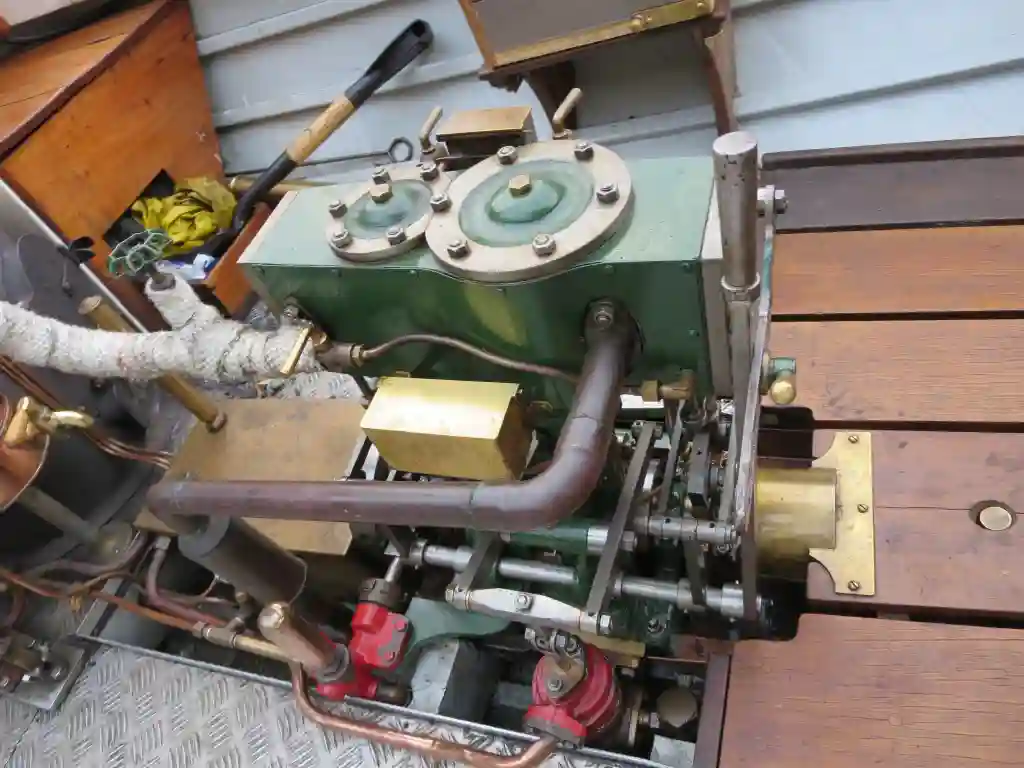
We manufacture Steam Engines for Marine applications. The modern steam engine, steam launch boiler, steam launch boat for sale, the marine steam engine for sale
Steam engines are devices that convert the energy stored in steam into mechanical work. They played a crucial role in the Industrial Revolution and have a rich history in powering various machines and vehicles. Here are some key points about steam engines:
- Basic Principle: Steam engines operate based on the principle of converting heat energy into mechanical work. This is accomplished by heating water to produce steam, which then expands and drives a piston or turbine.
- Invention and Early Development: The development of the steam engine is often credited to Thomas Savery and Thomas Newcomen in the early 18th century. However, it was James Watt’s improvements in the late 18th century that made steam engines more efficient and practical.
- James Watt’s Improvements: James Watt, a Scottish engineer, introduced several improvements to the steam engine, including a separate condenser and a rotary motion mechanism. These innovations significantly increased the engine’s efficiency and made it more suitable for a wide range of applications.
- Industrial Revolution: The steam engine played a crucial role in the Industrial Revolution, powering factories, mills, and transportation systems. It replaced traditional water and windmills and provided a more reliable and versatile source of power.
- Steam Locomotives: Steam engines were widely used in the transportation sector, particularly in locomotives. Steam locomotives were essential for the expansion of railways in the 19th century, enabling faster and more efficient transportation of goods and people.
- Marine Applications: Steam engines also revolutionized maritime transport. Steamships replaced sailing vessels, offering more control over navigation and allowing ships to travel independent of wind conditions.
- Decline and Successors: While steam engines were dominant for much of the 19th and early 20th centuries, they eventually faced competition from internal combustion engines and electric motors. However, steam power is still used in some niche applications today, and historical steam engines are preserved in museums.
- Types of Steam Engines:
- Reciprocating Steam Engines: These engines use pistons to convert the linear motion of a piston into a rotating motion.
- Steam Turbines: These engines use steam to drive a turbine, which generates rotary motion. They are often more efficient than reciprocating engines for large-scale power generation.
- Modern Applications: While steam engines are no longer the primary source of power in most industries, they are still used in certain applications, such as power plants, where they generate electricity.
Understanding the principles and history of steam engines provides valuable insights into the development of technology and its impact on society during the Industrial Revolution.
Steam Engines
Basic Principle
The basic principle behind a steam engine involves the conversion of heat energy into mechanical work through the expansion of steam. Here’s a more detailed explanation of the basic principles:
- Boiling Water to Produce Steam: The process begins with the heating of water to produce steam. This is typically done in a boiler, where water is heated by burning fuel such as coal, wood, or oil. The heat energy causes the water to boil and turn into steam.
- Expansion of Steam: The steam produced is then directed into a cylinder that contains a piston. As the steam enters the cylinder, it expands, creating pressure against the piston.
- Piston Movement: The pressure from the expanding steam forces the piston to move. The movement of the piston can be linear (back and forth) in reciprocating engines or rotary in the case of a turbine.
- Mechanical Work: The movement of the piston or turbine is connected to a mechanism that performs mechanical work. In reciprocating engines, the linear motion of the piston can be used to turn a crankshaft, which can then be connected to various machines or devices to perform tasks. In turbines, the rotary motion directly drives a generator or other machinery.
- Condensation and Return: After performing work, the steam needs to be condensed back into water to complete the cycle. This is often done using a separate condenser. The condensed water is then returned to the boiler to be heated again, restarting the cycle.
This process is known as the Rankine cycle, and it is the fundamental operating principle of many steam engines. The efficiency of a steam engine depends on factors such as the pressure and temperature of the steam, the design of the engine, and the heat exchange processes involved.
The basic principles of steam engines were first put into practical use during the 18th century, and they played a pivotal role in the Industrial Revolution by providing a reliable and efficient source of power for various applications, ranging from factories to transportation.
Boiling Water to Produce Steam
Boiling water to produce steam is a fundamental process in the operation of steam engines, steam turbines, and various industrial applications. The transformation of water into steam involves the application of heat to raise the temperature of the water to its boiling point and beyond. Here are the key steps in boiling water to produce steam:
- Heating the Water:
- The process begins by heating water. This can be achieved using various heat sources, including but not limited to:
- Combustion: Burning fossil fuels such as coal, oil, or natural gas in a boiler or furnace.
