that align with the community’s sustainability goals. By combining solar, wind, hydropower, biomass, and energy storage systems, eco-villages can create self-sufficient and resilient energy solutions. Integration of advanced distribution and energy management systems ensures that the community has reliable power while minimizing waste and environmental impact. The implementation phase also emphasizes community involvement, regulatory compliance, and environmental stewardship, ensuring that the energy system meets the needs of the village both now and in the future.
6. Operation, Maintenance, and Long-Term Sustainability of Small-Scale Power Plants for Eco-Villages
Once the small-scale power plant is constructed and commissioned, the next step involves the ongoing operation, maintenance, and monitoring to ensure long-term sustainability. Proper operation and maintenance are key to ensuring that the renewable energy systems continue to function efficiently and cost-effectively, while maintaining the ecological integrity of the eco-village.
6.1. Ongoing Operation of the Power Plant
The operation of the power plant involves the day-to-day management of the energy production, distribution, and storage systems. Operators are responsible for ensuring that the power plant continues to provide reliable electricity to the eco-village without significant disruptions.
6.1.1. Monitoring Energy Production and Consumption
Continuous monitoring of energy production and consumption is essential to optimize the performance of the renewable energy systems. Smart meters, sensors, and energy management software can provide real-time data on energy output, usage patterns, and system efficiency.
- Energy Management Software: These systems track energy generation and consumption, optimize battery usage, and balance supply and demand. Advanced software can help detect problems early, ensuring quick responses to system issues.
- Real-Time Data: Monitoring systems provide valuable insights into the performance of solar panels, wind turbines, micro-hydro systems, and biomass plants. Operators can use this data to identify inefficiencies or malfunctions.
- Energy Forecasting: By analyzing weather patterns, energy demand forecasts, and historical data, eco-villages can predict energy availability and adjust consumption patterns accordingly.
6.1.2. Demand Response and Load Management
Demand response and load management strategies ensure that the power plant can meet the fluctuating energy demands of the eco-village without overloading the system.
- Peak Shaving: Managing electricity consumption during peak periods (e.g., evenings when residents use most appliances) helps reduce the strain on energy generation and storage.
- Smart Appliances and Grid Integration: Appliances such as washing machines, dishwashers, and electric heating can be linked to the grid or energy management system to operate during off-peak times, thus reducing overall demand.
- Energy Efficiency Education: Regular community workshops can help residents understand how to use energy more efficiently, further reducing overall consumption.
6.2. Maintenance of Renewable Energy Systems
Routine maintenance is crucial for ensuring that renewable energy systems are functioning optimally and avoiding costly repairs or downtime. The maintenance schedule will vary depending on the technology being used.
6.2.1. Solar Power System Maintenance
- Panel Cleaning: Dust, dirt, and debris can reduce the efficiency of solar panels. Cleaning the panels periodically helps maintain optimal performance.
- Inverter and Battery Checkups: Inverters should be checked regularly to ensure they are converting DC electricity to AC efficiently. Battery storage systems require inspection for signs of wear, and their charge cycles should be monitored to ensure they are working correctly.
- System Inspections: Electrical connections and wiring should be inspected periodically to ensure there are no loose connections or signs of wear.
6.2.2. Wind Power System Maintenance
- Turbine Inspections: Wind turbines should be checked for mechanical wear, including rotor blades, bearings, and gearboxes. Regular inspections of the turbine structure are also important to prevent corrosion or other damage.
- Lubrication: Regular lubrication of turbine components reduces friction and prevents unnecessary wear and tear on moving parts.
- Monitoring and Diagnostics: Vibration sensors, wind speed measurements, and power generation data can help identify potential issues before they become serious problems.
6.2.3. Micro-Hydropower System Maintenance
- Waterway Maintenance: The water intake, penstock, and turbines should be cleaned regularly to prevent debris and sediment buildup, which can decrease efficiency.
- Mechanical Parts Inspection: Turbines, generators, and control systems should be inspected for wear and corrosion, particularly in areas exposed to water.
- Flow Monitoring: It’s important to regularly measure water flow and ensure that the system is not blocked or operating at suboptimal levels.
6.2.4. Biomass and Biogas System Maintenance
- Biomass Storage: The biomass feedstock (wood, agricultural waste, etc.) should be stored properly to prevent spoilage, contamination, or pest infestations.
- Biogas Digester Maintenance: Anaerobic digesters require periodic maintenance, including cleaning, checking gas storage pressure, and ensuring that the organic waste is being broken down efficiently.
- Ash and Byproduct Management: For biomass systems, ash should be regularly removed from the combustion area, and any byproducts from combustion or digestion should be handled in an environmentally responsible manner.
6.3. Energy Storage System Management
Storage systems, especially batteries, require particular attention to ensure their longevity and functionality.
- Battery Health Checks: Lithium-ion or lead-acid batteries should be regularly inspected for signs of degradation (e.g., loss of capacity, leakage). Ensuring that batteries are charged and discharged properly extends their lifespan.
- Battery Replacement: Depending on the type of battery used, replacement may be necessary every 5–15 years.
- Thermal Storage Monitoring: For thermal energy storage systems, periodic checks on the insulation and storage tanks are necessary to ensure there are no leaks or loss of heat.
6.4. Long-Term Sustainability Considerations
Ensuring the long-term sustainability of a small-scale power plant in an eco-village involves not only maintaining the systems in optimal working condition but also evaluating new technologies and practices as they emerge.
6.4.1. Upgrades and Technological Innovations
As technology continues to evolve, eco-villages may need to consider upgrading their systems to take advantage of new developments. For example:
- Battery Technology: Advances in battery technology (such as solid-state batteries) could provide more efficient and longer-lasting storage solutions.
- Smart Grid Integration: More advanced smart grid technologies can improve the efficiency of energy distribution and management, helping eco-villages better integrate with the wider energy network if desired.
6.4.2. Repurposing and Recycling
- Recycling Materials: At the end of a system’s life cycle, components should be recycled or repurposed where possible. Solar panels, wind turbines, and batteries all contain materials that can be reclaimed and reused in new products.
- Sustainable Practices: As eco-villages prioritize sustainability, it’s crucial to incorporate circular economy principles into power plant management—such as designing systems with the end-of-life stage in mind and minimizing waste generation throughout the life of the system.
6.4.3. Community Engagement and Education
Ongoing education and community engagement are critical for the continued success of the eco-village power plant. Residents should be encouraged to participate in energy-saving programs, maintain awareness of their energy consumption, and contribute to energy generation (such as by reducing usage during peak times or participating in community clean energy initiatives).
- Workshops and Training: Regular workshops on topics like renewable energy maintenance, energy conservation techniques, and how to troubleshoot simple issues will empower residents to take an active role in the operation of the power plant.
- Community Feedback: Creating avenues for community feedback ensures that any concerns or suggestions regarding the energy system can be addressed promptly. Regular surveys and meetings can help gauge the satisfaction of the community with the energy systems and make necessary adjustments.
6.5. Financial Sustainability and Economic Considerations
To ensure the financial sustainability of the power plant, eco-villages should consider various strategies for cost management and funding:
- Revenue from Energy Sales: If the eco-village is connected to the grid or has a microgrid, revenue from energy sales or net metering can offset some of the operational costs.
- Grants and Incentives: Many governments and international organizations offer financial incentives and grants to promote renewable energy in small-scale or rural settings. Eco-villages should explore available funding opportunities to reduce the initial capital expenditure.
- Cost Management: Ongoing cost management involves optimizing energy consumption, maintaining systems for longevity, and ensuring that replacement parts and maintenance are done cost-effectively.
Conclusion
The operation and maintenance of small-scale power plants in eco-villages are essential to ensure the continued success and sustainability of these renewable energy systems. By adopting rigorous maintenance schedules, monitoring energy production, and implementing energy storage systems, eco-villages can provide reliable and clean energy to their residents. Long-term sustainability can be achieved through the adoption of new technologies, recycling, and community engagement, ensuring that the eco-village power plant remains a resilient and environmentally responsible energy source for generations to come.
7. Economic and Social Impacts of Small-Scale Power Plants for Eco-Villages
The deployment of small-scale power plants in eco-villages not only contributes to environmental sustainability but can also have significant economic and social benefits for the community. By providing clean, renewable energy, eco-villages can reduce energy costs, create jobs, foster local economic development, and improve the overall quality of life for residents.
7.1. Economic Benefits
7.1.1. Reduced Energy Costs
One of the most immediate economic benefits of a small-scale power plant is the reduction in energy costs. By generating energy locally through renewable sources, eco-villages can avoid purchasing electricity from the grid, which is often expensive, especially in remote areas.
- Lower Utility Bills: Residents of the eco-village benefit from lower energy bills as they rely on locally generated renewable energy rather than traditional power suppliers.
- Energy Independence: Small-scale power plants reduce the village’s dependence on external energy providers, making the community more resilient to energy price fluctuations and supply disruptions.
7.1.2. Job Creation
Building and operating a renewable energy system creates various employment opportunities within the eco-village and the surrounding community.
- Construction Jobs: The installation of renewable energy systems (solar panels, wind turbines, hydropower infrastructure, etc.) requires skilled labor such as electricians, engineers, and construction workers.
- Operation and Maintenance Jobs: Once the power plant is operational, there are ongoing job opportunities in maintenance, energy management, and monitoring. Local residents can be trained to take on these roles, leading to the development of a skilled workforce.
- Training and Capacity Building: Many eco-villages also invest in local training programs to equip residents with the skills needed for green energy jobs. This can foster long-term economic development by creating a workforce that is skilled in renewable energy technologies.
7.1.3. Increased Local Economic Activity
By generating its own energy, an eco-village can also stimulate other sectors of the local economy, such as agriculture, small businesses, and tourism.
- Supporting Local Agriculture: Renewable energy can support agricultural activities by providing power for irrigation systems, greenhouses, and processing facilities. This can lead to increased crop yields and more efficient farming practices.
- Business Opportunities: With access to low-cost energy, eco-villages can attract small businesses that rely on energy-intensive operations. This can create a more vibrant local economy, with businesses growing in parallel with the village’s development.
- Sustainable Tourism: Eco-villages that focus on sustainability and renewable energy can attract eco-tourists and visitors interested in sustainable living. This type of tourism often generates income for the local community and raises awareness of renewable energy solutions.
7.1.4. Long-Term Financial Savings
Over the long term, eco-villages can achieve substantial financial savings as renewable energy systems often have low operating costs once they are installed.
- Initial Investment: While the upfront costs of installing renewable energy systems can be significant, these costs are often offset by savings on energy bills over time. The cost of renewable energy technologies has been steadily decreasing, making them increasingly affordable for small communities.
- Return on Investment (ROI): In many cases, the ROI of renewable energy projects can be realized within a few years, particularly when combined with incentives like tax credits, grants, or subsidies from local or national governments.
7.2. Social Benefits
7.2.1. Energy Access and Equity
One of the major social benefits of small-scale power plants in eco-villages is improved energy access. Many rural communities and eco-villages, particularly in developing countries, may have unreliable or no access to the national grid. Small-scale renewable energy solutions can provide affordable and consistent electricity to these communities.
- Improved Quality of Life: Reliable electricity improves daily life in numerous ways, including lighting homes, powering appliances, enabling communication, and supporting education and healthcare facilities.
- Equity and Inclusion: Renewable energy systems in eco-villages can be designed to ensure equitable access, meaning all members of the community benefit from clean and affordable energy. This can reduce disparities in energy access, particularly for disadvantaged groups.
7.2.2. Community Empowerment
The development of small-scale power plants often involves community participation, which can lead to greater social cohesion and a sense of ownership over the energy systems.
- Participatory Decision-Making: Involving residents in decisions about energy system design, implementation, and operation fosters a sense of ownership and empowerment. This participatory approach strengthens community bonds and encourages collective action.
- Local Governance and Leadership: The operation of the energy system can serve as a platform for local leadership and governance, with community members taking responsibility for energy management and distribution. This can also provide leadership training for individuals within the community.
- Cultural and Social Values: Small-scale renewable energy systems often align with the cultural values of eco-villages, which prioritize sustainability, community well-being, and environmental stewardship. This can create a shared sense of purpose and strengthen the village’s social fabric.
7.2.3. Health and Well-Being
Access to reliable and clean energy has significant positive impacts on health and well-being.
- Improved Indoor Air Quality: In many developing communities, reliance on traditional biomass fuels (wood, charcoal, etc.) for cooking leads to poor indoor air quality and respiratory illnesses. By switching to renewable energy systems, eco-villages can reduce indoor air pollution and improve health outcomes.
- Access to Healthcare: Reliable electricity supports healthcare facilities, providing power for lights, medical equipment, refrigeration for vaccines, and communication systems. This is particularly important in remote eco-villages where healthcare facilities may have limited access to energy.
- Education and Learning: With electricity, children and adults in the eco-village have access to better learning environments, including light for evening study and access to digital educational resources.
7.2.4. Strengthening Community Resilience
Small-scale power plants enhance the overall resilience of eco-villages to climate change and other external shocks. By reducing dependence on external energy sources, eco-villages become more adaptable in the face of disruptions to energy supply.
- Climate Resilience: Renewable energy systems, such as solar, wind, and hydropower, are less vulnerable to the impacts of climate change compared to conventional energy infrastructure. This is particularly important in areas prone to energy shortages or extreme weather events.
- Self-Sufficiency and Disaster Recovery: In the event of natural disasters or economic crises, eco-villages with their own renewable energy systems are more likely to maintain power and continue functioning, providing essential services to the community during emergencies.
7.3. Challenges and Considerations
While small-scale power plants bring significant economic and social benefits, there are some challenges to consider:
- Upfront Capital Investment: The initial investment required to build renewable energy systems can be a barrier for some communities. However, this can be mitigated through financing options, grants, and subsidies.
- Technical Skills and Capacity: Maintaining renewable energy systems requires technical skills that may not always be readily available within the community. Training programs and partnerships with external experts can help address this gap.
- System Reliability: Renewable energy systems depend on natural resources, and their performance can be affected by factors such as weather conditions or seasonal variations. Energy storage solutions and grid connections can help mitigate these issues.
- Cultural Acceptance: The introduction of new energy technologies may face resistance if not aligned with local cultural values or practices. Involving the community in the planning and decision-making process helps ensure acceptance.
Conclusion
Small-scale power plants in eco-villages can have profound economic and social impacts. These systems reduce energy costs, create jobs, foster local economic activity, and improve the overall quality of life for residents. They also enhance energy access, promote community empowerment, and improve public health. While challenges such as upfront capital costs and technical training need to be addressed, the long-term benefits of renewable energy systems in eco-villages make them an essential investment for building sustainable, resilient, and prosperous communities.
8. Small-Scale Power Plant for Residential Complexes
Small-scale power plants for residential complexes are becoming increasingly popular as cities and urban areas look for ways to reduce their carbon footprint, lower energy costs, and enhance energy security. By generating electricity locally through renewable sources, residential complexes can ensure a more sustainable and cost-effective energy supply while contributing to the overall reduction of grid demand.
8.1. Overview of Small-Scale Power Systems for Residential Complexes
Residential complexes, particularly multi-unit buildings or gated communities, present a unique opportunity for small-scale power plants. These systems can include a variety of renewable energy technologies, such as solar, wind, geothermal, and combined heat and power (CHP) systems, to meet the energy needs of the entire complex.
8.1.1. Solar Photovoltaic Systems
Solar energy is one of the most widely used forms of renewable energy for residential complexes. Photovoltaic (PV) solar panels are installed on rooftops or in open spaces within the complex to harness sunlight and convert it into electricity.
- Roof-Top Solar Panels: In multi-story buildings, the roofs can be used to install solar panels. The energy produced can be consumed by residents or stored in batteries for later use.
- Solar Power for Shared Areas: In residential complexes, solar energy can be used to power common areas such as hallways, elevators, lighting in parking lots, and recreational areas, reducing the overall energy consumption from the grid.
- Net Metering: If the system generates excess electricity, it can be sold back to the grid through a net metering agreement, providing the complex with additional revenue or credits for future use.
8.1.2. Wind Energy Systems
Wind energy is another viable option for residential complexes, particularly in areas with consistent wind speeds. Small-scale wind turbines can be used in conjunction with other renewable systems.
- Vertical Axis Wind Turbines (VAWTs): These turbines are often better suited for urban environments because they are less sensitive to wind direction and are quieter than horizontal turbines.
- Micro Wind Turbines: These are small turbines that can be installed on the rooftop or other structures within the complex. They are designed for low wind speed areas and are generally suitable for urban settings where space is limited.
8.1.3. Combined Heat and Power (CHP) Systems
CHP systems, also known as cogeneration, can provide both electricity and heating by utilizing a single fuel source, such as natural gas, biomass, or biogas. This system is especially useful for residential complexes that require both electricity and heating services.
- Energy Efficiency: CHP systems are highly efficient because they capture and use heat that would otherwise be wasted during power generation.
- District Heating: The waste heat from a CHP system can be used to provide heating to apartments, shared spaces, and hot water to residents, thereby reducing the need for separate heating systems.
8.1.4. Geothermal Systems
Geothermal energy can be used for heating and cooling purposes in residential complexes. Geothermal heat pumps can be installed to provide consistent temperature control by utilizing the Earth’s natural temperature.
- Ground-Source Heat Pumps (GSHPs): These systems can be used for heating in the winter and cooling in the summer. They are highly efficient and can lower energy costs for residential complexes.
- Geothermal District Heating: Larger residential complexes can implement district-wide geothermal heating and cooling systems, where a central geothermal plant distributes thermal energy to each building or unit.
8.1.5. Energy Storage Solutions
Energy storage solutions, such as batteries, are an important component of small-scale power plants for residential complexes. Storage systems allow excess energy generated by renewable sources (like solar or wind) to be stored and used when generation is low or demand is high.
- Lithium-Ion Batteries: These are commonly used for residential energy storage because they are compact, efficient, and have a long lifespan.
- Community Energy Storage: In larger residential complexes, a shared energy storage system can be implemented, where excess energy from the whole complex is stored for later use.
8.2. Design and Implementation of Small-Scale Power Plants for Residential Complexes
8.2.1. Site Assessment and Feasibility Study
Before implementing a small-scale power plant, a thorough site assessment and feasibility study must be conducted. This will help determine which renewable energy technologies are most appropriate based on factors such as location, climate, energy demand, and available space.
- Solar Irradiance: For solar power, it’s important to assess the amount of sunlight the complex will receive throughout the year.
- Wind Assessment: For wind power, wind speed and consistency need to be measured.
- Energy Demand: A study of the energy consumption patterns of the residents will help determine the size and scale of the renewable energy systems required.
8.2.2. Sizing the System
Sizing the renewable energy system is crucial to ensure that it meets the energy demands of the residential complex. An appropriately sized system will ensure that the residents have reliable energy while minimizing the costs associated with over-sizing the system.
- Energy Modeling: Advanced modeling tools can help simulate the energy needs of the complex and the generation capacity of the proposed renewable systems.
- Storage Considerations: Storage capacity must be matched to the energy generation profile. In places where there is high variability in renewable energy production, larger storage systems may be required.
8.2.3. Grid Connection and Hybrid Systems
While many residential complexes can rely primarily on renewable energy, it is often beneficial to connect to the grid as a backup or for energy trading.
- Grid-Tied Systems: A grid connection allows for the sale of excess energy to the utility company (net metering) or for purchasing electricity when renewable generation is insufficient.
- Hybrid Systems: A hybrid system combines multiple energy sources (e.g., solar and wind) with energy storage and grid connections to ensure a constant, reliable energy supply to the complex.
8.2.4. Financing and Incentives
The installation of renewable energy systems can be costly, but various financing options and government incentives can help reduce the upfront investment.
- Government Incentives: Many governments offer subsidies, grants, or tax incentives to encourage the adoption of renewable energy. Residential complexes can benefit from these financial mechanisms.
- Power Purchase Agreements (PPAs): These agreements allow the residents or developers to finance the system by purchasing energy at a fixed rate, which can often be lower than conventional electricity prices.
- Community Financing Models: In some cases, the costs of renewable energy systems can be shared by the residents or through community-based energy cooperatives, allowing for a shared investment and benefit.
8.3. Operation and Maintenance of Small-Scale Power Plants
The ongoing operation and maintenance (O&M) of renewable energy systems is essential to ensure that the power plant continues to function effectively.
8.3.1. Monitoring Systems
Advanced monitoring systems allow operators to track the performance of renewable energy systems in real-time.
- Remote Monitoring: Many modern systems offer remote monitoring capabilities, allowing operators to diagnose problems and optimize energy production without being physically on-site.
- System Optimization: Monitoring data helps identify inefficiencies in energy generation or consumption, enabling corrective actions to be taken before issues become serious.
8.3.2. Regular Maintenance
Routine maintenance is essential for keeping renewable energy systems in optimal condition.
- Solar PV Systems: Regular cleaning of panels and checks on inverters and battery systems are important for maintaining high efficiency.
- Wind Turbines: Wind turbines require periodic inspections, lubrication, and repairs to keep moving parts functioning smoothly.
- Geothermal Heat Pumps: The pumps should be inspected annually for system leaks and efficiency.
8.3.3. Battery and Storage Maintenance
Batteries require periodic checks to ensure they are charging and discharging correctly. Maintenance schedules should be followed based on the type of battery (e.g., lithium-ion or lead-acid) to extend their lifespan.
8.4. Social and Environmental Impact
8.4.1. Energy Equity
By providing renewable energy to residential complexes, energy costs can be significantly lowered, improving energy equity among residents. Additionally, community members in lower-income areas can benefit from more affordable and stable energy prices.
8.4.2. Carbon Footprint Reduction
Renewable energy systems reduce the overall carbon footprint of the residential complex by reducing reliance on fossil fuels and the grid. This helps mitigate climate change by decreasing greenhouse gas emissions.
8.4.3. Environmental Conservation
Renewable energy systems, such as solar and wind, have minimal environmental impact compared to conventional energy sources. Moreover, using energy-efficient technologies and reducing waste through smart energy management contribute to preserving natural resources.
8.5. Conclusion
Small-scale power plants for residential complexes offer a promising solution to meet the growing demand for sustainable, cost-effective energy in urban settings. By harnessing renewable energy sources such as solar, wind, geothermal, and CHP systems, residential complexes can reduce energy costs, enhance energy security, and promote environmental sustainability. With careful planning, financing, and ongoing maintenance, small-scale power plants can provide long-term benefits for residents, communities, and the planet.
8.1.6. Biomass Energy Systems
Biomass energy systems can also be integrated into residential complexes, especially if there is an available source of organic waste or biomass. Biomass is a renewable energy source derived from organic materials, including agricultural residues, food waste, and wood.
- Biomass Boilers: These systems can provide heating and hot water for residential units by burning biomass materials. Biomass boilers are more sustainable than traditional gas or oil boilers since they use organic waste that would otherwise decompose and release methane—a potent greenhouse gas—into the atmosphere.
- Biogas Production: In some residential complexes, organic waste can be collected and converted into biogas through anaerobic digestion. The biogas can then be used to generate electricity or provide heating.
- Closed-Loop Systems: In a closed-loop biomass energy system, organic waste from the complex itself is used to generate power, creating a circular energy cycle that helps reduce waste and reliance on external resources.
8.1.7. Combined Renewable Energy Systems
Some residential complexes benefit from using a combination of renewable energy sources to ensure a more stable and reliable power supply. These hybrid systems may integrate solar, wind, and biomass technologies, taking advantage of the complementary characteristics of different energy sources.
- Solar-Wind Hybrid Systems: Combining solar and wind energy can help balance energy production by ensuring that when solar energy is not available (during cloudy days or at night), wind energy can pick up the slack.
- Solar-Biomass Hybrid Systems: Biomass systems can be integrated with solar to provide consistent power generation, especially in areas where solar irradiance is low in certain seasons. Biomass can supplement the power supply when solar generation is insufficient, such as during the winter months when sunlight is weaker.
Hybrid systems provide resilience by ensuring that the community continues to receive power even if one energy source is temporarily unavailable.
8.2. Design and Implementation of Small-Scale Power Plants for Residential Complexes (Continued)
8.2.5. Grid Integration and Microgrid Systems
As energy demands grow and renewable energy technologies become more widespread, residential complexes are increasingly adopting microgrids—small, localized energy systems that can operate independently or in parallel with the main grid.
- Microgrids: These systems are capable of generating, storing, and distributing energy to a specific area, such as a residential complex. Microgrids are ideal for regions where grid reliability is poor or energy security is a concern.
- Islanding Mode: In the event of a grid failure, a microgrid can operate in “islanding mode,” providing power to the residential complex even when the grid is down. This makes them highly reliable for emergency situations.
- Energy Management Systems: Microgrids rely on sophisticated energy management systems to optimize the distribution of energy across the residential complex. These systems monitor energy demand, available generation, and storage capacity to ensure that power is delivered efficiently and cost-effectively.
8.2.6. System Configuration and Energy Flow
The configuration of a small-scale power plant for a residential complex involves determining how energy is generated, stored, and distributed to different parts of the complex.
- Decentralized vs. Centralized Systems: Decentralized systems involve small energy production units scattered throughout the complex, while centralized systems aggregate generation at a single point (e.g., a rooftop array or a central CHP system) and distribute energy from there. Each approach has its pros and cons depending on the complexity and layout of the residential complex.
- Energy Distribution: The design should include the installation of inverters, controllers, and transformers to regulate the voltage and current for different appliances and common areas. Additionally, there should be clear wiring and infrastructure in place to prevent overloads and ensure that power is evenly distributed.
8.3. Operation and Maintenance of Small-Scale Power Plants (Continued)
8.3.4. Energy Audits and Performance Monitoring
To ensure the continuous effectiveness of small-scale power systems, it is essential to conduct regular energy audits and performance monitoring. These processes help identify inefficiencies and provide insights into how the energy system can be optimized.
- Energy Audits: Audits are conducted to assess the energy consumption of the entire complex. By identifying areas of high energy use or inefficiency, the complex can implement strategies for conservation, such as upgrading lighting systems, improving insulation, or optimizing heating and cooling practices.
- Performance Monitoring: Real-time data from renewable energy systems allows for constant monitoring of system performance. Software tools can analyze this data to predict energy generation patterns, prevent system failures, and ensure that power production aligns with the complex’s needs.
8.3.5. Technological Upgrades and Adaptation
Renewable energy technology is rapidly evolving, and it is essential to adapt to new innovations to maintain system efficiency. The technology used in small-scale power plants should be periodically updated to keep up with improvements in energy storage, generation, and distribution.
- Upgrading to Smart Grids: Residential complexes can consider upgrading their energy systems to integrate smart grids, which allow for better communication and management of energy usage.
- Integration of New Storage Solutions: As battery storage technology improves, residential complexes can adopt newer and more efficient storage solutions, such as solid-state batteries, to improve reliability and reduce energy costs.
8.4. Social and Environmental Impact (Continued)
8.4.4. Community Engagement and Education
Successful integration of small-scale power plants in residential complexes often requires strong community engagement and education. Residents need to understand how the system works and how they can benefit from it.
- Educational Programs: Hosting workshops, training sessions, or providing educational resources about renewable energy can foster community support and participation. Educating residents on how to optimize their energy usage can contribute to overall energy savings and efficiency.
- Community Feedback and Involvement: Actively involving residents in the decision-making process related to energy systems, such as choosing energy-efficient technologies or determining energy use policies, can ensure that the systems meet the specific needs of the community and promote a sense of ownership.
8.4.5. Contribution to Sustainable Urban Development
Incorporating small-scale power plants in residential complexes is a step towards achieving sustainable urban development. These systems align with broader sustainability goals, such as the United Nations’ Sustainable Development Goals (SDGs), including Goal 7: Affordable and Clean Energy, and Goal 11: Sustainable Cities and Communities.
- Urban Sustainability: Residential complexes with integrated renewable energy systems can serve as models for sustainable living in cities, showcasing how urban areas can reduce their environmental impact while maintaining a high standard of living.
- Inspiring Broader Adoption: By demonstrating the effectiveness of renewable energy systems in residential settings, these projects inspire broader adoption across the city or region. This creates a ripple effect, encouraging other buildings and communities to invest in renewable energy solutions.
8.4.6. Resilience to Energy Price Fluctuations
A key advantage of small-scale power plants is their ability to shield residential complexes from the volatility of energy prices. Energy prices, particularly for fossil fuels, can fluctuate dramatically due to geopolitical factors, natural disasters, or market changes.
- Stable Energy Prices: With renewable energy systems in place, residents can benefit from more predictable and stable energy costs. In some cases, the financial savings from local energy generation can help insulate the complex from rising utility costs.
- Long-Term Financial Benefits: Even though the initial investment may be high, over time, the financial benefits of reducing reliance on external energy sources will outweigh the costs, particularly as technology improves and operational costs decrease.
8.5. Conclusion
Small-scale power plants for residential complexes provide a comprehensive solution to energy challenges in urban areas. By utilizing renewable energy sources such as solar, wind, geothermal, and biomass, these systems can reduce energy costs, enhance energy security, and contribute to sustainable living. The integration of energy storage, smart grid technologies, and hybrid systems further improves reliability and efficiency, while community involvement and education ensure long-term success.
As technology evolves and urban demand for sustainable energy solutions grows, small-scale power plants in residential complexes will play a key role in shaping the future of energy in cities, helping to create resilient, sustainable, and environmentally responsible urban communities.
4o mini
Şunu dedin:
go on 2
ChatGPT:
8.6. Future Trends and Innovations in Small-Scale Power Plants for Residential Complexes
As the demand for clean energy continues to grow and technology evolves, several future trends and innovations in small-scale power plants for residential complexes are emerging. These advancements aim to make residential power systems more efficient, flexible, and integrated into smart city frameworks.
8.6.1. Smart Home Integration
In the coming years, small-scale power plants for residential complexes will increasingly integrate with smart home technologies to optimize energy use and improve overall energy efficiency. Smart homes equipped with advanced sensors, energy management systems, and appliances that communicate with the power system can help reduce energy waste and maximize savings.
- Energy Management Systems (EMS): EMS platforms will allow residents to monitor their energy consumption in real-time, adjust heating/cooling settings, and optimize the use of appliances to reduce energy costs.
- Automated Energy Shifting: Through smart technologies, residents will be able to automatically shift high-energy-consuming activities, such as washing clothes or running the dishwasher, to times when renewable energy generation is at its peak (e.g., during daylight hours for solar-powered systems).
8.6.2. Artificial Intelligence and Machine Learning
Artificial intelligence (AI) and machine learning (ML) will play an increasingly important role in managing and optimizing small-scale power plants for residential complexes. These technologies can enhance the performance of renewable energy systems, enabling them to become more adaptable to changing weather conditions and energy demands.
- Predictive Analytics: AI algorithms can predict energy demand patterns, renewable energy generation, and system performance. This allows the system to dynamically adjust energy flows and optimize the distribution of electricity across the complex.
- Autonomous Optimization: AI-powered systems can make real-time adjustments to energy storage, grid interaction, and consumption, ensuring that the power plant operates at maximum efficiency, with minimal intervention from operators.
8.6.3. Vehicle-to-Grid (V2G) Technology
With the growing adoption of electric vehicles (EVs) in residential communities, Vehicle-to-Grid (V2G) technology is expected to become a crucial component of small-scale power plants for residential complexes. V2G allows EVs to not only charge from the grid but also feed stored energy back into the grid or local energy system when needed.
- Energy Storage on Wheels: Electric vehicles can act as mobile energy storage units. During times of excess renewable energy generation, EVs can charge, and when there is high demand or limited generation, EVs can discharge energy back into the complex’s energy system.
- Grid Stability: V2G can contribute to grid stability by providing additional energy reserves during peak periods, reducing the overall strain on the grid and enhancing the resilience of the residential complex’s energy supply.
8.6.4. Energy Sharing Platforms
The future of small-scale power plants for residential complexes may involve peer-to-peer (P2P) energy sharing platforms. These systems allow residents to trade excess energy with one another, creating a localized energy marketplace within the complex.
- Blockchain-Based Energy Trading: Blockchain technology can be used to securely track and record energy transactions within the community, ensuring transparency and trust in the energy-sharing process. This could allow residents to sell surplus solar energy to neighbors who are unable to meet their energy needs.
- Local Energy Networks: Energy-sharing platforms can extend beyond individual complexes to create neighborhood-level energy networks, where several small-scale power plants are interconnected and can share energy to balance demand and supply efficiently.
8.6.5. Advanced Energy Storage Technologies
As energy storage systems continue to improve, residential complexes will benefit from more advanced and cost-effective options, enabling them to store greater amounts of energy for longer periods. This will be particularly important for areas where renewable energy generation fluctuates significantly.
- Solid-State Batteries: These batteries, which replace the liquid electrolyte in traditional lithium-ion batteries with a solid electrolyte, promise to be safer, more energy-dense, and longer-lasting. Solid-state batteries could significantly enhance the capacity of energy storage systems in residential complexes.
- Flow Batteries: Flow batteries, which store energy in liquid form and use chemical reactions to release it, are another promising storage technology for residential power systems. They offer the potential for longer discharge times and larger energy storage capacities, making them suitable for large-scale residential energy storage.
8.6.6. Community-Scale Renewable Energy Projects
Another emerging trend is the growth of community-scale renewable energy projects that can provide energy to multiple residential complexes or entire neighborhoods. These projects can involve large-scale solar farms, wind turbines, or geothermal installations that generate power for surrounding areas.
- Community Solar Power: Residents of smaller homes or those with unsuitable rooftops for solar installations can participate in community solar programs, where they can purchase or lease a share of a larger solar farm located nearby.
- Shared Wind and Geothermal Systems: In addition to solar, shared wind and geothermal systems can be implemented on a larger scale to power multiple buildings or entire neighborhoods, providing a steady and reliable source of renewable energy.
8.6.7. Sustainability Certifications and Green Building Standards
The demand for sustainable construction and energy-efficient residential buildings is growing. In the future, small-scale power plants for residential complexes will be integrated into buildings that meet green building standards, such as LEED (Leadership in Energy and Environmental Design) or BREEAM (Building Research Establishment Environmental Assessment Method) certifications.
- Green Building Standards: By adopting energy-efficient building designs, construction materials, and renewable energy technologies, residential complexes can achieve sustainability certifications, making them more attractive to environmentally conscious buyers and renters.
- Net Zero Energy Buildings: A key future goal is the design of net zero energy buildings, which produce as much energy as they consume over the course of a year. These buildings rely heavily on renewable energy systems and energy-efficient designs to meet their energy needs.
8.6.8. Distributed Energy Resources (DERs) and Energy Communities
As the energy landscape evolves, Distributed Energy Resources (DERs)—small-scale, decentralized energy systems—are expected to become more prevalent in residential complexes. These resources include not just renewable energy generation but also energy storage, electric vehicles, and flexible energy management systems.
- Energy Communities: A growing trend involves creating energy communities, where residents collectively own and manage renewable energy systems. These communities can have greater control over their energy production, consumption, and costs, ensuring long-term sustainability and resilience.
- Virtual Power Plants (VPPs): These systems aggregate the energy produced by multiple DERs to create a virtual power plant. In the future, small-scale power plants in residential complexes may be part of a broader VPP, participating in energy markets and offering services like frequency regulation or peak shaving.
8.7. Conclusion: The Future of Small-Scale Power Plants in Residential Complexes
The future of small-scale power plants for residential complexes is dynamic, with numerous technological, economic, and social developments on the horizon. As renewable energy systems evolve and new technologies like AI, V2G, and advanced energy storage emerge, residential complexes will become more self-sufficient, resilient, and sustainable.
By integrating renewable energy technologies with smart home systems, creating local energy markets, and utilizing cutting-edge energy storage solutions, small-scale power plants will not only help reduce energy costs but also contribute to the broader goals of carbon reduction and sustainable urban development.
As these systems become more sophisticated, they will play a critical role in transforming residential communities into sustainable energy hubs, capable of supporting the energy needs of the future while protecting the environment and improving the quality of life for residents.
8.8. Challenges and Solutions for Small-Scale Power Plants in Residential Complexes
While small-scale power plants offer numerous advantages, there are challenges associated with their implementation and operation in residential complexes. Understanding and addressing these challenges is crucial for ensuring the success and sustainability of these systems.
8.8.1. High Initial Capital Costs
One of the primary challenges of small-scale power plants is the high initial capital cost of installing renewable energy systems and the necessary infrastructure. Although the long-term savings and environmental benefits often outweigh the initial investment, the upfront costs can be a barrier for many residential complexes, especially those in lower-income areas.
- Solution: Governments and financial institutions can play a crucial role in providing incentives, such as tax credits, grants, or low-interest loans, to offset the initial investment. Furthermore, power purchase agreements (PPAs) or community-funded solar programs allow residents to share in the costs and benefits of renewable energy without having to make a large initial investment.
- Energy-as-a-Service: Emerging models like “Energy-as-a-Service” allow residents to pay for the energy they use, similar to their traditional utility bills, while the renewable energy systems and maintenance are handled by third-party service providers. This lowers the barrier to entry for complexes that cannot afford to purchase and install systems outright.
8.8.2. Technological Complexity and Integration
Integrating multiple renewable energy systems, energy storage, and smart technologies in a residential complex can be technologically complex. Ensuring these systems work seamlessly together requires significant planning, design expertise, and ongoing monitoring.
- Solution: Partnering with experienced energy solution providers who have expertise in integrating various renewable technologies is essential. Modular and standardized solutions can simplify installation and reduce the potential for technical issues.
- System Integration Software: Advanced software tools that manage and optimize the integration of energy sources, storage systems, and grid interaction can improve the efficiency and reliability of the power plant. These systems can help monitor energy generation and consumption in real-time and automatically adjust the flow of energy where needed.
8.8.3. Energy Storage Limitations
Despite advancements in battery technology, energy storage remains a challenge for small-scale power plants, especially in residential complexes that rely on renewable sources like solar and wind, which are intermittent by nature.
- Solution: To address storage limitations, hybrid energy storage solutions (such as combining battery storage with thermal storage or flywheels) can be used to store excess energy more effectively. Additionally, community-wide storage systems can help increase storage capacity, allowing energy to be shared more effectively among different units within the complex.
- Virtual Storage: With the advent of virtual storage and demand response programs, excess energy can be stored in the form of virtual credits that can be used when needed. This system allows for better flexibility in managing energy use across a community.
8.8.4. Regulatory and Policy Barriers
The regulatory environment for renewable energy can vary widely by region, creating challenges for the adoption of small-scale power plants in residential complexes. Issues such as grid access, interconnection standards, and permitting processes can delay or increase the cost of implementation.
- Solution: Advocating for policy reforms that support decentralized renewable energy systems is key. Streamlined permitting processes, net metering policies (which allow excess energy to be sold back to the grid), and standardized interconnection agreements can simplify the deployment of small-scale power plants.
- Incentive Programs: Governments can implement incentive programs that reduce bureaucratic barriers and provide clear guidelines for integrating small-scale power plants into the existing grid infrastructure.
8.8.5. Resident Behavior and Energy Conservation
While the technical infrastructure is crucial, the success of small-scale power plants also depends on how residents use the energy. Without proactive energy conservation efforts and community-wide participation, the potential benefits of renewable energy systems may be reduced.
- Solution: Educational programs and awareness campaigns can help residents understand the importance of energy conservation and how to use the power generated by the small-scale systems efficiently. Additionally, integrating smart meters and energy management tools can encourage residents to monitor their consumption patterns and reduce waste.
- Demand Response Programs: Implementing demand response programs, where residents are incentivized to reduce their energy consumption during peak periods, can help balance supply and demand, ensuring that renewable energy systems are used optimally.
8.8.6. Reliability and Maintenance
The reliability and ongoing maintenance of small-scale power plants are critical to their long-term success. If systems experience frequent downtime or require expensive repairs, residents may lose confidence in the technology and revert to traditional energy sources.
- Solution: Establishing maintenance contracts and ensuring regular system check-ups and monitoring can help prevent breakdowns. Additionally, adopting modular systems with easily replaceable parts can reduce maintenance costs and increase system reliability.
- Remote Monitoring: Advanced remote monitoring systems allow operators to identify potential issues before they escalate, ensuring that maintenance can be performed proactively rather than reactively.
8.8.7. Limited Space for Installation
In densely populated urban areas, residential complexes may have limited space for installing large-scale renewable energy systems, such as rooftop solar panels or wind turbines.
- Solution: Utilizing shared spaces (e.g., communal rooftops, parking lots, or adjacent land) for installing renewable energy infrastructure can help maximize available space. In some cases, building-integrated photovoltaics (BIPV), which integrate solar cells directly into building materials like windows or facades, can provide an effective solution in spaces with limited surface area.
- Vertical Wind Turbines: In areas where horizontal wind turbines may not be feasible due to space or structural limitations, vertical-axis wind turbines (VAWTs) can be installed on rooftops or in smaller spaces. VAWTs are more compact and can operate in turbulent wind conditions typical of urban environments.
8.9. Case Studies and Examples
8.9.1. The Bullitt Center, Seattle, USA
The Bullitt Center is a well-known example of a commercial building with a small-scale power plant that incorporates renewable energy systems. This building features a 57,000 square foot rooftop solar array that generates enough energy to meet the building’s needs, alongside a rainwater collection system and a geothermal heating and cooling system. The Bullitt Center is a prime example of how integrating multiple renewable energy technologies can provide a self-sustaining, net-zero energy building that serves as a model for urban development.
- Key Takeaways: The integration of solar, geothermal, and water systems demonstrates the potential for residential complexes to adopt similar models. It also shows how community engagement, robust planning, and collaboration with technology providers can overcome the challenges of small-scale power plant implementation.
8.9.2. Freiburg Vauban District, Germany
The Vauban District in Freiburg, Germany, is a sustainable urban neighborhood that features a mix of residential buildings powered by small-scale renewable energy systems. The neighborhood is a leader in energy efficiency, utilizing solar, wind, and biomass energy to power homes, along with advanced energy storage and management systems. This community has embraced the concept of a “low-carbon economy,” using renewable energy to power homes, offices, and public spaces.
- Key Takeaways: Vauban’s success lies in its holistic approach to sustainability, integrating renewable energy with energy-efficient design, community involvement, and active monitoring. This case study highlights how small-scale power plants can be integrated into entire communities, not just individual buildings.
8.10. Conclusion
Small-scale power plants for residential complexes offer a promising pathway toward achieving sustainable energy use, reducing carbon emissions, and creating more resilient urban communities. While there are challenges—ranging from high upfront costs to technological integration—innovative solutions and technological advancements are continually addressing these obstacles.
By embracing emerging technologies, fostering community participation, and leveraging supportive policies, residential complexes can become pioneers in renewable energy adoption. As these systems evolve and become more affordable, small-scale power plants will play an increasingly important role in shaping the future of urban energy, creating self-sufficient, sustainable, and energy-efficient living spaces.
9. Small-Scale Power Plant for Wood Processing Mills
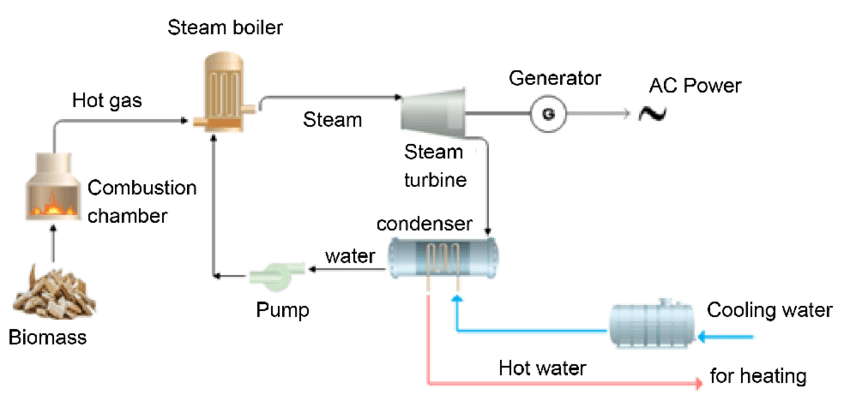
Wood processing mills, often integral to the forestry and paper industries, are key players in the manufacturing sector. These mills consume a significant amount of energy for various processes like drying, sawing, grinding, and waste disposal. Leveraging renewable energy through small-scale power plants presents a unique opportunity for these mills to reduce their energy costs, increase sustainability, and even become self-sufficient.
This section explores the potential for small-scale power plants in wood processing mills, highlighting the benefits, challenges, technologies involved, and examples of successful implementation.
9.1. Benefits of Small-Scale Power Plants for Wood Processing Mills
Small-scale power plants tailored to the needs of wood processing mills can offer several significant benefits:
9.1.1. Reduced Operational Costs
Wood processing mills are typically energy-intensive. By utilizing small-scale power plants, particularly biomass or combined heat and power (CHP) systems, mills can generate their own electricity and heat, leading to significant savings on utility bills.
9.1.2. Sustainable Waste Utilization
Wood processing mills produce a variety of byproducts, including sawdust, wood chips, bark, and other residues. These byproducts can be converted into renewable energy through technologies such as biomass gasification or combustion. By using waste material as fuel, mills can reduce the environmental impact of waste disposal while generating usable power.
9.1.3. Energy Security and Reliability
By generating their own energy, mills can achieve greater energy security and reduce dependence on external power providers. This is especially important in areas with unreliable grid connections or where energy prices are volatile.
9.1.4. Green Energy Certification and Market Appeal
Incorporating renewable energy through small-scale power plants can help wood processing mills obtain green certifications and sustainability labels, which can be used to appeal to environmentally conscious consumers and stakeholders. This can enhance the mill’s reputation in a competitive marketplace.
9.2. Suitable Technologies for Small-Scale Power Plants in Wood Processing Mills
Several technologies are particularly well-suited to wood processing mills, given the nature of the industry and the types of waste generated:
9.2.1. Biomass Power Generation
Biomass power generation is one of the most common and effective methods of utilizing wood waste to generate energy. Wood chips, sawdust, and bark can be combusted directly or converted into gas or liquid fuels for electricity production.
- Biomass Combustion: Involves the direct burning of wood byproducts to produce heat, which can be used to generate electricity through a steam turbine or an organic Rankine cycle (ORC) generator. This is a simple and well-established technology.
- Biomass Gasification: This process converts solid wood waste into a combustible gas, which can be burned in an internal combustion engine or gas turbine to produce electricity. Gasification systems are more efficient than direct combustion and can reduce emissions.
- Pelletized Biomass: Wood waste can be processed into pellets, which can be burned in specialized biomass power plants. This provides a more uniform and energy-dense fuel, making it ideal for mills with significant amounts of waste.
9.2.2. Combined Heat and Power (CHP) Systems
Combined Heat and Power (CHP) systems are particularly attractive for wood processing mills because they allow the simultaneous generation of both electricity and useful heat. This is particularly beneficial because many industrial processes in wood mills require heat (e.g., for drying wood), and CHP systems ensure that both energy needs are met efficiently.
- Cogeneration: Cogeneration systems recover heat from the combustion process and utilize it for space heating, drying processes, or even preheating water. This can improve the overall efficiency of the system and significantly lower operational costs.
- Organic Rankine Cycle (ORC): For mills that use lower temperature heat sources (e.g., wood waste), ORC technology is an effective solution for converting waste heat into electricity, improving overall energy efficiency.
9.2.3. Waste Heat Recovery
Wood processing mills often generate large amounts of waste heat during various production processes, such as drying and sawing. By incorporating waste heat recovery systems, mills can capture this heat and repurpose it for power generation or other heating applications.
- Heat Recovery Steam Generators (HRSG): These systems can capture heat from exhaust gases or other waste heat streams and convert it into steam, which can then be used to drive a turbine for electricity generation.
- Thermal Energy Storage: In some cases, thermal energy storage systems can store excess heat for later use, helping to balance energy supply and demand and improve overall system efficiency.
9.2.4. Solar and Wind Power
While biomass is the most obvious energy source for wood processing mills, solar and wind energy can also contribute to the power supply, especially in regions with abundant sunlight or wind resources.
- Solar Photovoltaic (PV): For mills with access to open land or roofs, solar PV systems can be installed to supplement biomass power. Solar power can help meet daytime energy demands and offset grid reliance during peak hours.
- Wind Power: Wind turbines can be used in areas with favorable wind conditions to generate additional power. Small-scale wind turbines can be integrated with other renewable energy technologies for a hybrid system.
9.3. Challenges of Implementing Small-Scale Power Plants in Wood Processing Mills
While the benefits of small-scale power plants are clear, there are several challenges that mills must address when implementing these systems:
9.3.1. Initial Capital Investment
The installation of small-scale power plants, especially biomass systems, can require a significant initial investment. This includes the costs of purchasing and installing boilers, turbines, generators, and other infrastructure.
- Solution: Securing financing through government incentives, subsidies, or partnerships with energy service providers can help offset the upfront costs. In some cases, power purchase agreements (PPAs) or energy-as-a-service models may help reduce the financial burden.
9.3.2. Feedstock Supply and Sustainability
For biomass systems to function effectively, a consistent supply of feedstock (wood waste) is essential. Wood processing mills must ensure that they have reliable access to enough byproducts to fuel the power generation system without compromising their primary production processes.
- Solution: A careful analysis of waste generation and potential feedstock suppliers is essential. Mills can also explore partnerships with nearby industries to source additional biomass, creating a local bioenergy supply chain that benefits multiple sectors.
9.3.3. Technology Integration and Maintenance
Integrating new energy generation technologies into existing mill operations can be challenging. Additionally, regular maintenance of power generation equipment is critical to avoid downtime.
- Solution: Mills can partner with experienced system integrators who specialize in industrial energy systems. Regular predictive maintenance and remote monitoring technologies can help identify potential issues before they cause major disruptions.
9.3.4. Environmental Concerns
While biomass is considered renewable, its use must be managed sustainably. Improper combustion or overharvesting of wood resources can result in emissions or environmental degradation.
- Solution: To mitigate environmental impacts, mills should adhere to sustainable sourcing practices for biomass and utilize clean combustion technologies to minimize emissions. Adopting carbon capture and storage (CCS) technologies or switching to lower-carbon biomass sources can further reduce environmental impact.
9.4. Case Studies and Examples
9.4.1. The Green Energy Plant at The Kymenlaakso Wood Processing Mill, Finland
The Kymenlaakso wood processing mill in Finland integrated a small-scale biomass power plant into its operations. The system utilizes sawdust, bark, and wood chips as feedstock to produce both electricity and heat for the mill’s operations. The biomass plant generates about 60% of the mill’s energy needs, reducing reliance on external energy sources and contributing to the mill’s sustainability efforts.
- Key Takeaways: This project demonstrates how wood processing mills can reduce their carbon footprint and energy costs by utilizing waste products for energy generation. Additionally, the integration of heat and power generation in a single system reduces operational complexity and improves energy efficiency.
9.4.2. The Sappi Lanaken Paper Mill, Belgium
The Sappi Lanaken paper mill in Belgium implemented a combined heat and power (CHP) biomass plant that uses bark and other wood residues as fuel. The system produces both electricity and thermal energy, significantly reducing the mill’s dependence on external energy sources. By utilizing local biomass resources, the plant also supports the local economy.
- Key Takeaways: This example highlights the importance of utilizing local feedstock and integrating power generation into the mill’s overall energy infrastructure. The project has also enhanced the mill’s sustainability credentials and improved its competitiveness in the global market.
9.5. Conclusion
Small-scale power plants offer significant potential for wood processing mills, providing an opportunity to reduce energy costs, improve sustainability, and create a more resilient energy infrastructure. Biomass power generation, CHP systems, and waste heat recovery technologies are particularly well-suited to these mills, given the abundant supply of wood waste and the energy needs of the industry.
While challenges such as high initial investment and feedstock management exist, solutions such as government incentives, strategic partnerships, and innovative energy models can help overcome these barriers. By adopting small-scale power plants, wood processing mills can contribute to a greener future while enhancing their operational efficiency and energy independence.
9.6. Economic and Environmental Impact of Small-Scale Power Plants in Wood Processing Mills
When considering the implementation of small-scale power plants, wood processing mills not only look at immediate energy benefits but also evaluate the long-term economic and environmental impacts. These impacts can be significant in terms of cost savings, revenue generation, and sustainability.
9.6.1. Economic Impact
The adoption of small-scale power plants can have a positive effect on the bottom line of wood processing mills. This is primarily through energy cost reductions, the potential for income generation from excess power, and greater operational efficiency.