- Nuclear Reaction: Utilizing the heat generated from nuclear fission reactions in nuclear power plants.
- Renewable Sources: Using solar energy or geothermal heat to raise the temperature of the water.
- The process begins by heating water. This can be achieved using various heat sources, including but not limited to:
- Boiling Point:
- As the water absorbs heat energy, its temperature rises. At standard atmospheric pressure, water boils at 100 degrees Celsius (212 degrees Fahrenheit). The transition from liquid to vapor occurs when the water reaches its boiling point.
- Formation of Steam:
- Once the water reaches its boiling point, additional heat energy applied to the water is absorbed as latent heat. This latent heat causes the water molecules to undergo a phase transition from liquid to vapor, forming steam.
- Pressure Considerations:
- The boiling point of water is influenced by pressure. In situations where pressure is elevated, such as in steam boilers, the boiling point is increased. This is why water can boil at temperatures higher than 100 degrees Celsius in steam boilers operating under pressure.
- Control of Steam Quality:
- The quality of steam produced is crucial for various applications. In some cases, steam must be superheated, meaning it is heated beyond its boiling point. Superheated steam is drier and contains more heat energy, making it suitable for specific industrial processes or power generation applications.
- Steam Generation in Boilers:
- In industrial settings and power plants, steam is often generated in boilers. Boilers are vessels designed to contain and heat water to produce steam. The steam produced in boilers can then be used for various applications, including electricity generation in power plants, industrial processes, and heating systems.
- Steam Engines and Turbines:
- In steam engines and turbines, the steam produced by boiling water is directed onto blades or vanes, causing them to move. The kinetic energy of the moving steam is then converted into mechanical energy, which can be used to perform work or generate electricity.
- Condensation and Recycling:
- After performing its work, steam can be condensed back into water, and the process can be repeated. In power plants, this condensed water is often returned to the boiler for reheating, creating a closed-loop cycle.
Boiling water to produce steam is a foundational process in steam-based technologies, providing a versatile and efficient means of converting heat energy into mechanical work or other useful forms of energy. The principles of this process have been integral to the development of steam engines, turbines, and various industrial applications for centuries.
Expansion of Steam
The expansion of steam is a crucial phase in the operation of steam engines and turbines. It is a thermodynamic process where high-pressure steam is allowed to expand, doing work as it moves a piston in a reciprocating engine or impinges on the blades of a turbine in a rotary engine. Understanding the expansion of steam is essential for optimizing the efficiency of these systems. Here’s a general overview of the expansion process:
- Admission of High-Pressure Steam:
- The expansion process begins with the admission of high-pressure steam into a cylinder or turbine. This steam is typically generated in a boiler through the heating of water.
- Expansion in a Cylinder (Reciprocating Engine):
- In a reciprocating steam engine, the high-pressure steam is directed into a cylinder where it pushes against a piston. As the steam expands, it performs work by moving the piston. The linear motion of the piston is then converted into rotary motion to drive machinery.
- Expansion in a Turbine (Rotary Engine):
- In a steam turbine, the high-pressure steam is directed onto the blades of the turbine rotor. The steam’s kinetic energy causes the rotor to rotate. The expansion of steam in the turbine is continuous, with multiple stages of blades designed to extract as much energy as possible from the steam.
- Isothermal Expansion (Idealized):
- In an idealized process, known as isothermal expansion, the steam expands while maintaining a constant temperature. However, in actual steam engines and turbines, the expansion is typically adiabatic, meaning it occurs without the transfer of heat to or from the surroundings.
- Adiabatic Expansion:
- During adiabatic expansion, the expanding steam does work on the moving parts of the engine or turbine, and its internal energy decreases. As a result, the steam’s temperature and pressure decrease.
- Efficiency Considerations:
- The efficiency of a steam engine or turbine is influenced by the expansion ratio, which is the ratio of the initial steam pressure to the final pressure after expansion. Higher expansion ratios generally lead to greater efficiency.
- Reheat (In Some Turbines):
- In some steam turbines, particularly in power plants, a reheat process may be employed. After partial expansion, the steam is returned to a high-pressure section for reheating before undergoing further expansion. Reheat can enhance efficiency by preventing the steam from becoming too wet during expansion.
- Exhaust:
- The steam, having expanded and performed work, exits the engine or turbine at a lower pressure and temperature. In a reciprocating engine, the exhaust steam may be condensed back into water for reuse. In a turbine, the exhaust steam may be condensed in a separate condenser or expelled directly, depending on the application.
Understanding and optimizing the expansion process is essential for maximizing the efficiency and performance of steam-based systems. Engineers carefully design steam engines and turbines to extract the maximum amount of work from the expanding steam while minimizing losses.
Piston Movement
The movement of a piston is a fundamental aspect of reciprocating engines, including steam engines and internal combustion engines. The piston is a cylindrical component that moves back and forth within a cylinder, and its motion is critical for the conversion of linear motion into rotary motion or to perform other mechanical work. Here are the key aspects of piston movement in reciprocating engines:
- Reciprocating Motion:
- The term “reciprocating” refers to the back-and-forth motion of the piston within the cylinder. This reciprocating motion is driven by the expansion and contraction of gases (such as steam or combustion gases) within the cylinder.
- Intake Stroke:
- In the four-stroke cycle of internal combustion engines, the piston’s movement begins with the intake stroke. During this stroke, the piston moves downward, creating a vacuum in the cylinder. This vacuum allows the intake valve to open, drawing in air (and fuel in the case of internal combustion engines) into the cylinder.
- Compression Stroke:
- Following the intake stroke, the piston begins the compression stroke. The piston moves upward, compressing the air (and fuel, if present) in the cylinder. This compression increases the temperature and pressure of the mixture, preparing it for ignition in internal combustion engines.
- Power (Combustion) Stroke:
- The power stroke is where the combustion of fuel (in internal combustion engines) or the expansion of steam (in steam engines) occurs. This phase generates a high-pressure force that drives the piston downward, producing mechanical work. In internal combustion engines, the combustion of fuel and air occurs after the compression stroke, leading to the expansion of high-pressure gases.
- Exhaust Stroke:
- After the power stroke, the piston begins the exhaust stroke. The piston moves upward, pushing the spent gases out of the cylinder through the open exhaust valve. This prepares the cylinder for the next intake stroke.
- Two-Stroke Cycle:
- In some engines, particularly smaller internal combustion engines, a two-stroke cycle is used. In a two-stroke engine, the intake and exhaust strokes occur in the same piston movement (one revolution of the crankshaft). This design simplifies the engine but may be less fuel-efficient than a four-stroke engine.
- Connecting Rod and Crankshaft:
- The reciprocating motion of the piston is converted into rotary motion by the connecting rod and crankshaft. The connecting rod connects the piston to the crankshaft, and as the piston moves back and forth, it causes the crankshaft to rotate.