- Energy Cost Reductions: By generating their own electricity and heat, mills can significantly reduce their dependence on grid electricity, especially in regions with high energy prices. The payback period for these systems can be as short as 3-5 years, depending on the scale and efficiency of the power plant.
- Revenue Generation: Mills can generate additional revenue by selling excess power back to the grid. Many countries and regions have feed-in tariff or net metering policies that incentivize businesses to feed surplus energy into the national grid. This not only provides financial returns but also offsets the cost of running the plant.
- Operational Efficiency: By integrating energy generation with industrial processes, mills can improve overall operational efficiency. Biomass power systems, for instance, can be combined with drying or heating operations, reducing the need for separate fuel sources and increasing the efficiency of both electricity generation and industrial production.
- Energy Independence: Small-scale power plants can provide energy security, allowing mills to operate without interruptions from external power grid failures or price fluctuations. This independence can protect the mill from market volatility, which is especially crucial in energy-dependent industries like wood processing.
9.6.2. Environmental Impact
From an environmental perspective, small-scale power plants in wood processing mills can significantly reduce carbon footprints, support sustainable resource management, and promote cleaner air and water.
- Carbon Emission Reduction: Traditional fossil fuel-based power generation contributes significantly to greenhouse gas (GHG) emissions. By using biomass from wood waste, mills can lower their carbon emissions. Biomass is considered carbon-neutral, as the CO2 released during combustion is balanced by the CO2 absorbed by the plants during their growth phase.
- Waste Reduction: Wood mills typically generate a substantial amount of wood waste. If not properly managed, this waste can end up in landfills or be incinerated, leading to air pollution and lost resources. By converting wood waste into energy, small-scale power plants help reduce landfill waste and lower environmental pollution, as the waste is used productively to generate energy.
- Sustainable Resource Management: The wood industry can promote the sustainable use of forest resources by ensuring that only waste byproducts are used for energy generation. Moreover, the use of waste products reduces the pressure to harvest additional trees, supporting the conservation of forests and biodiversity.
- Water Use Efficiency: Biomass power plants generally use less water compared to conventional power generation methods, such as coal or nuclear plants, which require large amounts of water for cooling. This can be beneficial for mills located in water-scarce regions, reducing their overall water consumption and environmental footprint.
9.7. Integration with the Circular Economy
Small-scale power plants in wood processing mills are inherently aligned with the principles of the circular economy. This concept focuses on reducing waste and making the most of resources by ensuring that products, materials, and energy are reused, refurbished, or recycled.
- Waste as a Resource: Wood mills can generate a range of byproducts—such as sawdust, wood chips, bark, and other residues—that can be converted into energy. Rather than these materials being discarded or incinerated, they can be repurposed as valuable resources. This not only reduces the environmental burden of waste disposal but also creates a closed-loop system where energy is generated from resources already present in the mill’s processes.
- Energy Efficiency: Incorporating energy-efficient technologies into the milling process reduces waste and lowers energy consumption. By optimizing energy use and utilizing waste materials to generate power, mills can decrease their reliance on external energy sources and further close the loop on resource utilization.
- Product Lifecycle Extension: By reducing waste and utilizing renewable energy, wood processing mills can extend the lifecycle of their products. For instance, utilizing waste heat from power generation for drying processes reduces the need for additional fuel inputs and lowers overall energy consumption, resulting in lower costs and greater efficiency over the long term.
9.8. Future Trends and Opportunities
As the demand for sustainable products and green energy continues to grow, there are emerging trends that could further enhance the effectiveness of small-scale power plants in wood processing mills.
9.8.1. Technological Advancements in Biomass Conversion
The technology behind biomass conversion is continually advancing, with improvements in efficiency, sustainability, and automation. Innovations such as biomass gasification and pyrolysis can help increase energy output while reducing emissions and waste. Additionally, biofuels derived from wood byproducts may provide alternative sources of energy, further diversifying the power generation mix.
- Next-Generation Biomass Boilers: Newer, more efficient biomass boilers are emerging that can handle a wider variety of feedstocks, reducing the need for specialized fuel processing. These boilers can improve combustion efficiency and lower emissions.
- Advanced Gasification Technology: Gasification offers higher efficiency than traditional biomass combustion. The development of advanced gasifiers that can handle different types of biomass and produce clean energy will enhance the viability of small-scale power plants in wood processing mills.
9.8.2. Hybrid Power Systems
In the future, hybrid power systems combining multiple renewable energy sources will become increasingly popular. For example, biomass systems can be integrated with solar or wind power to provide a more reliable and diverse energy supply, particularly in regions with variable renewable energy resources. These hybrid systems can ensure that wood processing mills maintain a steady and balanced energy supply regardless of weather conditions.
9.8.3. Digitalization and Smart Grids
The rise of smart grids and digital technologies will enable more efficient and flexible management of energy within wood processing mills. By using advanced sensors, IoT technology, and data analytics, mills can optimize their energy consumption, predict energy needs, and improve system maintenance. Additionally, smart grid integration can enable mills to feed surplus energy into the grid more efficiently, contributing to a decentralized and resilient energy system.
- Smart Metering and Energy Management: Smart meters and energy management systems can help mills monitor their energy use in real-time, making it easier to identify inefficiencies and adjust consumption patterns.
9.8.4. Enhanced Collaboration and Partnerships
To maximize the benefits of small-scale power plants, wood processing mills may seek partnerships with local energy providers, government bodies, and technology developers. By collaborating with stakeholders across the energy value chain, mills can access additional expertise, financing options, and incentives that make implementing renewable energy solutions more affordable and scalable.
9.9. Conclusion
Small-scale power plants for wood processing mills present a unique opportunity to address both economic and environmental challenges faced by the industry. By utilizing wood waste to generate energy, these systems can significantly reduce operational costs, support sustainability, and promote energy independence. Biomass power, combined heat and power (CHP) systems, and waste heat recovery technologies are particularly suited to the needs of wood mills.
While challenges exist in terms of initial capital investment and system integration, the long-term benefits—including cost savings, waste reduction, and improved sustainability—are compelling. By adopting small-scale power generation systems, wood processing mills can become leaders in the transition to a more sustainable, circular economy while enhancing their competitiveness in a rapidly evolving market.
9.10. Policy and Regulatory Support for Small-Scale Power Plants in Wood Processing Mills
Governments and regulatory bodies play a critical role in facilitating the transition of wood processing mills toward renewable energy solutions, including small-scale power plants. By providing incentives, tax credits, and regulatory frameworks that support renewable energy adoption, they can help overcome some of the financial and logistical barriers that mills face.
9.10.1. Government Incentives and Subsidies
Many countries offer financial incentives to encourage industries, including wood processing mills, to adopt renewable energy technologies. These incentives can significantly reduce the initial capital cost of installing small-scale power plants.
- Tax Credits: Tax credits such as the Investment Tax Credit (ITC) or Production Tax Credit (PTC), available in some countries, can offset the costs of installing renewable energy equipment, including biomass power systems.
- Grants and Subsidies: Some governments provide grants or low-interest loans for the installation of renewable energy systems, making the transition to small-scale power plants more affordable for mills. These programs are often designed to support the green economy and reduce industrial carbon footprints.
- Feed-in Tariffs and Power Purchase Agreements (PPAs): Feed-in tariffs guarantee a fixed payment for energy produced from renewable sources, providing a predictable revenue stream. PPAs between mills and utilities can help ensure the sale of excess power generated by the biomass or other renewable systems at favorable rates.
- Green Certificates and Carbon Credits: In many regions, renewable energy projects can earn green certificates or carbon credits, which can be sold to other industries looking to meet sustainability goals. These programs incentivize mills to invest in renewable energy and provide a return on investment over time.
9.10.2. Policy Support for Biomass and Renewable Energy
Effective policy frameworks are essential to ensure that small-scale power plants in wood processing mills can operate in a conducive environment. This includes setting clear standards for biomass power generation and supporting innovation in renewable energy technologies.
- Sustainability Standards for Biomass: Governments can set sustainability standards for biomass fuels, ensuring that wood waste used in power plants is sourced sustainably. This could include rules for ensuring that biomass feedstock is sourced from certified sustainable forests or that waste material does not contribute to deforestation.
- Energy Efficiency Standards: Setting energy efficiency standards for industrial processes, including wood processing mills, can help guide the implementation of small-scale power plants. By mandating efficiency improvements, governments can encourage the adoption of cutting-edge technologies like biomass gasification and combined heat and power (CHP) systems.
- Grid Access and Interconnection Policies: Many small-scale renewable energy systems, including biomass power plants, generate surplus electricity that can be fed back into the national grid. However, interconnection policies and grid access for small generators can be complex. Governments must streamline these processes to make it easier for mills to sell excess energy and participate in grid systems.
9.10.3. Regulatory Compliance and Environmental Standards
While the regulatory landscape can vary widely from region to region, there are typically several key environmental standards and regulations that small-scale power plants in wood processing mills must comply with. These standards often address issues such as air quality, emissions control, and land use.
- Emissions Standards: Biomass power plants must meet air quality standards, particularly regarding particulate matter (PM), nitrogen oxides (NOx), and carbon emissions. Advanced flue gas treatment and scrubbing technologies can help biomass plants comply with stringent emissions regulations, ensuring that they operate in an environmentally responsible manner.
- Waste Management Regulations: In addition to energy generation, wood processing mills produce waste, which can contribute to environmental issues such as water pollution or land degradation. Waste management regulations ensure that byproducts like wood chips, sawdust, and bark are disposed of or utilized in an environmentally sustainable manner.
- Land Use Permitting: In some cases, regulatory approval may be required for land use, particularly if biomass feedstock needs to be sourced from specific areas. Clear policies regarding land use can ensure that the expansion of renewable energy infrastructure does not result in environmental degradation or conflict with local communities.
9.11. Collaboration with Industry Associations and Research Institutions
To navigate the technical, financial, and regulatory challenges of implementing small-scale power plants, wood processing mills can collaborate with industry associations and research institutions. These partnerships can provide access to valuable knowledge, networks, and resources that support the successful integration of renewable energy technologies.
9.11.1. Industry Associations
Many industry associations, such as the Biomass Power Association, American Forest & Paper Association (AF&PA), and European Biomass Association (AEBIOM), advocate for policies and provide resources to support the adoption of renewable energy in wood processing mills. These organizations often have access to government lobbying efforts, market insights, and technical resources that mills can leverage to facilitate their transition to renewable energy.
- Networking Opportunities: Industry associations provide opportunities for wood processing mills to collaborate with other businesses, experts, and suppliers in the renewable energy space, helping them access solutions and innovations that can reduce costs and improve efficiency.
- Training and Certification Programs: Many associations offer training programs and certifications that help mills understand and comply with renewable energy standards and regulations. These programs can be especially useful for mill employees involved in the operation and maintenance of biomass power plants.
9.11.2. Research Institutions and Universities
Research institutions and universities play a key role in advancing biomass power generation technologies and identifying new opportunities for energy production from wood waste. Collaborating with these institutions allows mills to stay on the cutting edge of renewable energy innovations.
- R&D in Biomass Conversion: Universities and research centers are often at the forefront of biomass conversion technologies, developing new methods to improve the efficiency and environmental sustainability of biomass energy generation. Wood processing mills can partner with these institutions to pilot new technologies and incorporate them into their operations.
- Innovation in Energy Systems: Research on smart grid technology, energy storage solutions, and hybrid renewable systems can help wood mills improve the performance and reliability of their small-scale power plants. Collaborative efforts with research institutions can also help identify new feedstock materials or waste products that can be converted into energy.
- Public-Private Partnerships: Governments and private sector companies often collaborate on research projects that aim to improve the commercialization of renewable energy technologies. Through public-private partnerships, mills can access funding, expertise, and resources that may be unavailable through traditional channels.
9.12. Final Thoughts on Small-Scale Power Plants for Wood Processing Mills
The integration of small-scale power plants into wood processing mills offers a promising path toward reducing energy costs, enhancing sustainability, and boosting economic competitiveness. Biomass power generation, combined heat and power (CHP) systems, and waste heat recovery technologies provide innovative solutions to the energy challenges of this sector, especially by leveraging the waste products generated during processing.
The economic, environmental, and technological benefits are substantial, and as new technologies and supportive policies continue to evolve, wood processing mills will be better equipped to transition toward renewable energy sources. By collaborating with industry stakeholders, securing financial incentives, and adhering to environmental regulations, mills can successfully implement small-scale power plants that contribute to both their long-term success and the global transition to a low-carbon economy.
In summary, small-scale power plants not only help wood processing mills meet their energy needs but also enable them to play a critical role in the circular economy by turning waste into energy, reducing reliance on fossil fuels, and creating new opportunities for sustainability and innovation.
9.13. Case Studies of Successful Small-Scale Power Plants in Wood Processing Mills
To further illustrate the potential of small-scale power plants in wood processing mills, it is helpful to examine a few successful case studies where mills have integrated renewable energy solutions, particularly biomass-based systems. These examples highlight how the combination of technology, policy, and innovation can lead to positive outcomes for both the environment and the bottom line.
9.13.1. Case Study 1: Biomass Power Plant at a European Wood Processing Mill
Location: Finland
Mill Type: Sawmill and wood pellet manufacturing
Energy System: Biomass CHP system
A large sawmill and wood pellet manufacturing facility in Finland decided to implement a small-scale biomass combined heat and power (CHP) system to address rising energy costs and reduce its environmental impact. The mill primarily uses sawdust, wood chips, and bark from the production process as feedstock for the power plant.
- Energy Production: The biomass CHP system produces both heat and electricity, providing the mill with the energy required for its processing operations and allowing it to sell surplus electricity back to the national grid. The system has reduced the mill’s reliance on external energy sources, which has helped to stabilize energy costs and ensure energy security.
- Environmental Impact: The mill’s switch to a biomass-based power system has significantly reduced its carbon emissions by using wood waste that would otherwise be discarded or incinerated. It has helped to meet local emissions reduction targets while contributing to the sustainable management of forest resources.
- Economic Impact: The investment in the biomass CHP system paid off within five years, with the mill experiencing a reduction in energy costs of more than 40%. The sale of surplus electricity has also contributed to additional revenue streams. Additionally, the mill has enhanced its market competitiveness by positioning itself as an environmentally conscious and sustainable business.
9.13.2. Case Study 2: Waste-to-Energy Power Plant in a North American Wood Mill
Location: United States
Mill Type: Lumber mill
Energy System: Biomass gasification system
A lumber mill located in the southeastern United States faced significant challenges related to high energy costs and environmental compliance. After analyzing their energy usage and waste generation, the mill decided to install a biomass gasification system powered by wood waste, including sawdust and wood chips.
- Energy Production: The gasification system produces clean syngas, which is used to generate electricity and heat. This system enables the mill to meet its energy needs without relying on the local grid, providing both cost savings and energy independence. The process also generates heat that can be utilized for kiln drying, reducing reliance on external natural gas supplies.
- Environmental Impact: By utilizing wood waste to generate energy, the mill has significantly reduced its environmental footprint. The gasification system emits lower levels of pollutants compared to traditional biomass combustion, making it more environmentally friendly. The mill has reduced its greenhouse gas emissions by over 30% since the implementation of the system.
- Economic Impact: The mill’s biomass gasification system has been instrumental in reducing fuel costs. In the first year of operation, the mill reported a 30% reduction in energy costs and achieved a positive return on investment within four years. Additionally, the mill can now take advantage of local incentives and grants available for renewable energy projects, helping offset installation costs.
9.13.3. Case Study 3: Hybrid Renewable Energy System at a Wood Processing Mill in Brazil
Location: Brazil
Mill Type: Plywood mill
Energy System: Hybrid solar-biomass system
A plywood mill located in Brazil, which relies heavily on electricity for its machinery and heating processes, faced high operational costs due to fluctuating energy prices. The mill decided to integrate a hybrid renewable energy system consisting of solar panels and a biomass boiler.
- Energy Production: The solar panels help offset a portion of the mill’s electricity demand during the day, while the biomass boiler provides heat and electricity during peak production times. This hybrid system enables the mill to diversify its energy sources, providing both stability and redundancy in energy supply.
- Environmental Impact: The adoption of renewable energy reduces the mill’s reliance on fossil fuels, supporting Brazil’s efforts to meet its carbon reduction targets. The mill has significantly lowered its carbon footprint and is able to sell renewable energy certificates for the electricity generated by the biomass system.
- Economic Impact: The hybrid system has reduced the mill’s energy costs by approximately 35%, with the combined benefits of solar and biomass energy improving the mill’s overall financial performance. The system’s integration has also contributed to improved energy efficiency and allowed the mill to continue its operations without disruptions from energy shortages or grid failures.
9.13.4. Case Study 4: Biomass Power System in a Canadian Wood Processing Facility
Location: Canada
Mill Type: Sawmill
Energy System: Biomass heating and power generation system
A Canadian sawmill located in a remote region with limited access to the national grid decided to build its own small-scale biomass power plant to meet its energy needs. The mill primarily uses wood residues, including sawdust, bark, and wood chips, to generate electricity and heat.
- Energy Production: The biomass power system generates electricity through the combustion of wood residues, which is used to power the mill’s saws, dryers, and other equipment. The system also produces heat, which is used for space heating and drying processes. This combination of electricity and heat production through a combined heat and power (CHP) system ensures that the mill can maintain high efficiency and productivity.
- Environmental Impact: The biomass plant has enabled the mill to significantly reduce its reliance on fossil fuels, leading to a reduction in carbon emissions and other pollutants. The use of locally sourced wood residues has also minimized transportation emissions associated with the delivery of fuel, enhancing the mill’s sustainability.
- Economic Impact: By reducing energy costs, the mill has improved its profitability and achieved energy independence, which is especially valuable in a remote location where energy supply is unreliable. In addition, the mill receives financial benefits through carbon credits and other green incentives that help offset the initial capital costs of the biomass system.
9.14. Lessons Learned from Case Studies
These case studies offer several valuable lessons for wood processing mills considering the implementation of small-scale power plants:
- Waste-to-Energy is a Win-Win: The use of wood waste and residues for energy generation not only reduces the mill’s environmental impact but also provides a valuable resource for energy production. Mills can achieve both cost savings and sustainability by converting their byproducts into usable energy.
- Hybrid Systems Can Enhance Reliability: Integrating multiple renewable energy sources, such as combining solar and biomass power, can enhance system reliability and energy security. Hybrid systems can provide consistent power generation even in areas with variable renewable energy conditions.
- Financial Incentives Are Key: Government incentives, such as tax credits, subsidies, and feed-in tariffs, can significantly reduce the financial burden of installing renewable energy systems. Mills should take full advantage of these incentives to improve the financial viability of their projects.
- Collaboration with Experts is Crucial: Partnering with technology providers, engineering firms, and research institutions can help ensure the successful design, installation, and operation of small-scale power plants. Expertise in biomass technologies and energy systems is vital to overcoming technical challenges and ensuring optimal system performance.
- Long-Term Investment: While the upfront costs of installing small-scale power plants can be high, the long-term savings and environmental benefits often outweigh the initial investment. Mills should consider the return on investment (ROI) and payback period when evaluating the feasibility of these systems.
9.15. Conclusion
Small-scale power plants present a tremendous opportunity for wood processing mills to improve energy efficiency, reduce environmental impacts, and achieve long-term economic benefits. Biomass power systems, including CHP, gasification, and hybrid energy systems, are particularly well-suited to the needs of the industry, allowing mills to use their byproducts for energy generation while contributing to sustainability goals.
As technological advancements continue and governments provide more support through policies and incentives, the future for small-scale power plants in wood processing mills looks bright. By adopting these systems, mills not only enhance their financial stability but also become key players in the transition toward a more sustainable and circular economy.
9.16. Small Scale Power Plant for Renewable Energy Farms
Renewable energy farms, which primarily focus on producing energy through sustainable sources like wind, solar, or biomass, can significantly benefit from integrating small-scale power plants into their operations. These farms can take advantage of both the primary renewable energy generation technologies and complementary small-scale power systems to increase efficiency, lower costs, and reduce their environmental footprint.
9.16.1. Types of Renewable Energy Farms and Potential Integration of Small-Scale Power Plants
- Solar Farms
- Solar PV Systems: Solar energy is one of the most widely deployed renewable energy sources, especially in sunny regions. Solar farms generate electricity by converting sunlight into electricity using photovoltaic (PV) panels.
- Energy Storage: Integrating energy storage systems (like batteries) with small-scale power plants can help solar farms store excess energy produced during the day for use at night or during cloudy days. This helps ensure a constant supply of electricity.
- Wind Farms
- Small Wind Turbines: Wind farms use large turbines to generate electricity from wind. However, small-scale wind turbines can be added alongside larger installations to provide supplementary power in areas with lower wind speeds or to serve off-grid areas.
- Microgrid Integration: Wind farms can also integrate small-scale power plants into microgrids to provide localized energy solutions to nearby communities or agricultural operations.
- Biomass Farms
- Biomass Power Generation: Biomass farms use organic materials, such as agricultural residues, wood, or animal waste, to generate energy. Small-scale biomass power plants can provide localized power for farms or nearby rural communities while reducing reliance on fossil fuels.
- Combined Heat and Power (CHP): A biomass CHP system can be integrated into biomass farms to produce both electricity and thermal energy. This is particularly useful for agricultural operations that require heating for greenhouses, drying crops, or other processes.
- Hydropower Farms
- Micro-Hydropower Systems: In areas with small rivers or streams, small-scale micro-hydropower plants can be integrated into renewable energy farms to provide a consistent and reliable source of energy.
- Energy Storage and Grid Connection: Hydropower farms can incorporate small-scale power plants to store energy during periods of high flow and sell surplus electricity to the grid.
9.16.2. Benefits of Small-Scale Power Plants for Renewable Energy Farms
- Enhanced Energy Security
- By integrating small-scale power plants, renewable energy farms can ensure greater energy independence. These systems help diversify the energy sources, making the farm less dependent on any one technology or external energy providers.
- Improved Efficiency
- Small-scale power plants can be optimized to generate energy from a variety of sources, such as biomass waste, solar energy, or wind energy. This flexibility improves overall farm efficiency and ensures that energy is produced at optimal times.
- Reduced Operational Costs
- By supplementing their energy production, renewable energy farms can reduce their operating costs. This is particularly beneficial for operations that consume a lot of energy, such as irrigation systems or processing facilities. Over time, these systems also provide significant savings through reduced grid reliance.
- Environmental Sustainability
- Small-scale power plants help reduce the carbon footprint of renewable energy farms by utilizing sustainable technologies and waste materials, such as agricultural residues, for energy generation. These systems support a circular economy model by minimizing waste and using it as a resource.
- Grid Stability and Reliability
- Small-scale power plants can be integrated into microgrids, providing more localized and decentralized energy solutions. These systems increase the resilience of the farm’s energy infrastructure, especially in remote areas where grid connection may be unreliable or expensive.
- Grid Support and Revenue Generation
- Renewable energy farms with small-scale power plants can generate excess electricity during peak production times and sell it back to the grid. This not only provides additional revenue but also helps stabilize the local grid by providing renewable energy during periods of high demand.
9.16.3. Technological Considerations for Integrating Small-Scale Power Plants
- Hybrid Energy Systems
- Many renewable energy farms benefit from hybrid systems that combine different renewable technologies, such as solar, wind, and biomass. Small-scale power plants can complement the primary energy production systems by filling in the gaps during periods of low production, ensuring a reliable and steady energy supply.
- Energy Storage: For hybrid systems, integrating energy storage solutions such as batteries or pumped hydro storage is essential. This ensures that any surplus energy generated during peak times can be stored for use when generation is low.
- Energy Management Systems (EMS)
- Implementing an Energy Management System (EMS) is crucial for optimizing the integration of small-scale power plants. EMS software can monitor energy production, consumption, and storage, ensuring that power is distributed efficiently and that systems are operating optimally.
- Smart Grids and Digital Monitoring: Integrating smart grid technology allows renewable energy farms to better manage the flow of electricity between small-scale power plants, storage systems, and the grid.
- Grid Interconnection and Backup Systems
- Depending on the location and type of renewable energy farm, small-scale power plants must be capable of either grid-connected operation or work as stand-alone systems in off-grid settings. Backup power solutions are also important to ensure continuity in energy supply during grid outages.
9.16.4. Policy and Regulatory Support for Renewable Energy Farms
Government policies and regulatory frameworks play a critical role in the development of small-scale power plants on renewable energy farms. Several forms of support can incentivize renewable energy farms to invest in small-scale power systems:
- Subsidies and Grants
- Many countries provide subsidies or grants to renewable energy farms for the installation of small-scale power plants. These programs help reduce the upfront capital costs and make the projects more financially viable.
- Feed-in Tariffs (FiTs) and Power Purchase Agreements (PPAs)
- Feed-in tariffs or PPAs provide renewable energy farms with a guaranteed price for the electricity generated from small-scale power plants. This ensures that farms have a stable revenue stream and helps offset the costs of installation.
- Carbon Credit Programs
- Renewable energy farms can benefit from carbon credit programs that reward the reduction of greenhouse gas emissions. Small-scale power plants, particularly those utilizing biomass or waste-to-energy technologies, can earn carbon credits that can be sold or used to offset emissions elsewhere.
- Tax Incentives and Rebates
- Investment Tax Credits (ITC) and other tax rebates for renewable energy projects can help renewable energy farms reduce the financial burden of installing small-scale power plants. These incentives are often used to support the adoption of energy-efficient technologies and renewable energy systems.
- Environmental Regulations and Certifications
- Governments may set regulatory requirements for renewable energy farms to meet certain environmental standards, such as sustainable sourcing of biomass feedstock or compliance with emissions reduction targets. These regulations help ensure that the energy produced by small-scale power plants is both sustainable and environmentally responsible.
9.16.5. Challenges in Integrating Small-Scale Power Plants
- High Initial Investment
- The installation of small-scale power plants requires significant upfront capital investment, especially for technologies like biomass gasifiers, solar panels, or wind turbines. While long-term savings and incentives can offset these costs, securing financing can be a barrier for some renewable energy farms.
- Maintenance and Operational Complexity
- Operating a small-scale power plant requires ongoing maintenance and skilled personnel to ensure efficient operation. Renewable energy farms need to have the right expertise or partner with service providers to ensure the systems are functioning optimally.
- Regulatory Hurdles
- Navigating the complex regulatory landscape for small-scale power plants, including compliance with environmental standards and interconnection requirements, can be time-consuming and require expertise. Farms must ensure that they adhere to local laws and obtain the necessary permits before implementing power systems.
- Technology Limitations
- The efficiency and performance of small-scale power plants can be affected by local environmental conditions, such as inconsistent solar or wind availability. Renewable energy farms must carefully assess these factors before deciding on the appropriate small-scale power technologies to implement.
9.16.6. Case Studies of Small-Scale Power Plants in Renewable Energy Farms
- Case Study 1: Solar and Biomass Hybrid System in an Agricultural Energy Farm
- Location: India
- Farm Type: Agricultural energy farm
- Power System: Hybrid solar and biomass system
- An agricultural energy farm in India integrated a hybrid solar and biomass power system to supply energy to its operations. The farm used solar panels to provide daytime electricity and a biomass boiler to generate heat and power from agricultural residues. This combination reduced reliance on grid electricity and enhanced farm productivity by providing consistent power throughout the year.
- Case Study 2: Wind and Solar Hybrid Power System for a U.S. Renewable Energy Farm
- Location: United States
- Farm Type: Renewable energy farm (solar and wind)
- Power System: Wind and solar hybrid system
- A U.S. renewable energy farm combined both wind turbines and solar panels to generate electricity. Small-scale power plants were integrated into the farm’s operations to provide backup energy during periods of low wind or solar production. This hybrid system enhanced grid stability and allowed the farm to sell excess power back to the grid, providing additional revenue.
9.17. Conclusion
The integration of small-scale power plants into renewable energy farms is a promising strategy for improving energy production, reducing costs, and increasing sustainability. Whether through solar, wind, biomass, or hydropower, small-scale power plants enhance the energy resilience of renewable farms, ensure energy security, and provide significant financial and environmental benefits.
The successful integration of small-scale power systems requires careful consideration of technology choices, regulatory frameworks, and financial planning. However, with the right mix of government incentives, technological solutions, and strategic planning, renewable energy farms can significantly benefit from these power systems, contributing to a more sustainable and efficient energy future.
9.18. Advantages of Small-Scale Power Plants for Renewable Energy Farms
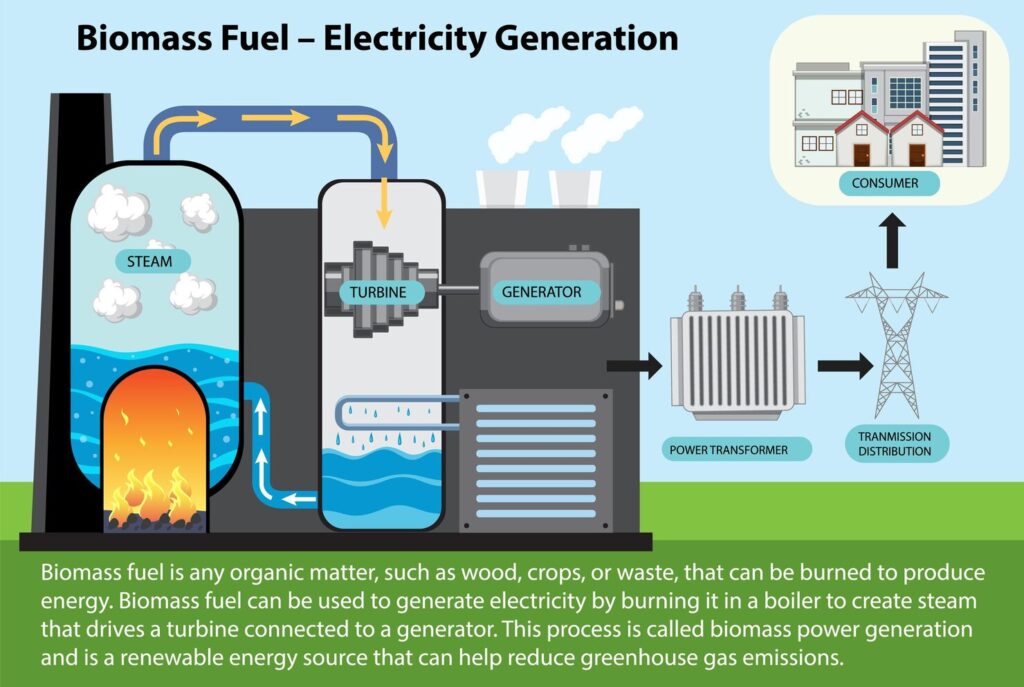
As renewable energy farms evolve to meet increasing demand for clean energy, small-scale power plants offer numerous benefits that enhance their operations, energy resilience, and financial viability. These plants complement larger renewable systems like wind and solar, providing reliable energy while promoting environmental sustainability.
9.18.1. Increased Energy Efficiency
Small-scale power plants help renewable energy farms optimize energy usage by utilizing local resources such as biomass (from agricultural or forestry waste), solar, or wind to produce power. By tapping into waste products or otherwise underutilized resources, these systems enhance overall energy efficiency. Additionally, smaller-scale generation can be designed to meet specific operational needs, providing greater flexibility.
- Example: A farm using biomass waste from its crops or livestock to power a biomass cogeneration unit can significantly reduce fuel costs, while simultaneously reducing waste.
9.18.2. Flexibility in Power Generation
Renewable energy farms often experience fluctuations in power generation due to the intermittent nature of solar and wind energy. Small-scale power plants can help address these fluctuations by generating additional power from other renewable sources, such as biomass or even hydropower in some cases. This flexibility ensures a steady and reliable energy supply, which is essential for farm operations that rely on consistent energy input.
- Example: During cloudy periods, a small biomass boiler can provide supplemental heat and electricity for the farm’s operations, ensuring uninterrupted production.
9.18.3. Contribution to Decarbonization
Integrating small-scale power plants into renewable energy farms helps to accelerate decarbonization efforts. By utilizing renewable resources for energy generation, small-scale power plants produce little to no carbon emissions, thus reducing the farm’s overall carbon footprint. Biomass systems, in particular, offer the opportunity to utilize agricultural and forestry residues, turning waste into a valuable resource, rather than allowing it to decompose and release greenhouse gases into the atmosphere.
- Example: By installing a small-scale biomass gasifier, a farm can generate power from waste materials that would otherwise be burned or left to decompose, which helps in mitigating methane emissions.
9.18.4. Diversification of Revenue Streams
Many renewable energy farms generate electricity for sale to the grid, but small-scale power plants allow for additional revenue streams. Farms can produce and sell excess power generated from renewable sources, such as solar or biomass, while also tapping into government incentives, including feed-in tariffs or power purchase agreements (PPAs). This diversification helps farm operations stabilize their income over time.
- Example: A wind farm with a small-scale biomass power plant could sell excess electricity back to the grid, generating revenue even during off-peak wind periods.
9.18.5. Reduced Grid Dependence
In areas with unreliable or expensive grid infrastructure, small-scale power plants enable renewable energy farms to become more independent from external energy providers. This autonomy is particularly valuable in remote locations, where energy supply may be limited. Small-scale power plants allow farms to operate off-grid or function as part of a microgrid, increasing energy security.
- Example: A biomass-based microgrid can be integrated into a renewable farm to provide consistent power to farm operations, including irrigation systems, processing plants, or livestock facilities, reducing the need to import electricity.
9.18.6. Job Creation and Local Economic Development
The implementation of small-scale power plants can also drive local economic growth. Establishing and operating small renewable energy systems creates new job opportunities, both directly within the power plant and indirectly through local supply chains. Farms can employ skilled workers for system installation, maintenance, and management, contributing to local economies and enhancing regional development.
- Example: A farm that invests in a small-scale biomass plant might employ workers to manage the plant’s operation and maintenance, benefiting the local community through the creation of jobs.
9.18.7. Scalability and Modular Design
Small-scale power plants are typically modular, allowing renewable energy farms to scale up their energy production based on demand and available resources. This makes them particularly well-suited for farms that may need to adjust their energy output as operations expand or as resource availability fluctuates (e.g., changes in solar irradiance or wind speeds). Small plants can be easily upgraded or integrated with other renewable technologies over time.
- Example: A modular wind turbine array or biomass plant can be expanded in phases, adding capacity as the farm’s energy requirements increase.
9.19. Future Trends and Innovations in Small-Scale Power Plants for Renewable Energy Farms
The landscape of renewable energy is rapidly evolving, and small-scale power plants are a key part of the future of energy generation. Several innovations and trends are shaping the future of small-scale power systems for renewable energy farms, which could lead to even more efficient, cost-effective, and environmentally friendly solutions.
9.19.1. Advancements in Energy Storage Technologies
Energy storage is one of the most significant challenges for intermittent renewable energy sources like solar and wind. New technologies in battery storage, such as solid-state batteries and flow batteries, could offer more reliable, cost-effective storage options for small-scale power plants. These innovations would allow renewable energy farms to store surplus energy for use during periods of low generation, increasing grid stability and reducing energy waste.
9.19.2. Integration of Artificial Intelligence (AI) and Machine Learning (ML)
AI and ML technologies are increasingly being integrated into energy management systems to optimize the performance of small-scale power plants. These technologies can analyze vast amounts of data from wind, solar, and biomass systems to predict energy production patterns, optimize load distribution, and improve the efficiency of power generation. As AI and ML continue to evolve, renewable energy farms will be able to make smarter, data-driven decisions that reduce costs and enhance performance.
9.19.3. Hybrid Systems and Multi-Technology Integration
The future of renewable energy farms lies in integrating multiple types of energy systems to maximize efficiency. For example, hybrid systems that combine solar, wind, biomass, and energy storage offer the ability to take advantage of different energy resources depending on the time of day and weather conditions. These hybrid systems can be further enhanced with microgrids, allowing farms to manage energy generation and storage autonomously.
9.19.4. Carbon Capture and Utilization (CCU) in Biomass Power Plants
Biomass power plants, while relatively clean compared to fossil fuel-based generation, still emit some carbon dioxide during the combustion process. Advances in carbon capture and utilization (CCU) technologies could allow small-scale biomass plants to capture CO2 and repurpose it into useful products, such as biofuels or building materials. This would further reduce the environmental impact of small-scale power systems, making them a truly carbon-neutral energy solution.
9.19.5. Community-Driven Renewable Energy Initiatives
In the future, we can expect to see an increase in community-driven renewable energy projects where small-scale power plants are installed not just on farms, but in collaboration with local communities. These projects might involve cooperatives, local governments, or private companies working together to create sustainable, decentralized energy solutions that benefit the entire community.
9.20. Conclusion
Small-scale power plants have the potential to transform renewable energy farms into more sustainable, efficient, and economically viable operations. By enabling these farms to diversify energy sources, reduce operational costs, and contribute to global decarbonization goals, small-scale power plants represent an important tool in the future of agriculture and renewable energy.
The integration of small-scale power systems into renewable energy farms enhances energy security, cost savings, and environmental sustainability, making them a crucial element of a clean, decentralized energy future. With advancements in technology and growing policy support, small-scale power plants will continue to play a pivotal role in enabling farms and communities to transition to self-sufficient and resilient energy solutions.
9.21. Implementation Strategies for Small-Scale Power Plants in Renewable Energy Farms
Successfully integrating small-scale power plants into renewable energy farms requires a structured approach, blending technical, economic, and policy considerations. The following strategies outline key factors for planning and executing small-scale power plant projects on renewable energy farms, ensuring optimal performance and long-term sustainability.
9.21.1. Site Assessment and Resource Mapping
Before integrating small-scale power plants, it’s essential to conduct a thorough site assessment and resource mapping. This process helps identify the best locations for power generation based on factors such as:
- Wind speeds: For wind turbines, determining consistent wind patterns and speeds is essential for selecting the right turbine size and model.
- Solar irradiance: In solar-powered farms, measuring sunlight levels across seasons can help determine the optimal layout and capacity of solar panels.
- Biomass availability: For biomass-based systems, mapping the availability of organic materials (such as crop residues or wood) is critical to ensure a steady and sustainable fuel supply.
- Hydropower potential: If small-scale hydropower is considered, the availability and flow characteristics of local water sources should be assessed.
Data collected during this phase allows farm managers to make informed decisions about which renewable technologies are best suited for their location and how to design an efficient, integrated power system.
9.21.2. System Design and Hybrid Integration
Designing an optimal small-scale power plant requires careful consideration of the renewable technologies available and how they can be integrated to create a hybrid system. Hybrid systems combine multiple renewable energy sources to ensure continuous power supply, particularly when one source may be intermittent or unreliable. Key components include:
- Energy storage systems: Battery storage or other forms of energy storage should be incorporated to manage excess generation and ensure consistent energy availability during low production periods.
- Microgrid architecture: A microgrid setup allows the renewable farm to operate autonomously, utilizing small-scale power plants to meet local energy demand without needing a grid connection. This is especially useful for off-grid farms or in regions where grid connectivity is unreliable.
- Control systems: Smart controls, based on energy management systems (EMS), can automatically switch between power generation sources (wind, solar, biomass) depending on availability, helping maximize energy production efficiency.
A detailed system design phase allows farm operators to create a seamless integration of technologies, ensuring that the farm meets its energy needs with the lowest possible environmental impact.
9.21.3. Financing and Funding Models
The initial cost of setting up small-scale power plants can be significant. However, there are various financing and funding models that renewable energy farms can explore to ease the capital burden:
- Government Grants and Incentives: Many governments offer financial support for renewable energy projects, including small-scale power plants. Grants, tax credits, or feed-in tariffs can offset some of the upfront costs and make projects financially viable.
- Power Purchase Agreements (PPAs): Through PPAs, farms can secure long-term contracts with utilities or private buyers, guaranteeing stable revenue streams that can help finance power plant construction. These agreements may include capacity payments to ensure the farm receives financial support even when power generation is intermittent.
- Private Investment and Loans: Depending on the farm’s financial standing, it may seek private investors or take out loans to finance the power plants. This model often requires demonstrating the long-term financial benefits of renewable energy and the ability to repay the investment through energy sales.
- Community Funding and Co-operatives: In some cases, small-scale power plants may be financed through community investment programs or co-operatives. This model allows local residents or organizations to invest in renewable energy infrastructure and share in the profits.
By utilizing these financing models, farms can reduce initial capital requirements while also generating a stable, long-term revenue stream from renewable energy sales.
9.21.4. Regulatory and Compliance Considerations
Understanding the regulatory landscape is a crucial part of integrating small-scale power plants into renewable energy farms. These regulations vary by country and region but often involve:
- Environmental Impact Assessments (EIAs): Small-scale power plants, particularly those using biomass or hydropower, may require EIAs to evaluate potential environmental impacts and ensure compliance with sustainability standards.
- Energy Market Regulations: Farmers must navigate grid connection rules, power purchase agreements, and interconnection requirements when selling surplus power to the grid. Understanding local and national regulations helps avoid delays and ensures that energy is marketed and sold legally.
- Land Use and Zoning Regulations: Depending on the location of the farm, local zoning laws may dictate where and how renewable energy technologies can be installed. Farms must ensure that they comply with these regulations to avoid fines and unnecessary complications.
- Incentive Program Eligibility: Farms must be aware of eligibility requirements for tax incentives, subsidies, or government rebates for renewable energy investments. Understanding the documentation and processes involved helps ensure the farm takes full advantage of available support.
Compliance with these regulations is essential to avoid costly delays and penalties. Working with legal and regulatory experts can streamline the process and ensure full compliance.
9.21.5. Installation and Commissioning
Once the design, financing, and regulatory requirements are in place, the installation and commissioning phase begins. Key steps include:
- Procurement of Equipment: Small-scale power plants require specialized equipment, including turbines, solar panels, biomass boilers, energy storage systems, and control systems. Ensuring that the farm selects the most appropriate and reliable technology is crucial for maximizing efficiency.
- Skilled Workforce: Having a skilled workforce to oversee the installation and ensure proper assembly of all components is essential. Often, farms will work with engineering, procurement, and construction (EPC) contractors to manage these tasks.
- Testing and Validation: Prior to full-scale operation, it’s important to test the entire system to ensure it functions as designed. This includes verifying performance benchmarks, such as energy output, reliability, and system responsiveness.
- Training: Operators and staff must be trained to manage and maintain the small-scale power plant. This includes understanding the day-to-day operations, troubleshooting, and responding to emergencies or malfunctions.
The commissioning phase is critical to ensuring that the power plant is installed properly and is capable of generating reliable energy from the outset.
9.21.6. Ongoing Maintenance and Optimization
After commissioning, ongoing maintenance and monitoring are necessary to ensure the small-scale power plants continue to perform optimally. Key considerations include:
- Routine Maintenance: Each technology (wind turbines, solar panels, biomass boilers, etc.) requires regular maintenance to ensure long-term performance. This may include cleaning, lubrication, calibration, and replacing worn-out parts.
- Remote Monitoring and Diagnostics: Many renewable energy farms use remote monitoring systems to track the performance of small-scale power plants in real-time. This allows farm managers to detect issues early and address them before they lead to larger problems or downtimes.
- Energy Production Analysis: Regular performance analysis ensures that energy output matches expectations and helps identify areas for improvement. Over time, performance optimization may include adjusting settings, upgrading components, or expanding the system to increase capacity.
By keeping the system well-maintained and optimized, renewable energy farms can ensure the continued success of small-scale power plants, maximizing their return on investment.
9.21.7. Community Engagement and Social Impact
Successful small-scale power plants should also involve community engagement to ensure local support and alignment with the needs of surrounding populations. This could include:
- Public Consultation: Engaging the local community early in the planning phase helps ensure that the project aligns with local priorities and addresses any concerns, such as environmental impact, land use, or noise from equipment.
- Educational Programs: Farms can offer educational programs or workshops to raise awareness about renewable energy benefits and the role of small-scale power plants in sustainable agriculture.
- Job Creation and Local Benefits: As mentioned earlier, small-scale power plants can create local employment opportunities, from construction to ongoing operation. These benefits should be clearly communicated to community members, helping to foster goodwill and support.
By fostering a positive relationship with the community, renewable energy farms can build strong partnerships and ensure long-term success.
9.22. Conclusion
Implementing small-scale power plants on renewable energy farms offers numerous advantages in terms of energy security, economic viability, environmental sustainability, and grid resilience. By carefully considering site assessment, system design, financing, regulatory compliance, installation, and ongoing maintenance, farms can successfully integrate these systems into their operations.
As renewable energy continues to play a pivotal role in the global energy transition, small-scale power plants will become increasingly important in maximizing the efficiency and profitability of renewable energy farms. With careful planning and effective execution, these systems can not only support farm operations but also contribute to a cleaner, more sustainable energy future.
9.23. Performance Monitoring and Optimization for Small-Scale Power Plants
Once small-scale power plants are operational, ongoing monitoring and optimization are critical to ensure they continue to meet energy demands efficiently. Real-time data collection, predictive analytics, and routine maintenance help optimize plant performance, extending the system’s lifespan and improving its overall energy output.
9.23.1. Real-Time Performance Monitoring
To achieve high efficiency, renewable energy farms with small-scale power plants should employ advanced monitoring systems that track system performance in real-time. These systems monitor key parameters, such as:
- Energy production: The amount of electricity generated by wind, solar, biomass, or other technologies.
- Fuel efficiency: In the case of biomass plants, monitoring the fuel-to-energy conversion efficiency.
- Component status: For instance, monitoring turbine blade rotation speed, solar panel efficiency, or boiler temperature to ensure they are functioning optimally.
- Storage levels: In hybrid systems with energy storage (such as batteries), it’s crucial to monitor energy storage levels to ensure efficient charging and discharging cycles.
A centralized monitoring platform can provide an overview of system performance, identifying any underperforming components or operational anomalies.
- Example: A farm might use IoT sensors integrated with the control system to transmit performance data remotely. This data can be viewed on a dashboard, allowing operators to detect system issues instantly, reducing downtime.
9.23.2. Predictive Maintenance and Diagnostics
Through the use of predictive maintenance technologies, small-scale power plants can preemptively address issues before they lead to equipment failure. By analyzing historical performance data, vibration patterns, or temperature fluctuations, predictive maintenance tools can forecast potential breakdowns or inefficiencies.
- Example: Vibration sensors installed on wind turbine blades can detect irregular patterns that might signal wear and tear. Operators can schedule maintenance before a failure occurs, preventing costly repairs and unplanned downtime.
Another example is the use of machine learning algorithms to analyze operational data and identify inefficiencies, optimizing the operation of biomass boilers or solar inverters.
9.23.3. Performance Benchmarking
Establishing performance benchmarks is essential to evaluating the efficiency of small-scale power plants. Farms should compare real-time data with established industry standards and past performance to ensure that the plant is meeting expectations. If deviations are detected, optimization techniques should be applied.
- Example: For a biomass plant, benchmarking could include tracking the efficiency of combustion against typical industry standards. If energy conversion is below expectations, the farm may adjust fuel types, boiler settings, or enhance combustion processes.
9.23.4. Energy Efficiency Optimization
Optimizing energy efficiency involves fine-tuning both the power plant and the energy management system to ensure that all resources are being used optimally. This may involve:
- Energy conversion efficiency: Ensuring that every unit of input (whether wind, sunlight, or biomass fuel) is being converted to usable energy with minimal loss.
- Power output adjustment: For example, adjusting the output of solar panels depending on sunlight intensity or adjusting wind turbine operation based on wind speed.
- Load balancing: Efficiently distributing power to the grid or local farm operations, balancing between stored energy and energy generated in real-time.
- Example: A farm using both solar and biomass may prioritize solar power during sunny days and rely on biomass during cloudy periods or at night. Using an automated energy management system (EMS), these decisions can be made in real time to optimize energy production and storage.
9.23.5. System Upgrades and Technological Integration
As renewable energy technologies advance, small-scale power plants must evolve to incorporate new, more efficient technologies. Regularly upgrading the power generation and storage systems can lead to:
- Higher energy yields: Newer technologies often have better conversion efficiencies, increasing the overall energy yield per unit of resource (e.g., solar or wind).
- Lower operational costs: Advanced technologies often reduce fuel or maintenance costs over time. For example, newer biomass gasification systems are more efficient and have lower fuel consumption than older models.
- Better integration: Upgrading control systems to integrate better smart grid features can help improve coordination between small-scale power plants and the larger grid, allowing for greater flexibility in managing power distribution.
- Example: A farm that previously relied on older wind turbines could upgrade to a more advanced, high-efficiency turbine model with improved blades and control systems, allowing them to capture more energy even during low-wind periods.
9.23.6. Environmental Impact Monitoring
Although small-scale power plants are generally cleaner than traditional fossil fuel-based power generation, continuous monitoring of environmental impacts is essential to ensure sustainability. This includes:
- Carbon emissions: For biomass plants, measuring emissions of greenhouse gases, such as carbon dioxide (CO₂), is necessary to confirm that the plant is within acceptable environmental limits.
- Air and water quality: Especially for biomass or hydropower plants, air and water quality should be regularly monitored to ensure that there are no harmful emissions or contamination.
Continuous monitoring of these factors helps farms meet local environmental regulations and improves the farm’s environmental footprint.
- Example: A biomass power plant may use a carbon capture system to minimize CO₂ emissions. Regular emissions testing ensures the system operates within legal limits and verifies that emissions remain minimal.
9.23.7. Data Analytics and Decision Support Systems
Using data analytics tools, farms can derive actionable insights from the large amounts of data generated by small-scale power plants. Decision support systems (DSS) can analyze this data to recommend operational adjustments that optimize power generation, system health, and financial performance.
- Example: A DSS might analyze wind and solar generation data, combined with historical performance, to predict future energy production patterns and recommend when to shift to backup power sources like biomass or storage.
Additionally, big data analytics can forecast the future performance of the farm, enabling managers to make more accurate predictions about energy needs and potential market conditions.
9.23.8. Long-Term Sustainability and Impact
Monitoring and optimization efforts must be aligned with the goal of long-term sustainability, which requires both economic and environmental factors to be balanced. For example:
- Ensuring the power plant’s lifetime and performance are maximized to make the best use of initial capital investments.
- Reducing environmental impact by minimizing resource use and waste generation.
Farms should also regularly review energy efficiency measures and compare them with evolving sustainability goals. Adjustments can be made if new technologies or environmental policies influence the operation of small-scale power plants.
- Example: A farm may set long-term goals for net-zero energy by adopting more efficient renewable technologies, optimizing energy consumption, and exploring carbon offset programs to balance emissions.
9.24. Conclusion: Ensuring the Longevity and Success of Small-Scale Power Plants
The effective monitoring and optimization of small-scale power plants are central to their continued success in renewable energy farms. By employing real-time performance monitoring, predictive maintenance, energy efficiency optimization, and leveraging new technologies, farms can ensure their power plants provide reliable, cost-effective, and sustainable energy over the long term.
As renewable energy systems become more complex and integrated, ongoing optimization will allow small-scale power plants to evolve, maximize efficiency, and contribute to the farm’s overall energy and economic goals. With the proper tools and strategies in place, renewable energy farms can ensure that their small-scale power plants continue to thrive, contributing to a cleaner, more resilient energy future.
9.25. Small-Scale Power Plant for Remote Research Stations
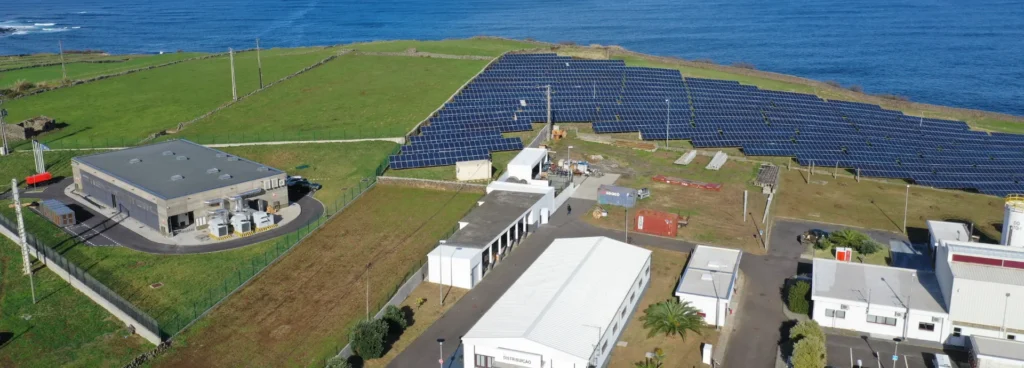
Remote research stations, often located in isolated or harsh environments, face significant challenges when it comes to power generation. These stations are typically located in areas far from the main electrical grid and are required to operate in extreme conditions, such as high altitudes, deserts, polar regions, or remote islands. Small-scale power plants for these locations are crucial to ensure the station has a reliable and sustainable energy supply for scientific experiments, staff living quarters, communication systems, and other essential operations.
9.25.1. Energy Requirements for Remote Research Stations
Before designing a small-scale power plant for a remote research station, it is essential to determine the specific energy needs of the station. These needs can vary widely depending on the size and type of research being conducted. Typical energy consumers include:
- Laboratories and research equipment: Many remote research stations house sensitive and energy-intensive equipment, such as microscopes, imaging devices, or field sensors.
- Living facilities: Energy for heating, cooling, lighting, and cooking for researchers and staff is a significant component of overall energy consumption.
- Communication systems: Satellite phones, internet connections, and other communication equipment often require reliable energy sources to ensure continuous operation.
- Water pumping and treatment: In remote areas, water must often be pumped, purified, and treated for consumption, requiring additional power resources.
The power system must be robust enough to meet these demands while considering the unpredictable nature of energy production and consumption in such isolated environments.
9.25.2. Suitable Renewable Energy Technologies
Given the remote location of these stations, renewable energy technologies are often the best solution for providing a reliable and sustainable power supply. The most suitable technologies include:
- Solar Power: Solar panels are particularly effective in sunny regions or places with long daylight hours, such as the Arctic during summer months or desert environments. Solar energy systems can provide a stable and renewable source of power for research stations.
- Hybrid Systems: Solar power is often used in combination with other renewable energy sources, such as wind or biomass, to ensure a continuous energy supply, especially in regions where sunlight may not be constant.
- Wind Power: For remote stations in areas with consistent wind patterns, wind turbines are a great option. Wind is often available during cloudy or nighttime periods when solar power may not be sufficient.
- Micro wind turbines: In some cases, smaller wind turbines are better suited to the scale and energy needs of a research station, particularly in low-wind areas.
- Biomass Energy: In research stations located near forests, agricultural lands, or other sources of organic material, biomass can be a valuable energy source. Biomass plants can use wood, agricultural waste, or other local organic materials for combustion or gasification to generate electricity.
- Micro-hydropower: If the research station is located near a flowing water source, micro-hydropower systems can provide a stable and continuous energy supply. These systems are especially effective in remote locations where water is abundant and reliable.
- Geothermal Energy: For stations located in geothermal hotspots, geothermal energy can be an incredibly effective source of power. It provides continuous, reliable energy regardless of weather conditions.
9.25.3. Hybrid Systems for Enhanced Reliability
A hybrid system combining multiple renewable energy sources can provide the most reliable and consistent energy supply for remote research stations. Common hybrid systems include:
- Solar + Wind: This combination is especially effective in areas with variable weather patterns. Solar power can generate energy during the day, while wind power can contribute during the night or in cloudy conditions.
- Solar + Biomass: Biomass energy can be used during periods of low solar radiation, such as cloudy days or during the night, while solar panels can generate power when sunlight is available.
- Wind + Hydro: For research stations located near water sources, combining wind and hydropower can ensure consistent energy production. Wind can supplement hydroelectric generation during periods of low water flow, and vice versa.
The key advantage of hybrid systems is that they compensate for the intermittent nature of individual renewable energy sources. They provide a more stable energy supply, which is critical for the successful operation of remote research stations.
9.25.4. Energy Storage and Management
Since remote research stations often operate in off-grid conditions, effective energy storage and management systems are crucial to ensure continuous power availability. The use of energy storage systems ensures that excess power generated during peak periods can be stored for later use, such as at night or during periods of low energy production.
- Battery Storage: Lithium-ion batteries, flow batteries, and lead-acid batteries are common storage options for small-scale power plants. These systems can store energy generated by solar panels, wind turbines, or other sources and provide power when needed.
- Thermal Storage: For stations using geothermal or biomass energy, thermal storage systems can store heat for later use in heating or electricity generation. This can be particularly useful for maintaining heating during long winter periods.
- Smart Energy Management: The integration of smart grids and energy management systems (EMS) enables remote research stations to manage energy production, storage, and consumption more efficiently. These systems can optimize energy flows, balance demand and supply, and prioritize critical loads.
- Demand Response: Using smart meters and sensors, research stations can implement demand-response programs to shift non-essential power usage to off-peak hours, thus improving overall energy efficiency.
9.25.5. Key Considerations for Remote Research Station Power Systems
When designing a small-scale power plant for a remote research station, several critical factors must be considered to ensure its success:
- Energy Demand and Load Assessment: A thorough energy audit should be conducted to understand the specific energy needs of the research station. This includes both base load (continuous) power requirements and peak loads (short-term spikes in demand). Proper assessment ensures that the power system is adequately sized.
- Extreme Weather Conditions: The station’s location may be subject to extreme temperatures, heavy snow, high winds, or other harsh environmental conditions. The power system must be designed to withstand these conditions, and components such as wind turbines or solar panels should be built to endure these extreme weather conditions.
- Redundancy and Reliability: Since power outages can severely impact research activities, reliability is paramount. The power system should include redundancy for critical components, such as backup generators and redundant power sources, to ensure continuity of power supply.
- Remote Monitoring and Control: Many remote research stations are located far from urban centers, which makes regular physical maintenance difficult. Therefore, remote monitoring technologies allow operators to check the performance of the power plant and detect potential issues without needing to travel to the site. Data collected through these systems can also be used to improve efficiency and predict maintenance needs.
- Environmental and Sustainability Considerations: The power system should minimize environmental impacts, and efforts should be made to ensure that any fuel sources, such as biomass, are sustainably sourced. Proper waste management practices for biomass or geothermal systems must also be implemented to ensure minimal environmental disturbance.
9.25.6. Maintenance and Support
Regular maintenance is critical to ensure the longevity and performance of power systems in remote locations. Due to the isolated nature of these stations, maintenance schedules should take into account the difficulty of sending repair teams. Key maintenance activities include:
- Routine Inspections: Scheduled inspections of solar panels, wind turbines, biomass boilers, or other equipment ensure that minor issues are detected early before they develop into major problems.
- Component Replacement: Over time, parts of the power system, such as solar inverters, turbine blades, or batteries, may wear out and require replacement.
- Remote Troubleshooting: Many systems include remote diagnostics, allowing technicians to assess and fix issues without needing to visit the site. In cases where on-site intervention is necessary, drones or remote-controlled robots can be used for inspections in particularly hard-to-reach areas.
9.25.7. Cost Considerations
The cost of implementing small-scale power plants in remote research stations can be high, especially when considering the expenses for specialized equipment and installation in difficult-to-access areas. However, long-term savings can be achieved through:
- Reduced fuel dependency: By using renewable energy, research stations reduce their reliance on costly and sometimes difficult-to-transport fossil fuels, which can significantly cut operational costs over time.
- Reduced transportation and maintenance costs: With efficient, automated systems for energy management and remote monitoring, the need for frequent on-site maintenance visits is minimized, further reducing costs.
9.25.8. Conclusion
Small-scale power plants for remote research stations are a crucial component of ensuring that research activities can continue uninterrupted in isolated and harsh environments. By integrating renewable energy sources, energy storage, and management systems, these stations can be self-sufficient and resilient to external challenges.
The use of solar, wind, biomass, and hydropower systems, in combination with hybrid systems and reliable storage, allows research stations to maintain energy independence, reduce environmental impact, and enhance sustainability. While initial capital costs can be high, the long-term benefits of sustainability, reliability, and cost savings make small-scale renewable energy solutions a smart investment for remote research facilities.
9.25.9. Case Studies of Small-Scale Power Plants at Remote Research Stations
To illustrate the effectiveness of small-scale power plants in remote research stations, here are a few case studies of research facilities that have successfully implemented such systems:
9.25.9.1. Arctic Research Station: Solar and Wind Hybrid System
Located in the Arctic Circle, the Arctic Research Station faced extreme cold temperatures, long winters with limited sunlight, and powerful winds. To provide a sustainable energy solution, the station implemented a hybrid power system consisting of solar panels, wind turbines, and battery storage.
- Energy Solution:
- Solar Panels: The system uses high-efficiency polycrystalline solar panels that operate effectively in low-light conditions, providing power during daylight hours.
- Wind Turbines: With average wind speeds exceeding 10 m/s, the wind turbines generate a significant portion of the energy, especially during the long winter months.
- Energy Storage: Lithium-ion batteries store excess energy generated during periods of high sunlight and wind, ensuring a continuous power supply even during the polar night.
- Outcome: The hybrid system has significantly reduced the station’s reliance on diesel generators, which were previously used as backup power sources. It has also provided a reliable, low-emission energy supply, ensuring that the station can continue its research operations throughout the year, even under extreme weather conditions.
9.25.9.2. Antarctic Research Station: Geothermal and Solar Energy
In the Antarctic, the South Pole Research Station is one of the most isolated scientific stations in the world, with temperatures often dropping below -50°C. The station operates for long months without sunlight and experiences frequent snowstorms, making traditional power sources like fossil fuels both expensive and unreliable.
- Energy Solution:
- Geothermal Energy: The station took advantage of its location near geothermal hot springs to install a small-scale geothermal power plant. This plant provides a continuous, stable power supply, unaffected by the cold or snowstorms.
- Solar Power: During the summer months when sunlight is available for extended periods, solar panels supplement geothermal power generation.
- Battery Storage: A network of sodium-sulfur batteries stores energy generated by both geothermal and solar systems to provide backup power during the winter months when sunlight is unavailable.
- Outcome: The geothermal system provides a base load of energy, reducing the need for expensive and polluting diesel generators. The integration of solar energy during the summer months maximizes energy production when conditions are favorable. This energy mix ensures that the station remains powered year-round, with reduced environmental impact and reliance on external fuel supplies.
9.25.9.3. Desert Research Station: Solar-Powered Energy System
Located in a remote desert region, the Desert Research Station faced issues with extreme temperatures and unreliable access to fuel supplies. The station opted for a purely solar-powered solution, with an emphasis on energy independence.
- Energy Solution:
- Photovoltaic Solar Panels: The station installed large-scale solar arrays, taking advantage of the consistent high levels of solar radiation.
- Solar Thermal Systems: In addition to photovoltaic panels, solar thermal collectors were used for water heating, further reducing the need for electricity to power water heaters and cooling systems.
- Energy Storage: The system uses flow batteries for long-term energy storage, enabling the station to maintain power during the night and on cloudy days.
- Outcome: This all-solar system has allowed the Desert Research Station to completely eliminate diesel generators. It has successfully reduced operational costs and carbon emissions. The station can now focus on research without worrying about power supply, even during the hottest and sunniest periods when the energy demand spikes.
9.25.9.4. Remote Island Research Facility: Wind and Micro-Hydropower
On a remote island in the Pacific, a research station studying marine biology and climate change needed a reliable power supply to support lab equipment, living quarters, and essential communication systems. The island’s isolated location and limited transportation options made fossil fuel-based power unreliable and costly.
- Energy Solution:
- Wind Turbines: The island receives consistent trade winds, making small-scale wind turbines ideal for power generation. A combination of horizontal-axis and vertical-axis turbines was used to optimize energy capture from varying wind directions.
- Micro-Hydropower: A small stream flowing near the facility provided a steady source of power through a micro-hydropower system. The system uses a small turbine and generator to produce electricity from the water flow.
- Energy Storage: Lithium iron phosphate (LiFePO4) batteries were deployed to store excess energy generated by the wind turbines and micro-hydro system for use during periods of low wind or water flow.
- Outcome: This hybrid power system provides a reliable and resilient energy supply that is resistant to environmental disruption. The island research station has significantly reduced its reliance on imported fuel, cutting operational costs and environmental impact. The use of both wind and hydro ensures that the station’s power supply remains steady even if one energy source fluctuates.
9.25.9.5. Forest Research Station: Biomass and Solar Power
In a remote forest research station located near a sustainable supply of wood and plant waste, the station sought to reduce its reliance on fossil fuels by utilizing locally sourced biomass.
- Energy Solution:
- Biomass Combustion: The research station installed a biomass gasification unit, which converts wood chips and other plant-based materials into a gaseous fuel that can be used for electricity generation.
- Solar Power: Complementing the biomass system, solar panels provide additional energy during the day, reducing the station’s fuel consumption.
- Energy Storage: The system incorporates advanced lead-acid batteries for energy storage, allowing for a steady supply of power during periods of low sunlight or when biomass fuel is being processed.
- Outcome: By utilizing local biomass resources, the research station significantly reduced its carbon footprint and dependence on external fuel supplies. The combined biomass and solar system provides a cost-effective, environmentally friendly power solution, supporting the research activities without compromising sustainability.
9.26. Conclusion: Powering the Future of Remote Research Stations
Small-scale power plants are essential for the continued operation of remote research stations, where access to the electrical grid is limited or nonexistent. Renewable energy solutions, such as solar, wind, biomass, micro-hydro, geothermal, and their hybrid combinations, offer sustainable, reliable, and cost-effective alternatives to traditional power sources.
By integrating energy storage and smart management systems, remote research stations can enhance their energy resilience, ensuring that vital research activities continue uninterrupted, even in the most extreme conditions. Through case studies and real-world examples, it is clear that small-scale power plants can effectively meet the unique energy demands of remote research stations, providing both operational efficiency and environmental sustainability.
These innovations in energy systems not only support scientific progress in isolated locations but also contribute to the broader goal of transitioning to a more sustainable and resilient global energy future.
9.27. Challenges and Opportunities for Small-Scale Power Plants at Remote Research Stations
While small-scale power plants provide numerous benefits for remote research stations, there are still challenges that must be addressed to ensure their optimal performance and long-term sustainability. These challenges often involve technological, logistical, financial, and environmental considerations. However, these challenges also present opportunities for innovation, research, and improved solutions.
9.27.1. Technological Challenges
- Energy Storage Limitations: While batteries and other energy storage systems are critical for ensuring continuous power in the absence of sunlight or wind, storage technologies still have limitations. Issues such as battery degradation, limited capacity, and high cost can reduce the efficiency and reliability of storage systems over time.
- Opportunity: Ongoing advancements in solid-state batteries, flow batteries, and long-duration energy storage technologies offer the potential to overcome current limitations. Research into these new energy storage technologies can help provide longer-lasting, more cost-effective solutions.
- Energy System Integration: Integrating multiple energy sources (e.g., solar, wind, hydro, and biomass) into a cohesive power system that works efficiently across various environmental conditions can be complex. Ensuring smooth operation and minimal downtime requires advanced energy management systems and smart grids.
- Opportunity: The development of more advanced control algorithms and machine learning models for energy optimization can improve the integration of different power generation sources. These systems can dynamically adjust based on weather patterns and demand fluctuations, enhancing system performance and reducing the risk of energy shortages.
- Reliability in Harsh Conditions: Extreme weather conditions, such as heavy snow, extreme cold, or high winds, can damage or hinder the performance of renewable energy systems. For example, solar panels may get covered in snow, and wind turbines may require de-icing to maintain functionality in cold climates.
- Opportunity: Investing in weather-resistant components, such as heavily coated solar panels and turbines designed for Arctic conditions, can enhance the resilience of small-scale power systems. Additionally, automated de-icing technologies and self-cleaning panels could improve system efficiency and reduce maintenance needs.
9.27.2. Logistical Challenges
- Access to Spare Parts and Maintenance Services: Remote research stations often face logistical challenges when it comes to maintaining their power systems. Access to spare parts or skilled technicians can be limited, especially in extremely isolated areas.
- Opportunity: One solution is to implement modular systems that allow for easy replacement of components without requiring specialized skills. Research stations can also invest in local workforce training to ensure that on-site staff can perform basic maintenance and repairs. Additionally, remote diagnostic technologies allow technicians to troubleshoot and perform system updates remotely, reducing the need for on-site visits.
- Fuel Delivery and Supply: Although renewable energy systems reduce the need for fossil fuels, backup power systems, such as diesel generators, may still be necessary in case of extended periods of low energy production from renewables. Delivering fuel to remote research stations can be expensive and logistically difficult.
- Opportunity: Further development of biofuels, synthetic fuels, and local energy generation from biomass could help reduce the need for external fuel deliveries. Research stations can explore using local organic waste or by-products for biomass systems, reducing reliance on imported fuels.
- Infrastructure for Energy Distribution: Remote research stations may have limited infrastructure for distributing electricity across the station. The need for power in distant areas (e.g., laboratories, research equipment, staff quarters) may require long transmission lines that are difficult and costly to maintain.
- Opportunity: The use of microgrids within research stations allows for localized energy distribution, reducing transmission losses and improving overall efficiency. Wireless power transmission technologies also show promise for powering remote areas without the need for extensive cabling.
9.27.3. Financial and Economic Challenges
- High Initial Costs: The upfront cost of installing renewable energy systems and energy storage technologies can be prohibitively high for some remote research stations, particularly those with limited funding.
- Opportunity: Government grants, private investments, and public-private partnerships can provide the necessary financial backing to offset the initial investment costs. Additionally, the long-term savings from reduced fuel consumption and maintenance costs can make these investments financially viable over time.
- Economic Viability of Hybrid Systems: While hybrid systems combining multiple renewable energy sources offer enhanced reliability, the cost of integrating such systems may be higher than traditional single-source systems. The costs associated with multiple energy generation systems and storage solutions need to be carefully balanced against operational savings.
- Opportunity: Over time, as renewable energy technologies become more widely adopted, their costs are expected to decrease. The economies of scale will make hybrid systems more affordable. Furthermore, energy-as-a-service models—where energy providers share the responsibility for managing and maintaining the system—can reduce the upfront costs for research stations.
9.27.4. Environmental Challenges
- Impact of Energy Systems on the Ecosystem: Although renewable energy systems are generally considered environmentally friendly, their installation and operation can have unintended consequences on local ecosystems. For example, the construction of wind turbines or hydroelectric plants could impact wildlife habitats or aquatic ecosystems.
- Opportunity: Conducting thorough environmental impact assessments before installation ensures that renewable energy systems are designed and located in ways that minimize environmental disruption. Developing low-impact technologies, such as bird-friendly wind turbines or fish-friendly hydropower turbines, can reduce potential ecological harm.
- Waste Disposal and Sustainability: Some renewable energy systems, such as biomass or solar power systems, generate waste products that need to be managed. For example, old solar panels, batteries, and biomass residues can create disposal challenges if not handled properly.
- Opportunity: Research into circular economy models, where materials are reused or recycled, can help reduce waste. In addition, biomass waste can be processed into biochar or used for other agricultural purposes, turning waste into a valuable resource. Moreover, advances in recycling technologies for solar panels and batteries will help reduce their environmental footprint.
9.27.5. Social and Cultural Challenges
- Community Engagement and Support: In some cases, remote research stations may be located near indigenous or local communities that may have concerns about the environmental impact of power systems. These communities may also have limited experience with renewable energy technologies.
- Opportunity: Engaging local communities in the planning and implementation process is crucial to ensuring that energy solutions are culturally appropriate and beneficial. Offering educational programs and involving the local population in the operation and maintenance of energy systems can help build support and create local jobs, contributing to sustainable development.
- Training and Capacity Building: The operation of advanced renewable energy systems requires skilled personnel. However, remote locations often struggle to attract and retain qualified energy technicians or researchers.
- Opportunity: Remote training programs, such as online courses or virtual training simulations, can help staff acquire the skills needed to operate and maintain energy systems. Moreover, local technician training can provide long-term benefits to both the research station and the surrounding community by building local expertise.
9.27.6. Conclusion: Path Forward
While the challenges faced by small-scale power plants at remote research stations are considerable, they are not insurmountable. Technological advancements, financial models, and smart solutions to logistical and environmental challenges present many opportunities for innovation. By addressing these obstacles, research stations can become more energy resilient, sustainable, and self-sufficient.
Investments in renewable energy and energy storage, combined with effective energy management systems, offer the potential to significantly reduce the environmental and financial burdens of remote research operations. As technology continues to evolve, small-scale power plants will increasingly play a critical role in supporting scientific research in remote and challenging environments while advancing the global transition to renewable energy.
9.28. Future Trends and Innovations in Small-Scale Power Plants for Remote Research Stations
As remote research stations face increasing energy demands and the need for sustainability, the future of small-scale power plants is evolving rapidly. New technological advancements, energy strategies, and environmental considerations will drive innovation in this sector. The following trends and innovations are expected to shape the future of energy systems for remote research stations.
9.28.1. Advanced Renewable Energy Technologies
- Next-Generation Solar Panels: Traditional solar panels are already widely used, but next-generation solar technologies such as perovskite solar cells and organic photovoltaics are emerging as highly efficient, flexible, and low-cost alternatives. These new materials have the potential to provide higher energy conversion rates, even in less-than-ideal weather conditions, which is crucial for research stations located in remote areas with limited sunlight.
- Opportunity: As perovskite and organic solar panels become more affordable and scalable, they could be implemented in remote stations to boost energy generation while reducing the overall costs of installation and maintenance.
- Floating Solar Power: For stations near bodies of water, floating solar farms can offer a unique advantage. By utilizing water surfaces such as lakes, rivers, and reservoirs for solar energy production, these systems take advantage of cooling effects from the water, which can improve panel efficiency. Floating solar power also reduces the need for land, making it ideal for stations where space is limited.
- Opportunity: Hybrid systems combining floating solar and small-scale hydroelectric plants could offer a reliable and sustainable power solution in areas with water resources.
- Advanced Wind Turbines: Newer, more efficient vertical-axis wind turbines (VAWTs) and smaller horizontal-axis turbines are being developed specifically for low-wind environments and locations with variable weather conditions. These compact designs are more resilient and can generate power at lower speeds.
- Opportunity: Research stations in remote areas with unpredictable wind patterns can benefit from these advanced wind turbines, ensuring more consistent and reliable power production. Small-scale turbines with smart control systems will also allow for real-time monitoring and optimization of energy generation.
9.28.2. Energy Storage Innovations
- Solid-State Batteries: Solid-state batteries represent the next frontier in energy storage technology, offering higher energy densities, faster charging times, and greater safety than traditional lithium-ion batteries. These batteries could be ideal for use in remote locations where energy storage is crucial to balancing intermittent renewable energy generation.
- Opportunity: The development of affordable and scalable solid-state batteries could significantly improve the reliability and longevity of energy storage systems in remote research stations, reducing the need for frequent replacements and boosting overall energy security.
- Hydrogen Energy Storage: The use of hydrogen as a form of energy storage is gaining attention due to its potential for large-scale, long-duration storage. Renewable electricity can be used to produce hydrogen through electrolysis, which can then be stored and converted back into electricity when needed using fuel cells or combustion turbines.
- Opportunity: Research stations with access to both renewable energy generation (solar, wind) and water sources could explore hydrogen energy storage as a long-term solution for seasonal energy supply. This technology could help ensure continuous power during periods of low renewable generation.
- Thermal Energy Storage: Thermal storage systems, such as molten salt and phase-change materials, allow excess energy to be stored in the form of heat. This heat can later be converted back into electricity or used for heating purposes during periods of high demand or low energy generation.
- Opportunity: Research stations in colder climates could integrate thermal storage systems into their energy infrastructure, providing reliable heat and power during the winter months when energy needs spike.
9.28.3. Microgrids and Smart Energy Management
- Microgrids for Energy Resilience: Microgrids are localized energy networks that can operate independently from the main power grid. These systems can seamlessly integrate multiple power sources—such as solar, wind, hydro, and biomass—and can function autonomously or be connected to the larger grid. Microgrids are especially beneficial in remote research stations because they can provide localized energy independence, reducing the vulnerability of the station to power outages.
- Opportunity: The integration of smart microgrids in remote stations will allow for real-time monitoring and predictive maintenance, optimizing power distribution and improving the overall efficiency of the energy system. These systems can also incorporate demand-side management, adjusting energy consumption based on availability and priorities.
- Smart Energy Management Systems (EMS): Advanced EMS use artificial intelligence (AI) and machine learning (ML) to optimize energy production, storage, and distribution. By analyzing data on weather patterns, energy usage, and system performance, these systems can make decisions that minimize waste, reduce costs, and ensure continuous power availability.
- Opportunity: The widespread use of AI-driven energy management systems will help remote research stations dynamically balance energy supply and demand, ensuring that energy is used efficiently while minimizing costs and environmental impact. These systems could also incorporate demand forecasting to predict periods of high or low energy consumption and optimize storage and generation accordingly.
9.28.4. Hybrid Power Systems and Energy Diversification
- Hybrid Systems Combining Multiple Renewables: The combination of different renewable energy sources in a hybrid system allows for redundancy and increased reliability. For example, solar-wind hybrid systems are becoming popular because they complement each other: solar power is strongest during the day, while wind power often peaks at night or during stormy conditions.
- Opportunity: Research stations can take advantage of hybrid renewable energy systems to ensure that energy generation is continuous, even if one source is temporarily unavailable due to weather or seasonal fluctuations.
- Bioenergy and Waste-to-Energy Systems: In addition to solar, wind, and hydropower, bioenergy offers an attractive option for small-scale power generation, particularly in areas with access to organic waste or biomass. Stations could implement biogas systems or waste-to-energy technologies that convert organic waste into usable power.
- Opportunity: By integrating waste-to-energy systems, research stations can make use of local resources, such as plant matter, wood chips, or even agricultural by-products. This reduces waste disposal costs and provides a reliable, sustainable power source.
9.28.5. Resilience and Sustainability
- Adaptable and Modular Power Systems: One of the key trends in small-scale power plants is the shift toward modular systems that can be easily scaled or adapted to changing energy demands. These systems are flexible, allowing stations to add additional capacity as their energy needs grow or as new technologies become available.
- Opportunity: Modular systems, combined with smart controllers, enable stations to quickly adapt to changing environmental conditions, such as increased energy demand during extreme weather events or new research equipment that requires more power.
- Circular Economy Models: As sustainability becomes a top priority, small-scale power plants will increasingly adopt circular economy principles, which prioritize the reuse, recycling, and repurposing of materials. This includes the recycling of old solar panels, wind turbines, and batteries, as well as the use of sustainable materials in the construction and maintenance of energy systems.
- Opportunity: By embracing circular economy practices, remote research stations can ensure that their energy infrastructure remains sustainable over the long term, reducing the environmental impact of both energy generation and system disposal.
9.28.6. Conclusion: A Sustainable and Resilient Future for Remote Research Stations
The future of small-scale power plants for remote research stations is marked by increasing technological sophistication, energy resilience, and environmental sustainability. Emerging renewable energy technologies, advanced energy storage solutions, and smart microgrids will empower remote stations to reduce their reliance on traditional fuel sources, minimize costs, and improve energy security.
The ongoing integration of hybrid systems, smart energy management, and modular technologies will offer research stations a greater degree of energy independence, ensuring that they can operate efficiently and sustainably, regardless of location or environmental challenges. As the world transitions to cleaner, more sustainable energy systems, remote research stations will play a critical role in advancing scientific knowledge while demonstrating the potential of renewable energy in the most extreme conditions.
Through continued innovation, investment, and collaboration, small-scale power plants for remote research stations will contribute to a cleaner, more resilient energy future, fostering global sustainability while supporting groundbreaking research in some of the most isolated places on Earth.
9.29. Small Scale Power Plants for Grid Support
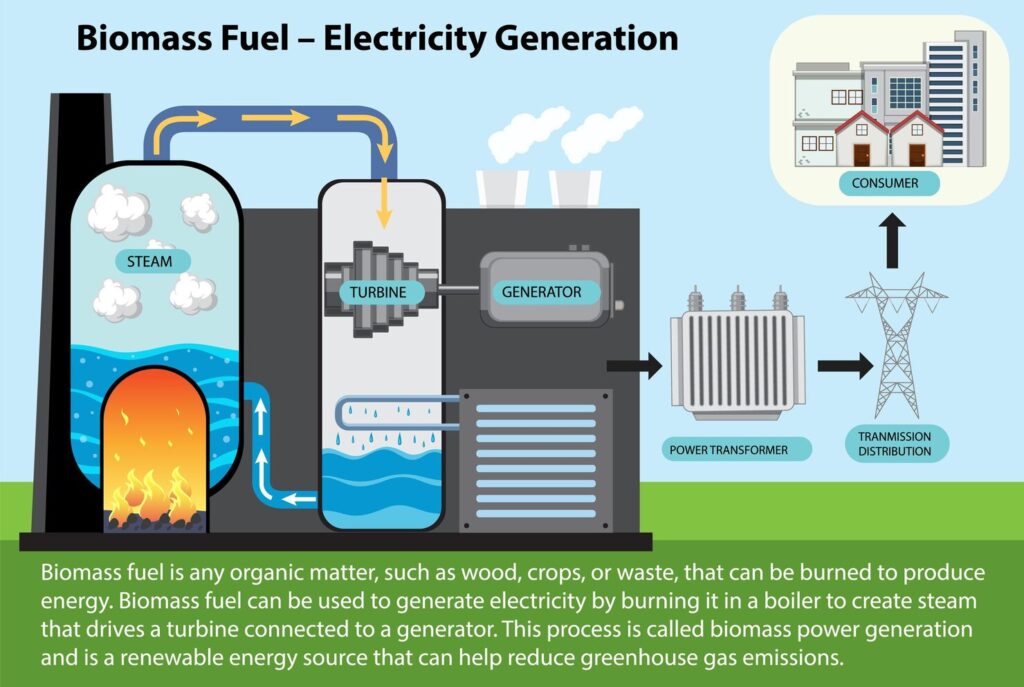
Small-scale power plants can play a significant role in enhancing the stability and reliability of the power grid, especially as more renewable energy sources are integrated into national or regional grids. These power plants, through various technological and operational innovations, can serve as essential assets for balancing supply and demand, maintaining voltage stability, and providing backup during grid disruptions. The following sections explore the contributions, challenges, and opportunities of small-scale power plants designed specifically for grid support.
9.29.1. Role of Small-Scale Power Plants in Grid Stability
As the global energy landscape shifts toward decentralized and renewable energy sources, maintaining grid reliability has become increasingly complex. Small-scale power plants, whether powered by renewables like wind, solar, or biomass, or by backup generation systems like natural gas or hydropower, can support the grid in the following ways:
- Frequency Regulation: Small-scale power plants can quickly adjust output to match fluctuations in grid demand, helping maintain grid frequency within acceptable limits. This is especially important as renewable energy sources, like wind and solar, can be intermittent, causing rapid changes in power generation.
- Opportunity: Battery storage integrated with small-scale power plants can enhance frequency regulation by providing fast-responding storage solutions that can release energy when renewable generation is low or absorb energy during peak production, stabilizing the grid in real-time.
- Voltage Support: Small-scale plants can be designed to provide reactive power (or voltage support) to the grid, which helps maintain voltage levels within operational limits. This is essential in regions where long transmission lines or the integration of large-scale renewable sources can cause voltage instability.
- Opportunity: Flexible power inverters and smart grid technology can enable small-scale power plants to provide voltage support dynamically, adjusting output and characteristics based on real-time grid needs.
- Backup Power and Grid Resilience: Small-scale power plants can provide critical backup power in the event of grid failure or natural disasters. In some cases, they can also help during periods of peak demand or when the grid is undergoing maintenance or upgrades.
- Opportunity: Small-scale plants with energy storage systems can offer black start capabilities, meaning they can start up without external power supply and help restore the grid after a total blackout.
9.29.2. Technologies Enabling Small-Scale Power Plants for Grid Support
- Microgrids: Microgrids are localized energy networks that can operate independently or in parallel with the main grid. Small-scale power plants, when connected to microgrids, can help ensure grid stability by providing localized energy generation and storage. They also support resilience in cases of power outages or grid failures.
- Opportunity: Hybrid microgrid systems that integrate renewable energy sources with backup generation and storage systems can serve as grid assets, reducing dependence on centralized power plants while enhancing grid reliability.
- Demand Response Programs: Small-scale power plants can participate in demand response (DR) programs, which incentivize consumers to reduce or shift energy usage during peak demand times. These programs help balance supply and demand, reducing the strain on the grid and preventing blackouts.
- Opportunity: By leveraging smart meters, IoT technology, and real-time communication systems, small-scale plants can seamlessly participate in DR programs, improving overall grid efficiency and stability.
- Battery Energy Storage Systems (BESS): Battery systems paired with small-scale power plants allow for rapid energy storage and dispatch, which can support grid frequency regulation and provide backup power. BESS technology can help overcome the intermittency of renewable energy sources by storing excess power for later use.
- Opportunity: The integration of large-scale battery banks with small-scale plants allows for increased grid reliability, enabling plants to dispatch power when demand peaks and stabilize grid fluctuations caused by renewable energy generation.
- Virtual Power Plants (VPPs): A virtual power plant is a network of small-scale distributed energy resources, including power plants, batteries, and renewable energy sources, that are aggregated and operated as a single unit. VPPs provide grid support by pooling resources to supply power when needed and optimizing energy production.
- Opportunity: A VPP can enhance grid flexibility and reliability by coordinating and dispatching power from a variety of distributed resources, which can reduce the need for traditional fossil fuel-based peaking plants and provide a cleaner, more sustainable energy source.
9.29.3. Operational Challenges for Small-Scale Power Plants in Grid Support
While small-scale power plants offer significant potential for grid support, they also face several operational challenges that need to be addressed for optimal performance.
- Intermittency of Renewable Sources: Many small-scale power plants rely on renewable energy sources such as solar or wind, which can be intermittent. This creates a challenge in maintaining a consistent and reliable power supply to the grid.
- Opportunity: Advanced energy storage technologies (e.g., solid-state batteries, flow batteries, hydrogen storage) can mitigate the intermittency of renewable power sources by storing excess energy and discharging it when renewable generation is low, enhancing grid stability.
- Grid Compatibility and Integration: Connecting small-scale power plants to the grid requires careful consideration of voltage and frequency synchronization. Compatibility with the grid requires the use of specialized inverters and energy management systems that ensure that power generation from distributed sources is properly integrated into the larger grid infrastructure.
- Opportunity: The development of smart grid infrastructure and advanced inverters that can dynamically adjust to varying power generation levels will help simplify integration and ensure that small-scale plants provide seamless support to the grid.
- Regulatory and Market Challenges: The regulatory framework governing grid interactions and market participation can be complex and varies from region to region. Small-scale power plants may encounter barriers when attempting to participate in grid support functions such as frequency regulation, voltage support, or demand response programs.
- Opportunity: Policymakers can support the integration of small-scale plants into grid operations by developing flexible regulatory frameworks and creating market structures that incentivize participation in grid support activities. Programs such as capacity markets or ancillary service markets could be expanded to accommodate small-scale power plants.
9.29.4. Economic and Financial Considerations
- Cost-Effectiveness: Small-scale power plants generally have higher upfront costs compared to large-scale power plants, and their economic viability depends on several factors, such as capacity, operational efficiency, and integration with the grid. For grid support, these plants must be designed to provide consistent services at a competitive price.
- Opportunity: Financial incentives and subsidies for small-scale plants providing grid services, such as tax credits for energy storage or renewable generation, can improve their economic attractiveness. Additionally, pay-for-performance models that reward small-scale plants for their grid services can improve financial sustainability.
- Operational Efficiency: Small-scale plants need to optimize their efficiency to be cost-competitive with larger, centralized power plants. This includes minimizing downtime, ensuring proper maintenance, and reducing fuel consumption.
- Opportunity: Through the use of predictive analytics and condition-based maintenance, small-scale plants can reduce operational costs and improve uptime, enhancing their value as grid support assets.
9.29.5. Environmental and Sustainability Considerations
- Low-Carbon Emissions: One of the key advantages of small-scale power plants is their potential to reduce carbon emissions compared to conventional, fossil-fuel-based grid generation. Small-scale plants powered by renewables such as solar, wind, and biomass can significantly decrease the overall carbon footprint of the grid.
- Opportunity: Integrating small-scale power plants into the grid not only supports grid stability but also contributes to decarbonizing the energy sector, aligning with global sustainability goals and reducing the dependence on polluting fossil fuel plants.
- Land and Resource Use: Small-scale power plants, especially those powered by renewable sources, can reduce land use and environmental disruption compared to larger, centralized plants. However, some renewable technologies (such as large-scale hydropower or bioenergy) still require significant land or natural resources.
- Opportunity: The design of small-scale plants should prioritize minimizing environmental impact, using modular and low-footprint technologies, such as floating solar panels or vertical wind turbines, to reduce land-use competition with agricultural, industrial, or conservation needs.
9.29.6. Conclusion: A New Era of Grid Support with Small-Scale Power Plants
Small-scale power plants are poised to become a fundamental part of the energy landscape, providing essential grid support and enhancing resilience in power systems. By integrating renewable generation, energy storage, microgrids, and smart technologies, these plants can stabilize grids, ensure backup power, and reduce the reliance on fossil fuels.
As technological innovations and policy frameworks evolve, small-scale power plants will continue to play a significant role in shaping the future of energy. By embracing the full potential of these systems, the grid will become more flexible, resilient, and sustainable, contributing to a cleaner, more reliable energy future for all.
9.30. Small-Scale Power Plants for Industrial and Commercial Applications
Small-scale power plants can significantly benefit industrial and commercial sectors by providing reliable, cost-effective, and sustainable energy solutions. These applications focus on reducing operational costs, enhancing energy efficiency, and increasing energy independence while supporting environmental goals. As industries move toward sustainable energy practices, small-scale power plants can become a crucial component of energy strategies for businesses across various sectors, from manufacturing to commercial establishments.
9.30.1. Advantages of Small-Scale Power Plants for Industrial and Commercial Use
- Cost Reduction: Small-scale power plants, particularly those utilizing renewable resources such as solar, wind, and biomass, offer businesses the opportunity to reduce energy costs by generating their own electricity on-site. This reduces reliance on grid power, which can fluctuate in price depending on market conditions and grid demand.
- Opportunity: By implementing energy generation and storage systems such as solar photovoltaic (PV) panels paired with battery storage, industries can lower their utility bills, reduce demand charges, and gain financial stability. Moreover, businesses that adopt renewable energy solutions can take advantage of government incentives and subsidies, lowering initial capital costs.
- Energy Independence and Reliability: Small-scale power plants provide businesses with a degree of energy independence, ensuring that they have a stable and reliable power supply even during grid outages or disruptions. This is especially crucial for industries that rely on continuous operations or sensitive equipment, such as data centers, manufacturing plants, or cold storage facilities.
- Opportunity: By establishing microgrids or standalone renewable energy systems, businesses can safeguard their operations from grid instability, maintain productivity during outages, and increase operational continuity.
- Sustainability and Corporate Responsibility: As companies increasingly prioritize sustainability, small-scale power plants provide an opportunity to reduce their carbon footprint and contribute to environmental goals. The shift toward green energy can enhance a company’s brand reputation and improve its standing with investors, customers, and regulatory authorities.
- Opportunity: Transitioning to solar, wind, or biomass energy can enable industries to achieve sustainability targets, meet carbon reduction commitments, and improve their public image as environmentally responsible organizations.
9.30.2. Technologies and Applications for Small-Scale Power Plants in Industry and Commerce
- Solar Power Systems: Solar energy is one of the most common and accessible technologies for small-scale power plants. Commercial and industrial businesses can install rooftop solar arrays or ground-mounted PV systems to generate power during daylight hours. In combination with energy storage, solar power can supply energy during peak periods and after sunset.
- Opportunity: Businesses can offset electricity costs and reduce reliance on expensive peak-hour electricity rates by using solar-plus-storage systems, where the solar array generates energy during the day and the battery stores it for later use.
- Combined Heat and Power (CHP) Systems: CHP systems, also known as cogeneration, simultaneously generate electricity and useful heat from a single fuel source. Small-scale CHP plants are highly efficient and can be powered by a variety of fuels, including natural gas, biomass, or even waste heat from industrial processes.
- Opportunity: By utilizing waste heat recovery technologies, businesses can reduce energy consumption, improve efficiency, and decrease operating costs. Industries like food processing, paper mills, and chemical manufacturing can benefit from CHP plants that use waste products to generate both electricity and thermal energy.
- Biomass and Waste-to-Energy: Biomass power plants convert organic waste materials—such as agricultural residues, wood chips, or food waste—into energy. Small-scale biomass plants can help industries manage waste while generating power. These systems also reduce dependence on fossil fuels and can provide a sustainable solution for both energy and waste management.
- Opportunity: Industries with large quantities of organic waste, such as agricultural producers or food manufacturers, can use biomass power systems to generate on-site energy and reduce waste disposal costs. Additionally, biogas plants can convert organic waste into methane, which can be used for electricity generation or heating.
- Wind Power Systems: Wind power can also be used in small-scale power plants, especially in areas with consistent wind resources. Small-scale wind turbines can be installed on-site to supply energy for industrial or commercial operations, particularly in rural areas or facilities with available land.
- Opportunity: Wind power is a viable option for businesses located in regions with strong wind resources. By installing small-scale turbines, industries can diversify their energy sources, reduce their reliance on the grid, and mitigate the impact of fluctuating electricity prices.
- Hydropower for Industrial Use: Small-scale hydropower plants can be an ideal solution for businesses located near rivers or streams. These plants use the natural flow of water to generate electricity and can provide a steady and reliable power source for operations.
- Opportunity: For industries located near water sources, such as sawmills, mining operations, or food processors, small-scale hydropower systems can provide consistent and renewable energy while also reducing environmental impacts compared to traditional large-scale hydropower projects.
9.30.3. Integration with the Grid and Hybrid Systems
- Grid-Tied Small-Scale Power Plants: In many cases, small-scale power plants can be connected to the local grid, allowing businesses to sell excess energy back to the utility or draw power from the grid when needed. This grid connection enhances energy security and offers flexibility in power generation.
- Opportunity: Small-scale power plants connected to the grid can allow businesses to participate in net metering programs, where they receive compensation for excess power generated. Additionally, the grid connection provides a backup energy source in case of renewable generation interruptions.
- Hybrid Systems: Hybrid systems combine multiple energy sources to increase efficiency, reliability, and flexibility. For example, a solar-wind hybrid system can generate energy during both sunny and windy conditions, maximizing energy output. Other hybrid systems might integrate solar with battery storage or biomass with CHP for enhanced energy flexibility.
- Opportunity: By using hybrid systems, businesses can create a reliable, low-cost, and sustainable energy mix that reduces reliance on any single energy source, making their operations more resilient to fluctuations in resource availability or energy market prices.
9.30.4. Operational Challenges and Considerations
- Initial Investment Costs: While small-scale power plants can offer long-term cost savings, they often require a significant initial investment in infrastructure, equipment, and installation. This may be a barrier for some industries, particularly small businesses or those with limited capital.
- Opportunity: Governments, utilities, and financial institutions can support the transition to small-scale energy solutions by offering subsidies, low-interest loans, and tax incentives to offset the initial capital costs. Additionally, energy-as-a-service models, such as power purchase agreements (PPAs) or third-party financing, can help reduce upfront costs.
- Regulatory and Permitting Challenges: Small-scale power plants may face regulatory hurdles, including the need for permits, zoning approvals, and compliance with environmental regulations. Navigating these processes can be time-consuming and complex.
- Opportunity: Governments and regulatory bodies can streamline the permitting process for small-scale energy systems, offering clear guidelines and fast-tracking approvals for businesses looking to invest in renewable energy infrastructure.
- Ongoing Maintenance and Operations: Small-scale power plants require regular maintenance and monitoring to ensure optimal performance. Depending on the technology used, maintenance can involve checking equipment, cleaning panels, servicing turbines, and managing storage systems.
- Opportunity: Implementing remote monitoring systems, predictive maintenance tools, and service contracts can help businesses ensure that their power plants operate efficiently and minimize downtime, leading to longer system lifespans and reduced operational costs.
9.30.5. Conclusion: Small-Scale Power Plants as Catalysts for Industrial and Commercial Sustainability
Small-scale power plants offer a wide range of benefits for industrial and commercial applications, providing businesses with the tools they need to reduce energy costs, enhance reliability, improve sustainability, and foster energy independence. As technological advancements in renewable energy, energy storage, and hybrid systems continue to evolve, the potential for small-scale power plants to play a key role in shaping the future of industrial energy will only grow.
By embracing clean, decentralized energy solutions, industries can not only meet their operational needs but also contribute to broader sustainability goals, reduce their environmental impact, and position themselves as leaders in the green energy transition. Whether through solar, wind, biomass, or hybrid systems, small-scale power plants provide businesses with the flexibility, reliability, and cost-effectiveness they need to thrive in an increasingly energy-conscious world.
9.31. Small-Scale Power Plants for Wastewater Treatment Plants
Wastewater treatment plants (WWTPs) are critical infrastructure in communities and industrial processes, responsible for cleaning and managing wastewater to meet environmental and health standards. These plants can significantly benefit from small-scale power generation systems, both to reduce operational costs and to improve sustainability. Small-scale power plants integrated into wastewater treatment facilities can enhance energy efficiency, reduce dependency on the grid, and even provide valuable byproducts such as heat or biogas that can further support plant operations.
9.31.1. Role of Small-Scale Power Plants in Wastewater Treatment Plants
- Energy Recovery: WWTPs require a large amount of energy to pump, treat, and treat wastewater. Small-scale power plants, especially those using biogas recovery from anaerobic digestion of organic waste in the wastewater, can provide a sustainable and renewable source of energy. This energy can help offset the plant’s operational energy costs.
- Opportunity: Biogas produced from sewage sludge can be captured and used in a combined heat and power (CHP) system, which not only generates electricity but also provides useful heat for the plant’s operations, such as heating tanks and other equipment. The energy recovered can reduce the plant’s reliance on external electricity sources.
- Carbon Footprint Reduction: With increasing environmental concerns, wastewater treatment plants are looking to reduce their carbon emissions. Small-scale power plants that utilize renewable energy technologies such as solar or wind can help offset emissions from the energy-intensive processes in wastewater treatment, such as aeration, pumping, and heating.
- Opportunity: WWTPs can install solar panels on the rooftops of treatment buildings or on nearby land to reduce their reliance on fossil-fuel-based power. This also helps contribute to local renewable energy targets and reduces the environmental footprint of the facility.
- Decentralized Energy for Remote WWTPs: In rural or remote locations where access to the grid may be unreliable or unavailable, small-scale power plants can provide a decentralized and reliable power source for WWTPs. This can be particularly important in places where a continuous supply of electricity is necessary to ensure effective wastewater treatment.
- Opportunity: Using off-grid solar or wind power in combination with energy storage systems can provide a continuous energy supply to WWTPs, ensuring their operations run smoothly without interruptions due to power outages.
9.31.2. Technologies for Small-Scale Power Generation at WWTPs
- Biogas Generation and Utilization: WWTPs naturally generate biogas as a byproduct of the anaerobic digestion process, which breaks down organic waste in the wastewater. This biogas is primarily composed of methane, which can be captured and used to fuel small-scale power plants, typically through internal combustion engines or fuel cells.
- Opportunity: By integrating biogas-powered small-scale CHP systems, WWTPs can self-generate electricity and useful thermal energy to support their operations, reducing reliance on grid electricity. Additionally, excess biogas can be purified and used for other purposes, such as in vehicles or as a backup fuel supply.
- Solar Power: Small-scale solar PV systems are increasingly being used to generate electricity for wastewater treatment plants, especially in areas where sunlight is abundant. Solar panels can be installed on roofs, land near the facility, or even on floating platforms on treatment ponds.
- Opportunity: The integration of solar power with battery storage allows WWTPs to operate autonomously during peak periods, reducing costs and the environmental impact. Solar power can also be used to power auxiliary systems in the plant, including lighting, pumps, and monitoring equipment.
- Wind Power: In areas with consistent wind resources, small-scale wind turbines can supplement the energy needs of a WWTP. Wind turbines can be installed on-site or nearby, helping to provide a continuous supply of energy throughout the year.
- Opportunity: Hybrid systems combining wind and solar can increase the reliability of small-scale power generation, as wind can help supplement solar power when sunlight is unavailable. In windy regions, this could provide a reliable renewable energy source to reduce grid dependency.
- Micro-Hydropower: If the WWTP is located near a river or stream, small-scale micro-hydropower systems can harness the energy from flowing water to generate electricity. These systems can provide consistent, renewable energy and are especially useful for WWTPs in remote locations.
- Opportunity: Micro-hydropower is a reliable and consistent source of energy, providing a steady power supply for WWTPs. Integrating this technology into treatment plants can provide off-grid, renewable energy generation, decreasing operational energy costs and increasing resilience to energy disruptions.
9.31.3. Operational Benefits and Considerations
- Cost Savings: By generating their own power, wastewater treatment plants can significantly lower energy costs, which typically represent a large portion of operational expenses. These savings can help offset the capital costs of implementing small-scale power plants, while also reducing overall operational expenditures.
- Opportunity: Long-term financial savings can be achieved by integrating solar, biogas, or wind energy systems that minimize grid energy purchases. Additionally, power generation systems that produce both heat and electricity, like CHP, can further reduce energy costs by maximizing efficiency.
- Energy Efficiency: Small-scale power plants designed to use renewable resources, such as solar, wind, or biogas, can increase the overall energy efficiency of wastewater treatment plants. By providing locally generated power, these systems help eliminate transmission losses associated with electricity transported over long distances.
- Opportunity: Renewable energy technologies, such as biogas or solar, can be optimized with smart grid technology and real-time monitoring systems to ensure that energy production and consumption are efficiently managed, reducing waste and ensuring stable operations.
- Environmental Impact: Integrating small-scale power plants in WWTPs allows these facilities to reduce their carbon footprint and environmental impact. By relying on renewable energy sources, WWTPs can contribute to local sustainability efforts and align with global decarbonization goals.
- Opportunity: As WWTPs look to become more environmentally responsible, using biogas, solar, and wind power can drastically reduce greenhouse gas emissions compared to conventional grid power, ensuring cleaner, more sustainable wastewater treatment processes.
9.31.4. Challenges and Limitations
- Initial Investment: One of the primary barriers for many WWTPs in adopting small-scale power plants is the capital expenditure required for installation. This includes the cost of renewable energy systems, energy storage, and integration with existing plant infrastructure.
- Opportunity: Governments and utilities can offer grants, incentives, and low-interest loans to offset initial investment costs, encouraging WWTPs to transition to renewable energy solutions. Performance-based incentives could also be implemented, where plants are rewarded based on energy savings or emissions reductions.
- Operational Complexity: Integrating a small-scale power plant into an existing WWTP can require complex engineering and modifications to plant systems. These modifications could include upgrading electrical infrastructure, implementing energy storage, and ensuring proper coordination with the plant’s existing operations.
- Opportunity: With proper planning and collaboration with engineering consultants and technology providers, WWTPs can ensure that their small-scale power plants are seamlessly integrated into their existing infrastructure. Energy management systems can automate power distribution, optimizing energy flow and reducing the complexity of plant operations.
- Intermittency of Renewable Resources: For plants relying on intermittent renewable energy sources like solar and wind, managing the variability in energy supply can be challenging. Without proper storage systems, periods of low energy production could affect the reliability of power to the WWTP.
- Opportunity: To mitigate intermittency, energy storage systems such as lithium-ion batteries or pumped hydro storage can be integrated to store excess energy produced during peak production periods. This stored energy can be used during low-production periods to maintain plant operations without interruption.
9.31.5. Conclusion: Small-Scale Power Plants as Essential Components for Sustainable Wastewater Treatment
Small-scale power plants provide significant potential for wastewater treatment plants to improve their energy efficiency, reduce costs, and contribute to sustainability efforts. By utilizing renewable energy sources such as biogas, solar, wind, and micro-hydropower, these plants can generate the energy needed to operate treatment processes while minimizing environmental impact.
With the right technologies and strategies in place, WWTPs can lower operational costs, reduce greenhouse gas emissions, and become more resilient to power disruptions. As environmental regulations become stricter and energy prices continue to fluctuate, small-scale power plants offer a valuable opportunity for wastewater treatment facilities to enhance their long-term sustainability while ensuring the continued delivery of essential wastewater management services.