- Linear and Rotary Motion Conversion:
- The linear motion of the piston is transformed into rotary motion by the crankshaft. The crankshaft is equipped with crank throws that are offset from the crankshaft’s centerline. As the connecting rod pivots around the crank throw, it converts the piston’s linear motion into the rotary motion of the crankshaft.
- Balancing:
- In multi-cylinder engines, the arrangement of cylinders and the firing order are designed to balance the forces on the crankshaft and minimize vibrations. Balancing is essential for smooth engine operation and reduced wear on components.
The controlled movement of the piston through the intake, compression, power, and exhaust strokes is fundamental to the operation of reciprocating engines. This process enables the conversion of thermal energy from combustion or steam expansion into useful mechanical work.
Mechanical Work
The mechanical work done by a steam engine involves the conversion of energy from the expansion of steam into useful work. This process is fundamental to the operation of steam engines, which can be either reciprocating engines or turbines. Here’s a general overview of how mechanical work is done by a steam engine:
- Generation of Steam:
- The process begins with the generation of steam. This typically involves heating water in a boiler until it reaches its boiling point, and the water transforms into steam.
- Admission of Steam to the Cylinder or Turbine:
- In a reciprocating steam engine, the steam is admitted to a cylinder where it pushes against a piston, causing it to move. In a steam turbine, the steam is directed onto the blades of the turbine rotor, imparting kinetic energy to the rotor.
- Expansion of Steam:
- As the steam expands, it does work by pushing against the piston in a reciprocating engine or by moving the blades of a turbine in a rotary engine. The expansion of steam is crucial for extracting the maximum amount of energy from the steam.
- Reciprocating Engine:
- In a reciprocating engine, the expansion of steam in the cylinder does work by moving the piston. The linear motion of the piston is then converted into rotary motion through a connecting rod and crankshaft. This rotary motion is the mechanical work output of the engine and can be used to drive machinery or generate electricity.
- Turbine:
- In a steam turbine, the expansion of steam causes the rotor blades to rotate. The kinetic energy of the rotating blades is the mechanical work output of the turbine. The rotor is connected to a shaft, and the rotary motion of the shaft can be used to drive electrical generators, pumps, or other machinery.
- Control of Steam Flow:
- The control of steam flow is essential for regulating the speed and power output of the steam engine. This is typically achieved through the use of valves or nozzles that control the admission and exhaust of steam.
- Exhaust:
- After performing work, the exhaust steam is typically released from the engine or turbine. In some cases, the exhaust steam is condensed back into water and returned to the boiler for reuse in a closed-loop cycle.
- Efficiency Considerations:
- The efficiency of a steam engine is influenced by factors such as the expansion ratio (the ratio of initial pressure to final pressure during expansion), the design of the engine, and the heat losses in the system. Engineers aim to optimize these factors to achieve higher efficiency and better performance.
In summary, the mechanical work done by a steam engine involves the conversion of thermal energy from the expansion of steam into useful rotary or linear motion. This work output is harnessed to drive machinery, generate electricity, or perform other tasks in various industrial and power generation applications.
Condensation and Return
Condensation and return play a crucial role in the operation of steam-based systems, especially in the context of steam engines and power plants. These processes are part of a closed-loop cycle that allows for the efficient use of water and the recycling of steam. Here’s how condensation and return typically work:
- Condensation:
- After steam has performed its work in a steam engine or turbine, it needs to be condensed back into water to complete the cycle. Condensation is the process of transforming steam into liquid water. This is typically achieved by exposing the steam to a cooling medium, such as cold water or air.
- Condenser:
- In many steam-based systems, a separate component called a condenser is employed for the condensation process. The condenser provides a surface or system where steam can release its latent heat and undergo the phase change from a vapor to a liquid. This releases a significant amount of heat energy.
- Cooling Medium:
- The condenser is in contact with a cooling medium, which absorbs the heat from the steam. Common cooling mediums include circulating water, air, or a combination of both. The choice of cooling medium depends on the specific requirements and design of the system.
- Heat Exchange:
- Heat exchange occurs between the steam and the cooling medium in the condenser. As the steam loses heat, it undergoes condensation, and the resulting liquid water is then collected.
- Return (Recovery):
- The liquid water produced through condensation is returned to the boiler to be reheated and transformed back into steam. This return process is crucial for maintaining a closed-loop system, preventing the continuous consumption of water and allowing for the efficient use of resources.
- Closed-Loop Cycle:
- The combination of condensation and return creates a closed-loop cycle known as a Rankine cycle in the context of steam power plants. This cycle involves the following stages: generation of steam in the boiler, expansion of steam to perform work in the engine or turbine, condensation of steam in the condenser, and return of the condensed water to the boiler for reheating.
- Efficiency and Sustainability:
- The condensation and return process enhances the overall efficiency of steam-based systems. By recovering the water used in the system, these processes contribute to sustainable and resource-efficient operation.
- Application in Power Plants:
- In power plants, particularly those using steam turbines, the condensation and return process is a crucial part of thermal power generation. The condenser is often a large component connected to the turbine, and the condensed water is returned to the boiler through a series of pumps for reheating.
- Use of Cooling Towers:
- In some systems, especially those with limited access to natural bodies of water, cooling towers may be used to dissipate the heat absorbed by the cooling medium. The cooling tower releases heat to the atmosphere, and the cooled water can then be returned to the condenser.
- Water Treatment:
- Water used in the steam cycle is often treated to remove impurities that could adversely affect the performance and longevity of the system. Water treatment helps prevent scaling, corrosion, and other issues that can impact the efficiency of the condensation and return process.
Condensation and return are integral components of steam-based systems, contributing to their efficiency, sustainability, and the conservation of water resources. These processes are key elements in the broader field of thermodynamics and power generation.