9.32. Small-Scale Power Plants for Agriculture and Farm Operations
Agricultural operations, including farms and greenhouses, are energy-intensive businesses that often rely on a steady supply of electricity to power irrigation systems, heating for greenhouses, and various farming machinery. Small-scale power plants offer a sustainable and cost-effective solution to meet these energy needs while also providing an opportunity to lower operating costs, reduce dependency on the grid, and support environmentally friendly farming practices. The integration of renewable energy technologies, such as solar, wind, and biomass, can significantly improve the sustainability and profitability of farm operations.
9.32.1. Role of Small-Scale Power Plants in Agriculture
- Energy Independence: Small-scale power plants allow farmers to reduce their reliance on traditional utility companies, providing them with energy independence. By generating their own power on-site, farmers can ensure a more stable and predictable energy supply, which is especially important in rural or remote areas where grid connectivity can be unreliable or costly.
- Opportunity: Farms that use solar photovoltaic (PV) systems, wind turbines, or biomass generators can power their irrigation pumps, ventilation systems for livestock barns, and other essential equipment without depending on grid power. This is particularly beneficial for farms in areas prone to grid outages or high energy costs.
- Cost Reduction: Energy costs can represent a significant portion of farm operational expenses. Small-scale power plants can help reduce energy bills and provide farmers with a more predictable and manageable electricity cost structure. In the case of renewable energy systems, the long-term savings on energy costs can be substantial, especially once the initial investment in the system has been recouped.
- Opportunity: By utilizing solar energy or biomass systems to generate electricity, farmers can cut their reliance on expensive grid power or diesel generators. Additionally, some farmers may even be able to sell excess power back to the grid, benefiting from net metering or feed-in tariffs.
- Sustainability and Environmental Stewardship: As the agricultural sector faces increasing pressure to reduce its environmental impact, small-scale power plants can provide a sustainable energy solution. By switching to renewable energy sources such as wind, solar, or biomass, farms can lower their carbon footprint, contribute to environmental protection, and meet sustainability goals.
- Opportunity: Farmers transitioning to renewable energy sources can promote themselves as green or sustainable producers, appealing to environmentally conscious consumers. This can also open opportunities for green certifications or participation in carbon offset programs.
9.32.2. Technologies for Small-Scale Power Generation on Farms
- Solar Power Systems: Solar energy is one of the most widely adopted small-scale power generation technologies for farms. Solar panels can be installed on farm rooftops, on the ground, or integrated into existing structures, such as barns or greenhouses. Solar power is ideal for daytime energy use like irrigation, cooling systems, or powering farming machinery.
- Opportunity: Farms can install solar arrays large enough to meet their energy needs and potentially store energy in battery storage systems for use during nighttime or cloudy days. The integration of solar water pumps can also help reduce the energy cost of irrigation.
- Wind Power: Wind turbines are another viable option for farms located in areas with consistent wind resources. Small-scale wind turbines can provide energy for farm operations such as irrigation, lighting, and refrigeration. Wind power is particularly well-suited for large farms or rural properties with abundant open space.
- Opportunity: Farms located in wind-prone areas can install small to medium-sized turbines to help meet their energy demands. A hybrid system that combines wind and solar power can provide a continuous supply of energy, optimizing production during both windy and sunny days.
- Biomass Power: Farms can take advantage of organic waste materials—such as crop residues, wood chips, manure, or food waste—to generate energy through biomass power systems. These systems convert organic materials into biogas or biofuels, which can be used to generate electricity and heat.
- Opportunity: Farms with access to organic waste can integrate biogas generators into their operations, using the waste to produce both electricity and heat. This can power farm machinery, drying equipment, and heating systems for greenhouses or animal shelters. The use of biomass also contributes to waste management, turning organic waste into valuable energy.
- Hydropower for Irrigation: For farms located near flowing water, small-scale micro-hydropower systems can generate electricity. These systems harness the energy of flowing rivers or streams to power farm operations. Micro-hydro systems are highly efficient and can provide consistent energy output.
- Opportunity: Farms with access to a nearby river, stream, or irrigation canal can utilize micro-hydro turbines to generate reliable energy. This system can provide continuous power, which is essential for operations like irrigation systems or livestock watering.
- Combined Heat and Power (CHP): CHP systems allow farmers to generate both electricity and useful heat from the same fuel source, increasing efficiency and reducing waste. These systems can run on biomass, natural gas, or even animal waste. The heat produced can be used for purposes such as greenhouse heating, crop drying, or livestock barn ventilation.
- Opportunity: By utilizing biogas or biomass for CHP systems, farms can generate both energy and heat, improving overall energy efficiency. This is particularly beneficial for farms that need to manage waste heat for greenhouse operations, livestock heating, or water heating.
9.32.3. Operational Benefits and Considerations
- Energy Security and Reliability: Small-scale power plants help farms ensure that they have a reliable and constant energy supply, even in remote or off-grid locations. The consistent availability of energy for critical agricultural processes, such as irrigation or animal husbandry, helps prevent disruptions to farm operations.
- Opportunity: Farmers can implement battery storage systems alongside renewable energy technologies to ensure that power is available when it is needed most. Energy management systems can help monitor energy production and consumption, ensuring that the power supply is optimized.
- Flexibility in Power Use: Small-scale power plants can support a wide range of farm operations. The energy produced can be used for pumps, cooling systems, lighting, and agriculture machinery, while the heat generated can be used for greenhouse heating, crop drying, or livestock heating.
- Opportunity: By integrating solar, wind, and biomass power generation systems with farm operations, farms can create a flexible and diverse energy supply that meets both electricity and heating needs, reducing energy costs and enhancing sustainability.
- Return on Investment (ROI): The initial investment in small-scale power generation systems for farms may be high, but the long-term savings and environmental benefits make it a financially viable option. As energy costs continue to rise, the return on investment (ROI) from generating and using on-site renewable energy becomes increasingly attractive for farmers.
- Opportunity: With the right combination of technologies and incentives, farms can achieve substantial energy cost savings and reduce dependence on grid power, resulting in a strong ROI over the lifespan of the energy systems. Additionally, participation in net metering or feed-in tariffs can help farmers recover costs and generate additional revenue.
9.32.4. Challenges and Limitations
- Initial Capital Investment: Small-scale power plants often require a substantial upfront investment, particularly for renewable energy systems such as solar, wind, or biomass. This can be a barrier for small or medium-sized farms that may not have access to the necessary capital.
- Opportunity: Government grants, subsidies, and low-interest loans are available in many regions to assist farmers in covering the initial costs of renewable energy systems. Additionally, energy-as-a-service models such as power purchase agreements (PPAs) or financing options can help alleviate upfront costs.
- Intermittency of Renewable Energy: Renewable energy sources such as solar and wind are subject to variability and intermittency, meaning that there may be periods when energy production is lower than expected. This could lead to challenges in ensuring a steady and reliable energy supply.
- Opportunity: To mitigate this, farms can integrate energy storage systems such as batteries or thermal energy storage to store excess energy produced during peak periods and provide power during periods of low generation. Hybrid systems combining different renewable sources (solar, wind, biomass) can also help ensure continuous power generation.
- Land Availability: Installing solar panels or wind turbines on farms requires space. For smaller farms or those with limited land, this can present a challenge. Additionally, the size of the renewable energy system must match the farm’s energy needs.
- Opportunity: Farms can use multi-use land for energy generation, such as installing solar panels over existing structures like barns or greenhouses, or utilizing agriculture solar systems (agrivoltaics), where crops and solar panels coexist. This dual-purpose land use can increase energy generation without taking away from productive agricultural activities.
9.32.5. Conclusion: Small-Scale Power Plants as Key Drivers of Agricultural Sustainability
Small-scale power plants present a significant opportunity for the agricultural sector to reduce energy costs, increase sustainability, and improve energy independence. By adopting renewable energy technologies such as solar, wind, biomass, and micro-hydropower, farms can improve operational efficiency, enhance sustainability, and help mitigate the environmental impact of agriculture.
The integration of small-scale power systems can help farmers adapt to changing energy demands, lower operational costs, and reduce dependence on fossil fuels. Moreover, renewable energy solutions can support the long-term viability of agricultural operations by increasing resilience to energy price fluctuations and power disruptions. With the right technologies and financing models, small-scale power plants can become an integral part of modern farming practices, supporting both economic growth and environmental stewardship.
9.33. Small-Scale Power Plants for Rubber Manufacturing Plants
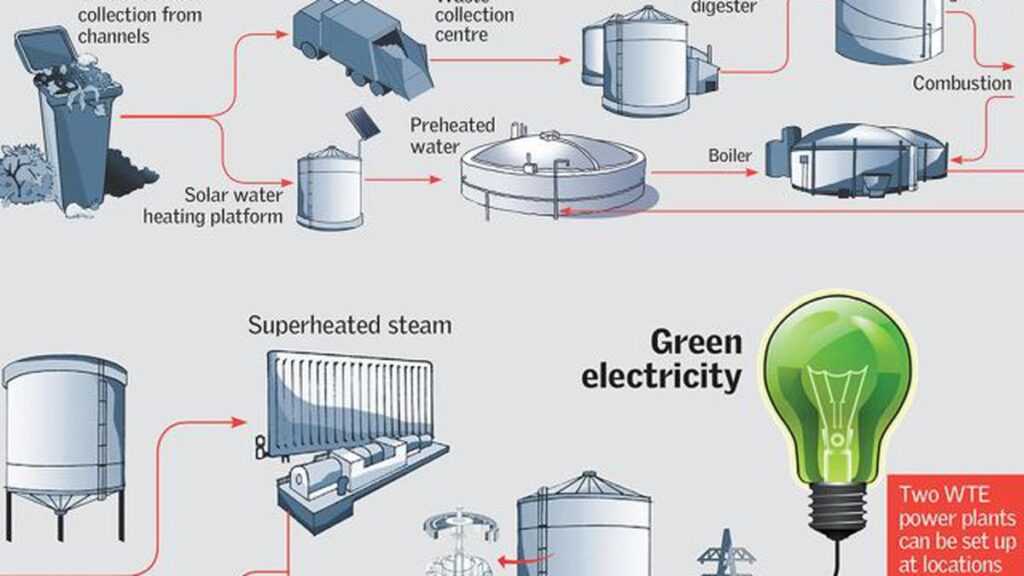
Rubber manufacturing plants, which produce a wide range of rubber products for industries such as automotive, healthcare, and construction, often require a substantial amount of energy for their operations. These plants use energy-intensive processes, including mixing, molding, vulcanization, and extrusion, all of which require stable and reliable electricity. Small-scale power plants can provide an efficient and sustainable solution to meet the energy needs of rubber manufacturing facilities, helping reduce operational costs, improve energy resilience, and support environmental sustainability goals.
9.33.1. Role of Small-Scale Power Plants in Rubber Manufacturing
- Energy Supply for Energy-Intensive Processes: Rubber manufacturing involves several energy-consuming processes such as vulcanization, molding, extrusion, and mixing. These processes require reliable and consistent power to ensure product quality and production efficiency. Small-scale power plants can provide the necessary electricity, reducing the dependency on grid power and ensuring operational continuity.
- Opportunity: By integrating renewable energy systems, such as biogas, solar, or wind, rubber plants can reduce their reliance on the grid and generate energy on-site to power these critical processes. This can help ensure continuous production even during grid outages, particularly in regions where electricity supply may be unreliable.
- Cost Reduction and Energy Efficiency: Energy costs can be a significant part of the operational expenses for rubber manufacturing plants. Small-scale power generation systems, particularly those that utilize renewable energy sources, can help reduce energy costs over the long term. Additionally, integrating systems like combined heat and power (CHP) can improve the overall energy efficiency by generating both electricity and useful heat from the same energy source.
- Opportunity: Small-scale biomass-based CHP systems could be integrated into rubber manufacturing plants, using organic waste from production processes (e.g., latex waste, rubber dust, or biomass from other industries) to generate electricity and heat. This can lead to significant cost savings while reducing the carbon footprint of the plant.
- Sustainability and Green Manufacturing: Rubber manufacturing is under increasing scrutiny due to its environmental impact, particularly in relation to carbon emissions and waste generation. Small-scale power plants based on renewable energy sources, such as solar, biogas, or biomass, can significantly reduce the plant’s carbon footprint and align the plant with sustainability initiatives.
- Opportunity: Transitioning to renewable energy sources for power generation can help rubber plants meet environmental goals, comply with stricter emissions regulations, and appeal to environmentally conscious customers. These renewable sources help reduce reliance on fossil fuels, lowering emissions associated with traditional grid electricity.
9.33.2. Technologies for Small-Scale Power Generation in Rubber Manufacturing
- Biomass Power Systems: Rubber manufacturing plants can benefit from biomass-based power generation, especially if they produce a significant amount of organic waste. Biomass, such as rubber waste, wood chips, or other agricultural residues, can be converted into biogas or biomass briquettes, which can then be used to generate electricity and heat.
- Opportunity: Rubber plants can use biomass boilers or biogas digesters to produce energy on-site. These systems could use waste products such as latex slurry, rubber dust, or agricultural waste to produce bioenergy, which could help meet both electricity and heating needs for production processes, such as vulcanization and drying.
- Solar Power: Solar photovoltaic (PV) systems are increasingly being adopted by industrial plants, including rubber manufacturing facilities. Solar panels can be installed on rooftops or open land to generate electricity, reducing the need for grid power. Solar systems can complement energy demand during daylight hours and, when paired with energy storage, can provide reliable power even after sunset.
- Opportunity: Rubber manufacturing plants can install large-scale solar arrays to generate electricity for the production line. With proper energy storage systems, excess power can be stored during sunny periods and used during non-daylight hours, ensuring a stable energy supply for operations.
- Wind Power: For rubber plants located in areas with consistent wind resources, small-scale wind turbines can be installed to generate electricity. These turbines can supplement the power needs of the plant, especially for non-critical processes that require lower energy demands.
- Opportunity: In regions with good wind conditions, wind turbines could provide a steady, renewable energy source for rubber plants. These turbines could be integrated with solar systems or biomass generators in hybrid setups to increase overall energy resilience.
- Combined Heat and Power (CHP): CHP systems that use biomass or natural gas as a fuel source can offer dual benefits by generating both electricity and useful heat. Heat generated in the process can be used for processes such as vulcanization, which requires high temperatures, or for heating the plant.
- Opportunity: Rubber manufacturing processes, such as vulcanization, require substantial amounts of heat. A biomass-based CHP system could produce the necessary heat for these processes while simultaneously generating electricity, improving the energy efficiency of the entire plant.
9.33.3. Operational Benefits and Considerations
- Energy Security: By adopting small-scale power plants, rubber manufacturing plants can enhance their energy security. Power disruptions can lead to costly production delays, so having on-site power generation capabilities provides a level of resilience against grid instability or outages.
- Opportunity: Small-scale power systems such as biomass-based generators or solar-powered systems can help ensure that production processes run without interruptions. In remote areas with poor grid infrastructure, these systems can become essential to maintaining production continuity.
- Improved Operational Efficiency: Small-scale power plants, particularly those using CHP technology, can significantly enhance energy efficiency by simultaneously generating electricity and useful heat. This reduces waste and improves the plant’s overall energy use.
- Opportunity: The integration of CHP systems in rubber manufacturing plants can provide a cost-effective way to meet both electricity and heating needs, reducing fuel consumption and improving energy performance.
- Cost Savings: Small-scale power plants can help rubber plants reduce their operational costs by minimizing dependence on grid electricity. Over time, the savings from generating power on-site can help offset the capital costs of the initial investment in renewable energy systems.
- Opportunity: Rubber plants that install biomass systems, solar PV, or wind turbines can reduce their utility bills. In regions with high energy costs, these systems can offer significant long-term savings, contributing to a more sustainable financial model.
- Regulatory Compliance: Rubber manufacturing plants are subject to increasing environmental regulations, especially regarding carbon emissions. Using small-scale renewable power generation can help the plant meet these regulations by reducing reliance on fossil-fuel-based energy.
- Opportunity: By transitioning to biogas, solar, or wind power, rubber plants can significantly reduce their carbon footprint, positioning themselves as leaders in sustainability. This can also improve their reputation with customers who prioritize green manufacturing practices.
9.33.4. Challenges and Limitations
- High Initial Investment: The installation of small-scale power plants requires a significant capital investment, especially for renewable energy systems. This can be a barrier for rubber manufacturing plants, particularly small- and medium-sized operations that may not have the financial resources for large upfront costs.
- Opportunity: Governments and local utilities may offer incentives, tax breaks, or subsidies to reduce the financial burden of installing renewable energy systems. Additionally, financing programs such as power purchase agreements (PPAs) or leasing options can help make these systems more affordable.
- Land and Space Requirements: Installing renewable energy systems such as solar arrays or wind turbines requires space, which may be a limiting factor for rubber plants located in urban or congested areas. Similarly, biomass-based systems may require a supply of feedstock, which could be difficult to procure.
- Opportunity: Rubber plants located on large plots of land can use multi-purpose spaces for renewable energy installations. For example, solar panels can be installed on rooftops or unused land to reduce the land footprint. Additionally, partnerships with nearby agricultural or forestry operations could help secure a consistent biomass supply.
- Intermittency of Renewable Energy: Renewable energy sources like solar and wind are intermittent, meaning they do not always produce power when it is needed most. Rubber plants that rely heavily on these sources may need to manage variability in energy production.
- Opportunity: Integrating battery storage systems with solar or wind energy can store excess energy produced during peak generation times and release it when energy demand is high or generation is low. Additionally, combining biomass with renewable systems can provide a more stable, reliable energy mix.
9.33.5. Conclusion: Small-Scale Power Plants for Rubber Manufacturing
Small-scale power plants can significantly benefit rubber manufacturing plants by reducing energy costs, improving energy security, and supporting environmental sustainability. Technologies such as biomass, solar, wind, and combined heat and power (CHP) can provide reliable and renewable energy for the energy-intensive processes involved in rubber production. While challenges such as initial capital costs and space requirements exist, the long-term operational savings, environmental benefits, and potential for energy independence make small-scale power plants a valuable investment for rubber manufacturing facilities.
By integrating these renewable energy systems, rubber manufacturing plants can reduce their carbon footprint, improve their resilience to energy price fluctuations, and create more sustainable production practices. With the right financial and technical support, small-scale power plants can become a cornerstone of efficient, green manufacturing in the rubber industry.
9.33.6. Key Considerations for Implementing Small-Scale Power Plants in Rubber Manufacturing
- Energy Management Systems: Integrating energy management systems (EMS) into small-scale power plants is crucial for optimizing energy generation and consumption. These systems monitor energy production and usage, identify inefficiencies, and ensure that renewable energy sources are maximally utilized. EMS can also control the operation of backup power systems (such as grid power or generators) when renewable energy production is insufficient.
- Opportunity: Implementing an EMS allows rubber plants to better balance energy demand with available generation capacity, reducing reliance on non-renewable sources and minimizing energy waste. This system can automatically switch between grid power, renewable sources, and stored energy, ensuring a consistent and reliable power supply.
- Scalability of Systems: The scalability of small-scale power generation systems is another key factor in their suitability for rubber manufacturing plants. Depending on the size and energy needs of the plant, different solutions can be tailored to suit the operational requirements, allowing for growth or adaptation to changing energy demands.
- Opportunity: Rubber manufacturing plants can start with smaller installations and expand as their energy needs grow. For example, they may begin with a solar PV system or a biogas CHP unit and gradually increase capacity over time as energy demand increases or as new processes are introduced. This allows for flexibility in adapting to future needs and financial considerations.
- Integration with Existing Infrastructure: The integration of small-scale power generation systems into existing infrastructure is essential to minimize disruption to ongoing production processes. Careful planning is required to ensure that renewable energy installations do not interfere with the manufacturing operations and that power generation systems are seamlessly integrated into the plant’s electrical grid.
- Opportunity: Rubber plants can work with energy experts to design systems that are compatible with existing electrical and mechanical infrastructure. For example, solar panels can be installed on rooftops or unused land, and biomass systems can be integrated into existing waste disposal operations, reducing installation costs and complexity.
- Technology Optimization: While small-scale power systems like solar, wind, and biomass are relatively mature technologies, continuous optimization is necessary to ensure they are performing at their best. Technological advancements, such as improved efficiency of solar panels, biogas digesters, and wind turbines, as well as the development of smart grids and battery storage systems, can enhance the overall effectiveness of power generation.
- Opportunity: Rubber plants can adopt the latest technologies in solar energy storage, energy-efficient wind turbines, or next-generation biomass boilers to improve the efficiency and reliability of their energy supply. Additionally, integrating smart grid systems can allow for real-time monitoring and dynamic adjustment of energy usage based on demand and supply fluctuations.
9.33.7. Case Studies and Examples
- Case Study 1: Biogas Power for Rubber Manufacturing in Southeast Asia
A rubber manufacturing facility in Southeast Asia implemented a small-scale biogas-based CHP system using organic waste from their production process, including latex residues, rubber dust, and other organic waste from the surrounding area. The biogas system produces both electricity and heat, which is used for high-temperature processes like vulcanization. This system significantly reduced the plant’s dependence on external electricity sources and cut down on energy costs by utilizing local waste resources. Furthermore, the plant was able to lower its carbon emissions by reducing reliance on fossil fuels and implementing a more sustainable waste management approach.- Opportunity: Other rubber manufacturing plants in regions with abundant organic waste could replicate this model, leveraging biomass and biogas systems to create a more circular production process while cutting energy costs and enhancing sustainability.
- Case Study 2: Solar and Wind Hybrid System for Rubber Plant in Europe
A rubber manufacturing plant in a wind-prone region of Europe installed a hybrid solar and wind energy system to meet its energy demands. The plant uses solar PV panels on the roofs of its buildings and wind turbines on nearby land to generate electricity. By combining both energy sources, the plant reduces its dependence on grid electricity, ensuring a continuous power supply even during periods of low sunlight or wind. The plant also installed battery storage systems to store excess energy produced during peak generation times, which helps meet energy needs during off-peak hours.- Opportunity: Rubber plants in areas with diverse weather patterns, such as sunny and windy regions, can optimize the use of solar and wind energy in tandem to create a more reliable and efficient energy supply. Hybrid systems can reduce energy costs while ensuring a consistent power supply.
9.33.8. Future Trends and Innovations in Small-Scale Power for Rubber Manufacturing
- Energy Storage Solutions: As renewable energy systems become more common, energy storage technologies are evolving to support intermittent energy sources like solar and wind. Battery storage systems and thermal storage will play a significant role in ensuring continuous energy supply and providing flexibility for rubber manufacturing plants. By storing excess energy when production is high and releasing it during demand peaks, these systems help smooth out fluctuations in energy generation and consumption.
- Opportunity: The development of high-efficiency batteries and thermal energy storage systems will make it easier for rubber manufacturing plants to optimize energy generation and consumption. Investing in these technologies will allow plants to create a more resilient and flexible energy system, maximizing the efficiency of their renewable power sources.
- Smart Grid Integration: The integration of small-scale power systems with smart grids will provide rubber plants with more control over their energy usage. Smart grids allow for real-time monitoring of energy production and consumption, enabling plants to adjust power usage to match energy availability, reduce waste, and optimize system performance.
- Opportunity: Rubber plants can benefit from adopting smart grid technologies that help monitor and manage energy flows efficiently. These systems can help reduce energy costs, improve system reliability, and ensure that the renewable energy generated is being used effectively.
- Advanced Biomass Technologies: Innovations in biomass energy production, such as more efficient biogas digesters or waste-to-energy technologies, will provide further opportunities for rubber manufacturing plants to reduce waste and improve energy efficiency. Biomass gasification and pyrolysis technologies can convert a wider range of organic waste into energy, expanding the possibilities for utilizing waste materials.
- Opportunity: Rubber manufacturing plants can explore new biomass technologies to increase the efficiency of waste-to-energy systems, reduce their dependence on fossil fuels, and lower energy costs. These technologies will help further align rubber plants with circular economy principles, turning waste materials into valuable resources.
9.33.9. Conclusion: Moving Toward a Sustainable Future
Small-scale power plants represent an important step for rubber manufacturing plants to increase energy resilience, reduce costs, and enhance environmental sustainability. By integrating renewable energy technologies such as biomass, solar, and wind, along with combined heat and power (CHP) systems, rubber plants can meet their energy needs in a more cost-effective and environmentally friendly manner.
Incorporating energy-efficient solutions such as energy storage, smart grids, and advanced biomass technologies will further optimize these systems, enabling plants to reduce reliance on traditional grid power and increase operational efficiency. With the growing demand for sustainable manufacturing practices, rubber manufacturing plants that adopt small-scale power generation will be better positioned to meet environmental goals, reduce their carbon footprint, and improve long-term financial performance.
9.33.10. Steps for Implementing Small-Scale Power Plants in Rubber Manufacturing Plants
To successfully integrate small-scale power plants into rubber manufacturing facilities, careful planning and execution are required. Here are the key steps in implementing a small-scale power generation system:
- Conduct an Energy Audit:
- Before proceeding with any installation, an energy audit should be conducted to assess the facility’s energy consumption patterns. This audit will help determine the energy needs of the plant, identify peak consumption periods, and understand the efficiency of existing systems.
- The energy audit will guide the selection of the most appropriate power generation technology based on the plant’s specific needs, production schedule, and the potential for renewable energy generation.
- Opportunity: Through a detailed audit, rubber manufacturing plants can uncover opportunities to reduce energy waste and identify areas where small-scale power plants can deliver the most value.
- Evaluate Renewable Energy Resources:
- Assess the availability of renewable resources like solar radiation, wind speeds, or organic waste (for biomass) to determine the viability of different technologies.
- For instance, solar energy is highly feasible in regions with abundant sunlight, while wind power can be a better option in wind-rich areas. Biomass can be effective if the plant generates or has access to organic waste materials.
- Opportunity: Rubber plants can tailor their small-scale power systems to local conditions. For example, in tropical regions with abundant biomass waste, a biomass CHP system could be highly effective, while in regions with high sunlight, solar PV systems would be more suitable.
- Select the Appropriate Technology:
- Based on the findings from the energy audit and renewable resource evaluation, select the most appropriate power generation technology or combination of technologies. A hybrid approach may be ideal in many cases, combining solar, wind, biomass, or CHP systems to create a resilient and diverse energy portfolio.
- For example, a solar PV system might meet daytime energy needs, while biomass CHP could provide heat and electricity during non-sunny hours.
- Opportunity: Rubber manufacturing plants can choose a technology mix that not only meets their current energy demands but also provides flexibility to scale up as the plant grows or energy needs fluctuate.
- Design and Integrate the Power System:
- The selected power generation system must be designed to integrate seamlessly with the plant’s existing infrastructure. This involves determining the size of the system, connection points to the electrical grid, and any necessary modifications to accommodate renewable energy sources.
- For instance, biomass-based systems may require modifications to waste management processes, while solar or wind systems may need specific installation areas (such as rooftops or open land) to maximize energy generation.
- Opportunity: Integrating renewable power systems with the plant’s infrastructure can be done in phases to minimize disruptions to ongoing production. This allows for smooth transitions and ensures that each energy source is deployed effectively.
- Obtain Financing and Incentives:
- Small-scale power systems often come with significant upfront capital costs. It’s important to secure financing, which could include loans, grants, or equity investments. Many governments offer incentives, such as tax breaks, subsidies, or green funding programs, to support the adoption of renewable energy technologies.
- Opportunity: Rubber manufacturing plants should explore green financing options, such as low-interest loans for renewable energy projects or power purchase agreements (PPAs) that allow the plant to lease the power generation equipment and only pay for the energy it consumes. Additionally, government incentives can significantly reduce the financial burden of implementing renewable energy systems.
- Install the Power Generation System:
- Once financing is in place, the installation of the power generation system can begin. This involves procuring the necessary equipment, hiring contractors, and coordinating logistics for the installation of components such as solar panels, wind turbines, or biomass boilers.
- It is essential to ensure that installation is completed according to safety standards and operational requirements. Additionally, proper testing and commissioning of the system should be conducted before it is integrated into the plant’s energy supply chain.
- Opportunity: Rubber plants can work with experienced renewable energy contractors and project managers to ensure a smooth installation process. It’s crucial to have clear timelines and coordination to minimize downtime during the installation phase.
- Monitor and Optimize Performance:
- Once the system is operational, continuous monitoring is essential to ensure that the power generation system is functioning as expected. Regular maintenance and performance analysis can identify potential issues, optimize energy production, and ensure maximum efficiency.
- An energy management system (EMS) can be used to track energy generation, consumption, and storage, allowing plant managers to make real-time adjustments and optimize energy usage.
- Opportunity: Implementing an EMS or using remote monitoring tools can help the plant identify energy inefficiencies and adjust operations for optimal performance. This system also provides data that can be used for troubleshooting and long-term improvements.
- Evaluate and Scale:
- After the system has been in operation for a certain period, it’s important to assess its performance. Evaluate whether the energy generation system meets the plant’s energy needs, and if there are areas for improvement or expansion.
- If energy consumption has increased or if there is a demand for additional power, the plant may consider scaling the system up by adding more solar panels, wind turbines, or increasing the biomass feedstock capacity.
- Opportunity: As the plant’s energy needs evolve, additional renewable generation capacity can be added to the system, allowing for continuous improvement in energy efficiency. The integration of battery storage systems can also be explored to further optimize energy usage.
9.33.11. Key Benefits for Rubber Manufacturing Plants
- Reduced Energy Costs: By generating power on-site using renewable energy sources, rubber manufacturing plants can significantly reduce their electricity bills. Over time, this can lead to substantial cost savings and improved profitability.
- Increased Energy Security: Small-scale power generation systems, especially those that utilize renewable energy, reduce dependence on external grid power. This provides the plant with greater energy security and resilience against disruptions or power outages.
- Environmental Benefits: Renewable energy technologies, such as solar, wind, and biomass, help lower the plant’s carbon footprint by reducing reliance on fossil fuels. This aligns with sustainability goals and supports the plant’s compliance with environmental regulations.
- Brand Reputation and Customer Appeal: Adopting renewable energy systems improves the company’s brand reputation as an environmentally responsible manufacturer. It may also appeal to customers who are increasingly prioritizing sustainability in their supply chain decisions.
- Operational Flexibility: Hybrid systems that combine different renewable energy sources (e.g., solar and wind or biomass and solar) provide flexibility and allow for energy generation during different periods of the day and in varying weather conditions. This helps ensure a steady, reliable energy supply.
- Compliance with Regulations: As regulations around carbon emissions and energy efficiency become stricter, rubber manufacturing plants adopting renewable energy systems can stay ahead of regulatory requirements, reducing potential compliance costs and risks.
9.33.12. Challenges and Mitigation Strategies
While small-scale power plants offer numerous advantages, there are also challenges that rubber manufacturing plants must address:
- High Initial Capital Investment: The upfront costs for renewable energy systems can be high. However, these costs can be mitigated through government incentives, financing options, or power purchase agreements (PPAs) that reduce initial investment requirements.
- Intermittency of Renewable Energy: Solar and wind energy systems are subject to variability, which can lead to periods of low energy generation. Integrating energy storage systems like batteries or thermal storage can help smooth out these fluctuations and ensure a consistent power supply.
- Integration with Existing Infrastructure: Integrating renewable energy systems into existing manufacturing infrastructure requires careful planning. However, partnering with experienced energy consultants and engineers can ensure smooth integration and minimize operational disruptions.
9.33.13. Conclusion: Small-Scale Power Plants for Rubber Manufacturing
In conclusion, implementing small-scale power plants in rubber manufacturing plants offers a pathway toward cost savings, energy security, and environmental sustainability. Technologies such as biomass, solar, wind, and combined heat and power (CHP) systems can significantly enhance the energy efficiency of rubber production processes while reducing the plant’s dependence on grid electricity.
With proper planning, financing, and technology integration, rubber manufacturing plants can benefit from reliable, renewable energy sources that support long-term operational growth and environmental stewardship.
9.34. Small-Scale Power Plant for Textile Factories
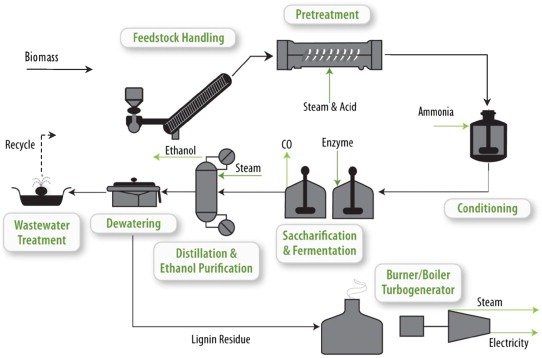
Textile factories are energy-intensive industries that require large amounts of electricity to power machinery, lighting, heating, and cooling systems. Small-scale power plants can help textile manufacturers reduce their energy costs, increase sustainability, and ensure more reliable energy supplies. In this section, we will explore the different types of small-scale power plants suitable for textile factories and the considerations for their implementation.
9.34.1. Energy Demands of Textile Factories
Textile factories use significant amounts of energy, especially for operations like spinning, weaving, dyeing, finishing, and drying. Energy needs can fluctuate depending on the scale of operations, the machinery used, and the type of textile being produced. Some of the key energy demands in textile factories include:
- High-Temperature Processes: Dyeing and finishing require high temperatures, which are energy-intensive processes.
- Machinery Operation: Spinning, weaving, and knitting machines consume a large amount of electrical energy for their operation.
- Lighting: Large factories often have extensive lighting systems, both indoors and outdoors.
- Compressed Air Systems: These systems are used for cleaning and for powering certain machines and are another source of energy consumption.
- Heating, Ventilation, and Air Conditioning (HVAC): HVAC systems are critical for maintaining a comfortable environment and preventing damage to sensitive textile materials during production.
A reliable and cost-effective power generation system can help textile factories meet these high energy demands while improving efficiency and sustainability.
9.34.2. Potential Power Solutions for Textile Factories
Small-scale power plants for textile factories should ideally offer a combination of cost savings, reliability, and environmental benefits. The following renewable energy sources can be considered:
- Biomass Energy:
- Biomass is an attractive option for textile factories, especially those located near agricultural or forestry operations, which can provide a steady supply of organic materials. These materials could include wood chips, agricultural residues, or even fabric waste from the factory itself. Biomass power plants can generate both electricity and heat, which is highly valuable for textile production processes such as dyeing and drying.
- Combined Heat and Power (CHP) systems can be implemented to maximize the efficiency of biomass power plants, using the heat generated for industrial processes.
- Opportunity: A textile factory can turn its own organic waste into a valuable energy source, reducing waste disposal costs while generating clean energy.
- Solar Power:
- Solar PV (Photovoltaic) systems can be deployed to harness the sun’s energy and generate electricity. Solar power can be particularly effective in regions with abundant sunlight. Solar panels can be installed on factory rooftops or open land adjacent to the facility, depending on available space.
- Solar power is best suited for providing electricity during the day when energy demand in textile factories is at its peak.
- Opportunity: By installing a solar PV system, textile factories can reduce their reliance on grid electricity during the day and cut down on electricity bills.
- Wind Energy:
- If the textile factory is located in a wind-rich area, small wind turbines can be a viable option to generate renewable electricity. Wind turbines are typically more effective in locations where wind speeds are consistent.
- Opportunity: In regions with strong winds, wind turbines can complement solar power generation, especially during periods when solar energy is not available, providing a consistent energy source.
- Energy Storage Systems:
- Energy storage technologies such as batteries or thermal storage systems can be integrated with solar, wind, or biomass power plants to store excess energy generated during peak production times. This stored energy can then be used during periods of high demand or when renewable generation is low.
- Opportunity: By incorporating energy storage, textile factories can reduce their reliance on grid electricity during evening or nighttime hours, providing a 24/7 renewable energy supply.
- Cogeneration (Combined Heat and Power – CHP):
- CHP systems combine power generation with heat recovery. Biomass or natural gas can be used to generate electricity while simultaneously capturing the waste heat produced during this process to use in factory operations.
- Opportunity: CHP systems offer a highly efficient solution for textile factories, as they can use the same fuel for both electricity generation and heating purposes.
9.34.3. Key Considerations for Implementing Small-Scale Power Plants in Textile Factories
Several key factors must be considered when implementing small-scale power plants in textile factories:
- Energy Needs Assessment:
- A thorough energy audit must be conducted to assess the factory’s energy requirements and identify opportunities for energy savings. Understanding peak demand times, base load requirements, and specific energy-intensive processes will help determine the right mix of power generation technologies.
- Opportunity: Conducting an energy audit will provide insight into areas where energy consumption can be optimized or shifted to renewable sources. For example, non-essential systems can be powered during off-peak hours to reduce energy costs.
- Integration with Existing Infrastructure:
- The power generation system must be integrated with the factory’s existing electrical grid, ensuring that it complements current energy use patterns without causing disruptions. For instance, during peak energy demand periods, the power system should seamlessly feed into the factory’s grid, and energy storage can be used when renewable generation is low.
- Opportunity: Hybrid systems that combine solar, wind, and biomass energy with energy storage can provide a stable and reliable power supply while reducing dependence on external energy sources.
- Financing and Incentives:
- Implementing small-scale renewable energy systems can be costly, and financing options must be explored. Government incentives, such as tax credits, subsidies, or low-interest loans, are available in many regions to encourage the adoption of renewable energy.
- Opportunity: Textile factories can take advantage of these financial incentives to reduce the upfront cost of renewable energy installations. Green financing options and Power Purchase Agreements (PPAs) are also viable alternatives for reducing financial barriers.
- Scalability and Flexibility:
- Power systems should be scalable, allowing for expansion as the factory’s energy needs grow. Modular renewable energy technologies such as solar PV or biomass boilers can be expanded over time, allowing for more capacity as production increases.
- Opportunity: By selecting modular and scalable technologies, textile factories can start with smaller systems and expand as their needs evolve, optimizing their investment over time.
- Maintenance and Operation:
- Regular maintenance is required to ensure the continued efficient operation of renewable energy systems. Monitoring systems should be installed to track performance and identify issues early. Trained personnel should also be in place for regular upkeep of equipment.
- Opportunity: Investing in predictive maintenance technologies and employing remote monitoring systems can help textile factories optimize operations and minimize downtime.
9.34.4. Case Studies and Examples
- Case Study 1: Solar Power at a Textile Factory in India
A textile factory in India installed a solar PV system on the rooftop of its facility, generating a significant portion of its electricity needs. By leveraging the abundant sunlight, the factory reduced its dependence on grid electricity during the day, leading to reduced operational costs. Additionally, the factory was able to sell excess energy back to the grid, generating additional revenue.- Opportunity: Other textile factories in regions with high solar potential can replicate this model to reduce their energy costs and contribute to grid stability.
- Case Study 2: Biomass CHP for Textile Factory in Southeast Asia
A textile factory in Southeast Asia implemented a biomass-based combined heat and power (CHP) system using local agricultural residues such as rice husks. The biomass system generated both heat and electricity for the factory, reducing reliance on external electricity sources and helping to cut costs. The heat generated was used for high-temperature processes like dyeing, while the electricity powered machinery and lighting.- Opportunity: Other textile factories in rural areas with access to biomass feedstock can implement CHP systems to improve energy security and reduce waste.
9.34.5. Future Trends and Innovations
- Smart Grids and Energy Management Systems (EMS): The integration of smart grids and energy management systems (EMS) in textile factories will allow for real-time monitoring and optimization of energy generation and consumption. These systems can balance energy loads, ensuring that renewable energy is used effectively and that excess energy is stored or sold back to the grid.
- Opportunity: By adopting smart grids and EMS, textile factories can optimize energy usage, reduce waste, and increase the efficiency of their renewable energy systems.
- Advanced Energy Storage Solutions: As renewable energy technologies become more prevalent, the need for advanced energy storage solutions such as solid-state batteries or thermal storage will grow. These systems can store excess energy for later use, increasing the reliability and resilience of the factory’s energy supply.
- Opportunity: By incorporating advanced storage solutions, textile factories can ensure a consistent energy supply, even during periods of low renewable energy generation.
9.34.6. Conclusion: The Path Toward Sustainable Power for Textile Factories
In conclusion, small-scale power plants offer textile factories the opportunity to reduce energy costs, increase sustainability, and enhance energy security. By adopting renewable energy technologies such as biomass, solar, and wind, textile manufacturers can significantly decrease their reliance on grid power while also lowering their environmental impact. With proper planning, financing, and integration, small-scale power systems can play a pivotal role in creating more sustainable and efficient textile manufacturing operations.
9.34.7. Steps for Implementing Small-Scale Power Plants in Textile Factories
To successfully implement small-scale power plants in textile factories, a step-by-step approach is essential. Below are the key phases and actions that should be followed for smooth installation, integration, and operation:
- Conduct an Energy Audit and Feasibility Study:
- The first step is to assess the factory’s energy consumption, identify areas of high energy demand, and determine the potential for energy savings. The energy audit should also include an analysis of the factory’s current energy sources, peak demand times, and opportunities to reduce consumption.
- During this phase, a feasibility study for renewable energy solutions should be carried out, considering factors like available space, renewable resource availability (solar, wind, biomass), and the technical and economic feasibility of various options.
- Opportunity: Conducting an in-depth energy audit will help identify areas where renewable energy systems can be integrated, ensuring that the chosen technology addresses the most critical energy needs of the factory.
- Evaluate Renewable Resource Availability:
- For solar and wind energy, it is essential to evaluate local solar irradiance (for solar power) and wind patterns (for wind energy) to ensure these resources are viable. A site assessment will help identify the most suitable location for the installation of solar panels, wind turbines, or biomass processing equipment.
- For biomass, it is important to evaluate the availability of local organic materials such as agricultural residues, wood chips, or textile waste. Proximity to these resources will influence the practicality and cost-effectiveness of a biomass-based power plant.
- Opportunity: A thorough evaluation of local resources will ensure that the factory selects an energy generation system that leverages abundant and cost-effective resources, increasing the overall cost-efficiency and sustainability of the power system.
- Design the System and Integrate with Existing Infrastructure:
- Once the renewable energy resources are evaluated, the next step is designing the power generation system, including the size and layout of solar panels, wind turbines, or biomass systems. The system design should also account for integration with the existing electrical grid and the factory’s energy demand profile.
- A key aspect of this step is ensuring that the new energy systems are seamlessly connected to the factory’s internal power grid to avoid disruptions and maintain reliable energy supply. In some cases, a hybrid system may be required, combining multiple renewable energy sources (such as solar and biomass) with energy storage solutions to ensure continuous power generation.
- Opportunity: Using modular designs for systems like solar panels and biomass boilers allows for scalable and flexible installations that can grow with the factory’s energy needs over time.
- Secure Financing and Explore Incentives:
- Given the initial investment costs for renewable energy systems, securing financing is a crucial step. Explore financing options such as green loans, subsidies, tax incentives, or power purchase agreements (PPAs) offered by governments and financial institutions to support renewable energy investments.
- Additionally, the factory should research available incentives for renewable energy installations, such as tax credits or grants, which can significantly reduce upfront costs.
- Opportunity: By securing green financing options or participating in energy subsidy programs, textile factories can reduce the financial burden of implementing renewable energy systems and speed up their return on investment.
- Procure Equipment and Hire Skilled Contractors:
- Once financing is in place, the next step is to procure the necessary equipment (solar panels, wind turbines, biomass boilers, energy storage systems, etc.) and hire contractors with experience in installing renewable energy systems. This phase includes ordering and transporting equipment, as well as finalizing contracts for installation and ongoing maintenance.
- Opportunity: Working with experienced contractors ensures that the systems are installed correctly and comply with industry standards, preventing issues down the line.
- Install the Renewable Energy System:
- The installation phase involves the physical setup of the power generation equipment and integration into the factory’s energy infrastructure. This may include mounting solar panels on rooftops, constructing wind turbines on-site, or setting up biomass processing facilities.
- During installation, the factory must ensure that the safety standards are followed, and there is minimal disruption to production activities. Additionally, energy storage systems should be connected at this stage to store excess energy generated by renewable sources.
- Opportunity: Installing the system during scheduled plant downtimes or in phases can minimize disruptions to production and allow for smooth integration with existing operations.
- Test the System and Train Personnel:
- Once the system is installed, thorough testing should be conducted to ensure that everything is functioning as planned. This includes checking that energy is being generated and fed into the factory grid, and that energy storage systems are working correctly.
- Factory personnel must be trained to operate and maintain the system, including understanding how to monitor energy generation, conduct basic troubleshooting, and perform regular maintenance.
- Opportunity: By offering staff training programs, textile factories can ensure that their employees are equipped to handle and optimize the renewable energy system, leading to long-term efficiency gains.
- Ongoing Monitoring, Maintenance, and Optimization:
- After the system is operational, continuous monitoring is necessary to track performance, identify inefficiencies, and ensure that the system is meeting the factory’s energy needs. Maintenance schedules should be established to keep the equipment in optimal condition and prevent breakdowns.
- Additionally, it is important to regularly evaluate the system’s performance and make adjustments to improve efficiency, such as upgrading energy storage capacity or adding more renewable generation capacity.
- Opportunity: Using smart monitoring tools and predictive maintenance systems can help identify potential issues early and optimize energy usage, reducing downtime and improving system performance.
- Evaluate Performance and Scale Up if Necessary:
- After the system has been operational for some time, the factory should assess whether it is meeting its energy needs. If energy consumption increases or if renewable generation capacity is insufficient, the system can be scaled up by adding more renewable energy resources (e.g., additional solar panels or wind turbines) or enhancing energy storage capacity.
- Opportunity: By evaluating the system’s performance regularly and scaling it as needed, the textile factory can ensure that its energy supply remains sustainable and reliable as the business grows.
9.34.8. Conclusion: Achieving Energy Sustainability in Textile Manufacturing
Implementing small-scale power plants in textile factories offers numerous benefits, including reduced energy costs, improved energy security, and increased sustainability. By investing in renewable energy sources such as solar, wind, and biomass, textile manufacturers can minimize their dependence on grid electricity, reduce their carbon footprint, and enhance the overall efficiency of their operations.
With the right planning, financing, and technology selection, textile factories can transition toward more sustainable energy solutions that not only support their production needs but also align with global sustainability goals. Through proper implementation and ongoing optimization, small-scale renewable energy systems can become a key component of a textile factory’s energy strategy, providing long-term benefits for the environment, the business, and the community.
9.34.9. Addressing Challenges in Implementing Small-Scale Power Plants for Textile Factories
While the implementation of small-scale power plants in textile factories offers numerous benefits, there are also challenges that need to be addressed to ensure successful adoption. These challenges include technical, financial, and operational hurdles that could impact the overall effectiveness of the system. Below are some common challenges and potential solutions:
- High Initial Capital Investment:
- One of the primary challenges of implementing renewable energy systems is the high upfront cost. Solar panels, wind turbines, biomass boilers, and energy storage systems require significant initial investment, which might be a barrier for many textile factories, especially small and medium-sized enterprises (SMEs).
- Explore financing options such as green loans, tax incentives, and government grants that can help offset the initial capital costs. Additionally, factories could explore Power Purchase Agreements (PPAs), which allow the factory to pay for renewable energy over time instead of upfront costs.
- Financing models, such as Energy-as-a-Service (EaaS), where third-party investors handle the installation and maintenance, and the factory pays for the energy it consumes, can also help minimize the financial burden.
- Integration with Existing Systems:
- Integrating renewable energy systems into an existing energy infrastructure can be complex, especially when dealing with older electrical systems or factories that lack a centralized energy management system. This can lead to inefficiencies or disruptions in production.
- A thorough site assessment and energy audit are crucial before selecting the type of power plant. By understanding the energy demands and current infrastructure, the new system can be designed to complement and integrate seamlessly with the factory’s operations.
- It may be beneficial to implement a hybrid energy system that incorporates both renewable energy sources and grid electricity, with the option of utilizing energy storage to manage intermittent energy production.
- Intermittency of Renewable Energy Sources:
- Renewable energy sources like solar and wind are intermittent, meaning they are not always available when needed. This could lead to energy shortages during periods of low sun or wind or when the energy demand exceeds the renewable generation capacity.
- Energy storage systems, such as batteries or thermal storage, can help mitigate intermittency by storing excess energy generated during peak production times and making it available when demand is high or renewable generation is low.
- A hybrid system that integrates biomass or natural gas backup generators can also provide a reliable power supply when renewable energy production is insufficient.
- Space Constraints:
- Some textile factories, particularly those located in urban areas or on limited land, may face space constraints that make it difficult to install large-scale solar panels or wind turbines. This can be a barrier to implementing renewable energy solutions.
- For space-constrained locations, solar panels can be installed on rooftops or over unused areas like parking lots or land adjacent to the facility. Thin-film solar technology is also a good option for factories with limited space, as it can be installed on walls or other vertical surfaces.
- Small-scale wind turbines or even vertical axis wind turbines (VAWTs) are compact and can be installed in confined spaces, making them suitable for urban environments.
- Energy Storage Management:
- The management of energy storage systems can be challenging, particularly when dealing with fluctuations in renewable energy generation and varying demand levels. Poor management can lead to energy waste or insufficient stored energy.
- Advanced energy management systems (EMS) can help monitor and control energy storage, ensuring that the factory uses the right amount of energy at the right time. These systems can track energy generation, storage levels, and consumption patterns, optimizing energy use and minimizing waste.
- Investing in smart meters and remote monitoring can help factory managers track energy usage in real-time and make informed decisions about energy consumption and storage.
- Maintenance and Operational Costs:
- While renewable energy systems generally have low operational costs, ongoing maintenance and monitoring are required to ensure their continued efficiency. These costs can add up over time, especially if the systems are not properly maintained.
- Predictive maintenance technologies, such as sensors and IoT-based monitoring systems, can help identify potential issues before they become major problems. Regular maintenance schedules and training programs for staff can also ensure the systems operate at peak performance.
- Outsourcing maintenance to specialized service providers can also be a cost-effective solution, especially for smaller textile factories that may not have the in-house expertise to handle complex renewable energy systems.
- Regulatory and Policy Barriers:
- Regulatory and policy barriers, such as grid access issues, lack of incentives, or complex permitting processes, can hinder the implementation of small-scale renewable energy systems in textile factories.
- Textile factories can work closely with local authorities and energy regulators to ensure compliance with regulations and seek out incentives that support renewable energy adoption. Staying informed about policy changes and advocating for pro-renewable policies can help create a more supportive environment for renewable energy projects.
- Collaboration with energy providers and participation in power purchase agreements (PPAs) can also help bypass some of the barriers associated with grid access.
- Supply Chain Considerations:
- The integration of renewable energy may require significant changes to the factory’s supply chain operations. For example, sourcing biomass fuel may involve new logistics, or the installation of renewable energy infrastructure may disrupt existing production workflows.
- Careful planning and communication with suppliers, logistics partners, and contractors can help ensure that any changes to the supply chain are efficiently implemented. Additionally, businesses should prioritize local sourcing of renewable resources to reduce logistical complexity and associated costs.
- Supply chain optimization can also be achieved by using sustainable materials or by developing closed-loop systems where waste products from textile manufacturing are repurposed for energy generation, reducing overall costs and environmental impact.
9.34.10. The Long-Term Benefits of Small-Scale Power Plants for Textile Factories
Despite the challenges, the long-term benefits of implementing small-scale renewable energy systems for textile factories are significant. These benefits can include:
- Lower Energy Costs: By generating their own renewable energy, textile factories can significantly reduce their dependence on expensive grid electricity, leading to long-term savings on energy bills. Over time, the system’s return on investment (ROI) will make the initial capital investment worthwhile.
- Sustainability and Environmental Impact: Transitioning to renewable energy reduces the factory’s carbon footprint, contributing to global efforts to combat climate change. This also aligns with growing consumer demand for sustainably produced goods, providing the factory with a competitive edge.
- Energy Security: Small-scale power plants offer energy security by reducing the factory’s vulnerability to grid outages or fluctuations in electricity prices. This is particularly important for energy-intensive industries like textiles, where production schedules and output depend on a reliable energy supply.
- Improved Corporate Image: Adopting renewable energy solutions enhances the factory’s reputation as an environmentally responsible business. This can improve relations with stakeholders, attract eco-conscious consumers, and lead to new business opportunities in a sustainability-focused market.
- Regulatory Compliance and Incentives: As governments around the world increasingly implement policies that require businesses to reduce their carbon emissions, adopting renewable energy can help textile factories stay ahead of regulatory requirements. Additionally, factories can take advantage of available tax credits and incentives that encourage renewable energy adoption.
- Long-Term Operational Efficiency: Once in place, small-scale renewable energy systems require minimal operational costs and can provide a reliable, efficient energy source for the long term. Additionally, systems can be scaled and adapted as production demands increase, ensuring that the factory’s energy needs are met sustainably for years to come.
9.34.11. Conclusion: A Path Forward for Sustainable Textile Manufacturing
In conclusion, small-scale power plants are a promising solution for textile factories aiming to improve energy efficiency, reduce costs, and contribute to sustainability. With careful planning, financing, and integration, renewable energy systems can address the growing energy needs of the textile industry while minimizing environmental impacts.
By leveraging renewable resources such as solar, wind, and biomass, textile factories can not only meet their energy needs but also position themselves as leaders in sustainable manufacturing. The transition to renewable energy will provide long-term economic, environmental, and operational benefits, allowing textile manufacturers to thrive in a competitive, eco-conscious global market.
9.34.12. Technological Innovations Enhancing Small-Scale Power Plants for Textile Factories
As renewable energy technologies continue to evolve, there are several cutting-edge innovations that are enhancing the effectiveness and feasibility of small-scale power plants for textile factories. These technologies not only improve energy generation but also increase operational efficiency, reduce costs, and enhance the overall sustainability of the factory. Below are some of the most promising innovations in the field of small-scale power generation:
- Solar Panel Technology Advancements:
- The efficiency of solar panels has significantly improved over the past decade. New innovations, such as bifacial solar panels, capture sunlight on both the front and back sides, thereby increasing energy yield without requiring additional space.
- Perovskite solar cells are another emerging technology that promises higher efficiency rates and lower production costs compared to traditional silicon-based solar panels. These panels are lighter, flexible, and can be applied to a variety of surfaces, including building facades and rooftops, which makes them ideal for textile factories with limited space.
- By adopting bifacial or perovskite solar technologies, textile factories can maximize energy production from available space and benefit from reduced costs in the long run.
- Smart Energy Management Systems (EMS):
- Smart EMS use advanced algorithms and real-time data to optimize energy generation, consumption, and storage. These systems allow factory managers to make data-driven decisions regarding energy use, monitor the performance of renewable energy assets, and adjust energy storage levels according to demand and generation patterns.
- Integration with the Internet of Things (IoT) enables remote monitoring of energy systems, which helps in identifying and addressing inefficiencies, malfunctions, or unexpected performance drops early. By using predictive analytics, these systems can even forecast energy production based on weather patterns, optimizing energy use and storage ahead of time.
- Implementing smart EMS can dramatically improve operational efficiency, reduce waste, and lower maintenance costs, ensuring that the factory benefits from optimal energy performance throughout the year.
- Energy Storage Innovations:
- Energy storage technology plays a crucial role in ensuring the reliability of small-scale renewable energy systems, especially when relying on intermittent sources like solar and wind. Advanced battery technologies, such as lithium-ion, solid-state batteries, and flow batteries, are helping to increase storage capacity and decrease costs.
- Additionally, thermal energy storage systems are gaining popularity, particularly in biomass-based power plants. These systems store excess heat generated during power production and use it when needed, such as during periods of low renewable energy generation.
- Investing in advanced storage solutions will ensure that textile factories can store excess energy for use during non-production hours or when renewable generation is low, ensuring consistent and reliable power.
- Waste Heat Recovery Systems:
- Many textile factories produce substantial amounts of waste heat from various manufacturing processes. This heat can often be captured and converted into usable energy through waste heat recovery systems (WHRS). These systems, which include heat exchangers and organic Rankine cycle (ORC) systems, allow factories to repurpose waste heat into electricity, reducing their reliance on external power sources.
- Biogas recovery from waste materials, such as textile scraps, can also contribute to the factory’s power generation efforts. By converting organic waste into biogas, factories can create a closed-loop energy system that uses waste as a resource.
- By implementing waste heat recovery technologies, textile factories can drastically improve energy efficiency and reduce the environmental impact of their operations. It also offers an additional source of power that can complement renewable energy systems.
- Microgrid and Distributed Energy Systems:
- The concept of microgrids is gaining traction in industrial settings, including textile factories. A microgrid is a small-scale power system that can operate independently or in conjunction with the larger grid. It can integrate renewable energy sources, storage, and backup generators into a single system that is controlled locally.
- By using a microgrid, textile factories can manage energy more efficiently and maintain power supply even during grid outages. This is particularly beneficial in remote locations or in areas with unreliable grid infrastructure.
- Implementing a microgrid allows textile factories to gain greater control over their energy supply and enhance energy resilience. Additionally, microgrids enable factories to contribute to grid stability by generating and storing energy locally.
- Advanced Biomass Conversion Technologies:
- Biomass energy, sourced from organic materials such as agricultural residues, wood chips, and even textile waste, is a promising option for many textile factories. New biomass conversion technologies, including pyrolysis, gasification, and anaerobic digestion, allow for more efficient conversion of organic materials into usable power.
- Biofuels, which can be produced from biomass and used in both boilers and internal combustion engines, are another option to generate energy from waste. This reduces waste disposal costs while generating clean energy for the factory.
- By adopting advanced biomass technologies, textile factories can reduce waste, lower energy costs, and achieve higher energy conversion efficiencies, making biomass an even more viable option for power generation.
- Floating Solar Farms:
- Floating solar farms are a relatively new development in the solar energy sector. These systems consist of solar panels mounted on floating platforms, which are installed on bodies of water such as ponds, lakes, or even reservoirs. Textile factories that have nearby water sources can explore floating solar as a means to optimize space utilization and boost energy generation.
- Implementing floating solar can increase power generation capacity without requiring additional land space, offering a great solution for textile factories located near water bodies.
- Artificial Intelligence (AI) for Energy Optimization:
- Artificial Intelligence (AI) is increasingly being used in energy management and optimization. AI-driven systems can analyze energy consumption patterns and predict energy demands, allowing for dynamic optimization of power generation and distribution.
- Machine learning algorithms can also improve the performance of renewable energy systems, predict system failures before they occur, and even adjust energy production in real-time based on factors like weather, energy demand, and grid conditions.
- By incorporating AI technologies, textile factories can optimize energy use, reduce costs, and ensure more efficient operation of renewable energy systems, providing a cutting-edge solution for sustainable energy management.
- Hybrid Systems with Multi-Source Integration:
- A hybrid energy system that integrates multiple renewable energy sources (e.g., solar, wind, and biomass) with backup generators and energy storage can provide more reliable and constant energy output, reducing the dependency on any single energy source.
- These systems can automatically switch between energy sources based on availability, ensuring that the factory always has a reliable energy supply regardless of environmental conditions.
- Adopting hybrid systems ensures that textile factories can generate energy from the most efficient sources at any given time, thus reducing energy costs and improving energy resilience.
9.34.13. Key Takeaways
- Renewable energy adoption is a powerful way for textile factories to reduce energy costs, enhance sustainability, and improve energy resilience.
- Advances in solar, wind, biomass, and energy storage technologies are making renewable energy systems more viable and cost-effective for textile manufacturers.
- The integration of AI, smart energy management, and microgrid systems enables greater efficiency, reliability, and control over energy usage, making small-scale power plants more effective.
- Hybrid systems and advanced biomass technologies offer unique solutions for addressing energy intermittency and optimizing the use of renewable resources.
With the right combination of technologies, planning, and financing, textile factories can transition to renewable energy systems that not only lower costs but also contribute to a more sustainable future for the industry.
Conclusion: Moving Towards a Greener Future for Textile Manufacturing
Small-scale renewable energy systems present a transformative opportunity for textile factories to enhance energy efficiency, reduce their environmental footprint, and strengthen their competitiveness in a sustainability-driven market. By staying at the forefront of technological advancements, textile manufacturers can benefit from cutting-edge innovations while driving the global transition to a cleaner, greener economy.
9.35. Small Scale Power Plant for Food Processing Plants
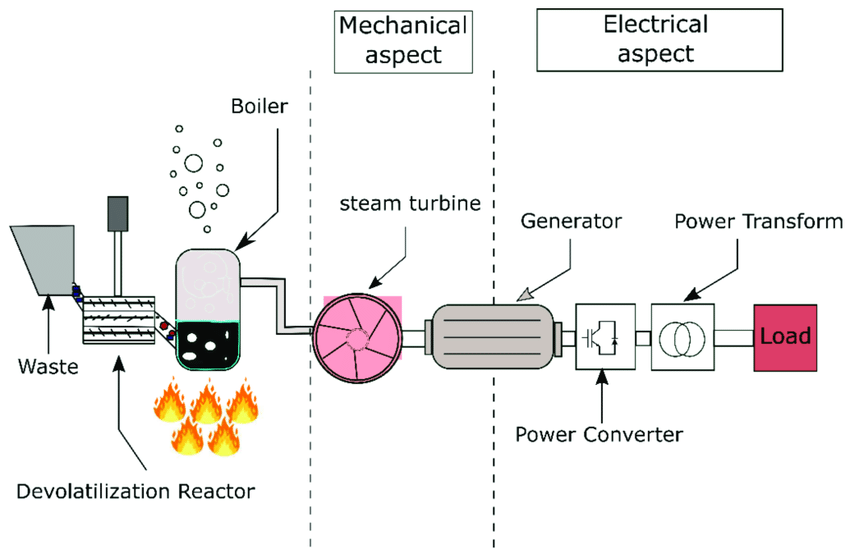
The food processing industry is energy-intensive, requiring consistent and reliable energy for various processes such as cooking, drying, refrigeration, and packaging. The high energy demand, combined with rising energy costs and the increasing focus on sustainability, makes the adoption of small-scale power plants particularly attractive for food processing plants. These small-scale systems can provide renewable, cost-effective energy while also reducing the environmental impact of food processing operations.
9.35.1. Benefits of Small-Scale Power Plants for Food Processing Plants
- Reduced Energy Costs:
- Food processing plants often rely heavily on electricity for machinery, heating, and cooling. By generating their own power through renewable sources such as solar, wind, or biomass, these plants can significantly reduce their dependence on the grid, cutting down on energy costs over the long term.
- Sustainability and Reduced Carbon Footprint:
- The food processing industry has a significant environmental impact due to high energy consumption. By adopting renewable energy sources, such as solar, biomass, or even biogas generated from food waste, these plants can significantly reduce their carbon emissions and contribute to global sustainability goals.
- Energy Resilience and Reliability:
- The ability to generate power onsite ensures that food processing plants are less vulnerable to grid outages or fluctuations, which can disrupt production schedules. Backup power solutions and energy storage systems provide additional security in case of power interruptions.
- Improved Waste Management:
- Many food processing plants generate organic waste that can be converted into energy through biogas production or biomass energy systems. This not only helps manage waste more efficiently but also creates a closed-loop energy system, reducing reliance on external energy sources.
- Enhanced Corporate Image:
- In the face of growing consumer demand for sustainability, food processing plants that adopt renewable energy solutions can enhance their brand image as environmentally responsible businesses. This can attract eco-conscious consumers and improve market positioning.
9.35.2. Types of Small-Scale Power Plants for Food Processing Plants
- Solar Power Systems:
- Solar energy is one of the most popular and accessible renewable energy sources for food processing plants. Solar panels can be installed on rooftops or on nearby land, providing a reliable source of energy for day-to-day operations.
- Advantages:
- Low operational costs after installation.
- Modular and scalable to meet varying energy needs.
- Can be integrated with energy storage systems to provide power during the night or cloudy periods.
- Challenges:
- The effectiveness of solar power is dependent on local sunlight conditions, and the initial capital investment can be significant, although subsidies and incentives can help offset costs.
- Wind Power:
- Wind turbines can provide an effective energy solution for food processing plants located in areas with consistent wind patterns. Wind energy can be used either as a standalone solution or in combination with other renewable energy sources.
- Advantages:
- Reliable in areas with consistent wind speeds.
- Can be integrated with solar power for hybrid renewable energy solutions.
- Challenges:
- Wind power is site-specific, meaning it may not be feasible in areas without reliable wind resources. Noise and visual impact concerns can also be factors to consider.
- Biomass Power:
- Biomass energy is particularly relevant to food processing plants because these facilities often produce significant amounts of organic waste, such as fruit peels, vegetable scraps, or even animal by-products. Biomass power plants convert this waste into heat or electricity through combustion or anaerobic digestion.
- Advantages:
- Utilizes waste products, reducing disposal costs and creating a circular economy.
- Can be a stable and consistent source of energy when properly managed.
- Challenges:
- Requires a consistent supply of biomass material. Handling and storing biomass can be labor-intensive.
- The technology is more complex compared to solar or wind, requiring specialized equipment and skilled personnel.
- Biogas Production:
- Food processing plants can set up biogas digesters to convert organic waste (such as food scraps, wastewater sludge, or agricultural residues) into biogas. The biogas can be used to generate electricity or heat, providing a reliable and renewable energy source.
- Advantages:
- Offers a reliable source of energy, especially for facilities with significant organic waste.
- Helps in managing waste and reducing methane emissions from landfills.
- Challenges:
- Initial setup costs can be high, and the system requires ongoing maintenance to ensure optimal performance.
- The efficiency of biogas production depends on the quality and type of organic waste available.
- Combined Heat and Power (CHP) Systems:
- CHP systems, also known as cogeneration systems, can be used in food processing plants to simultaneously produce electricity and useful heat from a single energy source, such as natural gas, biomass, or biogas.
- Advantages:
- Increases overall energy efficiency by using waste heat from electricity generation for heating purposes.
- Reduces fuel consumption and lowers emissions.
- Challenges:
- Requires a constant source of fuel (biomass, natural gas, etc.), and the system can be complex to manage.
- High capital costs and long payback periods can be a barrier for some food processors.
- Hydropower (Small-Scale):
- If the food processing plant is located near a water source, such as a river or stream, small-scale hydropower systems can be considered. These systems convert flowing water into electricity and can provide a reliable power source.
- Advantages:
- Consistent power generation if water flow is reliable.
- Low operational costs once the system is set up.
- Challenges:
- Site-specific, requiring a suitable water source and infrastructure for installation.
- Potential environmental impact, such as disruptions to local ecosystems or water quality.
9.35.3. Challenges in Implementing Small-Scale Power Plants for Food Processing Plants
- High Initial Capital Investment:
- While renewable energy technologies such as solar and wind have become more affordable in recent years, the upfront investment required for installation, including equipment and infrastructure, can still be significant. For food processing plants, this can be a barrier, especially for smaller operations with limited budgets.
- Seek out government incentives, grants, or Power Purchase Agreements (PPAs) to reduce upfront costs. Third-party financing models, where investors fund the installation and the food plant pays for the energy produced, can also help reduce the initial financial burden.
- Space Constraints:
- Food processing plants, particularly those located in urban or industrial areas, may have limited space for installing large solar arrays or wind turbines. This can make it challenging to generate sufficient renewable energy to meet operational needs.
- Install renewable energy systems on rooftops or unused land areas. Thin-film solar panels or solar windows can also be employed to maximize space utilization. In cases where land is not available, biogas systems using food waste can be a more space-efficient solution.
- Intermittent Nature of Renewable Energy:
- Renewable energy sources like solar and wind are intermittent, meaning energy generation can vary based on weather conditions, time of day, or seasons. This can cause instability in energy supply, especially if the plant relies heavily on renewable sources.
- Implement energy storage solutions, such as batteries or thermal storage systems, to store excess energy generated during peak production times for use when generation is low.
- Use hybrid systems that integrate renewable energy with backup generators (e.g., natural gas or biomass) to ensure a continuous power supply.
- Maintenance and Technical Expertise:
- Renewable energy systems require regular maintenance to ensure they operate efficiently. Food processing plants may not have the in-house expertise to manage these systems, leading to operational downtime or reduced efficiency.
- Engage with third-party service providers or energy management companies that specialize in renewable energy systems to provide ongoing support and maintenance.
- Train in-house personnel on basic system operation and troubleshooting to reduce dependence on external experts.
- Regulatory and Policy Challenges:
- Regulatory requirements and incentives for renewable energy adoption can vary depending on the country, region, or even local municipality. Navigating these regulations can be complex, especially for food processing plants that are looking to implement new technologies.
- Work with local energy consultants and regulatory bodies to understand relevant policies, incentives, and grid connection requirements. Participate in industry forums or networks to stay updated on regulatory changes and best practices.
9.35.4. Conclusion: The Future of Small-Scale Power Plants in Food Processing
The future of small-scale power plants in food processing is promising. With a growing emphasis on sustainability, energy independence, and cost reduction, food processing plants can benefit significantly from adopting renewable energy systems. Solar, wind, biomass, and biogas technologies offer scalable and reliable energy solutions that not only reduce costs but also contribute to the overall environmental sustainability of the industry.
By addressing the challenges of high initial costs, space constraints, and intermittency through the use of energy storage, hybrid systems, and expert partnerships, food processing plants can leverage renewable energy to improve operational efficiency and enhance their corporate reputation. As renewable energy technologies continue to evolve, the economic and environmental benefits of small-scale power plants will only increase, making them a viable option for food processing plants around the world.
9.35.5. Detailed Considerations for Implementing Small-Scale Power Plants in Food Processing Plants
When considering the implementation of small-scale power plants, food processing plants must evaluate several key factors to ensure the success and sustainability of the system. This involves assessing their specific energy needs, available renewable resources, and potential return on investment. Here are the critical considerations that should be made:
- Energy Demand Assessment:
- A thorough assessment of the food processing plant’s energy demand is the first step in determining the appropriate renewable energy system. This includes evaluating peak and off-peak energy use, which will help identify the size and type of system required.
- Load Profiling: Understanding the energy consumption patterns during different production cycles and operational hours will allow for the accurate sizing of energy generation and storage systems.
- Energy Optimization: Once the plant’s demand is assessed, potential energy-saving measures such as improving energy efficiency in machinery, lighting, and heating can be implemented to reduce the overall energy load, making the renewable energy system more cost-effective.
- Site Assessment and Renewable Resource Evaluation:
- A site assessment is essential to determine the feasibility of different renewable energy technologies. Factors such as solar insolation, wind speeds, and the availability of biomass feedstock must be considered.
- Solar Potential: The location of the plant should be assessed for its solar potential. This involves analyzing roof space for solar panels and the average number of sunlight hours throughout the year.
- Wind Potential: Wind turbine installation requires reliable and consistent wind patterns. Wind speed data from local meteorological stations can help determine the viability of wind energy.
- Biomass Availability: If opting for biomass or biogas systems, the plant’s access to organic waste and surrounding agricultural residues must be evaluated to ensure a continuous and reliable fuel supply.
- Integration with Existing Infrastructure:
- Integrating the small-scale power plant into the food processing plant’s existing electrical and mechanical systems is crucial for minimizing disruptions and ensuring smooth operation. This may involve modifying the plant’s electrical grid to accommodate renewable energy generation, energy storage, and backup systems.
- Microgrid Systems: A microgrid can be established within the food processing plant to manage and distribute locally generated renewable energy efficiently. This allows for greater control and optimization of energy use, especially when combined with energy storage solutions.
- Heat Utilization: Food processing plants often have significant thermal energy needs. By implementing combined heat and power (CHP) systems or waste heat recovery systems, the plant can make use of the excess heat generated during power production for industrial processes like drying, cooking, or sterilizing food products.
- Regulatory Compliance and Incentives:
- Local Regulations: Each region or country may have different regulations governing renewable energy installations, grid connection, and environmental impacts. It is critical to ensure compliance with all regulations, including obtaining necessary permits for installation and operation.
- Financial Incentives: Governments and energy organizations often offer financial incentives, rebates, and tax credits for renewable energy installations. Food processing plants should explore available incentives, grants, and subsidies to reduce the upfront capital investment.
- Renewable Energy Certificates (RECs): In some regions, food processing plants can earn renewable energy certificates for generating clean power. These certificates can be sold to utilities or other companies, generating additional revenue streams.
- Financial Analysis and Return on Investment (ROI):
- Conducting a detailed financial analysis is essential for determining the feasibility of small-scale power plant projects. This includes calculating the capital expenditure (CAPEX), operating expenditure (OPEX), and expected return on investment (ROI).
- Capital Costs: The capital costs include purchasing and installing renewable energy systems (solar panels, wind turbines, biogas digesters, etc.), energy storage systems, and any required infrastructure modifications.
- Operating Costs: Operating costs include maintenance, labor, and fuel (for biomass or biogas systems). Maintenance costs can vary depending on the type of renewable energy technology employed, but typically, solar and wind systems have lower maintenance costs compared to biomass and CHP systems.
- Payback Period: The payback period is a crucial metric for understanding how long it will take for the energy savings to offset the initial investment. A positive ROI and a reasonable payback period (usually 5–10 years) make the investment more attractive.
- Financial Support: Third-party financing options, such as Power Purchase Agreements (PPAs) or green bonds, can reduce the financial burden on food processing plants and offer more flexibility in funding the projects.
- Energy Storage Solutions:
- Storage technologies are key to ensuring that renewable energy systems provide consistent power to the food processing plant, especially when relying on intermittent sources such as solar and wind. There are several types of energy storage systems to consider:
- Lithium-ion batteries are the most common form of energy storage and are highly efficient. They provide fast response times and can be used for short- or long-term storage, depending on the plant’s needs.
- Flow batteries are another option, offering longer life cycles and scalability, making them suitable for larger systems with higher storage requirements.
- Thermal energy storage (e.g., molten salt or phase change materials) can be a good option if excess heat is generated by the renewable energy system. This stored heat can be used for thermal processes in food processing.
- Pumped hydro storage is viable in areas with access to water sources and can be a reliable solution for long-duration storage.
- Storage technologies are key to ensuring that renewable energy systems provide consistent power to the food processing plant, especially when relying on intermittent sources such as solar and wind. There are several types of energy storage systems to consider:
- Operational Training and Expertise:
- To ensure smooth and efficient operation of the renewable energy systems, staff members should receive adequate training. This includes training on system operation, maintenance, troubleshooting, and safety.
- Partnerships with Energy Service Providers: For complex systems like biomass power or CHP, food processing plants can partner with specialized energy service providers to manage system operations and maintenance. Outsourcing these responsibilities can ensure optimal performance and allow the plant’s internal resources to focus on production.
- System Monitoring and Data Analytics:
- Continuous monitoring of the renewable energy system is essential for detecting inefficiencies, performance degradation, and potential failures. Advanced data analytics can help track system performance, predict maintenance needs, and optimize energy production and consumption.
- IoT sensors and real-time data platforms can provide valuable insights into energy production, storage levels, and operational health, allowing for quick adjustments and long-term optimization.
- Scalability and Future Upgrades:
- Small-scale power plants should be designed with scalability in mind. As the food processing plant expands or its energy needs change, the renewable energy system should be flexible enough to scale up. This may include adding additional solar panels, wind turbines, or biomass digesters as energy demands grow.
- Modular systems offer the ability to add or upgrade components gradually, spreading the capital costs over time while ensuring the system evolves in tandem with the plant’s growth.
9.35.6. Conclusion: Strategic Path Forward for Food Processing Plants
Food processing plants can achieve substantial benefits from implementing small-scale power plants. Renewable energy solutions such as solar, wind, biomass, and biogas can reduce energy costs, enhance sustainability efforts, and improve operational resilience.
However, successful implementation requires careful planning and consideration of factors such as energy demand, available resources, financial analysis, and system integration. By leveraging advanced technologies, such as energy storage systems, smart energy management, and hybrid solutions, food processing plants can ensure a stable and reliable energy supply while contributing to their environmental goals.
Adopting renewable energy solutions not only aligns food processing plants with global sustainability efforts but also strengthens their competitiveness in a rapidly evolving market that prioritizes clean and responsible production practices. With a structured approach to planning, financing, and execution, food processing plants can transition to a greener future while securing long-term financial benefits.
9.35.7. Advanced Strategies for Optimizing Small-Scale Power Plants in Food Processing Plants
To further optimize the integration of small-scale power plants in food processing operations, businesses can implement advanced strategies that improve efficiency, lower costs, and enhance sustainability. These strategies include advanced technologies, system integrations, and optimization techniques that ensure the renewable energy systems perform at their highest potential.
- Advanced Energy Management Systems (EMS):
- An effective Energy Management System (EMS) is crucial to optimizing the generation, storage, and consumption of renewable energy. An EMS integrates real-time monitoring, data analytics, and predictive algorithms to manage energy use efficiently and minimize waste.
- Demand Response: The EMS can adjust energy usage based on demand patterns, shifting consumption to times when renewable energy generation is at its peak (e.g., during midday for solar or windy periods for wind energy). This reduces reliance on grid power and increases the plant’s overall energy independence.
- Automated Controls: Integrating automated systems that can dynamically adjust the energy flows between the renewable generation units, storage systems, and the plant’s energy consumption needs helps optimize energy efficiency, reduce costs, and ensure a reliable power supply.
- Hybrid Renewable Energy Systems:
- A hybrid renewable energy system combines multiple energy generation sources to ensure a consistent and reliable energy supply. For food processing plants, this could mean pairing solar panels with wind turbines or biomass systems. The hybrid approach allows for energy generation from diverse sources, reducing the risk of downtime due to intermittency.
- Hybrid Solar and Biomass: Solar power can handle daily energy needs while biomass energy can provide power during low-sun periods, such as evenings and cloudy days. Similarly, pairing wind and solar power provides complementary generation profiles, ensuring continuous energy supply.
- Energy Storage Integration: Hybrid systems often work best when combined with energy storage solutions, which can store excess power generated during peak production times for use during low generation periods. This ensures that the food processing plant remains powered even when renewable sources are not generating at full capacity.
- Grid Support and Net Metering:
- In many regions, food processing plants can benefit from net metering, a system where excess renewable energy generated onsite is fed back into the local electrical grid. This allows the plant to receive credit for surplus energy, reducing overall electricity costs.
- Grid Stabilization: Small-scale power plants can also provide grid support by acting as decentralized energy sources that help stabilize the grid during peak demand periods. This could lead to potential partnerships with utilities or local government programs that incentivize power generation from renewable sources.
- Virtual Power Plants (VPPs): A VPP is a network of distributed energy resources (DERs) like solar panels, wind turbines, and energy storage systems that can be aggregated and operated as a single virtual power plant. This allows small-scale power plants to participate in grid services and optimize their energy production and consumption.
- Smart Metering and Predictive Analytics:
- Smart metering involves using digital meters that record energy consumption in real-time. This data can be used for predictive analytics, helping food processing plants anticipate energy demands and adjust their energy systems accordingly.
- Predictive Maintenance: By analyzing historical performance data, predictive analytics can forecast when components of the renewable energy system are likely to need maintenance or are at risk of failure. This reduces unplanned downtime, extends equipment life, and ensures optimal performance.
- Energy Usage Forecasting: Combining smart metering with predictive algorithms can allow plants to forecast future energy needs based on historical usage patterns, production schedules, and external factors like weather. This allows for better energy management and more accurate integration of renewable energy systems.
- Advanced Heat Recovery and Utilization:
- In food processing plants, the need for heating is high, and much of the energy used is wasted as excess heat. By implementing advanced heat recovery systems, plants can capture waste heat from production processes or power generation and repurpose it for other needs within the plant.
- Waste Heat Recovery from Biomass or CHP Systems: Heat generated from biomass combustion or combined heat and power (CHP) systems can be recovered and utilized in food processing applications such as drying, sterilization, or pasteurization.
- Thermal Storage Solutions: Excess heat from renewable energy systems can be stored in thermal storage systems (e.g., molten salts or phase change materials). This stored heat can be used during periods of high thermal demand, optimizing energy consumption and reducing reliance on external heating systems.
- Circular Economy Principles:
- Waste-to-Energy: One of the key advantages of small-scale renewable power plants in food processing plants is the potential to turn waste into energy. This can include organic food waste, agricultural residues, and even wastewater treatment sludge.
- Closed-Loop Systems: By adopting circular economy principles, food processing plants can establish closed-loop systems where waste is used to generate energy for the plant’s operations. For example, food scraps can be converted into biogas through anaerobic digestion, which is then used to generate electricity or heat. This not only provides energy but also helps to reduce waste disposal costs and environmental impact.
- Zero-Waste Energy Solutions: Aiming for a zero-waste energy solution, where all by-products of the plant’s operations are used to generate energy or are recycled, contributes to significant cost savings and enhances the plant’s sustainability profile.
- Building-Integrated Renewable Technologies:
- For food processing plants with limited space or land area, building-integrated renewable technologies offer a solution. This can include solar panels integrated into the roof or solar windows that generate electricity while still serving as functional building materials.
- Solar Roof Tiles: Solar roof tiles, which serve as both roofing material and photovoltaic cells, can be installed on existing or new buildings, allowing for energy generation without taking up additional land space.
- Transparent Solar Panels: Transparent solar panels can be integrated into windows, allowing food processing plants to generate power without blocking natural light, making them ideal for buildings with large glass facades.
- Integration with Smart Factory Concepts:
- Integrating renewable energy systems with smart factory technologies can help food processing plants achieve higher levels of automation, efficiency, and optimization. This includes connecting renewable energy systems with Internet of Things (IoT) devices and advanced sensors that monitor both energy use and production processes.
- Real-Time Process Control: Smart sensors can be used to monitor the energy consumption of individual machines or processes, allowing for real-time adjustments. For example, if energy generation is temporarily low, energy-intensive machines can be slowed or shut down during off-peak periods, optimizing energy use and minimizing operational costs.
- Collaboration with Industry Partners:
- Small-scale power plant operators can benefit from collaborating with other industries, energy service providers, and research institutions. These partnerships can lead to shared resources, joint investments, and co-development of innovative technologies.
- Shared Resources: For example, a food processing plant might partner with a nearby agricultural facility or farm to utilize biomass waste or agricultural by-products for renewable energy generation. This collaboration can lower the cost of biomass procurement and ensure a consistent fuel supply for the energy system.
- Innovation and Research: By collaborating with research institutions, food processing plants can stay at the forefront of technological advancements in renewable energy and energy efficiency. This can also open up funding opportunities for joint innovation projects.
- Continuous Monitoring and System Optimization:
- After the installation of a small-scale power plant, continuous monitoring and system optimization are essential to ensure that the plant is running at peak efficiency. This involves regularly analyzing performance data and making adjustments to energy storage, generation, and consumption patterns.
- Continuous Improvement: Using data analytics and machine learning algorithms, food processing plants can continuously optimize energy usage based on real-time performance metrics and feedback. This ensures that the renewable energy systems remain highly efficient and cost-effective over time.
9.35.8. Conclusion: Advanced Strategies for Sustainable Power in Food Processing Plants
Adopting advanced strategies for optimizing small-scale power plants in food processing plants offers tremendous benefits in terms of energy efficiency, cost savings, sustainability, and operational resilience. By combining renewable energy technologies such as solar, wind, biomass, and biogas with smart management systems, energy storage, and circular economy principles, food processing plants can significantly reduce their reliance on external energy sources and enhance their environmental footprint.
Implementing hybrid renewable energy systems, utilizing advanced energy management tools, and integrating waste-to-energy solutions can create a comprehensive and highly efficient energy ecosystem that benefits both the plant and the broader community. Moreover, strategic collaborations with industry partners, research institutions, and technology providers can drive innovation and foster long-term sustainability.
As the global focus on sustainability intensifies, food processing plants that invest in advanced renewable energy solutions will not only meet regulatory requirements but also position themselves as leaders in sustainable manufacturing, gaining a competitive advantage in a market increasingly driven by environmental concerns.
9.35.9. Emerging Trends and Future Prospects for Small-Scale Power Plants in Food Processing Plants
The future of small-scale power plants in food processing plants looks promising as new technologies and industry trends continue to emerge. These developments will not only improve the performance and scalability of small-scale renewable energy systems but also contribute to greater sustainability and cost savings for food processors. Here are some key emerging trends and future prospects:
- Advancements in Energy Storage Technologies:
- Solid-State Batteries: Solid-state batteries, which promise higher energy density and greater safety compared to traditional lithium-ion batteries, are expected to revolutionize energy storage solutions for small-scale power plants. These batteries will allow for longer-lasting storage and quicker discharge, making renewable energy more reliable for food processing plants.
- Next-Generation Supercapacitors: Supercapacitors, known for their ability to charge and discharge rapidly, could complement traditional battery systems, providing a quick power boost when renewable energy generation drops. This technology is particularly useful for stabilizing energy fluctuations in food processing plants with high intermittent power usage.
- Hydrogen Storage: The development of efficient and cost-effective hydrogen storage systems could provide an innovative way to store excess renewable energy. Hydrogen produced from renewable energy sources can be stored and later converted back into electricity or used for heating, offering another layer of flexibility for small-scale power plants.
- Decentralized Energy Systems and Microgrids:
- Microgrid Expansion: As more food processing plants move toward localized, decentralized energy solutions, the role of microgrids will continue to grow. Microgrids allow plants to operate independently from the central grid in the event of outages or power disruptions. This is especially valuable for food processors located in remote areas or regions prone to grid instability.
- Blockchain for Energy Trading: The use of blockchain technology could transform the way small-scale power plants trade energy. By utilizing decentralized platforms, food processing plants could participate in energy trading with neighboring businesses, local grids, or energy markets, maximizing the value of excess energy produced. Blockchain can also enhance transparency and reduce transaction costs in energy exchanges.
- Peer-to-Peer Energy Sharing: Peer-to-peer (P2P) energy sharing is a concept where businesses, homes, and other energy producers exchange energy directly with each other, bypassing traditional energy distributors. For food processing plants, this could mean sharing excess renewable energy with nearby factories or local communities, further reducing operating costs and increasing energy independence.
- Integration of Artificial Intelligence (AI) and Machine Learning:
- AI-Driven Energy Optimization: The use of artificial intelligence (AI) and machine learning (ML) algorithms will allow food processing plants to further optimize energy generation, consumption, and storage. AI can predict energy demands based on factors such as weather patterns, production schedules, and historical energy use, enabling more efficient load management and real-time decision-making.
- Predictive Maintenance with AI: AI can also help in predictive maintenance by analyzing historical performance data of renewable energy systems and identifying patterns or anomalies that indicate when components are likely to fail. This proactive approach reduces downtime and maintenance costs, ensuring that systems operate smoothly.
- Energy Efficiency Modeling: Machine learning algorithms can model energy consumption patterns and recommend improvements to equipment or operational processes. By simulating different scenarios, AI can help identify the most cost-effective changes to optimize energy usage, thus enhancing overall system efficiency.
- Integration with Internet of Things (IoT) for Smart Manufacturing:
- IoT-Enabled Monitoring: IoT devices and sensors can continuously monitor the performance of renewable energy systems and energy consumption in real time. These smart sensors can be integrated with energy management platforms to provide comprehensive insights into energy use, allowing food processing plants to make data-driven decisions.
- Smart Equipment Integration: By linking IoT-enabled equipment in the food processing plant (e.g., ovens, refrigerators, and pumps) to the energy system, operations can be further optimized. These devices can adjust their energy usage based on real-time power availability, shifting energy consumption to periods of peak renewable energy generation and reducing reliance on grid power.
- Demand Forecasting: IoT systems can also support demand forecasting by gathering real-time data on production needs and using predictive analytics to anticipate power demand. This helps food processing plants adjust their energy usage more effectively and ensure that renewable energy is being used when available.
- Circular Economy and Waste Valorization:
- Circular Food Production Systems: Small-scale power plants can integrate with circular food production systems to maximize resource efficiency. For example, food waste that would otherwise go to landfills can be used to generate biogas or biomass for power production, creating a closed-loop energy system.
- Waste Valorization Technologies: Innovations in waste valorization technologies will enable food processing plants to convert different types of waste (such as food scraps, packaging materials, and agricultural residues) into valuable energy sources. Advanced anaerobic digestion processes, for example, can turn organic waste into biogas, which can be used to generate electricity or heat for the plant’s operations.
- Nutrient Recovery and Reuse: In addition to energy recovery, waste valorization can involve recovering valuable nutrients from food production waste. By integrating nutrient recovery with small-scale power plants, food processors can minimize waste while optimizing resource use, contributing to sustainability goals.
- Regulatory and Policy Developments:
- Carbon Pricing and Carbon Credits: As the global push for climate action intensifies, the implementation of carbon pricing mechanisms and carbon credit systems will incentivize food processing plants to adopt renewable energy solutions. By reducing their carbon footprint, plants can not only help mitigate climate change but also potentially generate additional revenue through the sale of carbon credits.
- Renewable Energy Standards and Mandates: Governments are increasingly setting renewable energy targets and mandates for industries, including food processing. These regulations may require businesses to adopt specific renewable energy technologies or to meet certain sustainability goals. Staying ahead of these requirements will provide food processing plants with long-term security and ensure compliance with local and international standards.
- Incentives for Clean Technology Adoption: Food processing plants that invest in renewable energy systems could benefit from government subsidies, tax credits, or grants designed to encourage the adoption of clean technologies. Policies aimed at increasing renewable energy adoption in the industrial sector are expected to become more widespread, offering greater financial support to businesses looking to reduce their environmental impact.
- Future-Proofing through Modular and Scalable Systems:
- Modular Renewable Energy Systems: The future of small-scale power plants in food processing will likely involve the use of modular and scalable systems. This allows for flexibility in scaling up energy generation capacity as the plant’s energy needs grow. For example, a food processor might start with a small solar installation and later expand it with additional panels, storage capacity, or backup systems as production demands increase.
- Scalable Storage Solutions: As food processing plants adopt larger renewable energy systems, scalable energy storage solutions (such as advanced batteries or hydrogen storage) will be essential for maintaining a reliable energy supply. The ability to add storage capacity as needed will ensure that small-scale power plants can meet changing energy demands.
- Retrofitting Existing Infrastructure: Existing food processing plants that wish to transition to renewable energy solutions can benefit from retrofitting their current infrastructure. Modular and scalable systems will allow for incremental upgrades, minimizing the need for large capital investments upfront and ensuring that energy solutions are tailored to the specific needs of the plant.
- Sustainability as a Competitive Advantage:
- Branding and Market Positioning: Food processing plants that adopt small-scale renewable energy solutions can differentiate themselves in the marketplace by emphasizing their sustainability credentials. As consumers and retailers increasingly prioritize environmentally friendly products, renewable energy adoption can improve a plant’s market positioning and appeal to eco-conscious consumers.
- Green Certifications: Obtaining certifications such as LEED (Leadership in Energy and Environmental Design) or ISO 50001 for energy management can demonstrate a commitment to sustainability and help food processing plants attract investment, reduce energy costs, and access new markets.
9.35.10. Conclusion: A Forward-Looking Approach to Small-Scale Power Plants in Food Processing
The future of small-scale power plants in food processing plants is set to be shaped by cutting-edge technologies, evolving regulatory landscapes, and the increasing demand for sustainable practices. By adopting advancements in energy storage, AI-driven optimization, IoT integration, and circular economy principles, food processors can further improve the efficiency and reliability of their renewable energy systems.
Additionally, the growing trend toward decentralized energy solutions, along with the rise of microgrids and energy-sharing platforms, will provide new opportunities for food processing plants to achieve energy independence and reduce operational costs. By staying ahead of emerging trends, food processing plants can not only reduce their environmental impact but also position themselves as leaders in a rapidly evolving industry that is driven by sustainability, innovation, and energy efficiency.
Investing in small-scale power plants for food processing will continue to offer long-term benefits, including reduced energy costs, enhanced operational resilience, and improved sustainability credentials. As the industry evolves, food processors that embrace renewable energy technologies will be well-positioned to meet future challenges while contributing to a more sustainable global food system.
9.36. Small-Scale Power Plant for Aluminum Production Plants
Aluminum production is a highly energy-intensive process, and small-scale power plants can play a significant role in reducing energy costs and improving sustainability for aluminum production plants. The combination of renewable energy technologies and energy management systems can enable aluminum manufacturers to become more energy-efficient, reduce emissions, and improve their overall environmental impact.
In this section, we will explore the various benefits and strategies for integrating small-scale power plants into aluminum production facilities, along with specific technologies, challenges, and emerging trends that could shape the future of energy use in the aluminum industry.
9.36.1. Key Energy Demands in Aluminum Production
Aluminum production, particularly through the Hall-Héroult process, which is the most common method for extracting aluminum from its ore (bauxite), requires a substantial amount of electricity. The primary energy-intensive steps include:
- Electrolysis: The Hall-Héroult process involves electrolytic reduction, where bauxite (aluminum ore) is dissolved in a molten cryolite bath, and an electric current is passed through it to produce aluminum. This electrolysis process is one of the largest energy consumers in the aluminum industry.
- Smelting and Refining: The process of converting bauxite into alumina (aluminum oxide) through the Bayer process also requires significant heat and electricity. High temperatures are needed to drive chemical reactions and separate the aluminum oxide from impurities.
- Recycling: Aluminum recycling, while much less energy-intensive than primary production, still requires energy for melting the aluminum scrap and refining it for reuse. However, recycling requires about 95% less energy than producing new aluminum from raw bauxite.
Given these high energy demands, integrating small-scale power plants in aluminum production plants can lead to significant savings and sustainability benefits.
9.36.2. Renewable Energy Integration in Aluminum Production Plants
Small-scale power plants using renewable energy sources can be used to meet the electricity and heat demands of aluminum production. Key renewable energy technologies suitable for integration include:
- Solar Power:
- Photovoltaic (PV) Systems: Solar power is an attractive option for aluminum production plants, particularly in regions with high solar irradiance. Photovoltaic systems can be installed on rooftops, factory buildings, or open land to generate electricity for on-site use.
- Solar Thermal: Solar thermal technology can be used to generate heat for smelting and refining processes in aluminum production. Concentrated solar power (CSP) systems can produce high-temperature steam for industrial use, reducing reliance on traditional fossil fuel-based heating systems.
- Wind Power:
- Wind Turbines: Small- to medium-scale wind turbines can be installed on-site or in nearby areas with consistent wind patterns to generate electricity. Wind energy can complement other renewable sources, especially when solar power is intermittent, such as during the night or cloudy days.
- Hybrid Wind and Solar: Combining wind and solar power provides a more consistent and reliable energy supply. Wind can generate electricity during the night or during cloudy periods when solar output is low.
- Biomass:
- Biomass Power Generation: Biomass energy from wood chips, agricultural residues, or other organic waste can be used in small-scale power plants to generate both electricity and heat. Biomass can be used to support processes that require high temperatures, such as those found in the Bayer process or in refining.
- Biogas from Waste: Organic waste from aluminum production, such as carbon residues or slag, can be used to produce biogas. This can be burned in internal combustion engines or turbines to generate electricity or used in heating systems to support energy needs.
- Hydropower:
- Micro-Hydropower Systems: In areas with access to water sources, micro-hydropower plants can be utilized to generate electricity. These systems are smaller in scale but can provide reliable, continuous energy, especially in remote locations or in facilities with access to rivers or streams.
- Pumped Storage: In areas with both hydropower resources and high energy demand, pumped storage can be implemented to store excess energy generated during low-demand periods and release it during peak demand times.
9.36.3. Energy Storage Solutions for Aluminum Production
To address the intermittent nature of renewable energy sources, aluminum production plants can integrate energy storage systems that allow them to store excess energy during periods of high generation and use it during times when renewable generation is low. Key energy storage solutions include:
- Battery Storage:
- Lithium-Ion Batteries: Lithium-ion batteries are commonly used for grid storage and can be used to store solar and wind energy. These batteries can provide fast-responding power to aluminum production plants when needed, helping to smooth out fluctuations in renewable energy generation.
- Solid-State Batteries: As discussed previously, solid-state batteries offer higher energy density and improved safety, which could be particularly beneficial for large-scale applications like aluminum production.
- Thermal Storage:
- Molten Salt Storage: In solar thermal systems, excess heat generated during sunny periods can be stored in molten salt systems. The stored heat can be used later to maintain temperatures in the aluminum smelting process or other energy-intensive steps.
- Phase Change Materials: Phase change materials (PCMs) can store heat in a solid-liquid state and release it when needed. These materials can be used for medium-temperature heat storage and can help provide consistent heating for smelting or refining processes in aluminum production.
- Pumped Hydro Storage:
- For aluminum plants located near water sources, pumped hydro storage can be used to store excess energy during periods of low demand. Water is pumped to a higher elevation during off-peak hours, and when energy is needed, it is released to generate electricity through turbines.
9.36.4. Benefits of Small-Scale Power Plants for Aluminum Production
Integrating small-scale renewable power plants into aluminum production offers several benefits:
- Cost Savings:
- Lower Energy Costs: Renewable energy systems, particularly solar and wind, can significantly lower electricity costs, which are typically one of the largest operational expenses in aluminum production. By reducing reliance on grid electricity, plants can protect themselves from volatile energy prices.
- Reduced Fuel Costs for Heating: Biomass and solar thermal systems can reduce the need for conventional fossil fuels used in the aluminum production process, leading to further cost savings.
- Environmental Impact:
- Reduced Greenhouse Gas Emissions: By replacing fossil fuel-based energy with clean, renewable sources, aluminum production plants can significantly reduce their carbon footprint. This is particularly important for industries seeking to meet environmental regulations or improve sustainability.
- Recycling and Circular Economy: Aluminum is highly recyclable, and by adopting small-scale power plants, plants can increase their recycling efforts while reducing waste and emissions. Energy from waste products, including aluminum scrap, can be used to generate additional renewable energy.
- Energy Security:
- Energy Independence: Small-scale power plants allow aluminum production plants to become more energy-independent by reducing their reliance on external electricity sources. This is especially critical in regions with unstable or unreliable grids.
- Grid Support: In some cases, aluminum production plants with small-scale power plants can contribute excess energy back to the local grid, supporting grid stability and potentially generating revenue through net metering.
- Regulatory Compliance and Sustainability Goals:
- Meeting Renewable Energy Standards: As governments introduce stricter renewable energy mandates and carbon reduction targets, integrating renewable energy into aluminum production will help ensure compliance with these regulations.
- Sustainability Branding: Companies adopting green energy technologies are often viewed more favorably by consumers, investors, and stakeholders. Aluminum manufacturers that integrate renewable energy can boost their sustainability credentials and enhance their market position.
9.36.5. Challenges and Considerations
While small-scale renewable power plants offer significant advantages, there are several challenges to consider:
- Initial Capital Investment: The installation of renewable energy systems and energy storage solutions requires a significant initial investment. However, over time, the cost savings from reduced energy expenses can offset these capital expenditures.
- Intermittency of Renewable Energy: Intermittency is a challenge for renewable energy sources like solar and wind. Integrating energy storage solutions and hybrid systems can help mitigate this issue, but careful planning is necessary to ensure continuous energy supply.
- Space and Infrastructure Requirements: Some renewable energy technologies, particularly solar and wind, require large amounts of space for installation. In crowded industrial areas, the availability of land can be a limiting factor.
- Technical Expertise and Maintenance: Maintaining and optimizing renewable energy systems requires specialized knowledge and expertise. Aluminum production plants will need to invest in skilled labor or work with service providers to ensure their systems remain operational and efficient.
9.36.6. Conclusion
Integrating small-scale power plants into aluminum production offers a promising solution for reducing energy costs, improving sustainability, and meeting regulatory requirements. By harnessing renewable energy sources such as solar, wind, biomass, and hydropower, aluminum plants can significantly lower their environmental impact and become more energy-independent.
The adoption of advanced energy storage solutions, smart energy management systems, and hybrid renewable energy systems will help aluminum production plants overcome the challenges posed by the intermittency of renewable sources. As technology continues to advance and renewable energy becomes more affordable, the potential for small-scale power plants in the aluminum industry will grow, making it an essential component of sustainable aluminum production in the future.
9.36.7. Opportunities for Hybrid Power Systems in Aluminum Production
Incorporating hybrid power systems in aluminum production plants can further enhance the reliability and efficiency of energy use, particularly when integrating multiple renewable energy sources. These hybrid systems combine different energy generation technologies—such as solar, wind, biomass, and even fossil fuels—into a single, complementary energy solution. This approach helps mitigate the variability and intermittency of renewable resources while optimizing the overall energy mix.
- Solar and Wind Hybrid Systems:
- Complementary Generation: Solar and wind power systems can complement each other in hybrid configurations. Solar energy is most abundant during the day, while wind energy often peaks during the night or in cloudy conditions. By combining these two sources, aluminum plants can achieve a more stable energy supply. During periods when one resource is not producing enough energy, the other can step in to meet the plant’s energy needs.
- Storage Integration: To further optimize the hybrid system, energy storage technologies such as batteries or thermal storage can be integrated. This would allow excess energy generated during the day or during periods of high wind to be stored and used during periods of low generation. This hybrid approach enhances the flexibility of the aluminum production plant’s energy supply, making it more resilient and less dependent on the grid.
- Biomass and Solar Hybrid Systems:
- Continuous Power Supply: Biomass energy systems can provide a consistent and reliable energy supply, making them an ideal partner for intermittent solar energy systems. During periods when solar generation is low (e.g., at night or during overcast days), biomass can provide a stable energy supply. Additionally, biomass can serve as an energy backup during periods of high demand, ensuring that aluminum production continues without disruption.
- Fuel Flexibility: Biomass-based power plants often use organic waste as fuel, which can be sourced locally or from other industrial processes, such as food production or agricultural activities. This allows for a highly localized and flexible energy supply, which can further reduce reliance on external energy sources and support sustainability goals.
- Microgrid Systems for Energy Independence:
- Local Energy Management: Microgrids are localized energy systems that allow aluminum production plants to operate independently from the central grid. A microgrid can integrate a combination of renewable energy generation, energy storage, and backup power sources (such as diesel or natural gas generators) to ensure a continuous and reliable energy supply.
- Grid Integration for Flexibility: In situations where aluminum plants produce excess renewable energy, they can sell it back to the local grid, generating revenue while reducing the need for external energy imports. Conversely, when renewable energy generation is insufficient, the microgrid can draw power from the grid or use stored energy to meet demand.
- Biogas and Solar Thermal Hybrid Systems:
- Reducing Heat Demand: Aluminum production requires high temperatures for processes like refining and smelting. By integrating solar thermal systems with biogas plants, aluminum production plants can generate heat during the day through solar collectors and supplement it with biogas during the night or when solar output is insufficient. This hybrid system can reduce fuel consumption and improve the overall energy efficiency of the plant.
- Sustainable Heat Production: Biogas produced from organic waste materials such as agricultural residues or plant byproducts can be used for heat generation, further supporting the plant’s sustainability efforts. This approach reduces reliance on fossil fuels for high-temperature processes, making it a more environmentally friendly option for heat-intensive industries like aluminum production.
- Hydropower and Biomass Hybrid Systems:
- Reliability and Resilience: Hydropower, particularly small-scale or micro-hydropower systems, can provide a stable and continuous energy supply. When combined with biomass energy, which can serve as a backup during dry seasons or low water flow periods, this hybrid system offers a reliable and renewable energy solution for aluminum production plants located near water sources.
- Energy Resilience: In areas where water availability may fluctuate due to seasonal changes or droughts, biomass can ensure that power generation remains consistent throughout the year. This combination of renewable energy sources helps aluminum plants avoid disruptions caused by weather conditions or climate variability.
9.36.8. Smart Grid Technologies for Enhanced Efficiency
Smart grid technologies can be incorporated into the energy management systems of aluminum production plants to enhance efficiency, optimize energy use, and ensure reliable power supply from renewable energy sources.
- Real-Time Monitoring and Control:
- Advanced Sensors and Data Analytics: By integrating sensors and data analytics into the plant’s energy infrastructure, aluminum production facilities can track real-time energy usage, production rates, and renewable energy generation. This data can be used to predict energy demand patterns, identify inefficiencies, and make proactive adjustments to energy systems.
- Energy Management Systems (EMS): An EMS can optimize energy consumption by coordinating the operation of renewable energy systems, energy storage devices, and backup generators. For example, an EMS can prioritize the use of renewable energy during times of high production and rely on grid power or backup generators only when necessary.
- Demand Response Programs:
- Flexible Energy Usage: Smart grid systems can enable demand response (DR) programs that allow aluminum production plants to adjust their energy consumption based on real-time price signals or grid demand. For instance, if the price of electricity is high during peak demand periods, the plant can delay non-essential operations or shift energy-intensive tasks to times when renewable generation is abundant or energy prices are lower.
- Grid Stabilization: By participating in demand response programs, aluminum plants can help stabilize the local grid. During periods of high grid demand, the plant can temporarily reduce its energy consumption, contributing to grid reliability and reducing the need for additional fossil fuel-based power generation.