Invention and Early Development
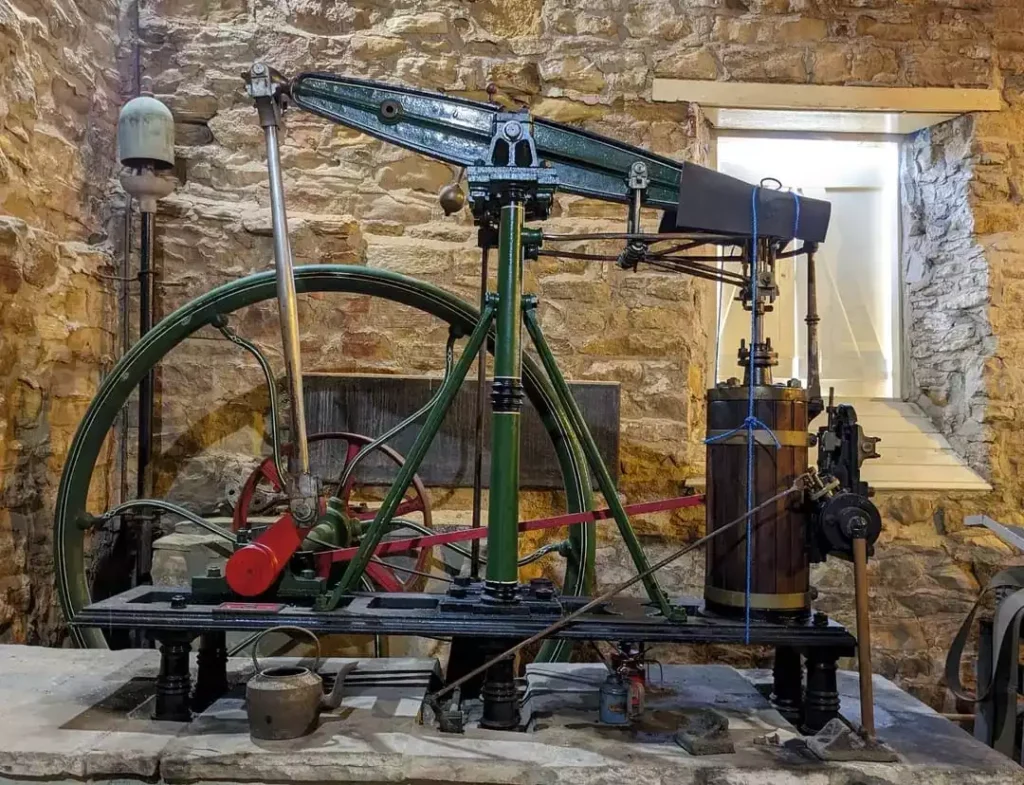
The invention and early development of the steam engine can be traced back to the 17th and 18th centuries, with several key figures contributing to its evolution. Here’s a brief overview of the major milestones:
- Early Steam Devices:
- Thomas Savery (1698): Thomas Savery, an English engineer, developed the first practical steam-powered device known as the “Savery Pump” or “Miner’s Friend.” It was designed to pump water out of mines by using steam to create a partial vacuum that drew water into the pump.
- Newcomen Engine:
- Thomas Newcomen (1712): Building on Savery’s work, Thomas Newcomen, an English blacksmith, developed the atmospheric steam engine around 1712. The Newcomen engine used a piston and cylinder arrangement. Steam was introduced into a cylinder, and then water was sprayed into the cylinder, causing the steam to condense and create a vacuum. Atmospheric pressure then pushed the piston down, performing mechanical work.
- James Watt’s Improvements:
- James Watt (1769): James Watt, a Scottish engineer, made significant improvements to the steam engine that transformed it into a more efficient and practical source of power. Watt introduced a separate condenser, which allowed the cylinder and piston to remain hot, increasing efficiency. He also developed a rotary motion mechanism, making the engine more versatile. Watt’s innovations, patented in 1769, marked a turning point in the history of steam engines.
- Widespread Adoption:
- Watt’s improved steam engine found widespread adoption in various industries, particularly in textile mills and factories. It played a crucial role in powering machinery during the early stages of the Industrial Revolution.
- Transportation Applications:
- As the technology advanced, steam engines were adapted for transportation. The development of steam locomotives and steamships in the early 19th century revolutionized land and sea transportation.
- George Stephenson and the Rocket (1829):
- George Stephenson, an English engineer, designed the “Rocket,” a steam locomotive that became famous for its speed and efficiency. The Rocket was a key development in the expansion of railways.
- Continued Innovation:
- Throughout the 19th century, engineers continued to innovate and refine steam engine technology. Compound engines, which used steam expansively in multiple stages, further improved efficiency.
The early development of the steam engine played a pivotal role in the Industrial Revolution by providing a reliable and powerful source of energy for mechanized production and transportation. The innovations of figures like Savery, Newcomen, and Watt laid the foundation for the technological advancements that followed.
James Watt’s Improvements
James Watt, a Scottish engineer, made several critical improvements to the design of the steam engine in the late 18th century. His innovations were instrumental in making the steam engine more efficient and practical. Here are the key improvements made by James Watt:
- Separate Condenser (1765): One of Watt’s most significant innovations was the introduction of a separate condenser in 1765. In earlier steam engines, the cooling and condensation of steam occurred within the same cylinder where the piston performed its work. Watt’s separate condenser allowed the cylinder and piston to remain hot, while the steam condensed in a separate chamber. This greatly improved the efficiency of the engine, as the cylinder no longer needed to be cooled and reheated during each cycle.
- Double-Acting Engine (1782): Watt developed the double-acting engine, which allowed steam to act on both sides of the piston. In earlier engines, steam was applied only on one side of the piston, and the return stroke was often powered by a counterweight. With the double-acting engine, steam acted on both sides of the piston, resulting in smoother and more continuous rotary motion.
- Rotary Motion (1788): Watt adapted the reciprocating motion of the piston to produce rotary motion. He achieved this by connecting the piston to a crankshaft through a linkage. This conversion to rotary motion made the steam engine more versatile and suitable for a broader range of applications, as rotary motion is easier to transmit and apply to various machinery.
- Governor (1788): Watt introduced a centrifugal governor to regulate the speed of the engine. The governor adjusted the amount of steam entering the cylinder based on the engine’s speed, maintaining a more consistent speed of rotation. This was crucial for the operation of machinery that required a constant speed.
- Parallel Motion (1784): Watt developed a parallel motion linkage to guide the piston rod’s vertical motion into a straight, horizontal motion. This mechanism reduced wear and tear on the engine parts, contributing to its reliability.
- Sun-and-Planet Gear (1781): In some of Watt’s later engines, he employed a sun-and-planet gear system to convert the reciprocating motion of the piston into rotary motion for the output shaft. This was another step toward creating smoother and more efficient power transmission.
These improvements collectively made Watt’s steam engine much more efficient, reliable, and adaptable to a wide range of industrial applications. Watt’s innovations played a crucial role in the Industrial Revolution, providing a powerful and efficient source of mechanical power for factories, mills, and transportation.
Industrial Revolution
The Industrial Revolution was a period of profound economic, technological, and social change that began in the late 18th century and continued into the 19th century. It marked the transition from agrarian and craft-based economies to industrialized and mechanized ones. The Industrial Revolution had far-reaching effects on almost every aspect of society, introducing new methods of production, transportation, and communication. Here are key aspects of the Industrial Revolution:
- Origins:
- The Industrial Revolution originated in Great Britain in the late 18th century. It was characterized by the shift from manual labor and traditional handicrafts to mechanized production facilitated by technological innovations.
- Technological Innovations:
- Steam Engine: The invention and improvement of the steam engine, particularly by James Watt, revolutionized power sources. Steam engines were used to power factories, mines, and later, transportation systems such as steamships and locomotives.
- Textile Machinery: Innovations in textile machinery, like the spinning jenny and power loom, transformed the textile industry, increasing production efficiency and output.