- Decentralized Energy Systems:
- Energy Sharing and Peer-to-Peer (P2P) Networks: Smart grid technologies can enable aluminum production plants to share excess energy with neighboring businesses or industrial complexes through peer-to-peer energy networks. This decentralized model allows for more efficient energy distribution, reducing waste and promoting collaborative sustainability efforts across industries.
- Predictive Analytics for Energy Optimization:
- AI and Machine Learning: By integrating artificial intelligence (AI) and machine learning algorithms into the smart grid system, aluminum production plants can predict fluctuations in energy demand and renewable energy availability. This enables more efficient load forecasting, energy storage management, and overall system optimization.
- Predictive Maintenance: AI can also be used to predict the performance and maintenance needs of renewable energy systems, energy storage, and other critical infrastructure. Proactive maintenance scheduling can prevent unplanned downtime and extend the lifespan of equipment.
9.36.9. Conclusion: Moving Towards Sustainable Aluminum Production
The integration of small-scale power plants powered by renewable energy sources in aluminum production represents a significant opportunity for the industry to reduce its environmental impact, lower operational costs, and improve its energy resilience. By adopting technologies such as solar, wind, biomass, micro-hydropower, and energy storage, aluminum production plants can meet their high energy demands in a sustainable and cost-effective manner.
Hybrid systems, microgrids, and smart grid technologies offer further opportunities for energy optimization, enabling aluminum producers to balance renewable energy generation with backup power sources and to adapt to changes in energy availability. As the global aluminum industry faces increasing pressure to reduce its carbon footprint, the transition to renewable energy will play a crucial role in achieving long-term sustainability goals.
By embracing these technologies and strategies, aluminum production plants can not only improve their energy efficiency but also position themselves as leaders in the global shift toward cleaner, more sustainable manufacturing processes. The future of aluminum production lies in the integration of renewable energy solutions, energy storage, and smart energy management, paving the way for a more sustainable and resilient industrial sector.
9.36.10. Economic Considerations for Small-Scale Power Plants in Aluminum Production
While the environmental and operational benefits of integrating small-scale power plants into aluminum production are clear, economic considerations are crucial for determining the feasibility and long-term viability of these solutions. The implementation of renewable energy systems and energy storage technologies often requires substantial upfront investments. However, these systems can offer significant returns over time through cost savings, reduced energy prices, and increased sustainability credentials.
In this section, we will discuss key economic factors and financial considerations for aluminum producers when integrating small-scale power plants.
9.36.10.1. Capital Costs and Financing
- Initial Investment:
- The capital cost of setting up a small-scale renewable energy system varies depending on the technology selected (e.g., solar, wind, biomass). For example, the cost of solar photovoltaic (PV) systems has dropped significantly over the years, making them more accessible for small-scale applications. Similarly, biomass systems, while more expensive than solar, can be cost-effective if the plant has access to local organic waste.
- In addition to the energy generation systems themselves, costs may include infrastructure upgrades, grid integration, storage systems, and maintenance.
- Financing Options:
- Government Incentives and Subsidies: Many governments provide subsidies, grants, and tax credits to encourage renewable energy adoption. These incentives can significantly reduce the upfront costs of renewable energy systems for aluminum production plants.
- Green Bonds: Aluminum producers can also raise capital through green bonds, which are specifically designed to finance environmentally friendly projects. These bonds attract investors looking to support sustainable ventures and can provide financing with favorable terms.
- Power Purchase Agreements (PPAs): Aluminum plants may explore long-term PPAs with renewable energy developers to procure clean electricity at a fixed price, reducing the financial burden of capital investment. These agreements can provide predictability in energy costs and hedge against volatile electricity prices.
- Financing through Third-Party Ownership:
- Energy as a Service: In some cases, third-party companies may finance the installation and maintenance of renewable energy systems and energy storage. This model, known as “Energy as a Service,” allows aluminum production plants to benefit from renewable energy without having to bear the full cost of installation upfront.
- Leasing Options: Renewable energy equipment leasing offers an alternative to full ownership, allowing the plant to pay for the energy systems over time. This model can help manage cash flow while still achieving sustainability goals.
9.36.10.2. Operational and Maintenance Costs
- Maintenance and Lifespan:
- While renewable energy systems generally have low operating and maintenance costs compared to conventional power plants, there are still ongoing expenses. Regular maintenance is required for systems such as wind turbines, solar panels, and biomass boilers to ensure optimal performance.
- Solar PV systems typically have a lifespan of 25–30 years, while wind turbines may last 20–25 years. Biomass systems and energy storage systems also have long operational lifespans but may require more frequent maintenance depending on the technology used.
- Cost of Energy Storage:
- Energy storage is crucial for managing the intermittency of renewable power, especially in hybrid systems. The cost of energy storage, particularly battery storage, can vary depending on the size of the system and the technology used. Lithium-ion batteries have become more affordable, but they are still a significant investment for larger-scale energy storage.
- Over time, the cost of battery storage is expected to continue decreasing, making it more economically viable for aluminum producers to integrate these systems. Other energy storage solutions, such as pumped hydro or compressed air, can also be considered, but they come with their own unique costs and infrastructure requirements.
9.36.10.3. Return on Investment (ROI) and Payback Period
- Energy Cost Savings:
- One of the primary economic benefits of integrating small-scale renewable energy systems is the reduction in energy costs. Aluminum production is highly energy-intensive, and the ability to generate electricity on-site can significantly lower electricity bills.
- The savings from reduced energy purchases will depend on factors such as the size of the renewable energy installation, local energy prices, and how much of the plant’s energy needs can be met through renewables. For example, a plant that relies primarily on solar power could expect significant savings during daylight hours, particularly in regions with high solar irradiance.
- Payback Period:
- The payback period for renewable energy investments typically ranges from 5 to 10 years, depending on the energy mix, system size, and financing options. However, this period can be shorter in regions where electricity prices are high or where government incentives are available.
- Over the long term, renewable energy investments can generate substantial savings, particularly if energy prices continue to rise or if the price of renewable energy systems continues to decrease.
- Increased Operational Efficiency:
- By reducing energy consumption from the grid, aluminum production plants can improve their overall operational efficiency. The use of local renewable energy also enhances energy security and reduces exposure to external energy price fluctuations, providing more stable cost projections.
- Furthermore, renewable energy adoption may help plants reduce their dependence on fossil fuels for high-temperature processes, leading to long-term cost savings.
9.36.10.4. Long-Term Economic Benefits
- Sustainability and Competitive Advantage:
- By adopting renewable energy systems, aluminum producers can enhance their sustainability profile, which can help improve their competitive positioning in the market. Consumers, investors, and regulators are increasingly prioritizing companies that adopt environmentally responsible practices.
- The ability to advertise a reduced carbon footprint and demonstrate a commitment to sustainability can attract more business, enhance brand reputation, and open new markets.
- Energy Resilience and Risk Mitigation:
- Aluminum plants that rely on renewable energy systems, particularly those with storage capabilities, are less vulnerable to energy supply disruptions. Renewable energy helps mitigate risks associated with grid instability, natural disasters, and fluctuating energy prices.
- Energy resilience also protects plants against regulatory changes, such as carbon pricing or emissions restrictions, which could increase the cost of using conventional energy sources.
- Carbon Credits and Offsets:
- Many countries offer carbon credits or carbon offset programs for businesses that reduce their greenhouse gas emissions. By integrating renewable energy into their operations, aluminum production plants may be eligible to sell carbon credits or offset their emissions.
- This can provide an additional revenue stream or reduce the overall cost of energy transitions.
9.36.10.5. Conclusion: Financial Feasibility and Future Outlook
The economic feasibility of integrating small-scale renewable power plants in aluminum production depends on various factors, including the local energy market, the scale of the plant’s energy needs, and available financing options. While the initial capital costs can be high, the long-term benefits—including energy cost savings, enhanced sustainability, and improved energy resilience—make these investments increasingly attractive for aluminum producers.
As renewable energy technology continues to mature and become more affordable, and as government policies increasingly favor sustainability and clean energy, the financial viability of small-scale power plants in the aluminum industry will only improve. Through strategic planning, investment in the right technologies, and thoughtful integration of energy storage solutions, aluminum production plants can achieve both operational savings and significant environmental benefits, leading to a more sustainable and economically resilient future.
9.36.11. Environmental Impact of Small-Scale Power Plants in Aluminum Production
The aluminum industry is one of the most energy-intensive manufacturing sectors, and its environmental footprint is substantial. As such, the transition to renewable energy sources in aluminum production has the potential to significantly reduce greenhouse gas emissions and mitigate other environmental impacts associated with traditional fossil fuel-based power generation.
This section explores the environmental impact of integrating small-scale renewable power plants into aluminum production processes, including reductions in carbon emissions, water usage, and air pollution.
9.36.11.1. Reduction in Greenhouse Gas Emissions
- Decarbonizing the Aluminum Industry:
- The aluminum production process is highly energy-intensive, with electricity being required for key stages like refining, smelting, and casting. By integrating renewable energy sources such as solar, wind, and biomass into these processes, aluminum plants can significantly reduce their reliance on fossil fuels like coal or natural gas, which are responsible for high levels of carbon emissions.
- Studies have shown that replacing fossil-fuel-powered electricity with renewable energy sources can reduce a plant’s carbon footprint by 50% or more. Depending on the energy mix and efficiency of the renewable systems, some plants may even achieve near-zero emissions in their electricity use.
- Carbon Intensity of Renewable Energy:
- Solar, wind, and hydroelectric power have very low carbon intensities, meaning they emit little to no carbon dioxide during their generation. In contrast, fossil fuel-based power generation, such as coal or natural gas, is highly carbon-intensive. By shifting to renewable energy, aluminum plants can lower the carbon intensity of their operations, contributing to global efforts to reduce carbon emissions and mitigate climate change.
- Furthermore, integrating energy storage systems can improve the consistency of renewable energy production, ensuring that emissions reductions are maintained even during periods of low renewable output, such as on cloudy or windless days.
- Lifecycle Emissions:
- It is important to consider not just operational emissions but also lifecycle emissions associated with renewable energy systems. While the production of renewable energy equipment such as solar panels or wind turbines has some associated emissions (mainly from manufacturing and transportation), these emissions are much lower than those of fossil fuel plants over the course of the systems’ lifetimes.
- Over the long term, the emissions savings from renewable energy significantly outweigh the initial emissions from equipment production, making renewable systems a net-positive solution for reducing the environmental impact of aluminum production.
9.36.11.2. Water Conservation
- Water Usage in Traditional Power Generation:
- Conventional power generation methods such as coal, natural gas, and nuclear plants require significant amounts of water for cooling purposes. The water is typically drawn from local rivers, lakes, or reservoirs, leading to potential ecological disruptions, including thermal pollution, aquatic habitat destruction, and reduced water quality.
- Aluminum production plants that rely on conventional power generation can contribute to these water-related environmental concerns, as water is used not only for cooling but also in refining processes.
- Reduced Water Consumption with Renewable Energy:
- Renewable energy systems such as solar, wind, and biomass typically require little to no water for operation, making them much more water-efficient than conventional energy sources. By shifting to small-scale renewable power plants, aluminum producers can reduce their water consumption and contribute to water conservation efforts.
- This reduction in water use is particularly important in regions facing water scarcity or drought conditions, where conserving water resources is a key environmental and social priority.
- Impact of Water-Free Power Generation:
- Solar PV systems, wind turbines, and small-scale hydropower systems (which generally use closed-loop cooling systems) have minimal or no water usage. This helps reduce the pressure on local water resources, allowing them to be used for other purposes such as irrigation or drinking water supply.
- Aluminum production plants powered by these renewable systems can boast water-efficient operations, which is a significant environmental benefit, especially for industries operating in arid regions or areas affected by water shortages.
9.36.11.3. Air Quality Improvement
- Air Pollution from Fossil-Fuel Power Plants:
- Fossil-fuel-powered power generation, particularly coal and oil plants, release large quantities of air pollutants such as sulfur dioxide (SO₂), nitrogen oxides (NOₓ), particulate matter (PM), and volatile organic compounds (VOCs). These pollutants contribute to poor air quality, smog formation, acid rain, and respiratory illnesses in nearby communities.
- Additionally, aluminum production itself releases greenhouse gases like perfluorocarbons (PFCs), which have extremely high global warming potentials. Traditional power generation methods exacerbate these issues by contributing further to poor air quality.
- Health Benefits of Renewable Energy:
- Replacing fossil fuels with renewable energy sources in aluminum production plants can lead to significant improvements in air quality. By reducing emissions of harmful pollutants, renewable energy not only decreases the industry’s carbon footprint but also lowers the overall pollution load in surrounding environments.
- For instance, the switch to wind and solar power reduces air pollution-related health risks, including respiratory diseases, cardiovascular issues, and premature deaths linked to air quality deterioration.
- The cleaner energy sources would also help to mitigate the formation of smog and acid rain, which can have severe environmental impacts, especially in regions downwind of heavy industrial areas.
- Lowering Industrial Emissions:
- By integrating renewable power sources into aluminum plants, the facility itself becomes less reliant on polluting fossil-fuel-based grid power. This reduction in emissions contributes to broader regional and national efforts to meet air quality standards and environmental regulations.
9.36.11.4. Waste Reduction
- Waste from Fossil-Fuel-Based Power Generation:
- Fossil-fuel-based power plants generate large amounts of waste, including ash, slag, and other byproducts. The disposal of these waste materials can lead to land contamination, water pollution, and other environmental issues.
- In contrast, renewable energy systems such as solar, wind, and hydropower generate little to no waste during operation. This results in less environmental pollution associated with the energy production process.
- Biomass Power and Waste Utilization:
- Biomass energy offers a unique opportunity to recycle organic waste materials. Many biomass power plants use waste from agricultural, industrial, or forestry operations as fuel. This helps to divert waste from landfills, reduce methane emissions from organic waste decomposition, and utilize materials that would otherwise go unused.
- Aluminum production plants that integrate biomass energy can further reduce waste by utilizing locally sourced organic materials, supporting circular economy principles.
- Recycling and Circular Economy in Aluminum Production:
- Aluminum is highly recyclable, and incorporating renewable energy into the aluminum production process supports a more sustainable production model. Using renewable energy for aluminum manufacturing can reduce the energy required for primary production, which traditionally involves high levels of energy-intensive processes.
- By promoting a circular economy approach, which involves recycling scrap aluminum and using renewable energy, aluminum producers can reduce both waste and their carbon footprint, enhancing overall environmental sustainability.
9.36.11.5. Conclusion: A Sustainable Path Forward for Aluminum Production
The integration of small-scale renewable energy systems in aluminum production offers a compelling pathway to significantly reduce the environmental impact of this energy-intensive industry. From reducing greenhouse gas emissions and water consumption to improving air quality and minimizing waste, renewable energy plays a critical role in transforming aluminum production into a more sustainable process.
As global pressure increases on industries to meet sustainability targets and reduce their environmental footprints, aluminum producers that adopt renewable energy will not only contribute to environmental protection but also position themselves as leaders in the global transition to cleaner and more responsible industrial practices.
The combination of reduced emissions, improved resource efficiency, and better overall environmental stewardship ensures that the aluminum production industry can remain both economically competitive and environmentally responsible in the years to come.
9.37. Small-Scale Power Plant for Steel Mills
The steel industry is one of the largest industrial sectors in the world, heavily dependent on energy-intensive processes. Steel mills traditionally rely on fossil fuels to power their operations, leading to high greenhouse gas emissions and large energy consumption. Small-scale power plants that utilize renewable energy sources provide an opportunity to transition steel production towards more sustainable practices, reducing its environmental impact and operational costs.
This section examines the potential for small-scale power plants in steel mills, including the types of renewable energy systems suitable for these applications, their benefits, and the challenges involved in integrating them into steel production processes.
9.37.1. Types of Renewable Energy Systems for Steel Mills
Small-scale power plants can be powered by various renewable energy technologies, which can be either fully standalone or integrated with the existing power grid. The choice of system depends on the geographical location of the steel mill, the scale of operations, and the availability of renewable energy resources. The most common renewable energy options include:
- Solar Power:
- Photovoltaic (PV) Systems: Solar PV panels can be installed on-site, either on rooftops or in adjacent land areas. These systems convert sunlight into electricity and can help meet the power needs of the mill, especially during daylight hours. With decreasing costs and increasing efficiency, solar PV has become a popular choice for small-scale power plants in industrial settings.
- Concentrated Solar Power (CSP): CSP systems use mirrors or lenses to focus sunlight on a small area, generating high-temperature heat that can drive turbines to produce electricity. While CSP is less common for small-scale applications, it may be viable for large steel mills in areas with high solar radiation.
- Wind Power:
- Onshore Wind Turbines: Wind power can be used to complement or replace grid electricity in steel mills. Small-scale wind turbines, ranging from a few kilowatts to several megawatts, can provide a reliable source of renewable energy. Wind farms can be installed in areas with sufficient wind speeds to ensure consistent electricity generation. Depending on the location, wind power can be a significant contributor to a steel mill’s energy requirements.
- Offshore Wind Power: In coastal areas, offshore wind farms can be another source of renewable energy. Although typically larger in scale, smaller offshore wind installations are increasingly becoming viable due to advancements in turbine technology.
- Biomass Power:
- Biomass Boilers: Steel mills can use biomass as a fuel source for heating and power generation. Biomass, such as wood chips, agricultural waste, and even waste materials from the steel production process itself, can be burned in boilers to generate steam or electricity. This provides a renewable energy option that also helps manage industrial waste.
- Biogas from Waste: Some steel mills can produce biogas from organic waste materials, including sludge, food scraps, and plant residues. This biogas can be used to generate electricity or heat, reducing reliance on fossil fuels.
- Hydropower:
- Small-Scale Hydropower: If the steel mill is located near a water source, small-scale hydropower systems can generate electricity using flowing water. These systems can range from micro-hydro plants that generate a few kilowatts to larger systems capable of supplying significant portions of the mill’s energy needs. Small-scale hydropower is particularly suitable for mills located near rivers or streams, offering a reliable and continuous source of energy.
- Geothermal Energy:
- Geothermal Heat Pumps: In regions with accessible geothermal resources, heat pumps can be used to supply heating and cooling for the mill’s operations. Geothermal energy is a reliable and constant source of heat, making it ideal for reducing the need for traditional heating systems.
- Geothermal Power Plants: In certain areas, geothermal power plants can produce electricity by harnessing the Earth’s natural heat. While this may not be feasible for all steel mills, it offers a long-term renewable energy solution in regions with abundant geothermal resources.
9.37.2. Benefits of Small-Scale Power Plants for Steel Mills
- Reduced Energy Costs:
- Steel production is highly energy-intensive, often making up a large portion of overall production costs. By integrating small-scale renewable power plants, steel mills can reduce their reliance on external energy sources, leading to lower energy bills.
- With renewable energy prices continuing to decline and operational costs becoming more predictable, the potential for long-term cost savings is substantial.
- Lower Carbon Footprint:
- Steel production is a major contributor to carbon emissions, with traditional methods of power generation accounting for a large portion of the emissions. By transitioning to renewable energy, steel mills can significantly reduce their carbon footprint.
- Adopting renewable energy systems helps align steel producers with global sustainability goals, potentially improving their corporate image and meeting regulatory requirements for emissions reductions.
- Energy Security and Reliability:
- A small-scale renewable energy system provides steel mills with greater energy security by reducing dependence on the grid and fossil fuel suppliers. The integration of local energy production ensures a more stable and reliable power supply, which is crucial for maintaining continuous operations in energy-intensive industries like steel production.
- Energy storage systems can further enhance reliability, allowing the mill to store excess energy generated during periods of high renewable output and use it during peak demand times or low-generation periods.
- Waste Reduction:
- Biomass power systems help steel mills manage waste by utilizing byproducts from production processes, agricultural residues, or organic waste. This contributes to the circular economy and reduces the need for landfill disposal or waste incineration, which can have environmental consequences.
- Steel production generates significant amounts of waste heat. Some of this heat can be captured and used in small-scale renewable systems, reducing energy losses and improving overall efficiency.
- Compliance with Environmental Regulations:
- Many countries are introducing stricter regulations to reduce industrial emissions and promote sustainability. By implementing renewable energy systems, steel mills can ensure they meet regulatory standards for emissions, waste management, and energy efficiency.
- In addition to regulatory compliance, many governments offer incentives such as tax credits, grants, and subsidies to industries that invest in renewable energy, which can help reduce initial investment costs.
9.37.3. Challenges of Integrating Small-Scale Power Plants in Steel Mills
- High Initial Capital Investment:
- One of the primary challenges for steel mills in adopting small-scale renewable energy systems is the high upfront cost of installation. While the operational costs of renewable systems are lower than those of traditional power generation, the initial investment for technologies such as solar panels, wind turbines, and biomass boilers can be significant.
- Financing options such as loans, grants, and subsidies from government programs, or the use of Power Purchase Agreements (PPAs), can help mitigate this barrier.
- Intermittency and Reliability of Renewable Energy:
- Many renewable energy sources, such as wind and solar power, are intermittent, meaning their generation depends on weather conditions. This can make it challenging to rely solely on renewable energy for continuous steel production.
- To address this issue, steel mills may need to integrate energy storage systems (e.g., batteries or thermal storage) to ensure a stable and reliable energy supply during periods of low renewable generation.
- Space and Location Constraints:
- Steel mills, especially those in urban or industrial areas, may have limited space for installing large-scale renewable energy systems. For instance, wind turbines require significant land area to be effective, and solar PV systems may be constrained by available roof space.
- To overcome space constraints, steel mills may need to explore hybrid solutions, combining multiple renewable energy sources (e.g., wind and solar) or leveraging off-site renewable energy through Power Purchase Agreements (PPAs).
- Integration with Existing Systems:
- Steel mills have complex and highly specialized energy needs, making the integration of renewable energy systems a technical challenge. Small-scale power plants must be designed to work in tandem with existing energy infrastructure and production processes.
- Collaboration with energy experts, engineers, and renewable energy providers is essential to ensure seamless integration and to optimize the performance of the renewable systems.
- Maintenance and Operation:
- While renewable energy systems generally require less maintenance than conventional power plants, regular maintenance and monitoring are still necessary to ensure they operate efficiently. For example, solar panels require periodic cleaning, and wind turbines need to be inspected for mechanical issues.
- Steel mills must have skilled personnel or external service contracts in place to maintain and repair renewable energy equipment to avoid disruptions to operations.
9.37.4. Conclusion: The Future of Small-Scale Power Plants in Steel Mills
The integration of small-scale renewable power plants in steel mills offers a compelling solution to reduce energy costs, decrease environmental impact, and improve energy security. By adopting technologies like solar, wind, biomass, and hydropower, steel producers can transition towards a more sustainable and efficient energy model.
While there are challenges, such as high initial costs, intermittency, and integration complexities, the long-term benefits of renewable energy far outweigh these hurdles. As renewable technologies continue to mature and energy storage solutions improve, the feasibility of small-scale power plants in steel mills will only increase.
Ultimately, by embracing renewable energy, steel mills can not only enhance their economic performance but also contribute to global sustainability efforts, positioning themselves as leaders in the transition to cleaner industrial practices.
9.37.5. Economic Considerations for Small-Scale Power Plants in Steel Mills
While the environmental benefits of small-scale renewable energy systems in steel mills are clear, the economic viability of such projects must also be carefully considered. In this section, we will examine the financial aspects of integrating renewable energy into steel mill operations, including the costs, potential savings, and return on investment.
9.37.5.1. Capital Costs and Investment
The initial investment required for small-scale renewable energy systems is one of the primary considerations for steel mills looking to adopt these technologies. These costs can vary significantly based on the type of system chosen and the size of the steel mill. Below are the key factors that influence capital investment:
- Technology Selection:
- Solar Power: The cost of installing photovoltaic (PV) systems has decreased significantly in recent years, making solar power one of the most affordable renewable energy options. However, the size of the installation required to meet a steel mill’s energy needs can still result in a substantial capital outlay, especially if large-scale ground-mounted solar farms are needed.
- Wind Power: The cost of small- to medium-scale wind turbines depends on the turbine’s capacity, height, and location. Wind projects may require significant land area, which could add to the overall cost. However, the operational costs for wind turbines are typically lower compared to solar installations.
- Biomass Energy: The capital costs for biomass boilers or gasifiers depend on the scale of the facility and the type of feedstock used. Steel mills that have easy access to waste biomass or agricultural residues may benefit from lower feedstock costs but may still face substantial initial investments for the required infrastructure.
- Hydropower: Small-scale hydropower systems can be relatively expensive to install, especially if infrastructure (such as dams or water channels) needs to be developed or upgraded. However, these systems often offer a long lifespan and low operating costs once in place.
- Geothermal Energy: The capital costs of geothermal energy can be high, particularly for deep geothermal systems, due to the drilling requirements. However, the long-term benefits of consistent energy production can make this investment worthwhile in geothermal-rich regions.
- Grid Connection and Infrastructure:
- Connecting a small-scale renewable power plant to the steel mill’s energy grid or to the national grid can involve additional costs. The infrastructure required for this connection, such as transformers, inverters, and electrical wiring, must be considered when calculating capital expenditures.
- In some cases, a steel mill may need to invest in energy storage systems to ensure a continuous power supply, which can add to the initial cost. These systems store excess energy generated during periods of high renewable output and release it when demand is higher than generation.
- Financing Options:
- Steel mills may explore various financing options to reduce the upfront burden of installing renewable energy systems. These include government grants, tax incentives, and low-interest loans aimed at supporting clean energy projects.
- Power Purchase Agreements (PPAs) with renewable energy providers or third-party investors can also be used to spread out the cost of installation and operation over time. Under a PPA, the steel mill agrees to buy renewable energy from the provider at a fixed price for a set period, helping to mitigate initial costs.
9.37.5.2. Operational Savings
The operational savings resulting from the integration of renewable energy into steel mills can be significant. These savings are primarily driven by reduced energy costs, but other factors also contribute to the overall financial benefit:
- Reduced Energy Bills:
- The biggest financial advantage of renewable energy for steel mills is the reduction in energy costs. Steel mills typically consume large amounts of electricity, much of which is purchased from the grid at market rates. By generating their own power through renewable sources, steel mills can lower their reliance on the grid and potentially eliminate a significant portion of their energy bills.
- Solar and wind power systems, once installed, have low operational costs, and the price of energy generated by these systems remains stable over time. In contrast, electricity prices from fossil fuel-based sources can fluctuate, making renewable energy a more predictable and reliable long-term investment.
- Long-Term Cost Efficiency:
- Renewable energy systems generally have lower maintenance and operational costs than traditional power plants. Wind turbines and solar panels have relatively few moving parts, reducing the likelihood of breakdowns. Biomass boilers and small hydropower systems, while requiring more maintenance, still have lower operating costs compared to fossil-fuel-powered plants.
- The operational lifespan of renewable energy systems, particularly solar and wind, is often 20-30 years or more, during which time the mill can enjoy significant savings on energy costs. These savings will likely offset the initial capital investment over time, leading to a positive return on investment.
- Energy Independence:
- Renewable energy can offer steel mills a degree of energy independence, reducing exposure to energy price volatility and supply disruptions. This is especially important for steel mills that operate in regions where grid electricity is expensive or unreliable.
- The ability to generate their own power provides steel mills with more control over their energy supply, leading to increased operational stability and predictability.
9.37.5.3. Return on Investment (ROI) and Payback Period
The return on investment (ROI) and payback period for small-scale renewable energy systems depend on several factors, including the cost of installation, ongoing operational savings, and available incentives. In general, renewable energy projects tend to have relatively long payback periods, but these are often offset by the benefits over the lifetime of the system.
- ROI Calculation:
- To calculate ROI, steel mills need to consider their total capital investment and compare it to the savings on energy costs over time. A common metric for evaluating ROI is the Internal Rate of Return (IRR), which reflects the percentage return on the initial investment over a set period.
- For example, a steel mill that invests in a solar PV system could calculate ROI by subtracting the initial capital costs (installation, infrastructure, etc.) from the savings on electricity bills over the expected lifespan of the system.
- Payback Period:
- The payback period is the time it takes for the energy savings to cover the initial investment. For renewable energy systems, this period can range from 5 to 10 years, depending on the scale of the project, the availability of subsidies or tax incentives, and the price of electricity in the region.
- Smaller systems, such as rooftop solar installations, tend to have shorter payback periods, while larger projects, such as wind farms or hydropower, may require longer periods to break even but offer larger long-term savings.
- Incentives and Subsidies:
- Government incentives and subsidies can significantly shorten the payback period and improve the overall ROI for renewable energy projects. Many countries offer tax credits, grants, or favorable loan terms to encourage industrial adoption of clean energy technologies.
- Steel mills should explore local, regional, or national policies and incentives that can help reduce the financial burden of installing renewable energy systems.
9.37.5.4. Risk Factors and Financial Stability
Although renewable energy systems offer significant long-term savings and environmental benefits, steel mills must also assess potential risks associated with the implementation of these technologies:
- Financing and Debt:
- Taking on additional debt to finance renewable energy installations can be a risk, especially for mills operating in highly competitive or volatile markets. If energy savings do not meet expectations, it could strain the financial stability of the company.
- Mills should carefully consider the financing terms and work with financial advisors to ensure that the project is financially feasible and sustainable.
- Energy Market Fluctuations:
- Although renewable energy systems provide more stable pricing compared to fossil fuels, steel mills must be aware of fluctuations in renewable energy prices (such as energy storage costs or grid electricity prices) that could affect their financial projections.
- To manage these risks, steel mills may want to secure long-term power purchase agreements (PPAs) or integrate hybrid energy systems that can balance renewable energy with backup power from the grid.
- Regulatory Risks:
- Changes in government policies, environmental regulations, or incentives can impact the economic viability of renewable energy projects. Steel mills must stay informed about potential regulatory shifts that could affect the costs or benefits of their renewable energy investments.
9.37.6. Conclusion: Economic Viability of Small-Scale Power Plants for Steel Mills
The economic benefits of small-scale renewable power plants in steel mills are substantial, with potential savings on energy costs, improved operational stability, and lower carbon footprints. However, the capital investment required for installation and integration must be carefully considered, as well as the financing options available to mitigate upfront costs.
Over the long term, the ROI for renewable energy systems is generally positive, with steel mills benefiting from reduced energy expenses, increased energy independence, and lower operational costs. By adopting renewable energy technologies such as solar, wind, biomass, and hydropower, steel mills can ensure greater cost efficiency, sustainability, and resilience in the face of fluctuating energy prices and environmental regulations.
In conclusion, small-scale renewable energy systems offer steel mills a clear pathway to both environmental and economic sustainability, aligning with global efforts to reduce industrial emissions while simultaneously enhancing profitability and operational efficiency.
9.37.7. Case Studies of Small-Scale Power Plants in Steel Mills
The practical application of small-scale power plants in steel mills is increasingly becoming a reality. Around the world, steel manufacturers are embracing renewable energy solutions, ranging from solar and wind to biomass and waste heat recovery. This section highlights several case studies of steel mills that have successfully integrated small-scale renewable energy systems, showcasing the benefits, challenges, and lessons learned from their experiences.
9.37.7.1. Case Study 1: Solar Power Integration at a Steel Mill in Spain
Location: Spain
Technology: Solar Photovoltaic (PV)
Capacity: 5 MW Solar Farm
A steel mill in Spain installed a 5 MW solar PV farm as part of its strategy to reduce energy costs and improve sustainability. The mill faced rising energy prices and the challenge of reducing its carbon footprint in response to European Union emissions regulations.
Project Details:
- The solar farm was installed on the rooftop of the steel mill, taking advantage of the abundant sunlight in the region.
- The system was designed to supply approximately 15% of the mill’s total electricity demand, reducing its reliance on grid power.
- The project cost was offset by government subsidies and tax incentives for renewable energy projects, lowering the capital investment needed.
- Over a period of 6 years, the mill achieved a payback period of approximately 7 years, with a significant reduction in electricity costs.
Outcomes:
- The solar system reduced the mill’s annual energy costs by approximately 10%, which provided a significant economic advantage over the long term.
- The mill’s carbon emissions were reduced by over 3,000 tons per year, contributing to its sustainability goals.
- The success of the project led to further investments in renewable energy, including wind power, to complement the solar generation.
Lessons Learned:
- Strategic planning for solar panel installation on underutilized areas (e.g., rooftops) can significantly reduce land use and maximize available space.
- Government incentives and subsidies were critical in lowering the initial investment and improving the financial feasibility of the project.
9.37.7.2. Case Study 2: Biomass Power at a Steel Plant in the United Kingdom
Location: United Kingdom
Technology: Biomass Boilers
Capacity: 10 MW
A steel plant in the UK installed a 10 MW biomass boiler system to reduce its dependence on fossil fuels and enhance its energy security. The plant, which primarily used natural gas for heating and power generation, aimed to transition to renewable sources as part of a broader sustainability initiative.
Project Details:
- The biomass boiler system was designed to burn a combination of wood chips, agricultural residues, and waste materials from the steel mill’s production processes.
- The installation was a retrofit to the existing boiler infrastructure, reducing the need for additional land or major site changes.
- The system provided around 25% of the plant’s energy requirements, with excess heat being used in other parts of the production process.
- The initial investment was supported by a combination of private financing and government incentives for renewable heat projects.
Outcomes:
- The plant saw a reduction of nearly 12,000 tons of CO2 emissions per year, significantly contributing to the plant’s sustainability targets.
- The biomass system provided stable and affordable energy, with costs lower than those associated with natural gas.
- The biomass system helped the plant diversify its energy sources, reducing the risk of energy price volatility from fossil fuels.
Lessons Learned:
- Integrating biomass systems into existing infrastructure can offer a cost-effective way to transition to renewable energy without requiring a complete overhaul of facilities.
- Careful selection of feedstock is crucial for maintaining system efficiency and controlling costs. In this case, a combination of waste materials from the steel mill’s own production process provided a reliable and cost-effective supply of biomass.
9.37.7.3. Case Study 3: Wind Power Integration at a Steel Mill in the United States
Location: United States
Technology: Wind Power
Capacity: 2 MW Wind Turbine
A large steel mill in the Midwest of the United States faced increasing energy costs and wanted to reduce its dependence on the grid. After evaluating multiple renewable energy options, the mill decided to install a small wind turbine to meet part of its energy needs.
Project Details:
- The wind turbine was located on a nearby piece of land with favorable wind conditions, allowing for efficient power generation.
- The turbine’s output was integrated into the plant’s energy grid, supplying around 5% of the mill’s energy needs during the year.
- The installation of the wind turbine was part of the company’s strategy to diversify its energy portfolio and contribute to clean energy goals.
Outcomes:
- The wind turbine has produced an average of 4,000 MWh per year, contributing significantly to the mill’s renewable energy generation.
- The project helped the steel mill reduce its carbon footprint by approximately 1,000 tons of CO2 per year.
- Energy savings from the wind turbine reduced the mill’s electricity costs by about 7% annually, contributing to long-term financial benefits.
Lessons Learned:
- Wind energy can be a viable option for steel mills located in areas with consistent and strong wind patterns. However, site evaluation is critical to ensure maximum efficiency.
- While the wind turbine only contributes a small portion of the mill’s total energy requirements, it still provides valuable savings and a green energy alternative.
- The successful integration of wind energy prompted the mill to explore additional renewable technologies, including hybrid systems that combine wind and solar power.
9.37.7.4. Case Study 4: Waste Heat Recovery in a Steel Mill in China
Location: China
Technology: Waste Heat Recovery System
Capacity: 15 MW
A large steel mill in China sought to reduce its energy consumption and improve overall plant efficiency by implementing a waste heat recovery system. Steel production generates significant amounts of excess heat, which can be captured and converted into usable energy.
Project Details:
- The mill installed a waste heat recovery system that captures heat from the exhaust gases and molten metal processes.
- The recovered heat was used to generate electricity and provide process heat, reducing the need for additional fuel inputs.
- The system was integrated with the mill’s existing infrastructure, allowing for seamless energy recovery without disrupting operations.
- The mill also invested in a small-scale thermal storage system to store excess heat for use during high-demand periods.
Outcomes:
- The waste heat recovery system provided approximately 15 MW of electricity, meeting up to 20% of the mill’s energy needs.
- The system reduced the mill’s natural gas consumption by 25%, leading to substantial cost savings.
- The integration of waste heat recovery contributed to a reduction in CO2 emissions by over 10,000 tons annually.
Lessons Learned:
- Waste heat recovery systems can be highly effective in energy-intensive industries like steel production, where significant heat is produced and often wasted.
- Integrating thermal storage with waste heat recovery can help address the intermittency of energy generation, providing a reliable energy source even when the heat recovery process is not operating at full capacity.
9.37.8. Future Trends and Innovations
As the steel industry continues to evolve, several emerging trends and innovations are shaping the future of small-scale power plants in steel mills. These developments aim to improve energy efficiency, reduce costs, and increase the adoption of renewable energy technologies. Some of the key future trends include:
- Hybrid Energy Systems:
- The integration of multiple renewable energy sources, such as solar, wind, and biomass, into a single energy generation system will become more common in steel mills. Hybrid systems can address the intermittency of individual renewable sources and provide a more stable energy supply.
- Advanced energy storage systems, such as batteries or thermal storage, will play a key role in optimizing hybrid systems, ensuring that steel mills can continue to operate efficiently even during periods of low renewable energy generation.
- Advanced Grid Integration:
- As the steel industry adopts more renewable energy sources, the need for smart grid technologies will increase. Smart grids enable better monitoring and management of energy generation, consumption, and storage, improving efficiency and reducing energy waste.
- Steel mills will increasingly participate in demand response programs, where energy usage is adjusted based on grid conditions, allowing for greater grid stability and cost savings.
- Decarbonization Technologies:
- Carbon capture, utilization, and storage (CCUS) technologies will be integrated into small-scale power plants in steel mills, enabling the capture and reuse of carbon dioxide emissions. This will help reduce the overall carbon footprint of steel production.
- Additionally, green hydrogen production from renewable energy sources may become a viable option for decarbonizing steel production, replacing coke and coal in blast furnaces with hydrogen to produce steel in a more sustainable manner.
- Digitalization and AI:
- The use of digital technologies, such as AI and IoT (Internet of Things), will enable steel mills to optimize their energy use and enhance the performance of renewable energy systems. AI algorithms can predict energy demand and supply, allowing for better energy management and forecasting.
9.37.9. Conclusion
Small-scale power plants offer steel mills a pathway to reduce energy costs, improve sustainability, and meet environmental regulations. The successful integration of renewable energy systems, such as solar, wind, biomass, and waste heat recovery, has been demonstrated in numerous case studies, highlighting the economic and environmental benefits of these projects.
As the steel industry continues to focus on sustainability and efficiency, small-scale renewable energy systems will become increasingly important. By adopting innovative energy solutions, steel mills can enhance their competitiveness, reduce their environmental impact, and contribute to the global transition to a cleaner, more sustainable energy future.
9.37.10. Challenges and Solutions in Implementing Small-Scale Power Plants for Steel Mills
While the integration of small-scale renewable energy systems in steel mills offers numerous advantages, it also presents challenges that must be addressed for successful implementation. These challenges include technical, economic, regulatory, and operational issues. In this section, we will explore the main challenges faced by steel mills when adopting renewable energy technologies and propose potential solutions to overcome these obstacles.
9.37.10.1. Technical Challenges
- Intermittency and Reliability of Renewable Energy:
- Challenge: Renewable energy sources such as solar and wind are intermittent, meaning their output can fluctuate based on weather conditions, time of day, or seasonal changes. Steel mills rely on a steady and reliable power supply, making intermittent energy a challenge to integrate into their operations.
- Solution: The use of energy storage systems such as batteries, thermal storage, or pumped hydro storage can help address intermittency. These systems store excess energy during periods of high renewable output and release it when generation is low. Additionally, integrating hybrid energy systems (e.g., combining solar and wind with backup fossil fuel generation) can provide a more stable and reliable power supply.
- Grid Integration and Compatibility:
- Challenge: Integrating small-scale renewable energy systems into existing grid infrastructure can be technically challenging. Steel mills may have outdated or incompatible electrical systems that need to be upgraded to accommodate renewable energy generation and ensure efficient energy distribution.
- Solution: Smart grid technologies can enhance the integration of renewable energy by allowing real-time monitoring, control, and optimization of energy flow. Upgrading grid infrastructure, such as installing advanced inverters, transformers, and controllers, can also improve compatibility and facilitate the seamless connection of renewable energy sources to the grid.
- System Sizing and Energy Storage:
- Challenge: Determining the correct size of a small-scale power plant is critical for ensuring it meets the energy needs of the steel mill without being oversized, which could lead to inefficiencies and higher costs. Similarly, sizing an energy storage system to match renewable generation is complex, as it requires accurate forecasting of energy consumption and production.
- Solution: Energy audits and detailed feasibility studies can help assess the mill’s energy consumption patterns and determine the optimal size of renewable energy systems and storage solutions. Using advanced AI and machine learning algorithms can improve forecasting and enable more accurate sizing of energy systems.
9.37.10.2. Economic Challenges
- High Initial Capital Investment:
- Challenge: The upfront costs of installing renewable energy systems can be significant, particularly for technologies like wind, biomass, or waste heat recovery. For steel mills operating on tight margins or in economically challenging environments, securing the necessary capital can be difficult.
- Solution: Steel mills can explore a variety of financing options to offset the initial capital outlay. These include government incentives, grants, and tax credits for renewable energy projects, as well as Power Purchase Agreements (PPAs), which allow mills to finance the installation through third-party investors. Leasing agreements for renewable energy systems or financing through green bonds can also reduce the upfront financial burden.
- Payback Period and ROI Uncertainty:
- Challenge: Renewable energy projects often have long payback periods, with ROI uncertain in the early stages. Steel mills may be hesitant to invest in renewable energy systems if they are uncertain about the return on investment or the length of time required to recoup their investment.
- Solution: Conducting detailed financial modeling that includes scenario analysis for energy price fluctuations and long-term savings can help mitigate uncertainty. In addition, government policies or private incentives aimed at reducing the payback period, such as feed-in tariffs or subsidized energy rates, can improve ROI and provide more predictable returns.
- Operational Costs and Maintenance:
- Challenge: While renewable energy systems generally have lower operational costs than fossil-fuel systems, they still require regular maintenance and oversight. Biomass, for example, involves fuel sourcing and handling, and wind turbines and solar panels require periodic inspections and cleaning.
- Solution: Developing a comprehensive maintenance and service plan is essential to ensure the ongoing efficiency and reliability of renewable energy systems. Additionally, predictive maintenance technologies using AI and IoT can reduce unplanned downtime and extend the life of renewable energy equipment.
9.37.10.3. Regulatory and Policy Challenges
- Uncertainty in Government Policies:
- Challenge: The regulatory environment surrounding renewable energy is often subject to change, with policies and incentives varying by region or country. Steel mills may be hesitant to invest in renewable energy systems if they are unsure about the future availability of subsidies, tax incentives, or favorable regulations.
- Solution: Steel mills should stay informed about current and emerging regulations in their region and engage with policymakers to advocate for long-term, stable renewable energy incentives. Long-term Power Purchase Agreements (PPAs) with renewable energy providers can also provide stability, as they often lock in favorable energy prices for an extended period.
- Compliance with Emission Regulations:
- Challenge: Steel mills are subject to stringent environmental regulations aimed at reducing carbon emissions and improving energy efficiency. While renewable energy systems can help meet these regulations, mills may face challenges in aligning their renewable energy efforts with compliance timelines.
- Solution: Strategic energy planning that incorporates both renewable energy adoption and energy efficiency measures can help steel mills meet regulatory requirements. In addition, working with environmental consultants and regulatory agencies can help steel mills navigate the complexities of compliance while optimizing their energy strategy.
9.37.10.4. Operational Challenges
- Integration with Existing Operations:
- Challenge: The installation of small-scale power plants may disrupt daily operations if not properly integrated. Steel mills require continuous power for their operations, and any interruption in power supply could result in production delays and financial losses.
- Solution: Careful project planning and phasing the installation of renewable energy systems during off-peak hours or planned shutdowns can minimize operational disruptions. Additionally, ensuring that renewable energy systems are integrated with the mill’s energy management system (EMS) allows for smoother transitions and better energy control.
- Energy Storage and Backup Power:
- Challenge: Renewable energy systems that rely on intermittent sources, such as solar or wind, need reliable energy storage or backup systems to ensure uninterrupted operation. Without sufficient storage or backup power, a steel mill may face operational challenges during periods of low renewable generation.
- Solution: Hybrid systems that combine renewable energy with backup power from grid electricity or fossil-fuel-based generators can provide a reliable energy supply. Investing in advanced energy storage technologies like lithium-ion batteries or compressed air energy storage (CAES) can also address intermittency and ensure reliable power when needed.
9.37.10.5. Environmental and Social Challenges
- Environmental Impact of Renewable Energy Systems:
- Challenge: While renewable energy is generally considered environmentally friendly, certain technologies, such as biomass, can have negative environmental impacts. For example, large-scale biomass operations may lead to land-use changes, deforestation, or air pollution from biomass combustion.
- Solution: Careful selection of sustainable biomass feedstocks, including agricultural waste and industrial byproducts, can mitigate environmental impact. Additionally, steel mills should consider environmental impact assessments (EIAs) before implementing renewable energy projects to ensure that they align with sustainability goals and minimize unintended consequences.
- Community and Stakeholder Engagement:
- Challenge: The implementation of renewable energy systems, particularly wind farms and solar fields, may face opposition from local communities or stakeholders. Concerns may include visual impacts, land use, or the perceived reliability of renewable energy sources.
- Solution: Community engagement is critical for the success of renewable energy projects. Steel mills should conduct public consultations and provide transparency regarding the benefits and potential impacts of renewable energy projects. Demonstrating the long-term benefits of renewable energy, such as job creation and environmental improvements, can help gain community support.
9.37.11. Conclusion: Overcoming Challenges to Achieve Sustainable Steel Production
While there are significant challenges in implementing small-scale power plants for steel mills, the potential benefits far outweigh the obstacles. By carefully addressing technical, economic, regulatory, operational, and environmental challenges, steel mills can successfully integrate renewable energy systems into their operations, leading to reduced energy costs, lower carbon emissions, and greater sustainability.
Solutions such as energy storage, hybrid systems, careful planning, and proactive community engagement will be key to overcoming challenges and ensuring that renewable energy projects are successful. As the global steel industry continues to prioritize sustainability and energy efficiency, small-scale power plants will play an increasingly important role in driving the transition to cleaner, more resilient steel production processes.
In the coming years, technological advancements, policy support, and strategic collaborations will further enhance the feasibility of renewable energy integration, making it easier for steel mills to capitalize on the economic and environmental benefits of small-scale power plants.
9.38. Small-Scale Power Plants for Cement Factories
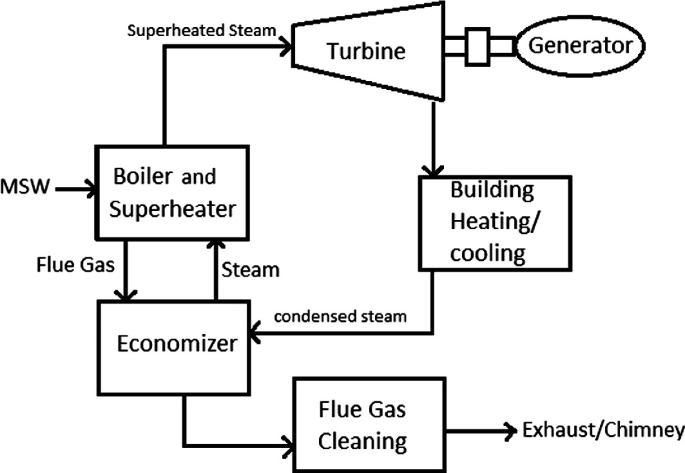
Cement production is an energy-intensive process, with the industry accounting for a substantial portion of global energy consumption and carbon emissions. Small-scale power plants can offer an effective solution to help cement factories reduce energy costs, improve sustainability, and minimize their environmental footprint. These systems, which typically rely on renewable energy sources such as solar, wind, biomass, and waste heat recovery, can provide clean and reliable energy for cement production.
This section discusses the benefits, challenges, and solutions associated with implementing small-scale power plants in cement factories. Additionally, several case studies of successful integration of renewable energy systems into cement plants are presented.
9.38.1. Benefits of Small-Scale Power Plants for Cement Factories
- Energy Cost Reduction:
- Cement factories are significant energy consumers, primarily relying on electricity and heat from fossil fuels such as coal or natural gas. By integrating small-scale power plants, particularly renewable energy systems, these factories can reduce their reliance on grid power and lower their energy costs in the long term.
- For example, using waste heat recovery systems can generate electricity from the waste heat produced during cement production, providing a free or low-cost energy source for the plant.
- Environmental Benefits:
- Cement production is one of the largest industrial sources of carbon emissions due to the high energy consumption and the chemical process of turning limestone into clinker. Small-scale power plants, especially those utilizing renewable energy sources like solar, wind, and biomass, can significantly reduce the carbon footprint of cement factories.
- Waste heat recovery systems also help reduce emissions by capturing and using heat that would otherwise be wasted, further lowering the factory’s environmental impact.
- Energy Independence:
- By generating a portion of their energy needs from renewable sources, cement factories can achieve greater energy independence. This is especially important in regions with unreliable energy grids or where energy costs are volatile.
- Small-scale power plants also provide resilience during periods of energy shortages or outages, helping maintain continuous production.
- Regulatory Compliance:
- As governments around the world impose stricter emissions regulations, cement factories are under pressure to reduce their environmental impact. Small-scale renewable energy systems can help cement factories meet these regulations by reducing their reliance on fossil fuels and lowering their greenhouse gas emissions.
- Positive Public Image:
- Adopting renewable energy technologies can enhance a cement factory’s reputation as an environmentally responsible company. This can help attract customers and investors who are increasingly concerned about sustainability.
9.38.2. Types of Small-Scale Power Plants for Cement Factories
Several types of small-scale power plants can be implemented in cement factories, depending on the available resources, energy needs, and geographic location. These include:
- Solar Power:
- Solar photovoltaic (PV) systems can be installed on the roofs of cement factories or nearby land to generate electricity. Solar energy is especially effective in regions with high levels of sunlight.
- In cement factories, solar power can be used to supply electricity for lighting, machinery, and other operational needs.
- Wind Power:
- If the cement factory is located in an area with consistent wind patterns, small wind turbines can be installed to generate electricity. Wind power can complement other renewable sources by providing electricity during periods when solar power is unavailable (e.g., at night or during cloudy weather).
- Biomass Energy:
- Biomass systems can convert organic materials such as wood, agricultural waste, or even waste from the cement production process into heat or electricity. Cement factories often have access to waste biomass, which can be an economical and sustainable energy source.
- Biomass can be used to generate electricity or replace fossil fuels in the production process, such as for kiln firing.
- Waste Heat Recovery:
- Cement production generates substantial amounts of waste heat during the burning of raw materials in kilns. Waste heat recovery (WHR) systems can capture this heat and convert it into electricity, helping cement factories utilize energy that would otherwise be lost.
- WHR systems typically consist of heat exchangers, steam turbines, and generators. The energy produced can be used for the factory’s internal needs or exported to the grid.
- Hydropower:
- In areas with access to flowing water, small-scale hydroelectric power systems can be installed to generate electricity. Hydropower is particularly effective in regions with consistent water flows, such as near rivers or streams.
- While small hydropower systems are less common in cement factories, they can be a viable option in certain locations.
9.38.3. Challenges of Implementing Small-Scale Power Plants in Cement Factories
- High Initial Investment:
- The upfront capital cost of installing renewable energy systems such as solar panels, wind turbines, or waste heat recovery units can be significant. While the long-term savings from energy cost reductions are substantial, the initial investment may be a barrier for some cement factories, especially those with tight budgets or low profit margins.
- Solution: To mitigate high upfront costs, cement factories can take advantage of government subsidies, grants, and tax incentives available for renewable energy projects. Additionally, power purchase agreements (PPAs) and leasing arrangements can help spread the cost over time.
- Integration with Existing Infrastructure:
- Cement factories often have complex energy systems that include a mix of electricity, heat, and fuels for kiln operation. Integrating small-scale power plants into existing infrastructure can be technically challenging and may require system upgrades or retrofitting.