- Iron and Steel Production: Advances in metallurgy and the development of new methods for producing iron and steel contributed to the construction of machinery, railways, and infrastructure.
- Mechanized Agriculture: The introduction of new agricultural technologies, such as the seed drill and the mechanization of farming, increased agricultural productivity.
- Factory System:
- The shift from small-scale, decentralized cottage industries to large-scale factories marked a significant change in production methods. Factories brought together machinery, labor, and raw materials under one roof, leading to increased production and efficiency.
- Transportation Revolution:
- The development of steam-powered locomotives and steamships revolutionized transportation. Railways and canals facilitated the movement of goods and people over long distances, connecting distant regions and expanding markets.
- Urbanization:
- The growth of industry and the rise of factories led to significant urbanization, as people moved from rural areas to cities in search of employment. This resulted in the rapid expansion of urban centers.
- Impact on Labor:
- The Industrial Revolution had profound effects on labor, leading to changes in working conditions, hours, and wages. Factory work was often characterized by long hours, low pay, and sometimes hazardous conditions.
- Economic Changes:
- The Industrial Revolution had a transformative impact on the global economy, leading to increased production, economic growth, and the accumulation of capital. It laid the groundwork for the development of capitalism as the dominant economic system.
- Social and Cultural Changes:
- The Industrial Revolution brought about changes in social structures and cultural norms. The rise of the middle class, new social hierarchies, and shifts in family dynamics were among the social changes during this period.
- Global Spread:
- While the Industrial Revolution began in Great Britain, its effects spread to other parts of Europe, North America, and eventually, the rest of the world, influencing patterns of development and economic systems globally.
The Industrial Revolution is considered a watershed moment in history, transforming societies and laying the foundation for the modern industrialized world. While it brought about unprecedented economic growth and technological progress, it also raised social and economic challenges, including issues related to labor rights, living conditions, and social inequality.
Steam Locomotives
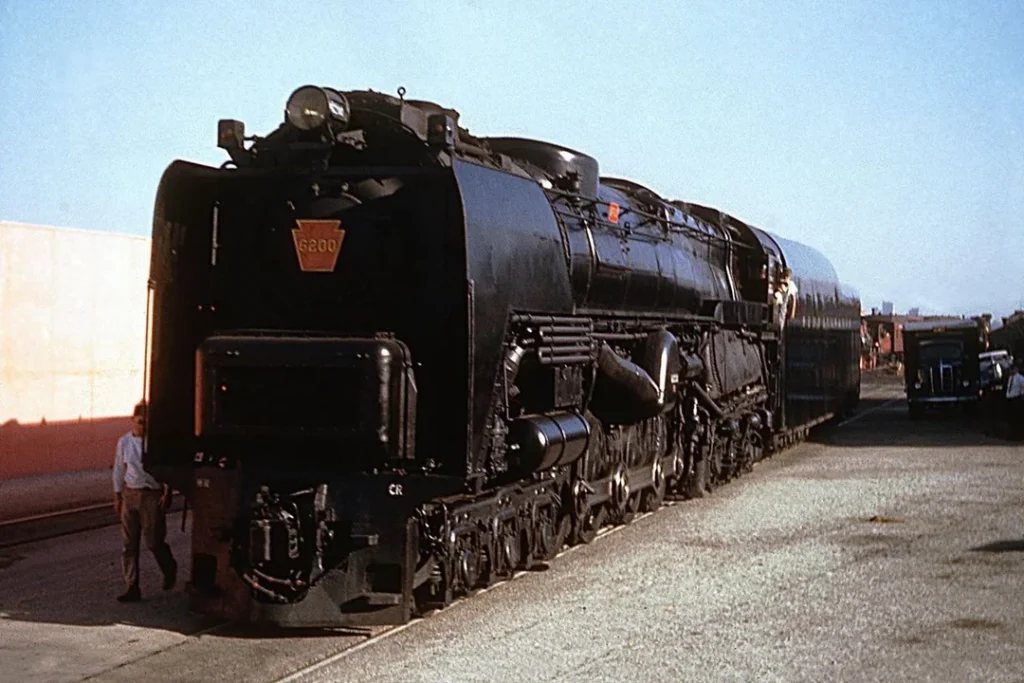
Steam locomotives were a key innovation during the early years of the Industrial Revolution and played a crucial role in transforming transportation. These powerful machines were the primary means of railway transportation for much of the 19th and early 20th centuries. Here are some key aspects of steam locomotives:
- Invention and Development:
- The development of steam locomotives was closely tied to the expansion of railways. The first full-scale working railway steam locomotive was built by George Stephenson, an English engineer, and his son Robert Stephenson. It was called “The Rocket” and was completed in 1829.
- Components of a Steam Locomotive:
- Boiler: The boiler is a crucial component where water is heated to produce steam. The steam is generated by burning coal or other fuels.
- Firebox: The firebox is located within the boiler and is where the fuel is burned, producing the heat necessary to generate steam.
- Cylinders and Pistons: Steam is directed into cylinders, where it expands and pushes pistons back and forth. The reciprocating motion of the pistons is then converted into rotary motion to drive the locomotive’s wheels.
- Drive Mechanism: The motion from the pistons is transmitted to the locomotive’s wheels through a drive mechanism, often a system of connecting rods and crankshafts.
- Smokestack (Chimney): The smokestack releases the exhaust gases from the burned fuel and steam, often creating the characteristic plume of smoke associated with steam locomotives.
- Railway Expansion:
- Steam locomotives played a pivotal role in the expansion of railways, enabling faster and more efficient transportation of goods and passengers. Railways became a critical part of industrial and economic development.
- Railway Speed Records:
- Steam locomotives set several speed records during their heyday. Notably, George Stephenson’s “Rocket” achieved a top speed of about 29 miles per hour during its demonstration in 1829.
- Varieties of Steam Locomotives:
- Different types of steam locomotives were developed to suit various needs. This included passenger locomotives designed for speed and comfort, freight locomotives for hauling heavy loads, and switcher locomotives for maneuvering within train yards.
- Golden Age of Steam:
- The 19th century is often referred to as the “Golden Age of Steam.” Steam locomotives became iconic symbols of progress and played a vital role in connecting distant regions, facilitating trade, and contributing to the growth of economies.
- Decline and Successors:
- While steam locomotives dominated railway transportation for many decades, they eventually faced competition from diesel and electric locomotives. By the mid-20th century, steam locomotives had largely been replaced by more efficient and cleaner technologies.
- Preservation:
- Despite their decline in regular use, many steam locomotives have been preserved and restored. Heritage railways and museums around the world showcase these historic machines, allowing people to experience the sights and sounds of steam-era rail travel.
The legacy of steam locomotives persists in cultural and historical contexts, and these powerful machines played a crucial role in shaping the modern transportation landscape.
Marine Applications with Steam Engines
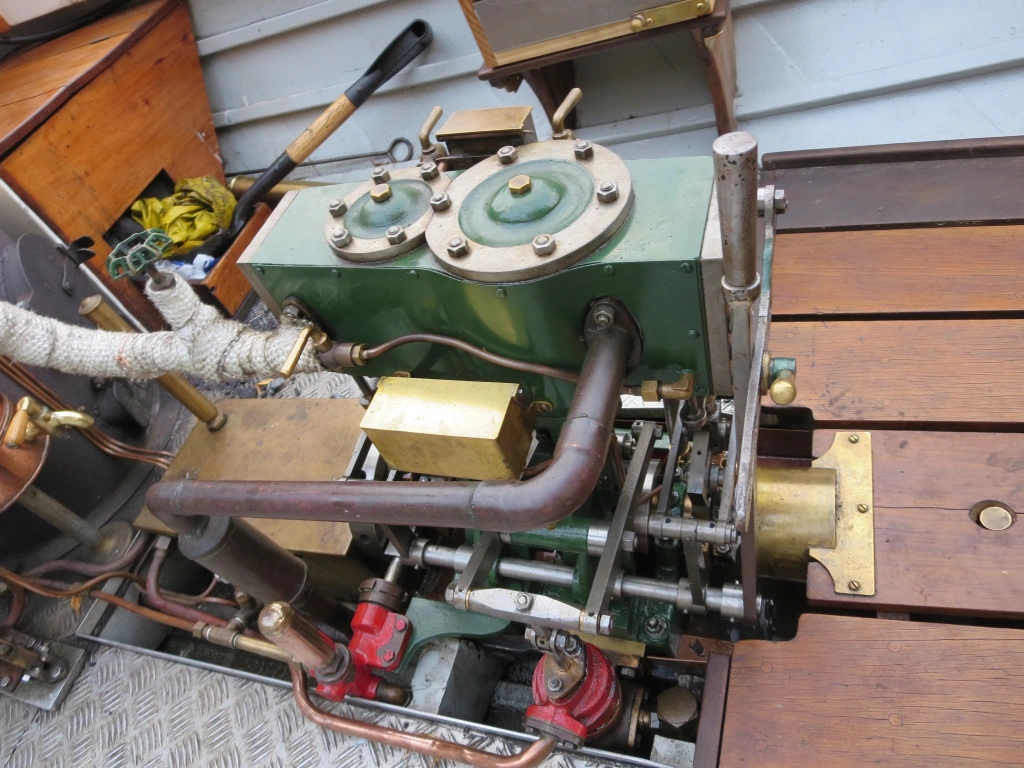
Steam engines had a significant impact on marine transportation during the 19th and early 20th centuries. They powered steamships, which replaced traditional sailing vessels and played a crucial role in maritime trade, exploration, and naval activities. Here are some key aspects of marine applications with steam engines:
- Steamships:
- Steamships were vessels powered by steam engines. They used steam to drive paddlewheels or propellers, providing a reliable and efficient means of propulsion. Steamships gradually replaced sailing ships, especially in the 19th century, as they could navigate independently of wind conditions.
- Early Steamships:
- The first practical steamship was the “Charlotte Dundas,” built by William Symington in 1802. However, it was the development of the more successful paddle-steamer, like Robert Fulton’s “Clermont” in 1807, that marked the beginning of steam-powered commercial shipping.
- Ocean Liners:
- Steam power transformed ocean travel, making it faster, more reliable, and less dependent on wind patterns. Steam-powered ocean liners became a popular mode of transportation for passengers and cargo across long distances.
- Improved Navigation and Efficiency:
- Steam power allowed ships to travel more efficiently and navigate with greater precision. This was particularly important for commercial and military vessels, reducing reliance on unpredictable wind patterns.
- Expansion of Trade and Exploration:
- Steamships played a crucial role in expanding global trade and facilitating exploration. They enabled more frequent and reliable connections between continents, opening up new markets and opportunities for commerce.
- Naval Applications:
- Steam power revolutionized naval warfare. Steam-powered warships, known as steamships of the line, became key components of naval fleets. They provided greater maneuverability and endurance, changing the dynamics of naval battles.
- Ironclads:
- The transition from wooden sailing ships to steam-powered ironclads marked a significant development in naval technology. Ironclads were warships with iron armor, powered by steam engines, and armed with heavy guns. They played a pivotal role in 19th-century naval warfare.
- Tugboats and Harbor Craft:
- Steam engines were used in smaller vessels, such as tugboats, to assist in the maneuvering of larger ships within harbors. This improved the efficiency of port operations and the docking of larger vessels.
- Efficiency Improvements:
- Advances in steam engine technology, such as compound engines and improved boilers, increased the efficiency and range of steam-powered vessels. This made steamships more economical and practical for long-distance travel.
- Transition to Diesel and Other Propulsion:
- While steam engines were dominant in marine applications for many years, they eventually faced competition from internal combustion engines, especially diesel engines. Diesel engines offered greater fuel efficiency and required less maintenance, leading to the gradual phasing out of steam-powered ships in the mid-20th century.
Despite the decline of steam-powered marine vessels in commercial use, steamships remain an integral part of maritime history, and some historic steamships are preserved as museum exhibits or still operate for tourism and educational purposes.
Decline and Successors
The decline of steam engines, particularly in industrial and transportation applications, was a gradual process that spanned several decades and was influenced by technological advancements and changing economic and environmental considerations. Here are some factors contributing to the decline of steam engines and their successors:
- Internal Combustion Engines:
- The development and widespread adoption of internal combustion engines, particularly diesel engines, played a significant role in the decline of steam engines. Internal combustion engines offered advantages such as higher fuel efficiency, faster start-up times, and reduced maintenance requirements.
- Dieselization:
- Diesel engines gradually replaced steam engines in various applications, including transportation (trains, ships, and trucks) and industrial settings. Diesel locomotives and ships became more popular due to their higher power-to-weight ratios and improved efficiency.
- Electricity:
- In many industrial applications, electric motors became a preferred alternative to steam engines. Electric motors provided a cleaner and more controllable source of power, and electricity could be generated centrally and transmitted over wires.
- Automobiles and Trucks:
- The widespread adoption of automobiles and trucks for personal and freight transportation contributed to the decline of steam-powered vehicles. Internal combustion engines became the standard for these modes of transportation due to their convenience and versatility.
- Advances in Turbine Technology:
- Steam turbines, a more advanced form of steam engine, continued to be used in certain applications, especially in power generation. However, even steam turbines faced competition from more efficient gas turbines in certain contexts, such as aircraft propulsion and power plants.
- Economic Considerations:
- The economic advantages of newer technologies, including lower operating costs and increased efficiency, played a crucial role in the decline of steam engines. As industries sought to improve productivity and reduce expenses, they often turned to more modern and efficient power sources.
- Environmental Concerns:
- The environmental impact of burning coal or other fossil fuels to generate steam became a concern. As environmental regulations and awareness increased, industries sought cleaner and more sustainable alternatives, contributing to the decline of steam power.