- Solution: A comprehensive feasibility study and detailed engineering assessments are essential before implementing a small-scale power plant. The study can identify the best system integration strategy and outline necessary infrastructure upgrades.
- Intermittency of Renewable Energy:
- Renewable energy sources such as solar and wind can be intermittent, meaning their power output can fluctuate depending on weather conditions. Cement factories need a reliable energy supply, so the variability of renewable sources can be a concern.
- Solution: To address intermittency, cement factories can combine renewable energy sources, such as pairing wind with solar, or integrate energy storage systems (e.g., batteries or thermal storage) to store excess energy for use during periods of low generation. Additionally, backup power from the grid or gas-fired generators can be used to ensure a stable supply.
- Space Constraints:
- Some renewable energy technologies, such as solar PV and wind turbines, require large amounts of land or rooftop space. Cement factories with limited space on-site may face difficulties in finding suitable areas for installation.
- Solution: Cement factories can consider installing solar panels on factory rooftops, parking lots, or other underutilized spaces. Wind turbines can be installed on nearby land or as part of a larger industrial park. In some cases, small-scale biomass systems can use waste materials from production, reducing the need for additional land.
- Regulatory Compliance and Permitting:
- The regulatory environment for renewable energy projects can be complex, with different rules and regulations depending on the region. Cement factories may face challenges in obtaining the necessary permits for renewable energy systems, particularly for technologies like wind turbines or hydropower.
- Solution: Cement factories should work closely with local authorities and regulatory agencies to ensure compliance with permitting requirements. Engaging with environmental consultants can help navigate the regulatory landscape and identify potential barriers.
9.38.4. Case Studies of Small-Scale Power Plants in Cement Factories
- Case Study 1: Solar Power at a Cement Factory in India
Location: India
Technology: Solar PV
Capacity: 2 MW
A cement factory in India installed a 2 MW solar PV system to supplement its electricity needs and reduce reliance on grid power. The plant faced high electricity costs, and the installation of solar panels on the factory rooftop offered a solution.
Outcomes:
- The solar system reduced the factory’s electricity costs by approximately 15%.
- The system helped the factory reduce its CO2 emissions by over 2,500 tons annually.
- The project paid back its initial investment in 6 years, providing long-term cost savings.
Case Study 2: Waste Heat Recovery at a Cement Plant in China
Location: China
Technology: Waste Heat Recovery (WHR)
Capacity: 7 MW
A cement plant in China implemented a waste heat recovery system to capture heat from its rotary kilns and generate electricity. The system provided a significant portion of the plant’s energy needs, reducing dependence on external power sources.
Outcomes:
- The WHR system generated 7 MW of electricity, meeting up to 20% of the plant’s total energy demand.
- The project reduced the factory’s CO2 emissions by over 15,000 tons per year.
- The system provided a payback period of 4 years, with ongoing savings in energy costs.
Case Study 3: Biomass Power at a Cement Factory in Brazil
Location: Brazil
Technology: Biomass
Capacity: 5 MW
A cement factory in Brazil switched to using biomass, primarily sourced from agricultural residues, to power its cement kilns. The factory also implemented a biomass-fired power plant to generate electricity for its operations.
Outcomes:
- Biomass energy reduced the factory’s reliance on fossil fuels by 50%.
- The system helped reduce carbon emissions by approximately 10,000 tons annually.
- The project was financially supported by government incentives for renewable energy projects.
9.38.5. Conclusion
Small-scale power plants offer cement factories an opportunity to reduce energy costs, decrease carbon emissions, and improve sustainability. Renewable energy sources such as solar, wind, biomass, and waste heat recovery can be integrated into cement production processes to provide reliable and cost-effective energy.
While there are challenges in implementing small-scale power plants, including high initial investment, space constraints, and integration with existing systems, these challenges can be mitigated through careful planning, government incentives, and technological solutions like energy storage. With growing pressure for industries to reduce their environmental footprint, small-scale power plants represent a critical tool in the transition to sustainable cement production.
9.39. Small-Scale Power Plants for Textile Factories
Textile manufacturing is an energy-intensive industry, often relying heavily on electricity for production processes like spinning, weaving, dyeing, and finishing. Small-scale power plants can provide textile factories with more sustainable and cost-effective energy solutions, reduce dependency on the grid, and help lower their carbon footprint. Renewable energy technologies, such as solar, wind, biomass, and hydropower, can be adapted for use in textile production, offering the possibility of a more eco-friendly and economically efficient operation.
This section explores the benefits, challenges, and potential solutions for integrating small-scale power plants into textile factories, along with specific examples of successful applications.
9.39.1. Benefits of Small-Scale Power Plants for Textile Factories
- Energy Cost Reduction:
- Textile factories are high consumers of electricity for machinery operation, lighting, and heating processes. By integrating small-scale renewable energy systems, these factories can significantly lower their electricity bills.
- For example, a solar power system installed on the roof of a textile factory can provide a substantial portion of the plant’s electricity demand during sunny hours, reducing reliance on expensive grid electricity.
- Environmental Sustainability:
- The textile industry is a major contributor to global carbon emissions due to its energy-intensive processes. Transitioning to small-scale renewable energy solutions can reduce a factory’s carbon footprint and improve its sustainability profile.
- Biomass energy, which can be sourced from cotton stalks, wood, or other organic materials, provides a renewable and low-carbon source of power for textile factories, reducing their dependence on fossil fuels.
- Regulatory Compliance and Green Certifications:
- Many countries are increasing environmental regulations on industrial emissions and energy consumption. Small-scale power plants can help textile factories meet these regulations by providing cleaner energy solutions and lowering emissions.
- Using renewable energy also helps textile factories earn certifications like ISO 14001 (environmental management) or LEED (Leadership in Energy and Environmental Design), which can boost their reputation and attract environmentally conscious customers.
- Energy Independence:
- By producing their own energy through renewable sources, textile factories can become less reliant on external power sources, offering resilience in the face of energy price fluctuations or power outages.
- Having a small-scale power plant in place ensures that critical processes can continue without interruption, even during grid failures or energy shortages.
- Improved Brand Image:
- Adopting clean and renewable energy technologies can enhance a textile factory’s reputation among consumers and businesses that prioritize sustainability. This can also appeal to eco-conscious buyers who prefer to support companies with environmentally responsible practices.
- Furthermore, it can differentiate the factory in a competitive market where sustainability is becoming an increasingly important factor in purchasing decisions.
9.39.2. Types of Small-Scale Power Plants for Textile Factories
- Solar Power:
- Solar photovoltaic (PV) systems are one of the most popular renewable energy sources for textile factories, especially in regions with abundant sunlight. Solar panels can be installed on the rooftops of factories or on nearby land to generate electricity.
- Solar energy can power lighting, HVAC systems, and machinery, reducing reliance on the grid during peak daylight hours.
- Wind Power:
- For textile factories located in areas with consistent wind conditions, wind turbines can provide a supplementary power source. Wind power can complement solar energy, especially in regions where wind speeds are high during periods of low solar activity.
- Wind power generation can be particularly useful for factories located in coastal or open plains areas with favorable wind conditions.
- Biomass Power:
- Biomass energy is an ideal option for textile factories that produce organic waste, such as cotton stalks, leaves, and sawdust, during the manufacturing process. Biomass systems can convert this waste into electricity or heat, providing a sustainable and cost-effective energy source.
- Biomass-fired boilers or gasifiers can be used to generate steam for textile processing or electricity to power factory operations.
- Hydropower:
- Small-scale hydroelectric power plants can be an option for textile factories located near streams or rivers with a reliable water flow. These systems use the kinetic energy of moving water to generate electricity.
- While small hydro systems are less common for textile factories, they can offer a consistent and reliable power source in areas with suitable geography.
- Waste-to-Energy (WTE):
- WTE technology can be used to convert textile waste, such as fabric scraps, plastic, and other discarded materials, into usable energy. This energy can then be used to power machinery or produce heat for dyeing and finishing processes.
- Implementing WTE solutions not only reduces waste but also transforms it into a valuable resource for powering factory operations.
9.39.3. Challenges of Implementing Small-Scale Power Plants in Textile Factories
- Initial Capital Investment:
- The upfront costs of installing renewable energy systems, such as solar panels, wind turbines, or biomass boilers, can be high. For smaller textile manufacturers, securing the necessary capital investment can be a barrier to adopting these technologies.
- Solution: Many governments and financial institutions offer subsidies, grants, and low-interest loans to encourage industrial investment in renewable energy. Textile factories can also consider power purchase agreements (PPAs) or leasing arrangements to spread costs over time.
- Integration with Existing Infrastructure:
- The integration of small-scale renewable energy systems into existing infrastructure can be technically challenging, especially if the factory’s power systems are outdated or not optimized for renewable energy inputs.
- Solution: A thorough feasibility study and planning phase is required to assess the factory’s energy needs and identify the most efficient way to integrate the new systems. This may involve upgrading electrical systems, installing energy storage, or modifying existing equipment.
- Intermittency of Renewable Energy Sources:
- Renewable energy sources like solar and wind are intermittent, which can lead to fluctuations in power generation. This can pose a challenge for textile factories that need a continuous and stable energy supply to maintain consistent production.
- Solution: The implementation of energy storage solutions (e.g., batteries or thermal storage) can help store excess energy generated during peak periods for use during off-peak times. Additionally, hybrid systems that combine multiple energy sources, such as wind and solar, can smooth out power generation inconsistencies.
- Space Constraints:
- Textile factories, particularly those located in urban areas, may have limited space for installing large renewable energy systems like solar PV or wind turbines. The need for space for production, storage, and machinery may limit the area available for energy generation equipment.
- Solution: Textile factories can make use of rooftop solar installations or repurpose other underutilized spaces for renewable energy production. Energy-efficient design can also reduce the overall demand for energy, helping mitigate space limitations.
- Maintenance and Operational Costs:
- Small-scale power plants require regular maintenance to ensure they operate efficiently and effectively. For textile factories, the costs and expertise required to maintain renewable energy systems may be a challenge.
- Solution: Factory managers should plan for maintenance costs and schedule regular inspections. Hiring or contracting specialized staff or external service providers can ensure that the systems are properly maintained without interrupting production.
9.39.4. Case Studies of Small-Scale Power Plants in Textile Factories
- Case Study 1: Solar Power at a Textile Factory in Bangladesh
Location: Bangladesh
Technology: Solar PV
Capacity: 1 MW
A textile factory in Bangladesh installed a 1 MW solar PV system to offset the high costs of grid electricity, which were affecting its profit margins. The system was mounted on the factory rooftop, which was ideal for harnessing solar energy.
Outcomes:
- The solar PV system met approximately 30% of the factory’s energy needs during peak daylight hours.
- The factory experienced a 20% reduction in energy costs, with an expected payback period of 5 years.
- The solar system helped the factory reduce CO2 emissions by over 1,200 tons annually.
Case Study 2: Biomass Power at a Textile Mill in India
Location: India
Technology: Biomass
Capacity: 5 MW
A textile mill in India switched from using coal to biomass to power its steam boilers. The biomass fuel was sourced from agricultural waste, such as cotton stalks, which the factory could obtain locally at a low cost.
Outcomes:
- The biomass system reduced the mill’s reliance on coal by 60%, saving significant amounts on fuel costs.
- The mill reduced its carbon emissions by approximately 10,000 tons annually.
- The project helped the factory meet local sustainability standards and enhanced its reputation as an eco-friendly producer.
Case Study 3: Wind Power at a Textile Factory in Turkey
Location: Turkey
Technology: Wind Power
Capacity: 3 MW
A textile factory in Turkey located near coastal wind corridors implemented a 3 MW wind turbine to supplement its energy supply. The wind turbine generated electricity to power the factory’s operations during windy periods.
Outcomes:
- The wind turbine provided 25% of the factory’s energy needs, significantly lowering energy costs.
- The factory saw a reduction in CO2 emissions of approximately 5,000 tons annually.
- The project’s initial costs were offset by the savings from energy production in less than 6 years.
9.39.5. Conclusion
Small-scale power plants offer textile factories a sustainable and cost-effective way to meet their energy needs while reducing their environmental impact. Renewable energy solutions such as solar, wind, biomass, and waste-to-energy can be integrated into textile manufacturing processes to lower energy costs, enhance operational efficiency, and improve sustainability.
While challenges such as capital investment, intermittency, and space constraints exist, these can be addressed through careful planning, government incentives, and advanced technology solutions. As the textile industry continues to focus on sustainability, small-scale renewable power plants represent an increasingly important strategy for greener, more cost-efficient production.
Small-scale power plants can be a transformative solution for textile factories, offering multiple benefits that enhance sustainability and reduce operational costs. The textile industry, known for its heavy energy consumption, can greatly benefit from integrating renewable energy technologies. These solutions are not only financially advantageous but also align with the growing global push for environmentally responsible manufacturing practices.
One of the primary benefits of small-scale power plants in textile factories is energy cost reduction. Given that textile production involves significant electricity use, from powering machinery to lighting and heating processes, implementing renewable energy solutions can lead to substantial savings. For instance, solar photovoltaic (PV) systems can be installed on factory rooftops to harness the abundant sunlight and generate electricity during peak daylight hours. Wind energy, biomass, and waste-to-energy technologies also present viable alternatives, each offering a complementary energy source that helps reduce reliance on the grid, further stabilizing energy costs.
The environmental sustainability of the textile industry is an ongoing concern, with manufacturing processes contributing to significant carbon emissions. By adopting renewable energy sources such as solar, wind, and biomass, textile factories can significantly reduce their carbon footprints. Biomass energy, in particular, offers a unique advantage by utilizing waste from cotton or other organic materials to generate power, creating a circular system of energy production. Solar and wind power can also reduce a factory’s emissions, ensuring that the factory operates more sustainably and contributes to a greener industry overall.
In addition to lowering emissions, small-scale power plants can help textile factories comply with increasingly stringent environmental regulations. Many countries are implementing laws that require industries to reduce their carbon footprints, and using renewable energy can help factories meet these requirements. Moreover, factories can earn certifications such as ISO 14001 or LEED, which are valuable for businesses aiming to demonstrate their commitment to sustainability and attract environmentally-conscious customers.
Another key advantage of small-scale power plants is energy independence. By producing their own electricity, textile factories become less reliant on external power suppliers, reducing exposure to price fluctuations or energy shortages. This independence is especially valuable in areas where power outages or grid instability can disrupt operations. With reliable on-site generation, factories can maintain continuous production and safeguard their operations against unforeseen disruptions.
Implementing renewable energy systems in textile factories can also enhance a company’s brand image. As consumers become more conscious of the environmental impact of the products they buy, companies that adopt sustainable practices often enjoy a competitive advantage. A textile factory that produces its products with renewable energy can position itself as an eco-friendly brand, attracting customers who prioritize sustainability.
For textile factories, the types of small-scale power plants to consider are varied and depend on factors such as location, available resources, and production needs. Solar PV is one of the most widely adopted solutions due to its versatility and efficiency. Rooftop solar installations are particularly well-suited for textile factories that have large, open roof spaces. Wind power, though less common, can be a viable option for factories located in areas with consistent wind patterns, particularly near coastal or plains regions. Wind turbines can supplement solar energy, providing a steady energy source when sunlight is insufficient.
Biomass energy is another excellent option for textile factories, particularly those with access to agricultural waste. Cotton stalks, wood chips, or other organic materials can be converted into energy through biomass boilers or gasifiers. This technology not only reduces the factory’s carbon footprint but also reduces the amount of waste sent to landfills. Biomass energy can power steam boilers for dyeing and finishing processes or generate electricity for general factory operations.
Hydropower, though less commonly implemented, can also serve as a small-scale power solution for factories located near rivers or streams with reliable water flow. Small hydroelectric plants can generate consistent electricity, often complementing other renewable energy sources like wind and solar. Waste-to-energy (WTE) technologies present another opportunity to create power from textile waste materials, including fabric scraps, plastic, and other manufacturing by-products. This can help textile factories reduce their waste while also providing a renewable energy source.
However, implementing small-scale power plants in textile factories does come with challenges. One of the primary obstacles is the initial capital investment. Renewable energy systems, particularly solar panels or wind turbines, require significant upfront costs, which can be a barrier for small or mid-sized textile factories. To overcome this, factory owners can explore government incentives, subsidies, or financing options that make these systems more accessible. Power purchase agreements (PPAs) or leasing options are other strategies that can help spread the costs of installation and maintenance over time.
Another challenge is integrating renewable energy systems into existing infrastructure. Older factories or those without modern electrical systems may face difficulties in accommodating new renewable technologies. Careful planning and feasibility studies are crucial to ensure that the installation is efficient and does not disrupt production. Upgrading the factory’s electrical system, installing energy storage solutions, and adjusting equipment may be necessary steps to ensure seamless integration.
Intermittency is another concern, particularly with solar and wind energy, as both are subject to fluctuating weather patterns. Energy storage systems, such as batteries, can mitigate this issue by storing excess energy during peak production periods for use when generation is low. A hybrid energy system combining multiple renewable sources can also help smooth out fluctuations and ensure a consistent power supply.
Space constraints, especially in urban areas, may limit the installation of large-scale renewable energy systems like wind turbines or large solar arrays. However, rooftop solar systems or energy-efficient design strategies can help overcome these limitations by optimizing the available space. In some cases, land adjacent to the factory or other underutilized areas can be repurposed for energy generation.
Maintaining small-scale power systems is another consideration. Renewable energy installations require regular maintenance to ensure their continued efficiency. Depending on the technology, this might involve cleaning solar panels, checking wind turbines, or inspecting biomass equipment. While the maintenance costs are generally lower than the operational costs of traditional power sources, proper planning is essential to avoid disruptions to production.
Despite these challenges, numerous textile factories worldwide have successfully implemented small-scale power plants. In Bangladesh, a textile factory installed a 1 MW solar PV system on its rooftop, offsetting 30% of its energy needs and reducing carbon emissions by over 1,200 tons annually. Similarly, in India, a textile mill transitioned from coal to biomass, sourcing organic waste from nearby farms. The biomass system reduced the mill’s coal consumption by 60%, lowered emissions by 10,000 tons, and saved the factory a significant amount on fuel costs.
Small-scale power plants present a promising solution for textile factories seeking to reduce energy costs, improve sustainability, and comply with environmental regulations. By embracing renewable energy, textile factories can not only save money and enhance their reputation but also contribute to the global shift toward more sustainable manufacturing practices.
Small-scale power plants in textile factories are increasingly being seen as essential in achieving sustainability goals while reducing operational costs and energy dependencies. The move toward renewable energy sources not only contributes to a factory’s bottom line but also helps in addressing the textile industry’s substantial environmental footprint.
Beyond the financial and environmental benefits, integrating renewable energy into textile factories has the potential to improve operational resilience. Power outages, grid instability, and fluctuating energy prices can all significantly disrupt manufacturing processes. A self-sustaining power system, such as one that relies on renewable energy sources, shields factories from such vulnerabilities. This kind of energy independence allows factories to continue operations smoothly, even during energy shortages or disruptions in grid supply.
Additionally, the adoption of small-scale power plants could catalyze the growth of a circular economy in the textile sector. For example, waste-to-energy solutions can directly address the issue of textile waste, a major environmental concern in the industry. Converting fabric scraps, offcuts, and other by-products into energy reduces the need to dispose of these materials in landfills while simultaneously generating electricity for the factory. This dual benefit—reducing waste and creating power—aligns well with sustainability efforts in industries that typically produce large amounts of waste.
Furthermore, energy-efficient technologies integrated alongside small-scale power plants can further optimize factory operations. Energy efficiency measures such as LED lighting, efficient heating systems, and advanced insulation techniques can reduce overall power demand. These measures, when combined with renewable energy production, contribute to a substantial decrease in both operational costs and energy consumption. This combination of energy generation and consumption optimization maximizes the benefits of small-scale power plants.
The overall impact of adopting renewable energy solutions in textile factories goes beyond immediate cost savings and environmental benefits. It sets a precedent for other industries to follow suit in adopting sustainable practices. As companies showcase their successful transitions to green energy, it encourages others in the sector to explore similar pathways, amplifying the collective impact on global sustainability goals.
Moreover, textile factories that successfully implement small-scale power plants can benefit from potential market opportunities. The growing trend of sustainable and ethical consumerism means that companies can use their clean energy initiatives as a competitive differentiator. Consumers are increasingly seeking products that are produced with minimal environmental impact, and businesses that actively showcase their renewable energy use can build brand loyalty and attract more customers who value sustainability.
Looking ahead, the combination of increasing regulatory pressures, advancements in renewable energy technology, and the ongoing need for cost-effective energy solutions positions small-scale power plants as a crucial aspect of the future of the textile industry. Governments and industry bodies are increasingly incentivizing businesses to adopt clean energy solutions, which will likely drive further adoption across the sector. From solar and wind to biomass and waste-to-energy systems, the range of renewable energy technologies available offers textile factories the flexibility to choose the best solutions based on their geographic location, available resources, and energy needs.
In conclusion, small-scale power plants offer textile factories not only an avenue for substantial cost savings but also an opportunity to lead the charge toward sustainability. By adopting renewable energy sources, textile manufacturers can reduce their environmental footprint, improve operational efficiency, and enhance their market positioning. This transformation not only benefits the individual factory but also contributes to the global push toward sustainable industry practices and a greener future for all.
Small Scale Power Plant for Agricultural Farms
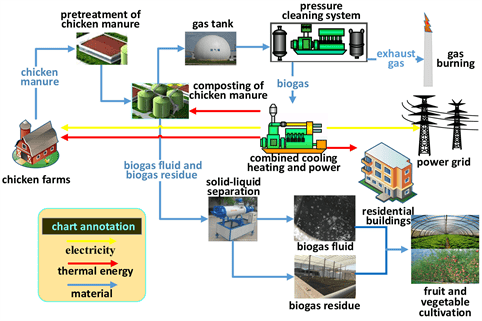
Small-scale power plants are increasingly becoming an essential component for agricultural farms, particularly as the need for sustainability, energy efficiency, and cost-effectiveness grows. Agriculture is a significant energy-consuming sector, and transitioning to renewable energy solutions can not only reduce operating costs but also improve the resilience of farms against energy price fluctuations and external supply disruptions. Moreover, renewable energy sources like solar, wind, biomass, and waste-to-energy are highly suited to the agricultural context due to their compatibility with farming operations and their ability to enhance sustainability in food production.
One of the most prominent benefits of small-scale power plants on agricultural farms is the reduction in energy costs. Farms rely on energy for a range of activities such as irrigation, refrigeration, processing, heating greenhouses, and powering farm equipment. Traditional energy sources, especially diesel or grid electricity, can become expensive, especially during peak usage times or when energy prices fluctuate. Installing a small-scale solar power system or biomass generator allows farms to generate their own electricity, reducing reliance on external energy providers and lowering overall energy expenditures. This independence from the grid is particularly valuable in rural areas, where energy supply may be less reliable.
Solar energy, for instance, is an ideal renewable solution for agricultural farms, especially in regions with abundant sunlight. Photovoltaic (PV) panels can be installed on the roofs of barns, sheds, or other farm structures, or they can be placed in open fields if land area permits. The electricity generated by these systems can be used to power irrigation pumps, water treatment systems, refrigeration for perishable produce, or even electric vehicles used for transportation. In addition, solar energy can be stored in batteries, allowing for electricity use at night or during cloudy days, thereby ensuring a continuous energy supply throughout the day.
Wind power also offers a reliable and complementary renewable energy source for agricultural farms. Farms located in rural or coastal areas with consistent wind patterns can install small to medium-sized wind turbines. Wind power can be used to power farm equipment, irrigation systems, or even electric fencing. In some cases, a hybrid approach combining solar and wind energy can ensure a steady power supply since wind and solar generation tend to be complementary; when solar energy is low, wind energy may be abundant and vice versa.
Another renewable energy option that suits agricultural farms is biomass energy. Farms often produce large quantities of organic waste, including crop residues, manure, or even food waste, which can be converted into energy. Biomass systems such as anaerobic digesters or biomass boilers can be used to produce electricity or heat from this waste. The use of agricultural by-products for power generation reduces waste while providing a sustainable energy source. For instance, manure can be processed in a biogas digester to produce methane, which is then used to generate electricity, heat water, or fuel vehicles.
Biogas systems offer a dual benefit to farmers by not only providing a renewable energy source but also managing waste effectively. The digestate, a byproduct of the biogas production process, can be used as a natural fertilizer, closing the loop of energy generation and waste management. This is particularly important for livestock farms where large quantities of manure are generated regularly. By using biogas to produce power, farms can reduce the environmental impact of waste while generating energy locally and cost-effectively.
Waste-to-energy (WTE) technologies also offer opportunities for agricultural farms to generate power from waste materials. This could include agricultural waste like straw, husks, or leaves, or even food processing waste, depending on the type of farm. By converting these materials into energy, farmers not only reduce waste but also avoid the high costs of waste disposal. WTE technologies can convert agricultural waste into electricity or thermal energy, supporting farm operations without relying on expensive fossil fuels or grid power.
Besides financial benefits, small-scale power plants in agriculture also support environmental sustainability. The farming industry is responsible for a significant share of greenhouse gas emissions, mainly from fossil fuel use and methane emissions from livestock. By using renewable energy, agricultural farms can drastically reduce their carbon footprint. Biomass and biogas systems, in particular, help to capture and utilize methane that would otherwise be released into the atmosphere, thus mitigating the environmental impact of agricultural waste.
Renewable energy systems also contribute to water conservation efforts on agricultural farms. For example, solar-powered irrigation systems are an effective solution for farms in regions with water scarcity. Solar panels can power pumps that deliver water to crops without relying on expensive and environmentally damaging diesel generators or grid electricity. This is particularly useful for small and medium-sized farms, where energy costs can account for a significant portion of operating expenses.
Energy independence is a key advantage for agricultural farms. By investing in small-scale power plants, farms can become less dependent on traditional energy sources, which are often subject to price hikes and supply disruptions. Power outages or grid instability can disrupt agricultural activities, leading to crop losses or equipment malfunctions. With a renewable energy system in place, such as a hybrid solar and wind power system, farmers can ensure a continuous energy supply, improving their resilience to external shocks.
One of the greatest benefits of small-scale power plants for agricultural farms is the positive impact on farm profitability. By reducing energy costs and improving operational efficiency, farmers can increase their profitability while ensuring long-term sustainability. Moreover, renewable energy can offer a way to diversify income streams for farms. For example, farmers with surplus energy may be able to sell it back to the grid, generating additional revenue and contributing to energy production in their communities.
Government incentives, grants, and subsidies are increasingly available for farmers who invest in renewable energy solutions. These financial support mechanisms make it easier for farms to implement small-scale power systems, reducing the initial capital investment required. Some governments offer tax credits, rebates, or low-interest loans to support farmers in transitioning to renewable energy, which can offset the costs of installation and maintenance.
Despite these advantages, there are challenges to implementing small-scale power plants on agricultural farms. One of the primary concerns is the upfront cost of installation, which can be a barrier for small or resource-limited farms. However, the long-term financial savings from reduced energy costs and the potential for income from energy sales can outweigh these initial costs over time. It’s important for farmers to conduct a feasibility study to determine the most cost-effective renewable energy system for their needs.
Additionally, the integration of renewable energy systems into existing farm infrastructure may require technical expertise and careful planning. Some farms may need to upgrade their electrical systems or install energy storage solutions, such as batteries, to store excess energy for later use. In areas with inconsistent sunlight or wind, energy storage becomes particularly important to ensure a reliable energy supply.
Despite these challenges, small-scale power plants present an exciting opportunity for agricultural farms to reduce their environmental impact, lower operating costs, and enhance energy resilience. As renewable energy technologies continue to evolve and become more affordable, their integration into agricultural operations will become increasingly common, ultimately contributing to a more sustainable and cost-effective future for farms.
In conclusion, small-scale power plants offer significant potential for agricultural farms to reduce energy costs, improve sustainability, and enhance operational efficiency. Solar, wind, biomass, and waste-to-energy technologies provide valuable opportunities for farms to harness local, renewable resources and reduce their dependence on external energy sources. By investing in small-scale power systems, farmers can improve their energy security, contribute to the fight against climate change, and ensure long-term financial success.
Small-scale power plants on agricultural farms can provide a wide range of benefits that align with both economic and environmental goals. The transition to renewable energy solutions is a smart investment for agricultural operations, offering long-term advantages in cost reduction, sustainability, and resilience. By integrating renewable energy technologies, farms can play a vital role in supporting global efforts to combat climate change while simultaneously improving their operational efficiency and productivity.
One of the primary advantages of small-scale power plants for agricultural farms is the reduction of energy costs. Agriculture, as a labor-intensive and energy-demanding sector, often relies heavily on electricity to power irrigation systems, refrigeration, heating greenhouses, and other essential activities. The costs associated with these energy demands can be substantial, particularly in regions where energy prices are volatile or when fossil fuels are the primary energy source. By investing in renewable energy systems such as solar, wind, or biomass, farms can significantly reduce their reliance on grid electricity, lowering their monthly energy bills and offering more predictable energy expenses.
Solar energy is one of the most accessible renewable energy options for agricultural farms. The installation of photovoltaic (PV) solar panels on barns, sheds, or fields allows farms to capture solar energy during the day, which can then be used to power irrigation systems, water pumps, lighting, and even cooling or heating systems for livestock or crops. In many rural areas, where electricity access may be intermittent or expensive, a solar power system offers reliable and independent energy generation. Additionally, solar power is a scalable solution, meaning farms of various sizes can install systems that meet their specific energy needs, from smaller systems for individual use to larger arrays for more energy-intensive operations.
Wind power is another renewable energy option that can complement solar energy, especially in areas with consistent wind patterns. Wind turbines can be installed on agricultural properties to generate electricity that powers equipment, lighting, or even heating systems for greenhouses. Wind power can be particularly beneficial for farms located in open, rural areas or coastal regions, where the wind is more consistent. When paired with solar energy, wind power helps create a hybrid system that can ensure continuous power supply, especially during periods when solar generation is low, such as cloudy or winter months.
Biomass energy is another powerful solution for farms, particularly those with access to organic waste, such as crop residues, livestock manure, or even food waste from farm operations. Biomass can be used to generate electricity or heat through processes like anaerobic digestion or combustion. For example, anaerobic digesters can convert manure and organic waste into biogas, which can then be used to generate electricity or fuel farm machinery. The use of biomass energy reduces the need for external fuel sources while also addressing the issue of waste disposal. In addition, the byproducts of biomass energy generation, such as compost or digestate, can be used as valuable fertilizers, creating a closed-loop system that enhances the farm’s sustainability and reduces waste.
Small-scale biomass power plants can also help farmers meet environmental regulations related to waste management and emissions. For example, in some regions, farmers are required to mitigate methane emissions from manure. By capturing and utilizing methane in a biogas system, farms can avoid the release of this potent greenhouse gas into the atmosphere while generating renewable energy.
Waste-to-energy (WTE) technologies can further enhance sustainability on agricultural farms. WTE systems can convert agricultural waste—such as crop residues, food processing by-products, and even plastic waste—into energy. These systems can generate electricity or thermal energy to power farm operations. Implementing waste-to-energy technologies not only provides a sustainable energy source but also helps farms reduce their waste disposal costs and minimize their environmental impact. As farms generate significant amounts of organic waste, WTE solutions can help close the loop by turning waste into a valuable resource.
The integration of renewable energy into farming operations also supports water conservation, a growing concern in many agricultural regions. Solar-powered water pumps and irrigation systems are an excellent way for farms to reduce their reliance on grid electricity for watering crops, particularly in areas where water scarcity is an issue. Solar-powered irrigation systems help reduce energy costs while ensuring that water is efficiently distributed to crops without the environmental impact of using diesel-powered pumps. In some cases, these solar systems can be paired with energy storage technologies like batteries, ensuring that power is available even during periods without sunlight, such as at night or on cloudy days.
Additionally, small-scale power plants offer agricultural farms the opportunity to increase their resilience and energy security. Traditional grid energy is subject to disruptions due to power outages, extreme weather events, or other unforeseen issues. For farms that rely on critical equipment—such as refrigeration for perishable products or irrigation for crops—a reliable energy supply is essential. By generating their own electricity from renewable sources, farms become less vulnerable to power shortages and outages. In the event of a power grid failure, farms with renewable energy systems can maintain operations and prevent losses in productivity, ensuring continued access to essential resources.
Energy independence is a key motivator for many farms that choose to install small-scale renewable power systems. When farms can generate their own energy, they are not subject to the fluctuating prices and external control of energy suppliers. In rural areas where access to the grid can be unreliable, self-sufficiency in energy is invaluable. In addition, many farms can take advantage of government incentives, tax credits, and grants that support the installation of renewable energy systems. These financial incentives help offset the initial capital costs associated with renewable energy technologies, making it easier for farms to transition to greener energy solutions without financial strain.
Furthermore, small-scale power plants can open new income-generating opportunities for farms. Farms that produce surplus renewable energy can often sell this energy back to the grid through power purchase agreements (PPAs) or net metering systems, creating a new revenue stream. By generating renewable energy, farms contribute to the broader energy ecosystem, supporting grid stability and reducing reliance on fossil fuels. This extra income can be used to reinvest in other aspects of the farm or to improve energy systems further, enhancing the farm’s sustainability in the long term.
As more agricultural operations implement renewable energy systems, the agricultural sector as a whole can significantly reduce its carbon footprint. The adoption of small-scale power plants helps decrease the reliance on fossil fuels, reducing greenhouse gas emissions associated with agricultural production. This is especially crucial as the agricultural sector is one of the largest contributors to global carbon emissions, and transitioning to renewable energy sources is a vital step in reducing the sector’s environmental impact.
In conclusion, small-scale power plants offer agricultural farms a range of benefits, including cost savings, energy independence, improved sustainability, and resilience against energy disruptions. By leveraging renewable energy sources like solar, wind, biomass, and waste-to-energy, farms can reduce their operating costs, contribute to environmental conservation, and enhance their productivity. As technology continues to advance and financial support mechanisms become more accessible, small-scale renewable energy solutions will become increasingly attractive for farms looking to secure a greener, more profitable future.
In addition to the environmental and economic benefits, small-scale power plants for agricultural farms can also enhance the farm’s long-term viability by improving operational efficiency and fostering innovation in farm management practices. With renewable energy systems in place, farms can adopt more advanced technologies, such as smart farming tools, automated irrigation, and precision agriculture systems that require consistent and reliable power sources.
Integration with Smart Farming Technologies
Small-scale power plants enable farms to integrate smart farming technologies, which can help optimize resource use and improve yields. For example, solar power can be used to run sensors and automated irrigation systems that monitor soil moisture, temperature, and other environmental factors. These systems can then automatically adjust irrigation schedules, ensuring that crops receive the right amount of water at the right time. This kind of precision irrigation reduces water wastage and ensures more efficient use of resources. The use of renewable energy for these advanced systems allows farms to not only optimize energy consumption but also improve crop yields by minimizing resource input, thereby making the farm more productive and sustainable.
In addition, renewable energy-powered systems can also support other smart farming solutions such as climate-controlled greenhouses, precision fertilization, and remote monitoring of crop health. These technologies are essential for increasing productivity, improving efficiency, and reducing costs, particularly in the face of challenges like climate change, labor shortages, and the need for sustainable farming practices.
Energy Storage and Management
An important consideration in implementing small-scale power plants on agricultural farms is energy storage. Since renewable energy generation can be intermittent, especially in solar and wind power systems, it is essential to incorporate energy storage solutions, such as batteries, to store excess energy. Energy storage ensures that farmers can access power when the sun isn’t shining or the wind isn’t blowing. This storage also provides energy security, as it mitigates the risk of power outages, ensuring that the farm’s operations continue smoothly even during times of low generation or grid instability.
Farmers can use energy storage to store excess energy generated during the day or during windy periods, which can then be used to power farm operations at night or when the energy demand is highest. This creates a reliable, uninterrupted power supply, reducing dependence on external grid electricity and enhancing the farm’s self-sufficiency. Additionally, energy management systems can be installed to optimize the use of stored energy, helping farmers to reduce overall energy consumption and minimize waste.
Carbon Footprint Reduction and Climate Change Mitigation
Agriculture is a major contributor to greenhouse gas emissions, particularly methane and nitrous oxide. These emissions arise from livestock, fertilizers, and fuel used in farming operations. By implementing small-scale power plants, particularly those based on biomass or biogas systems, farms can reduce their carbon footprint. Biomass systems, which convert organic materials such as crop waste, manure, and other agricultural by-products into energy, reduce the need for fossil fuels and prevent the release of greenhouse gases into the atmosphere.
Moreover, anaerobic digestion of livestock waste can capture methane, a potent greenhouse gas, and convert it into biogas, which can be used for electricity generation or heating. The use of biogas for power generation not only reduces emissions but also improves farm sustainability by reducing waste, providing renewable energy, and offering farmers an additional income stream by potentially selling excess power back to the grid.
Additionally, as global pressures to combat climate change continue to intensify, farms that adopt renewable energy systems can benefit from increasingly available carbon credits or other environmental incentives. Many governments and international organizations offer financial incentives to businesses that reduce their carbon footprints, and farms that transition to renewable energy could take advantage of these programs. These incentives can help offset installation costs, making renewable energy systems more affordable for farmers.
Resilience in the Face of Climate Change
Climate change is a growing concern for agricultural farms worldwide. More frequent and intense droughts, floods, and unpredictable weather patterns can disrupt farm operations, making the need for energy resilience even more urgent. Small-scale renewable energy systems offer farms a way to improve their resilience against these climate-related challenges. For example, farms using solar-powered irrigation systems are less vulnerable to water shortages, as they can continue to irrigate crops even during periods of drought or water scarcity.
In regions where grid power may be unreliable due to extreme weather events, small-scale renewable energy systems provide a reliable alternative that can keep farm operations running without interruption. Wind and solar systems are particularly well-suited to rural and remote areas, where infrastructure may be lacking or subject to frequent outages. By becoming energy-independent, farms can safeguard their productivity, reduce downtime, and maintain a steady income flow, even in the face of climate-related challenges.
Creating New Revenue Streams
Renewable energy systems also offer farms the opportunity to generate additional revenue by selling excess electricity to the grid. Many countries have net metering policies that allow farms to sell surplus energy back to the utility company, often at favorable rates. This provides a financial incentive for farms to invest in renewable energy, as the income from selling electricity can help offset the initial capital costs of installation.
In addition, farms that adopt renewable energy systems can use this as a marketing tool to attract customers who value sustainability and eco-friendly practices. Consumers are increasingly aware of the environmental impact of the products they buy, and farms that can demonstrate their commitment to sustainability through the use of clean energy may be able to command a premium price for their goods.
Community Engagement and Education
Farms that implement small-scale power plants can also act as leaders in their communities, promoting renewable energy adoption and sustainability. By demonstrating the benefits of renewable energy in agricultural operations, these farms can inspire others in the area to follow suit, helping to foster a culture of sustainability. In some cases, farms may even collaborate with local schools, community organizations, or government agencies to share their knowledge and experience with renewable energy, contributing to the wider adoption of clean energy solutions in rural areas.
Additionally, farmers who successfully implement small-scale renewable energy systems may have the opportunity to access government programs that support rural development and green energy initiatives. These programs can provide additional funding or resources that help support farmers in transitioning to renewable energy, further boosting the economic and environmental benefits.
Conclusion
The integration of small-scale power plants into agricultural farms offers a wealth of advantages, from reducing energy costs and increasing operational efficiency to promoting sustainability and improving resilience against climate change. By embracing renewable energy technologies like solar, wind, biomass, and waste-to-energy systems, farms can become more self-sufficient, reduce their environmental impact, and enhance their profitability. As energy costs rise and environmental concerns become more pressing, small-scale power plants provide a smart and sustainable solution for modern agriculture. With continued advancements in renewable energy technologies, financial incentives, and government support, the widespread adoption of small-scale power plants in agriculture is set to play a key role in shaping the future of farming.
Advancements in Renewable Energy Technologies for Agriculture
As renewable energy technologies continue to evolve, small-scale power plants for agricultural farms are becoming increasingly efficient and cost-effective. New innovations in solar panel design, wind turbine technology, and energy storage solutions are making it easier and more affordable for farmers to adopt these systems.
For example, advances in solar photovoltaic (PV) technology are improving the efficiency of solar panels, allowing farms to generate more energy with fewer panels. Additionally, the cost of solar panels has been steadily decreasing, making them more accessible to farms of various sizes. The ability to integrate solar power with battery storage systems also ensures that farms can store excess energy for use during periods when generation is low or demand is high. With improvements in battery technology, energy storage is becoming more affordable and capable of supporting farm operations around the clock.
Similarly, wind energy systems are becoming more efficient and better suited for smaller-scale applications. While traditional wind turbines may have been too large and costly for small farms, recent developments in small-scale wind turbines have made it possible for farmers to generate their own electricity in areas with suitable wind conditions. These new turbines are more affordable, easier to install, and require less maintenance, which makes them a viable option for agricultural operations seeking to diversify their energy sources.
Another promising development is the emergence of “agrivoltaics,” a concept that integrates solar power generation with agricultural activities. Agrivoltaics involves installing solar panels above crops or livestock areas to generate electricity while still allowing agricultural activities to take place beneath the panels. This approach maximizes land use, allowing farms to produce both food and energy. Agrivoltaics also offers the potential for better water retention and reduced heat stress for crops, which could be especially beneficial in regions experiencing increasing temperatures and water scarcity due to climate change.
Long-Term Sustainability and Circular Economy
Small-scale power plants can also play an essential role in creating a circular economy for farms. The concept of a circular economy emphasizes reducing waste, reusing resources, and maximizing efficiency throughout the production process. For agricultural farms, this model can be applied by using renewable energy to minimize external resource consumption and relying on the farm’s waste streams as valuable inputs for energy generation.
Biomass and waste-to-energy technologies are key components of this circular economy. Agricultural waste such as crop residues, manure, and food processing by-products can be converted into energy, helping to offset the need for fossil fuels. In addition to generating power, this approach addresses waste management concerns on the farm by turning what would otherwise be discarded into a valuable resource. Farmers can also use the byproducts of energy generation, such as compost or digestate from anaerobic digesters, as fertilizers for crops, creating a closed-loop system that benefits both the farm’s energy needs and soil health.
In a circular economy, small-scale power plants not only support energy independence but also contribute to reducing the environmental footprint of farming operations. By utilizing waste to generate energy and incorporating renewable energy systems into the farm’s operations, farmers can reduce the reliance on external resources, lower emissions, and improve the farm’s overall sustainability.
Collaboration and Community Impact
The adoption of small-scale renewable energy systems in agriculture can have broader social and economic benefits, especially in rural communities. As renewable energy infrastructure is developed on farms, it can help stimulate local economies by creating new jobs and supporting local industries. For example, farmers can collaborate with local energy service providers, engineers, and installers to develop and maintain their energy systems, creating employment opportunities in the renewable energy sector. Additionally, the installation of renewable energy systems may encourage the development of local supply chains for renewable technologies, further supporting regional economic growth.
Small-scale power plants can also help to strengthen rural communities by reducing energy poverty. Many rural areas, particularly those in developing countries, face challenges related to unreliable or unaffordable grid electricity. By implementing small-scale renewable energy solutions, agricultural farms can help address energy access issues for their communities. In some cases, farms with renewable energy systems may even share excess energy with local homes or businesses, enhancing energy access and fostering a sense of shared responsibility in promoting sustainable energy practices.
Conclusion: A Transformative Solution for Agriculture
The integration of small-scale renewable energy plants into agricultural operations represents a transformative opportunity to achieve greater sustainability, cost-effectiveness, and resilience. By utilizing solar, wind, biomass, and waste-to-energy technologies, farms can reduce their reliance on fossil fuels, lower energy costs, and improve their overall environmental footprint. Additionally, small-scale renewable energy systems offer farmers energy independence, resilience against climate disruptions, and the opportunity to generate additional revenue streams.
As technological advancements continue to improve the efficiency and affordability of renewable energy systems, and as financial support mechanisms become more accessible, small-scale power plants will become increasingly viable for agricultural operations of all sizes. Farms that embrace renewable energy solutions will be better positioned to navigate the challenges of climate change, energy volatility, and increasing consumer demand for sustainably produced goods.
By adopting renewable energy systems and contributing to the transition to a low-carbon economy, agricultural farms can play a critical role in addressing global sustainability challenges. Through innovation, collaboration, and a commitment to sustainable practices, small-scale power plants can help to ensure that agriculture remains a resilient, sustainable, and vital sector for generations to come.
Small Scale Power Plant for Gas Production Facilities
Small-scale power plants for gas production facilities offer a range of benefits that align with both operational needs and sustainability goals. Gas production, including natural gas, biogas, and other forms of gaseous fuels, requires a reliable and consistent energy supply to power various processes such as drilling, refining, and compression. Small-scale renewable energy systems, such as solar, wind, and biomass, can complement or fully meet the energy needs of gas production facilities, providing a more cost-effective and environmentally friendly solution.
Energy Independence and Cost Savings
One of the primary benefits of small-scale power plants for gas production facilities is the potential for energy independence and significant cost savings. Gas production operations require a substantial amount of energy for various processes, including drilling operations, gas compression, and water injection. Traditionally, these operations rely on grid electricity or on-site fossil fuel generation, both of which can be expensive and subject to price fluctuations. By incorporating renewable energy sources, such as solar or wind, gas production facilities can reduce their reliance on external energy sources and stabilize their energy costs.
For example, solar panels can be installed on-site to generate electricity for essential operations. During daylight hours, solar power can directly supply electricity to the gas production facility, reducing or eliminating the need to purchase power from the grid. Additionally, the integration of battery storage systems allows surplus energy generated during the day to be stored for use during nighttime or periods of high demand, ensuring continuous energy availability without the reliance on external sources. This energy independence provides financial stability and predictability, which is essential for gas production facilities looking to reduce operational costs.
Sustainability and Environmental Benefits
Another significant advantage of small-scale renewable energy systems in gas production facilities is the potential to reduce the environmental footprint of operations. The gas production sector, especially conventional natural gas production, has historically been associated with substantial greenhouse gas (GHG) emissions. The extraction, processing, and transport of natural gas contribute to the release of CO2 and methane, two major greenhouse gases responsible for global warming. By integrating renewable energy technologies into their operations, gas production facilities can offset some of their emissions, helping them meet sustainability targets and reduce their impact on climate change.
For example, solar and wind power generate electricity without producing emissions, providing a cleaner energy alternative to fossil fuel-based generation. Similarly, biogas systems, which convert organic waste into methane, can be used to generate electricity or provide heat for gas production facilities. Biomass energy, which uses agricultural waste or other organic materials, can be converted into electricity to power various gas production processes, reducing the need for grid electricity or fossil fuels.
By adopting renewable energy systems, gas production facilities can significantly reduce their carbon footprint and take a more proactive approach to environmental stewardship. This commitment to sustainability can help improve the facility’s public image, enhance corporate social responsibility (CSR) efforts, and meet regulatory requirements for emissions reduction.
Integration with Gas Production Processes
Renewable energy systems can be directly integrated into the gas production process to improve energy efficiency and reduce operational costs. For example, solar energy can be used to power various systems at a gas production facility, including monitoring equipment, safety systems, lighting, and heating. Wind power, particularly in areas with consistent wind patterns, can supplement the facility’s energy needs, ensuring that the production process continues without interruption.
Biogas and biomass systems, in particular, can be integrated into the gas production process in a way that creates a closed-loop energy system. For example, gas production facilities that deal with organic waste or by-products, such as agricultural or food processing waste, can convert this waste into biogas using anaerobic digestion. The biogas produced can then be used to generate electricity, heat, or even fuel the facility’s operations, further reducing the facility’s reliance on grid power or fossil fuels.
In addition, waste-to-energy (WTE) technologies can be deployed to convert non-recyclable waste materials into usable energy. Gas production facilities that generate waste during their operations—such as equipment waste, sludge, or spent materials—can use these WTE technologies to create additional energy while managing their waste. This reduces the need for external waste disposal services and helps further reduce the facility’s environmental footprint.
Energy Storage and Backup Systems
Energy storage is a critical component of renewable energy systems in gas production facilities, especially since renewable sources like solar and wind power are intermittent by nature. To ensure a constant and reliable energy supply, renewable energy systems should be paired with energy storage solutions such as batteries. These storage systems allow the facility to capture excess energy generated during periods of high production (e.g., during the day when solar energy is abundant) and store it for use during times when renewable generation is low (e.g., at night or during calm periods for wind power).
In addition to providing a steady energy supply, energy storage systems can also act as backup power during grid outages, which is particularly important for gas production facilities that rely on continuous power for operations. In the event of a power outage, stored energy from batteries can be used to keep critical systems running, ensuring minimal disruption to operations.
Carbon Credit Opportunities
As gas production facilities strive to reduce their environmental impact, adopting renewable energy systems can also open up opportunities for carbon credits. Many countries and regions offer carbon credit programs that incentivize businesses to reduce their carbon emissions by implementing green energy solutions. By adopting renewable energy technologies and reducing reliance on fossil fuels, gas production facilities can earn carbon credits, which can either be sold on the carbon market or used to offset their own emissions.
These carbon credits provide an additional revenue stream for gas production facilities, which can be used to further reinvest in sustainability projects or offset the costs of renewable energy system installations. This can enhance the financial benefits of adopting renewable energy systems while also contributing to global efforts to reduce greenhouse gas emissions.
Resilience and Risk Management
Gas production facilities, especially those located in remote or rural areas, may face challenges related to power reliability and energy access. Power outages or interruptions in energy supply can disrupt production and lead to significant financial losses. Small-scale renewable energy systems can improve the resilience of these facilities by providing a more reliable and independent energy source.
By using renewable energy sources such as solar, wind, or biogas, gas production facilities can reduce their vulnerability to power grid failures, ensuring that critical operations continue even in the face of external disruptions. For example, solar-powered backup systems can provide immediate support during an outage, while wind turbines can supplement power during periods of low sunlight. This energy independence can be particularly valuable for remote facilities that may not have access to reliable grid infrastructure.
Cost-Effectiveness and Financial Incentives
The initial capital investment for renewable energy systems, including solar panels, wind turbines, and biomass generators, can be significant. However, the long-term financial benefits of adopting renewable energy far outweigh the initial costs. Gas production facilities that invest in renewable energy systems can significantly reduce their energy bills, often recouping the initial investment over a period of several years through savings on electricity costs.
In many countries, government incentives, grants, and tax credits are available to help offset the upfront costs of renewable energy projects. These financial incentives can make renewable energy systems more accessible to gas production facilities, accelerating their adoption. In addition, the ability to sell excess energy back to the grid through net metering or power purchase agreements (PPAs) can generate additional income, further improving the financial feasibility of these projects.
Conclusion
Small-scale power plants for gas production facilities offer a variety of benefits, ranging from energy independence and cost savings to sustainability and operational resilience. By adopting renewable energy systems such as solar, wind, biomass, and waste-to-energy, gas production facilities can reduce their reliance on fossil fuels, decrease their environmental impact, and improve their bottom line. As the energy landscape evolves, renewable energy technologies will become increasingly important for gas production facilities seeking to future-proof their operations, meet regulatory requirements, and contribute to global sustainability efforts.
Incorporating renewable energy into gas production facilities is not just an environmental or financial decision; it is a strategic choice that helps ensure the long-term viability of operations. Through continued innovation and investment in renewable energy technologies, gas production facilities can reduce costs, increase resilience, and enhance their sustainability, all while contributing to the broader goal of reducing carbon emissions and mitigating the effects of climate change.