- Modernization and Automation:
- The trend towards modernization and automation in industries favored the adoption of more advanced and automated technologies. Steam engines, with their manual operation and maintenance requirements, became less attractive in comparison to newer, automated systems.
- Preservation and Niche Applications:
- Despite the decline of steam engines in mainstream applications, there has been an effort to preserve and celebrate these historic machines. Some steam locomotives and engines are still operational and used in tourist railways, museums, and special events.
- Cultural and Historical Significance:
- Steam engines continue to hold cultural and historical significance, and efforts are made to preserve them as a part of industrial heritage. Steam-powered vehicles and engines are often featured in museums and heritage events to showcase the technological advancements of the past.
While steam engines are no longer the primary source of power in most industries, their impact on the Industrial Revolution and subsequent technological developments is undeniable. The successors to steam engines, such as internal combustion engines and electric motors, have played a crucial role in shaping the modern world.
Reciprocating Steam Engines
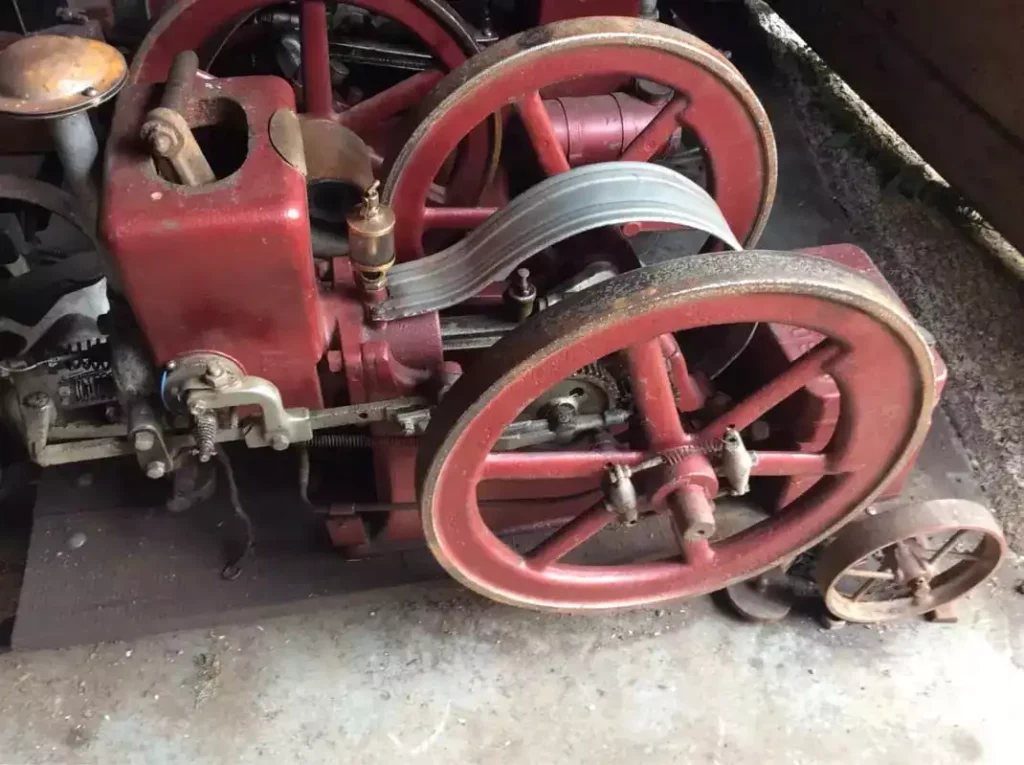
Reciprocating steam engines are a type of steam engine that converts the linear motion of a piston into rotary motion, which can be used to perform mechanical work. These engines played a crucial role in the Industrial Revolution and were widely used in various applications, including factories, mills, and early forms of transportation. Here are some key features and aspects of reciprocating steam engines:
- Basic Operation:
- The operation of reciprocating steam engines is based on the expansion of steam within a cylinder. Steam is admitted into one side of a cylinder, where it pushes a piston, causing it to move in one direction. As the steam expands and loses pressure, it is then exhausted from the cylinder, and the piston returns to its original position. The reciprocating motion of the piston is converted into rotary motion to perform work.
- Components:
- Cylinder: The cylinder is a key component where the reciprocating motion takes place. Steam is admitted into and exhausted from the cylinder during each cycle.
- Piston: The piston is a tightly fitting, movable component within the cylinder. It is connected to a crankshaft or other mechanism to convert its linear motion into rotary motion.
- Valves: Valves control the admission and exhaust of steam into and out of the cylinder. They play a crucial role in the timing of the engine’s operation.
- Single-Acting vs. Double-Acting Engines:
- In a single-acting steam engine, steam acts on one side of the piston, and an external force (such as a counterweight or a spring) is used to return the piston to its original position during the exhaust phase. In a double-acting steam engine, steam acts on both sides of the piston, producing power on both the forward and return strokes.
- Types of Reciprocating Engines:
- Beam Engine: This type of engine features a pivoted beam, connecting the piston to a crankshaft. Beam engines were often used in pumping stations, driving pumps to lift water.
- Corliss Engine: Named after its inventor George Henry Corliss, this engine features separate valves for admission and exhaust, allowing for greater control over the steam admission and improving efficiency.
- Watt’s Engine: James Watt’s improvements to the reciprocating steam engine, including the separate condenser, made it more efficient and practical for various applications.
- Applications:
- Reciprocating steam engines were used in a variety of applications during the 18th and 19th centuries. They powered factories, mills, and machinery in industrial settings. They were also used in early locomotives, ships, and even some early forms of electrical power generation.
- Advantages and Limitations:
- Reciprocating steam engines were a significant improvement over earlier steam engine designs. They provided a reliable source of power and were instrumental in the mechanization of various industries. However, they had limitations in terms of efficiency, especially when compared to later developments such as steam turbines.
- Legacy and Preservation:
- While reciprocating steam engines are no longer widely used for industrial power generation, some historic engines are preserved in museums and heritage sites. They serve as a testament to the technological advancements of the past and the role of steam power in shaping the Industrial Revolution.
Steam Turbines
Steam turbines are a type of steam engine that converts the energy stored in steam into mechanical energy through the continuous rotation of a turbine. They are widely used for power generation, propulsion systems in ships, and various industrial applications. Here are some key features and aspects of steam turbines:
- Basic Operation:
- Steam turbines operate on the principle of converting the kinetic energy of steam into mechanical energy. Steam is directed onto the blades of a turbine, causing the turbine to rotate. The rotational motion of the turbine is then used to drive a generator, a pump, or other machinery to perform work.
- Components:
- Rotor (Blades): The rotor is the rotating component of the steam turbine. It typically consists of a set of blades mounted on a shaft. The steam flow impinges on the blades, causing the rotor to rotate.