Small-Scale Power Plants for Gas Production Facilities
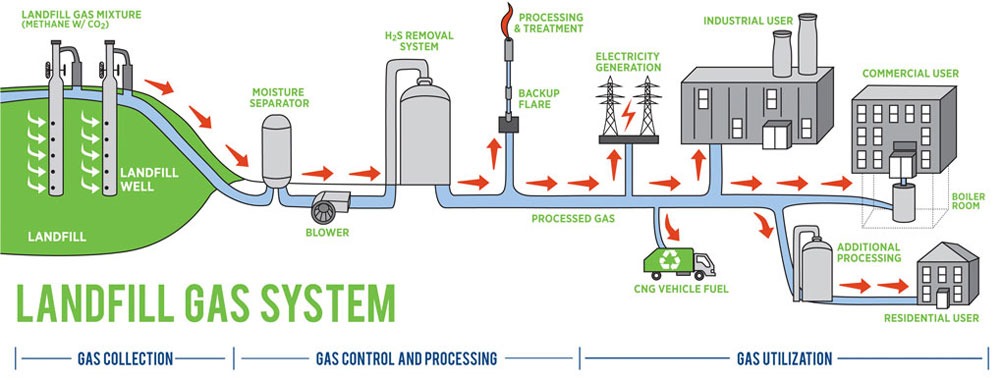
Energy Independence and Cost Savings
Small-scale power plants for gas production facilities are particularly valuable because they provide an opportunity for energy independence, reducing reliance on external energy sources and offering significant cost savings. Gas production facilities often operate in energy-intensive environments, requiring a reliable and steady energy supply for a wide variety of processes like drilling, compression, and transportation. Traditionally, these facilities depend on the grid or conventional fossil fuels to meet their energy needs, which can lead to high operating costs and exposure to fluctuating energy prices.
By implementing small-scale renewable energy solutions, such as solar, wind, or biomass systems, gas production facilities can generate their own electricity. This directly reduces their dependence on external energy providers and insulates them from the volatility of energy prices. For example, solar energy can be harnessed through photovoltaic (PV) panels installed at the production site. The energy generated from these panels can be used to power operations, including lighting, monitoring equipment, and non-essential systems, which lowers the overall electricity costs. This type of energy generation is highly cost-effective over time, especially as technology improves and installation costs decrease.
Additionally, facilities can pair renewable energy systems with energy storage solutions. Using batteries, surplus energy produced during the day can be stored and used during non-generation hours, ensuring continuous operation and minimizing any gaps in energy supply. In areas with frequent power outages or energy supply instability, energy storage also acts as a backup to maintain critical operations during periods when the external grid is unavailable.
The long-term cost-saving benefits are significant. By producing energy on-site, gas production facilities can potentially offset the cost of grid-supplied electricity, reducing operating expenses and increasing profitability. Additionally, some renewable energy sources like wind and solar have low operating and maintenance costs once installed, meaning that over time, the energy costs can decrease substantially.
Sustainability and Environmental Impact
Reducing environmental impact is another key advantage of integrating small-scale renewable energy into gas production operations. While gas itself is often seen as a cleaner alternative to coal, the production process can still have a significant environmental footprint due to emissions associated with drilling, transportation, and other operational activities. Furthermore, in certain cases, the natural gas extraction process may result in methane leaks, which are a potent greenhouse gas.
By incorporating renewable energy systems such as solar, wind, and biogas into their operations, gas production facilities can minimize their overall environmental impact. Solar and wind energy provide electricity without producing harmful emissions, and when coupled with energy storage systems, can substantially reduce the carbon footprint of a facility. This shift toward clean energy helps facilities lower their dependence on fossil fuels and reduce overall GHG emissions, aligning with global sustainability efforts.
Biogas and biomass systems, in particular, offer opportunities to reduce emissions while generating usable energy. Biogas production, for instance, captures methane from organic waste such as agricultural residue or food processing by-products and converts it into usable energy. Not only does this reduce methane emissions from organic waste, but it can also power gas production operations in an eco-friendly manner, creating a circular economy on-site.
By transitioning to renewable energy sources, gas production facilities can demonstrate leadership in environmental responsibility, improving their public image and corporate sustainability profile. Furthermore, adopting green energy technologies can help the facility meet stricter environmental regulations and achieve corporate sustainability goals. In many regions, governments are introducing stricter regulations on industrial emissions, and businesses that embrace cleaner technologies will be better positioned to comply with evolving environmental standards.
Integration with Gas Production Processes
Renewable energy systems can be seamlessly integrated into gas production operations, optimizing energy usage while reducing costs. For instance, solar energy can be directly applied to power electrical equipment, such as pumps, sensors, compressors, and monitoring systems, which are vital in the production and distribution of gas. In locations with strong and consistent wind patterns, wind energy could be harnessed to supplement or fully supply power needs, particularly during times when solar generation is not sufficient, such as at night or during cloudy days.
Biogas systems can be integrated into the overall energy generation process at gas production facilities, especially if the facility produces organic waste as a byproduct. Through anaerobic digestion, organic waste materials are broken down to produce biogas, which can then be burned to generate electricity. This not only powers the gas production facility but also helps manage waste in an environmentally responsible way. For example, waste materials such as food scraps, agricultural waste, or even sludge from other operations can be used to generate biogas, turning waste into a valuable resource.
In some cases, waste-to-energy technologies may be incorporated into the facility’s energy production. These systems convert non-recyclable waste materials into energy, providing an additional way to produce power while managing waste effectively. Gas production operations that generate a significant amount of waste can benefit from waste-to-energy systems, improving overall sustainability by reducing waste disposal costs and turning that waste into electricity.
These systems can significantly reduce the operational costs of running gas production facilities, while also contributing to the facility’s sustainability goals by utilizing available local resources to generate power.
Energy Storage and Backup Systems
Energy storage systems are a vital component of small-scale power plants, especially when renewable energy sources such as wind and solar are utilized. These sources are intermittent, meaning they do not always produce energy at the same rate or time that it is needed. To address this, energy storage systems, typically in the form of batteries, can be used to store excess energy generated during high-production periods, such as during the day when solar panels are generating power. This stored energy can then be drawn upon during periods when renewable energy generation is low, such as at night or during cloudy weather.
The use of energy storage also provides a backup system in the event of a grid failure or an outage in the facility’s main energy supply. In critical operations like gas production, where downtime can lead to significant losses, having a reliable backup energy source is crucial. Storage systems provide an additional layer of security by ensuring that power is always available, maintaining operations even in times of unexpected power disruptions.
Revenue Generation from Carbon Credits
Gas production facilities can also benefit from carbon credit programs. These programs incentivize businesses that reduce their carbon footprint by providing credits that can be traded on carbon markets. When gas production facilities integrate renewable energy systems, they reduce their GHG emissions, qualifying them for carbon credits. These credits can either be sold to other businesses looking to offset their own emissions or used to fulfill the facility’s emission reduction goals.
By generating additional revenue through carbon credits, gas production facilities can offset some of the initial costs associated with implementing renewable energy systems. This offers an added financial incentive to invest in renewable energy, improving the financial feasibility of the transition to clean energy.
Conclusion
Incorporating small-scale renewable energy systems into gas production facilities offers a variety of benefits that go beyond simple cost savings. These systems help gas producers become more energy independent, environmentally responsible, and resilient to external disruptions. Solar, wind, biogas, and waste-to-energy technologies all offer practical solutions for reducing operational costs, improving sustainability, and meeting evolving regulatory requirements.
By embracing renewable energy, gas production facilities can reduce their reliance on fossil fuels, mitigate their environmental impact, and enhance their overall efficiency. As the renewable energy landscape continues to evolve and technologies become more accessible and cost-effective, small-scale power plants are increasingly becoming a critical component of the future of gas production. They provide an opportunity to balance the demand for energy with the need to reduce emissions, supporting long-term sustainability goals and ensuring that gas production facilities can remain competitive in an increasingly green and energy-efficient market.
Resilience and Risk Management
One of the significant challenges for gas production facilities, particularly those in remote or offshore locations, is the vulnerability to energy supply interruptions. Natural disasters, grid failures, or energy price hikes can disrupt operations, leading to production downtimes that cost both time and money. Small-scale renewable energy systems can help mitigate these risks by providing more resilient and decentralized power generation solutions.
For instance, a facility located in a region prone to power outages or grid instability can benefit from having an independent energy generation system powered by solar, wind, or biomass. Solar power systems can provide constant energy during the day, while wind turbines can supplement energy during periods of low sunlight. With an energy storage solution (such as batteries), the facility can store excess energy generated during peak production times and use it when external power sources are unavailable.
Additionally, renewable energy systems provide a significant level of operational flexibility and backup capacity, which is essential for critical processes at gas production facilities. During grid outages, on-site renewable energy systems can be automatically switched on to maintain the power supply for essential operations, such as safety systems, gas monitoring equipment, and communication infrastructure. This reduces the facility’s vulnerability to external power disruptions, providing an extra layer of reliability and helping avoid costly interruptions.
Moreover, facilities using renewable energy systems can be more adaptable to regional energy policies and regulations, including growing decarbonization targets and carbon tax initiatives. As governments around the world introduce stricter emissions reduction measures, gas production companies that incorporate renewable energy into their operations can better meet these regulatory requirements, ensuring they remain compliant without having to make costly retrofits or changes later on.
Flexibility and Scalability
Another key advantage of small-scale renewable energy systems is their inherent flexibility and scalability. Unlike large centralized power plants, small-scale systems can be tailored to meet the specific energy needs of a gas production facility. This makes it easier to design a solution that matches the facility’s energy consumption profile while remaining cost-effective.
Solar, wind, and biogas systems can all be installed in stages, meaning a facility can start with a smaller system and expand it over time as energy needs grow or as technologies improve. For instance, if a facility initially installs a solar array to supplement its energy supply, it can expand the system as demand increases or as energy production from other sources fluctuates. Similarly, small-scale biogas plants can be scaled up or down based on the availability of organic waste materials and the facility’s energy requirements.
This scalability is particularly valuable for gas production operations that are in the early stages of development or those that may have varying energy demands over time. As production scales, renewable energy solutions can be incrementally expanded to match the increased power needs without requiring large upfront investments. Furthermore, renewable energy technologies like solar and wind have the potential to be deployed in modular formats, which increases the facility’s flexibility in terms of integration with other operational systems.
Long-Term Financial Benefits
While the initial capital investment for renewable energy systems can be significant, the long-term financial benefits are compelling. By switching to renewable energy, gas production facilities can significantly reduce their dependence on grid power or costly fossil fuel-generated electricity. Over time, the savings on electricity bills can more than compensate for the upfront investment in solar panels, wind turbines, or biogas equipment.
Additionally, renewable energy technologies like solar and wind have low maintenance costs once installed. Unlike fossil fuel-powered generators, which require ongoing fuel and maintenance expenses, renewable systems only require periodic inspections and maintenance, which keeps operational costs low. Furthermore, renewable energy equipment is designed for long lifespans, often with warranties extending beyond 20 years, providing a stable energy supply with minimal ongoing costs.
Government subsidies, tax incentives, and carbon credit revenue further improve the financial viability of small-scale power plants for gas production. Many governments worldwide offer incentives to businesses that invest in renewable energy, helping to offset the installation and operational costs. Carbon credits earned from reducing emissions through the use of renewable energy can also be sold to other companies looking to offset their own emissions. This revenue stream can provide additional financial relief and improve the return on investment for renewable energy projects.
Over the long term, the combination of savings, low maintenance costs, and potential revenue from carbon credits can turn a renewable energy investment into a major financial asset for gas production facilities.
Opportunities for Innovation
Small-scale renewable energy systems also present significant opportunities for innovation within the gas production industry. The integration of smart technologies, such as energy management systems, allows for greater control over energy production and consumption. These systems can optimize the usage of renewable energy by automatically switching between solar, wind, biogas, or grid power based on real-time conditions and energy demand. Smart meters and monitoring systems can also provide real-time data on energy generation, usage, and storage, enabling operators to make more informed decisions regarding energy management.
Moreover, advances in energy storage technology, such as solid-state batteries and grid-scale storage solutions, continue to improve the efficiency and affordability of renewable energy systems. Gas production facilities that invest in these innovations can benefit from enhanced energy storage capabilities, helping to maximize the use of renewable energy and reduce reliance on fossil fuels.
Additionally, research and development into hybrid energy systems that combine multiple renewable energy sources—such as solar-wind-biomass—could further enhance the overall sustainability and efficiency of gas production operations. These hybrid systems can be customized to exploit local resources and provide continuous power generation, even when one energy source is less available. For example, integrating solar panels and wind turbines can offer complementary energy production patterns—solar power is abundant during the day, while wind power may be available during the night or in windy weather conditions.
Public Perception and Corporate Image
Adopting small-scale renewable energy systems can have a positive impact on a gas production facility’s public image. As consumers, governments, and investors place increasing importance on sustainability, demonstrating a commitment to clean energy can enhance a company’s reputation. Consumers are more likely to support businesses that actively reduce their environmental footprint, while investors are increasingly prioritizing companies with strong sustainability practices.
By integrating renewable energy, gas production facilities can position themselves as leaders in the transition toward cleaner energy, helping to build trust with stakeholders and improve their competitive advantage. In some cases, being recognized for sustainable practices can even open up new markets, partnerships, and business opportunities.
Corporate social responsibility (CSR) initiatives focused on renewable energy adoption can also help gas production companies attract top talent and foster employee satisfaction. Many employees are motivated by working for companies that prioritize sustainability and contribute to positive environmental change. This focus on clean energy not only helps meet global climate goals but also improves employee morale and retention.
Conclusion
The integration of small-scale renewable energy systems into gas production facilities presents a wide range of benefits, from reducing operational costs and increasing energy independence to improving sustainability and public perception. Renewable energy sources like solar, wind, and biogas offer gas production facilities the opportunity to decrease their reliance on external energy sources, lower emissions, and create a more resilient and efficient energy infrastructure. With ongoing advancements in renewable energy technology, energy storage, and energy management systems, the future of small-scale power plants in the gas production industry looks promising.
As gas production facilities adopt renewable energy solutions, they not only position themselves as responsible corporate citizens but also take a strategic step toward future-proofing their operations. Whether it’s through cost savings, risk management, innovation, or environmental stewardship, small-scale renewable energy systems will continue to play an increasingly important role in the sustainable development of the gas production sector.
Cost Comparison with Traditional Power Supply Sources
The financial viability of small-scale power plants for gas production facilities is further highlighted when comparing the costs of renewable energy sources with traditional power supply options. Traditional energy sources, particularly those reliant on fossil fuels, often come with fluctuating prices and exposure to market volatility, which can impact a facility’s budget and long-term financial planning. In comparison, renewable energy technologies offer a more predictable and stable cost structure, especially over the long term.
For example, the cost of solar power generation has seen a dramatic decrease in recent years, making it one of the most cost-effective energy sources for small-scale power plants. The price per watt for solar panels has dropped significantly, while efficiency improvements in solar technology have led to greater energy production from the same amount of panel area. Similarly, wind energy costs have also decreased due to advancements in turbine technology and increased deployment, making wind power a more economically viable option for gas production facilities.
While the upfront capital cost of installing renewable energy systems such as solar panels or wind turbines may still be higher compared to traditional fossil fuel-based generators, the ongoing operational and maintenance costs are substantially lower. Traditional power generation methods often rely on expensive fuel inputs, such as natural gas, coal, or diesel, which can lead to rising costs, especially as fuel prices fluctuate. On the other hand, solar, wind, and other renewable energy sources typically have very low marginal costs, with much of the expense front-loaded in the installation phase. Once the system is installed, the ongoing energy production costs are minimal, and the facility can take full advantage of the free energy provided by the sun, wind, or biomass.
Additionally, with the integration of energy storage solutions, the cost of backup power becomes more predictable, further reducing the financial risks associated with relying on external power sources. The long-term return on investment (ROI) for renewable energy systems tends to be favorable, as the costs of operation become much lower over time compared to conventional energy supply options.
Local Economic Development and Job Creation
Another significant benefit of implementing small-scale power plants at gas production facilities is the potential for local economic development. Renewable energy systems such as solar, wind, and biogas require a combination of construction, installation, and ongoing maintenance, all of which create local jobs. In many cases, small-scale renewable energy projects are labor-intensive during the installation phase, requiring skilled workers such as electricians, engineers, and technicians. Once the system is operational, there are also opportunities for ongoing maintenance and repair, which can provide additional employment in the community.
In areas where gas production facilities are located, particularly in remote or underdeveloped regions, these job creation opportunities can have a significant positive impact on the local economy. By developing renewable energy infrastructure, gas production facilities can contribute to local job markets, improve workforce skills, and support local businesses. Additionally, renewable energy projects often create opportunities for collaboration between the facility and local governments or organizations, leading to enhanced community relations and potential partnerships that benefit both the business and the local economy.
Furthermore, the use of locally sourced renewable energy can foster a sense of energy security within the community. The local generation of power reduces reliance on outside suppliers and contributes to the local economy, keeping energy costs within the region and reducing the external financial outflows that would typically be spent on imported fossil fuels.
Energy Efficiency and Reduced Operational Costs
The integration of renewable energy into gas production facilities can also improve overall energy efficiency. Energy efficiency is a critical factor for any industrial operation, as reducing energy consumption can lead to significant cost savings and productivity gains. By adopting renewable energy sources, gas production facilities can make use of cleaner and more efficient energy production technologies, reducing energy waste and increasing operational efficiency.
For example, the installation of energy-efficient lighting and equipment powered by renewable energy can reduce the facility’s overall electricity consumption. Smart energy management systems can optimize the use of renewable energy and storage systems, ensuring that energy is used more effectively and reducing the facility’s overall demand on the grid. These smart systems can also provide real-time insights into energy consumption patterns, helping the facility identify areas for further efficiency improvements.
In addition to directly reducing energy costs, energy efficiency also contributes to a more sustainable operation by reducing the carbon footprint of the facility. As the facility uses less energy and relies more on clean, renewable sources, its environmental impact decreases, improving its reputation as an environmentally responsible operator.
Government Incentives and Subsidies
Governments worldwide are increasingly offering incentives, grants, and subsidies to encourage businesses to transition to renewable energy sources. For gas production facilities considering small-scale renewable energy plants, these financial incentives can significantly reduce the upfront costs of installation and accelerate the return on investment. These incentives are often aimed at reducing carbon emissions, promoting energy independence, and encouraging sustainable development in the industrial sector.
For example, tax credits, direct subsidies, or government-backed loans are commonly available for businesses investing in renewable energy systems. In many cases, these incentives can cover a portion of the initial capital costs associated with renewable energy installations. Additionally, renewable energy projects may qualify for grants aimed at reducing emissions, improving energy efficiency, or advancing technology development. By taking advantage of these financial programs, gas production facilities can lower their capital expenditures and increase the affordability of renewable energy integration.
Some regions may also offer feed-in tariffs or power purchase agreements (PPAs) for businesses generating renewable energy. These programs guarantee a fixed payment or purchase price for the energy generated, providing additional financial security and incentives for renewable energy projects. In some cases, businesses can even sell excess energy back to the grid, creating a new revenue stream.
Decentralized Energy Production and Grid Support
A key advantage of small-scale power plants for gas production facilities is the decentralized nature of energy generation. Traditional power plants are often large and centralized, relying on a complex grid infrastructure to transmit energy over long distances. This system can be inefficient and prone to vulnerabilities, such as transmission losses, grid failures, or power shortages.
In contrast, small-scale power plants allow for localized, decentralized energy generation. By producing energy on-site, gas production facilities reduce their reliance on the central grid, minimizing transmission losses and providing a more efficient energy supply. This decentralized approach also provides the facility with greater control over its energy production, reducing the risk of external disruptions and offering more flexibility in energy management.
In addition to benefiting the gas production facility, decentralized energy systems can support the broader energy grid by providing additional capacity during peak demand periods. When small-scale power plants generate excess energy, this power can be fed back into the grid, helping to stabilize the overall energy supply. This concept, known as “distributed generation,” is becoming increasingly important as industries and communities look for ways to support grid resilience and integrate renewable energy into the larger energy infrastructure.
Conclusion
Small-scale renewable energy systems for gas production facilities provide numerous financial, environmental, and operational benefits. From reducing energy costs and improving energy independence to creating local jobs and supporting sustainability goals, these systems offer a sustainable alternative to traditional fossil fuel-based energy solutions. Solar, wind, and biogas systems offer a flexible, cost-effective, and scalable approach to energy generation that can be tailored to the specific needs of gas production operations.
In addition to the financial savings, small-scale power plants enhance operational resilience, reduce carbon emissions, and contribute to the facility’s long-term sustainability. By integrating renewable energy, gas production facilities can improve their bottom line, meet evolving regulatory requirements, and enhance their reputation as responsible corporate citizens. As renewable energy technologies continue to advance and become more affordable, the role of small-scale power plants in the gas production sector will only grow, providing a cleaner, more efficient, and more reliable energy solution for the future.
Small Scale Power Plant for Oil Refineries
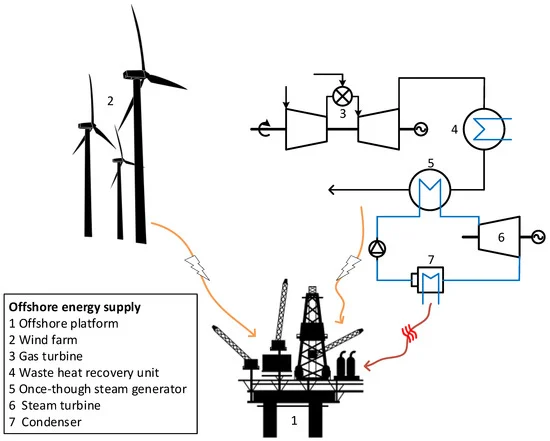
Energy Demand in Oil Refineries
Oil refineries are energy-intensive facilities that require large amounts of electricity and thermal energy to process crude oil into various refined products. The refining process includes several stages such as distillation, cracking, reforming, and treating, each of which consumes significant amounts of energy. Typically, oil refineries rely on the external grid or on-site power generation through fossil fuel combustion for their energy needs. However, as the demand for energy grows and environmental regulations become more stringent, oil refineries are increasingly looking for alternative power sources to reduce their reliance on fossil fuels and enhance operational efficiency.
Small-scale power plants powered by renewable energy can provide a sustainable, cost-effective solution to meet the energy needs of oil refineries. These power plants can supplement or even replace part of the energy supplied by the grid or fossil fuels, helping refineries lower their operating costs, reduce their carbon emissions, and achieve energy independence.
Integration of Renewable Energy in Refineries
Integrating renewable energy sources such as solar, wind, and biomass into an oil refinery’s power supply offers several benefits, including improved sustainability, reduced energy costs, and enhanced resilience. The integration of renewable energy technologies into existing refinery operations can be done without disrupting ongoing production, thanks to the modular and scalable nature of small-scale power plants.
- Solar Power: Solar power is a versatile renewable energy option that can be integrated into the roof space or land surrounding an oil refinery. Photovoltaic (PV) panels can be installed to generate electricity, which can be used to power refinery operations or stored for later use in energy storage systems like batteries. Solar energy is particularly advantageous in regions with abundant sunlight, providing a reliable source of power during the day and reducing the refinery’s dependence on external grid electricity.
- Wind Power: Wind energy is another renewable source that can complement solar power in regions where wind conditions are favorable. Small-scale wind turbines can be deployed on-site or in nearby areas to generate electricity. Wind power can be particularly useful during periods of low sunlight or at night, when solar power is not available. Combined with solar power, wind turbines provide a more consistent and reliable energy supply, helping to stabilize the refinery’s power grid.
- Biomass: Refineries often produce waste products like organic matter, which can be used as feedstock for biomass energy generation. Biomass power plants can convert these byproducts into electricity, providing an additional renewable energy source for the refinery while reducing waste disposal costs. Biomass energy systems can also operate continuously, providing a reliable base-load power source.
By diversifying the energy sources used at an oil refinery, small-scale power plants can provide a stable and cost-effective energy supply that reduces the refinery’s reliance on traditional energy sources, cuts energy costs, and supports sustainability goals.
Cost Savings and Efficiency
One of the primary reasons oil refineries are turning to small-scale renewable energy solutions is the potential for cost savings. Traditional fossil fuel-based power generation relies on purchasing expensive fuel, which is subject to market fluctuations. In contrast, small-scale renewable energy systems, once installed, have low operational and maintenance costs. With solar, wind, and biomass energy systems, refineries can benefit from free or low-cost energy generation, reducing their dependence on external energy suppliers.
Renewable energy systems can also improve the overall energy efficiency of oil refineries. By generating energy on-site, refineries can reduce transmission losses that typically occur when power is transmitted over long distances from the grid. Additionally, renewable energy systems can be paired with energy storage solutions to optimize energy use and ensure a consistent power supply. Excess energy generated during peak production times can be stored and used during periods of low production or high demand, helping to balance energy usage throughout the day.
Energy efficiency improvements extend beyond the direct use of renewable energy. By integrating advanced energy management systems, oil refineries can monitor and control energy consumption more effectively. These systems can optimize the use of renewable energy, ensuring that the refinery operates at maximum efficiency while minimizing waste. As a result, oil refineries can lower their energy consumption, reduce their carbon footprint, and achieve better overall energy performance.
Environmental Impact and Emissions Reduction
Oil refineries are major contributors to greenhouse gas (GHG) emissions, primarily due to their reliance on fossil fuels for energy production. These emissions contribute to climate change and can lead to increased environmental regulation and costs. By incorporating renewable energy systems, oil refineries can significantly reduce their GHG emissions and improve their environmental sustainability.
Solar, wind, and biomass power generation systems produce little to no carbon emissions, making them an ideal solution for refineries seeking to reduce their carbon footprint. For example, using solar power to replace conventional grid electricity can reduce emissions associated with coal- or natural gas-based power generation. Wind and biomass energy systems can provide similar emissions reductions, helping the refinery comply with stringent environmental regulations and meet sustainability targets.
Additionally, by using locally sourced renewable energy, oil refineries can reduce the need for imported fuels, helping to reduce their reliance on fossil fuel extraction and transportation, which also contributes to emissions. Over time, the transition to renewable energy can result in significant long-term reductions in a refinery’s overall environmental impact.
Energy Security and Resilience
Oil refineries are critical infrastructure, and any disruptions to their energy supply can result in costly downtime and production losses. Small-scale renewable energy systems can provide energy security and enhance resilience, especially in regions where the grid is unreliable or vulnerable to natural disasters.
With decentralized power generation, oil refineries are less dependent on external power sources and can maintain operations even in the event of grid failures or fuel shortages. Renewable energy systems such as solar panels, wind turbines, and biomass plants can continue generating power independently of the central grid, ensuring that the refinery has a constant supply of energy.
Energy storage systems, such as lithium-ion batteries, can further improve resilience by storing excess energy generated during peak production times and providing power during periods of grid instability or high demand. These systems can be integrated with renewable energy sources to create a hybrid energy system that offers both flexibility and reliability.
In the event of a grid outage or fuel supply disruption, on-site renewable energy generation can act as a backup, providing a critical layer of protection to ensure that refinery operations continue without interruption.
Regulatory Compliance and Corporate Responsibility
As governments worldwide introduce stricter regulations on emissions and energy consumption, oil refineries face growing pressure to reduce their environmental impact and adopt more sustainable practices. Small-scale renewable energy systems offer a way for refineries to comply with these regulations while also demonstrating corporate responsibility.
Governments are increasingly offering incentives, tax credits, and grants to businesses that invest in renewable energy, including oil refineries. By adopting renewable energy solutions, refineries can reduce their carbon emissions and improve their sustainability credentials, positioning themselves as leaders in the transition to clean energy. This not only helps to meet regulatory requirements but also improves the refinery’s public image, attracting investors and customers who value environmental sustainability.
Additionally, renewable energy adoption can help refineries avoid potential penalties or taxes related to carbon emissions and energy consumption. By proactively adopting clean energy technologies, refineries can reduce their exposure to future regulatory risks and improve their ability to adapt to evolving environmental policies.
Financial Incentives and ROI
Although the initial capital cost of installing renewable energy systems can be high, the long-term financial benefits make it a worthwhile investment for oil refineries. In addition to the savings on energy costs, oil refineries can take advantage of various financial incentives, such as government subsidies, tax credits, and grants, to reduce the upfront installation costs of renewable energy systems.
For example, tax incentives like the Investment Tax Credit (ITC) in the United States offer significant deductions for businesses that install renewable energy systems. Similar incentives are available in other countries, helping to offset the initial cost of renewable energy infrastructure. By taking advantage of these incentives, oil refineries can lower their capital expenditures and accelerate the return on investment (ROI) for renewable energy projects.
Once installed, renewable energy systems offer a predictable and stable source of energy, reducing the refinery’s exposure to fuel price fluctuations and energy market volatility. Over time, the savings on energy costs, combined with the environmental benefits and incentives, make renewable energy investments increasingly financially attractive.
Conclusion
Small-scale power plants powered by renewable energy sources offer significant advantages for oil refineries, including cost savings, improved energy security, reduced environmental impact, and compliance with evolving regulations. By integrating solar, wind, or biomass energy systems into their operations, oil refineries can reduce their reliance on fossil fuels, cut energy costs, and contribute to a more sustainable energy future. These systems provide a flexible, scalable, and resilient energy solution that enhances operational efficiency, reduces emissions, and ensures the refinery remains competitive in an increasingly environmentally conscious world.
As renewable energy technologies continue to advance and become more affordable, the role of small-scale power plants in oil refineries will only grow, providing an essential tool for the industry to transition toward cleaner, more sustainable energy practices. Through this transition, oil refineries can not only improve their bottom line but also play a crucial role in the global shift toward a low-carbon, sustainable energy economy.
Cost Savings and Return on Investment (ROI)
One of the most compelling reasons for oil refineries to invest in small-scale renewable energy systems is the potential for significant cost savings over the long term. Traditional fossil fuel-based power generation often involves high operational costs due to the purchase and transportation of fuel, which is subject to market fluctuations. In contrast, renewable energy systems, such as solar, wind, or biomass, have minimal fuel costs after the initial capital investment for equipment and installation.
The primary cost for renewable energy systems lies in the upfront investment for infrastructure, such as solar panels, wind turbines, or biomass conversion facilities. However, as the energy produced is essentially free (apart from minimal maintenance and operational expenses), these systems can quickly pay for themselves in terms of reduced energy costs.
The return on investment (ROI) for small-scale renewable energy plants at oil refineries is typically favorable due to the savings on energy bills, especially in regions with high energy costs or unreliable grid power. Additionally, the lifespan of renewable energy technologies, such as solar panels (which can last 25-30 years), provides an extended period of financial benefit.
Oil refineries can also save on energy procurement costs by generating a significant portion of their own energy on-site, reducing dependence on the grid or external suppliers. This can stabilize energy costs and shield the refinery from energy price volatility. As a result, small-scale renewable energy projects have the potential to provide a stable, long-term stream of savings and deliver a solid ROI for the refinery.
Improved Energy Efficiency Through Smart Systems
To further enhance energy efficiency, oil refineries can integrate smart energy management systems with their small-scale renewable energy plants. These systems help optimize energy production and consumption by analyzing real-time data and adjusting energy usage patterns to match production needs. For example, energy management software can predict peak demand times, monitor renewable energy production, and automatically control energy storage systems to ensure that the facility uses the most cost-effective energy available.
These smart systems can help minimize energy waste, identify inefficiencies, and ensure that the refinery is using its renewable energy sources as efficiently as possible. They can also help extend the life of the renewable energy equipment by preventing overuse and maintaining the systems at optimal performance levels.
In addition, the use of smart grids and energy storage systems can help oil refineries balance supply and demand, ensuring that excess energy generated by the renewable system during peak production times is stored for later use. This ensures that the refinery always has access to a stable energy supply, even when renewable energy production fluctuates due to weather or other factors.
Long-Term Sustainability and Corporate Image
Adopting renewable energy systems at oil refineries not only improves operational efficiency and reduces costs, but also enhances the refinery’s long-term sustainability. Many oil refineries are facing increasing pressure from governments, environmental groups, and stakeholders to reduce their carbon footprint and adopt more sustainable business practices. By integrating renewable energy, refineries can demonstrate their commitment to environmental responsibility and sustainability.
This shift toward cleaner energy sources can significantly improve the refinery’s public image and reputation. Consumers and investors are increasingly prioritizing companies that adopt sustainable and environmentally friendly practices. Refineries that embrace renewable energy are likely to benefit from improved relationships with regulatory bodies, a stronger corporate reputation, and increased interest from socially conscious investors.
In regions with stringent environmental regulations, adopting renewable energy solutions can also help oil refineries comply with local laws and avoid potential fines, penalties, or restrictions associated with carbon emissions. Renewable energy adoption can be an important part of a refinery’s overall strategy to meet regulatory requirements and maintain operations in a compliant manner.
Economic Diversification and Community Impact
In regions where oil refineries are located, particularly in rural or economically dependent areas, investing in renewable energy can offer significant economic diversification and community benefits. By building small-scale renewable energy plants, refineries can support local economies through job creation, training, and the development of renewable energy industries.
The installation and maintenance of renewable energy infrastructure, such as solar panels, wind turbines, and biomass facilities, require a skilled workforce. This creates opportunities for local workers and provides new employment opportunities in industries that may not have been prevalent in the region before. Additionally, refineries may be able to source components and materials for renewable energy systems locally, further boosting the local economy.
Renewable energy projects also help reduce reliance on imported fuels, thereby increasing energy independence for both the refinery and the surrounding community. By harnessing local renewable resources, refineries can contribute to the region’s energy security while simultaneously supporting a shift toward cleaner energy.
Energy Storage Solutions for Reliability
One of the challenges of renewable energy, especially solar and wind, is the intermittent nature of power generation. Solar energy production is limited to daylight hours, while wind power fluctuates depending on weather conditions. To ensure a continuous and reliable power supply, oil refineries can integrate energy storage solutions, such as batteries, into their renewable energy systems.
Energy storage allows refineries to store excess energy generated during periods of high renewable energy production and release it during times of low production or high energy demand. This helps ensure that the refinery has a constant supply of energy, even when renewable sources are not actively generating electricity.
Modern energy storage technologies, such as lithium-ion batteries, are becoming increasingly affordable and efficient. By integrating these systems with renewable energy sources, oil refineries can increase the reliability and stability of their power supply while reducing the need for backup generators that rely on fossil fuels.
Regulatory and Policy Support
Governments worldwide are increasingly offering support for renewable energy adoption through incentives, tax credits, and favorable policies. Oil refineries can take advantage of these policies to reduce the initial capital costs of installing renewable energy systems. These incentives may include investment tax credits, accelerated depreciation, and direct subsidies that make renewable energy projects more financially attractive.
In addition to financial incentives, some regions offer renewable energy targets or mandates that require companies to integrate a certain percentage of renewable energy into their operations. Refineries that adopt renewable energy technologies early can meet these targets ahead of schedule and avoid future penalties or regulatory challenges.
In some cases, refineries may also be able to sell excess renewable energy back to the grid or enter into power purchase agreements (PPAs) with utilities. These programs allow refineries to generate additional revenue streams by feeding surplus energy into the grid during times of low demand, which can help offset the initial investment in renewable energy infrastructure.
Conclusion
Small-scale power plants for oil refineries provide a valuable opportunity for the industry to reduce energy costs, improve sustainability, and enhance energy security. By integrating renewable energy sources such as solar, wind, and biomass, refineries can generate clean, cost-effective energy while reducing their reliance on fossil fuels and minimizing environmental impact.
The financial benefits of small-scale renewable energy systems, coupled with the potential for job creation, energy independence, and regulatory compliance, make these systems an attractive investment for oil refineries. Through strategic adoption of renewable energy, oil refineries can improve their bottom line, meet evolving sustainability requirements, and position themselves as leaders in the transition to a more sustainable energy future.
As renewable energy technologies continue to evolve and become more affordable, small-scale power plants will play an increasingly important role in helping oil refineries meet their energy needs while supporting environmental and economic goals.
Continuing with Small-Scale Power Plants for Oil Refineries:
Flexibility and Scalability of Renewable Energy Systems
One of the key advantages of small-scale power plants is their flexibility and scalability, which is especially beneficial for oil refineries. Depending on the energy needs and available space, refineries can design renewable energy systems that are perfectly sized to meet their specific demands. This scalability allows oil refineries to start with a modest renewable energy installation and expand the capacity over time as energy needs grow or new technologies become available.
For example, if a refinery experiences growth in production or has increased energy demands, additional renewable energy capacity, such as more solar panels or wind turbines, can be installed. This allows refineries to scale their renewable energy generation in line with their production needs, avoiding costly overcapacity while optimizing their energy supply.
The modular nature of renewable energy systems also allows refineries to adapt to changing conditions. If a refinery experiences seasonal variations in energy demand, a combination of renewable sources (e.g., solar, wind, biomass) can provide a balanced power supply year-round, while storage systems can smooth out fluctuations in energy production. By combining multiple types of renewable energy technologies, oil refineries can create a more robust and adaptable energy mix that ensures reliability even in changing environmental conditions.
Reduced Transmission and Distribution Losses
Another benefit of small-scale renewable energy systems at oil refineries is the reduction in transmission and distribution (T&D) losses. In traditional grid systems, energy is transmitted over long distances from power plants to end-users, which results in energy losses due to resistance in the transmission lines and transformers. This can account for a significant portion of energy waste.
By generating energy on-site using renewable sources, oil refineries eliminate the need to draw power from the grid over long distances, thus reducing T&D losses. This direct supply of power can result in higher efficiency and lower energy costs for the refinery. Moreover, minimizing grid reliance can also help refineries avoid energy price fluctuations caused by transmission bottlenecks or regional grid instability.
Energy Independence and Security
Energy independence is a critical consideration for many industries, especially in sectors like oil refining, where energy is a key component of operations. Small-scale renewable energy systems provide oil refineries with the ability to produce their own energy, reducing their dependence on external energy sources. This increases energy security, as refineries are less vulnerable to fluctuations in energy prices or disruptions in fuel supply chains.
Renewable energy systems such as solar, wind, or biomass provide a more stable, predictable energy source than fossil fuels, which are subject to price volatility. By investing in renewable energy, oil refineries can lock in long-term energy savings and avoid the risks associated with reliance on fossil fuel markets. For example, the price of natural gas or coal can fluctuate significantly based on geopolitical events, supply-demand imbalances, and regulatory changes. With renewable energy, refineries can generate their own power from local, sustainable resources, insulating themselves from these market uncertainties.
Moreover, renewable energy can improve resilience in the event of grid outages or energy supply disruptions caused by natural disasters, geopolitical tensions, or economic shifts. With on-site generation, oil refineries can continue operations even during times when external energy supplies are compromised, ensuring continuity of production and minimizing downtime.
Hybrid Systems for Optimized Performance
Many oil refineries are opting for hybrid systems that combine renewable energy sources with traditional power generation methods, creating a more reliable and cost-effective energy solution. A hybrid energy system allows refineries to take advantage of renewable energy when it is available while using traditional power generation as a backup during periods of low renewable energy production. This combination maximizes the efficiency of renewable energy sources while ensuring a continuous and stable power supply.
For instance, a refinery might integrate a small-scale wind or solar installation with a natural gas backup generator. During the day, when solar power is abundant, the refinery could rely primarily on solar energy. At night or during cloudy weather, the refinery could switch to natural gas to cover energy demands. The hybrid system ensures that the refinery always has access to sufficient power without the need for large, expensive energy storage solutions.
Hybrid systems can also optimize the use of biomass energy. For example, excess biomass produced from refinery operations can be used in biomass power plants to supplement renewable energy generation. This creates a closed-loop system where the refinery utilizes its own waste material to produce energy, further enhancing sustainability and reducing waste disposal costs.
Sustainability and Carbon Credit Opportunities
As global pressure to reduce carbon emissions continues to grow, many oil refineries are looking for ways to meet sustainability targets and reduce their carbon footprint. The adoption of small-scale renewable energy plants offers a clear path toward achieving these goals.
Refineries can take advantage of carbon credit programs that reward businesses for reducing their greenhouse gas (GHG) emissions. By shifting a portion of their energy consumption to renewable sources, oil refineries can reduce their overall carbon emissions, which may qualify them for carbon credits or offset schemes. These programs allow companies to sell or trade carbon credits to other businesses that need to meet emission reduction targets, creating an additional revenue stream.
In regions with carbon pricing or cap-and-trade schemes, reducing GHG emissions through renewable energy adoption can also help refineries avoid penalties or fines associated with their emissions. In this context, renewable energy investments offer both environmental and financial benefits.
Long-Term Viability and Technological Advancements
The renewable energy sector continues to evolve with technological advancements that improve the efficiency, cost-effectiveness, and performance of small-scale power plants. As new technologies emerge, oil refineries that adopt renewable energy solutions early on are well-positioned to benefit from these innovations.
For example, advances in solar photovoltaic (PV) technology, energy storage solutions, and wind turbine design are making renewable energy systems more efficient and affordable. In particular, the development of high-capacity battery storage systems and next-generation PV panels could enable refineries to generate more power while reducing the space required for renewable energy installations.
Additionally, the growing trend of digitalization and automation in energy systems is enabling more efficient management of energy production and consumption. Artificial intelligence (AI), machine learning (ML), and advanced analytics are helping refineries optimize energy usage, predict energy demand patterns, and improve system performance. These advancements in digital energy management tools further enhance the long-term viability and efficiency of renewable energy systems in oil refineries.
Conclusion
The integration of small-scale renewable energy plants into oil refineries provides a comprehensive solution to address a variety of challenges, including high energy costs, environmental regulations, and energy security concerns. By leveraging solar, wind, and biomass energy sources, refineries can reduce their reliance on fossil fuels, cut energy costs, and minimize their carbon footprint.
With the growing availability of energy storage solutions, hybrid systems, and advancements in renewable energy technology, small-scale power plants offer a reliable and cost-effective alternative to traditional energy sources. The benefits of renewable energy adoption extend beyond financial savings, contributing to enhanced energy security, operational resilience, and compliance with sustainability regulations.
As oil refineries continue to face increasing pressure to adopt cleaner, more sustainable energy solutions, small-scale renewable energy systems will play an increasingly important role in shaping the future of the industry. By embracing these technologies, refineries can position themselves as leaders in the transition to a more sustainable, low-carbon energy landscape, while also securing long-term financial and environmental benefits.
Reduced Environmental Impact and Compliance with Emissions Regulations
Oil refineries face significant environmental scrutiny, especially regarding their carbon emissions and the broader ecological footprint of their operations. The transition to small-scale renewable energy plants presents an effective strategy for reducing greenhouse gas (GHG) emissions, meeting environmental regulations, and improving the refinery’s sustainability credentials. Renewable energy systems, such as solar, wind, and biomass, have a much lower environmental impact compared to fossil fuels, helping to mitigate the negative effects of refinery operations on local air quality and ecosystems.
In many regions, refineries are subject to stringent emissions regulations aimed at curbing pollution and addressing climate change. By incorporating renewable energy into their operations, oil refineries can decrease their overall carbon output, which is an essential part of meeting government-imposed emissions reduction targets. This transition to cleaner energy sources can also help refineries avoid potential fines and penalties associated with non-compliance.
Furthermore, integrating renewable energy into the refinery’s energy mix can help improve its overall environmental performance, enhancing the refinery’s reputation and ensuring compliance with local and international environmental standards. As governments around the world adopt more aggressive climate policies, refineries that are proactive in reducing their carbon footprint and adopting green technologies will likely be better positioned to maintain operations in a regulatory-compliant manner.
Energy Resilience and Backup Power
Renewable energy sources like wind and solar, while beneficial, can sometimes be intermittent due to weather fluctuations or time-of-day constraints. For an oil refinery, ensuring a continuous and reliable power supply is crucial to maintaining operations and preventing costly downtimes. One effective solution to this challenge is the integration of backup power systems, including energy storage and hybrid generation systems.
Energy storage technologies, such as advanced batteries, play a critical role in buffering intermittent renewable energy. Solar or wind power can be stored during peak generation times and discharged when energy demand is high or when renewable production is low. This ability to store and deploy renewable energy on demand enhances the resilience of refinery operations and reduces reliance on grid power or backup fossil fuel generators. With proper energy management systems in place, oil refineries can smooth out fluctuations in renewable energy availability, ensuring that energy needs are met efficiently at all times.
Backup power solutions are especially critical for refineries that operate 24/7, where energy interruptions can cause significant losses in production. By integrating renewable energy with backup power systems, refineries ensure not only lower costs and reduced environmental impact but also increased operational stability.
Integration with Existing Infrastructure
One of the advantages of small-scale renewable energy solutions is that they can be integrated into existing refinery infrastructure with minimal disruption. Refineries, which often have large energy demands, typically already have complex electrical systems and substantial space for the installation of renewable energy technologies. This existing infrastructure can serve as a foundation for implementing renewable energy systems without requiring extensive modifications or significant operational downtime.
For example, large roofs on refinery buildings are ideal for solar panel installations. Similarly, wind turbines can be deployed on unused land surrounding the refinery, while biomass power plants can be integrated into the refinery’s waste management system. Refineries that already produce waste heat or biomass as part of their refining processes can find innovative ways to repurpose these byproducts to generate additional energy.
Moreover, the integration of renewable energy solutions does not necessarily require an overhaul of existing power generation systems. Instead, refineries can adopt a phased approach, gradually adding renewable energy capacity as they reduce dependence on traditional energy sources. This flexibility allows refineries to transition toward sustainability at their own pace, balancing the need for green energy with ongoing operational requirements.
Economic Growth in the Renewable Energy Sector
Investing in small-scale renewable energy systems at oil refineries not only benefits the refinery but also has the potential to stimulate local economies. By purchasing renewable energy equipment and sourcing energy components from local or regional suppliers, refineries can support the growth of the renewable energy sector, generating jobs and fostering economic development.
The installation and maintenance of renewable energy systems require specialized labor, creating employment opportunities in sectors such as solar panel installation, wind turbine manufacturing, energy storage solutions, and energy management systems. Local economies can benefit from this influx of new jobs, which can range from skilled labor to engineering and technical positions.
In regions where refineries are major employers, adopting renewable energy can provide a long-term boost to the economy. Additionally, renewable energy projects often involve collaboration with academic institutions and research organizations, further driving innovation and growth in the local energy sector.
Long-Term Financial Savings and Competitive Advantage
The financial savings from integrating small-scale renewable energy systems into oil refineries can accumulate significantly over time, giving refineries a competitive edge in the energy market. By reducing energy costs and reliance on traditional fossil fuels, refineries can maintain profitability even in volatile energy markets. As energy prices continue to fluctuate, the ability to generate power on-site offers refineries a stable, predictable cost structure that shields them from energy price hikes.
Furthermore, refineries that adopt renewable energy solutions position themselves as forward-thinking companies in the global energy landscape. As investors increasingly prioritize sustainability and environmental impact, refineries that embrace renewable energy can attract more capital and improve their standing in the market. This trend is particularly notable among institutional investors who are shifting towards Environmental, Social, and Governance (ESG)-focused investments.
Small-scale renewable energy projects also reduce operational costs in the long run, making them an attractive proposition for refineries looking to improve profitability while staying competitive. The reduced energy expenditure, combined with the potential to sell excess energy to the grid or enter into power purchase agreements (PPAs), adds additional revenue streams that help offset the upfront costs of renewable energy infrastructure.
Policy and Regulatory Support
Governments around the world are increasingly offering incentives to businesses, including oil refineries, to adopt renewable energy technologies. These incentives often come in the form of tax credits, grants, and subsidies aimed at reducing the initial capital cost of installing renewable energy systems. Refineries can take advantage of these programs to accelerate the adoption of renewable energy and maximize the financial benefits of their investments.
In addition to direct financial incentives, governments may provide renewable energy credits, carbon credits, or other regulatory rewards for adopting sustainable energy solutions. This creates an additional financial incentive for oil refineries to integrate renewable energy into their operations. By taking advantage of these opportunities, refineries can lower the cost barrier to adopting renewable energy and enhance the financial viability of their projects.
Moreover, by aligning with global and regional energy policies that support decarbonization and clean energy, oil refineries can future-proof their operations. Many governments are introducing stricter emissions regulations and pushing for net-zero targets. Refineries that proactively incorporate renewable energy into their energy mix are better equipped to comply with these regulations, avoiding potential penalties and ensuring long-term operational continuity.
Conclusion
Small-scale renewable energy systems offer significant advantages to oil refineries, ranging from cost savings and energy independence to environmental sustainability and enhanced operational resilience. By integrating renewable energy technologies like solar, wind, and biomass, oil refineries can meet their energy needs in a cleaner, more cost-effective manner while reducing their reliance on fossil fuels.
These systems are not only financially beneficial, but they also improve refineries’ compliance with increasingly stringent emissions regulations, enhance energy security, and position the refineries as leaders in sustainability. As renewable energy technologies continue to evolve, the integration of small-scale power plants at oil refineries will play an essential role in transforming the energy landscape and contributing to global efforts to reduce carbon emissions.
Ultimately, small-scale power plants provide oil refineries with an opportunity to balance profitability with environmental responsibility, fostering long-term sustainability while improving their bottom line. Through strategic investments in renewable energy, oil refineries can build a more resilient, competitive, and eco-friendly future.
EMS Power Machines
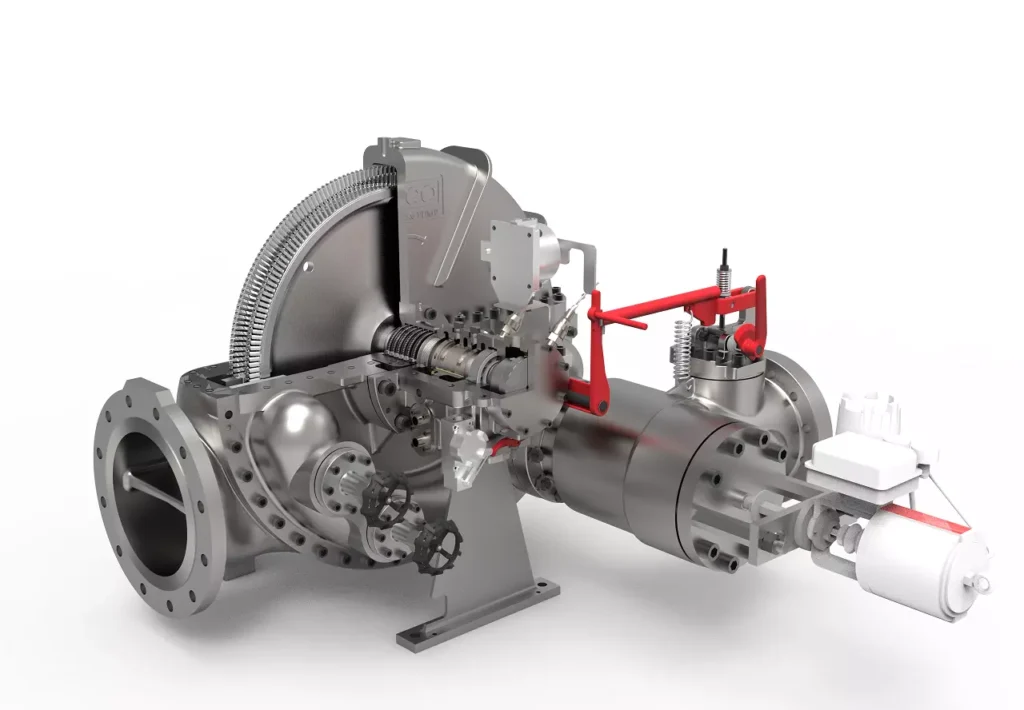
We design, manufacture and assembly Power Machines such as – diesel generators, electric motors, vibration motors, pumps, steam engines and steam turbines
EMS Power Machines is a global power engineering company, one of the five world leaders in the industry in terms of installed equipment. The companies included in the company have been operating in the energy market for more than 60 years.
EMS Power Machines manufactures steam turbines, gas turbines, hydroelectric turbines, generators, and other power equipment for thermal, nuclear, and hydroelectric power plants, as well as for various industries, transport, and marine energy.
EMS Power Machines is a major player in the global power industry, and its equipment is used in power plants all over the world. The company has a strong track record of innovation, and it is constantly developing new and improved technologies.
Here are some examples of Power Machines’ products and services:
- Steam turbines for thermal and nuclear power plants
- Gas turbines for combined cycle power plants and industrial applications
- Hydroelectric turbines for hydroelectric power plants
- Generators for all types of power plants
- Boilers for thermal power plants
- Condensers for thermal power plants
- Reheaters for thermal power plants
- Air preheaters for thermal power plants
- Feedwater pumps for thermal power plants
- Control systems for power plants
- Maintenance and repair services for power plants
EMS Power Machines is committed to providing its customers with high-quality products and services. The company has a strong reputation for reliability and innovation. Power Machines is a leading provider of power equipment and services, and it plays a vital role in the global power industry.
EMS Power Machines, which began in 1961 as a small factory of electric motors, has become a leading global supplier of electronic products for different segments. The search for excellence has resulted in the diversification of the business, adding to the electric motors products which provide from power generation to more efficient means of use.