- Stator (Nozzles or Guide Blades): The stator is the stationary part of the turbine that guides the steam flow onto the rotor blades, ensuring efficient energy transfer. It may consist of nozzles or guide blades.
- Types of Steam Turbines:
- Impulse Turbine: In an impulse turbine, steam is expanded in fixed nozzles, and the high-velocity steam jets impact the turbine blades, causing them to rotate.
- Reaction Turbine: In a reaction turbine, steam is expanded both in the nozzles and on the turbine blades. The pressure drop occurs gradually across the blades, contributing to the rotation of the turbine.
- Multi-Stage Turbines:
- Steam turbines are often arranged in multiple stages, each with its set of rotating and stationary blades. This arrangement allows for a more efficient extraction of energy from the steam and helps achieve higher overall efficiency.
- Condensing and Non-Condensing Turbines:
- Condensing Turbines: These turbines exhaust steam to a condenser, where it is condensed back into water. The vacuum created in the condenser improves the efficiency of the turbine.
- Non-Condensing Turbines: In non-condensing turbines, steam is exhausted directly to the atmosphere without being condensed. These turbines are often used in applications where the condensation process is not practical.
- Applications:
- Power Generation: Steam turbines are widely used for electricity generation in power plants. They can be found in various types of power plants, including coal-fired, nuclear, and gas-fired plants.
- Marine Propulsion: Steam turbines have been used in marine propulsion systems for ships. They were commonly employed in naval vessels and some commercial ships, providing a reliable and efficient means of propulsion.
- Industrial Processes: Steam turbines are utilized in various industrial processes to drive pumps, compressors, and other machinery. They are often chosen for their ability to provide continuous and reliable power.
- Efficiency:
- Steam turbines are known for their high efficiency, especially in large-scale power generation applications. The efficiency of a steam turbine is influenced by factors such as steam temperature, pressure, and the number of stages.
- Combined Heat and Power (CHP) Systems:
- Steam turbines are sometimes used in combined heat and power systems, where the waste heat from the turbine’s exhaust is utilized for heating purposes, increasing overall system efficiency.
- Advantages:
- Steam turbines offer several advantages, including high efficiency, reliability, and the ability to generate large amounts of power. They are particularly well-suited for continuous operation in power plants and industrial processes.
- Modern Developments:
- Advances in materials, design, and technology have led to the development of highly efficient and compact steam turbines. Combined with improvements in control systems, these developments continue to make steam turbines a vital component in power generation and industrial applications.
Steam turbines have a long history and remain an essential technology for power generation and various industrial processes. While newer technologies like gas turbines and advanced combustion engines have gained popularity, steam turbines continue to be a significant player in the energy landscape.
Modern Applications with Steam Engines
While traditional reciprocating steam engines have become less common in modern industrial and transportation settings, steam technology is still utilized in various applications. The most notable modern application is the steam turbine, which has found a place in power generation, particularly in large-scale electricity production. Here are some modern applications with steam engines:
- Power Plants:
- Steam Turbines: Modern power plants, including coal-fired, gas-fired, and nuclear power plants, often use steam turbines for electricity generation. The basic principle involves heating water to produce steam, which then drives a turbine connected to a generator. The rotating turbine converts the steam’s kinetic energy into electrical power.
- Combined Heat and Power (CHP) Systems:
- Cogeneration Plants: Some industrial facilities and district heating systems use combined heat and power (CHP) systems that incorporate steam turbines. In addition to electricity generation, these systems capture and utilize the waste heat produced during the process for heating purposes, improving overall energy efficiency.
- Renewable Energy:
- Geothermal Power Plants: In geothermal power generation, steam turbines are used to convert the energy from steam produced by natural heat from the Earth’s interior into electricity. The steam is extracted from underground reservoirs.
- Nuclear Power:
- Nuclear Reactors: Nuclear power plants harness the heat generated by nuclear fission reactions to produce steam, which then drives turbines for electricity generation. Nuclear power remains a significant source of low-carbon energy in various countries.
- Industrial Processes:
- Chemical and Petrochemical Industries: Steam is utilized in various industrial processes, and steam turbines may be employed to generate power for manufacturing plants within these sectors. This includes applications such as steam cracking in the petrochemical industry.
- Desalination Plants:
- Multi-Effect Distillation (MED): Some desalination plants use steam produced by steam turbines as part of the desalination process. MED systems utilize multiple stages of evaporation and condensation to produce fresh water from seawater.
- Research and Development:
- Experimental Applications: Steam engines, including some experimental and niche applications, may still be used in research and development settings to explore alternative energy sources or study specific engineering principles.
- Historical Preservation and Tourism:
- Heritage Railways: Some historical steam locomotives and engines are preserved and operated on heritage railways for tourism and educational purposes. These operational examples showcase the technology’s historical significance.
While traditional reciprocating steam engines are less common in mainstream industrial and transportation applications, steam technology, particularly in the form of steam turbines, continues to play a significant role in electricity generation and certain industrial processes. Advances in materials, design, and efficiency improvements contribute to the continued relevance of steam technology in specific niches.
Steam engines for small boats are an excellent option for those who want to power their watercraft using an eco-friendly and reliable source of energy. These engines use steam generated from heating water with an external source, such as wood, coal, or oil, to produce mechanical energy that drives a boat’s propeller. While steam engines have been around for more than 200 years, they continue to be a popular choice for powering small boats today.
Steam engines come in different sizes and configurations, making it possible to find the right one for your small boat. Generally, a steam engine for a small boat can range from 5 to 20 horsepower, with some models producing up to 100 horsepower. The size of the engine you need will depend on the size and weight of your boat, as well as how much speed you want to achieve.
One of the benefits of using a steam engine for a small boat is that it is relatively quiet and produces no pollution. Unlike gasoline or diesel engines, steam engines do not require any fuel storage on board, which means less space is needed for fuel and less weight is added to the boat. Additionally, steam engines can run on a variety of fuels, including wood, coal, or oil, which makes them a flexible option.
Steam engines for small boats consist of several components that work together to produce the mechanical energy needed to drive the boat. These components include a boiler, which heats the water to generate steam, a steam engine or turbine, which converts the steam’s energy into mechanical energy, a condenser, which turns the steam back into water, and a propeller, which uses the mechanical energy to move the boat through the water.
When using a steam engine for a small boat, it is important to follow proper safety protocols to prevent accidents. This includes making sure the boiler and all components are properly maintained and inspected regularly, following proper fuel handling procedures, and ensuring proper ventilation to prevent carbon monoxide buildup.
In conclusion, steam engines for small boats are a reliable and eco-friendly option for powering your watercraft. With proper maintenance and care, they can provide many years of reliable service, while also reducing your environmental impact. Whether you are a recreational boater or a commercial fisherman, a steam engine may be the right choice for your small boat.
Heat engines may be divided into two main classes, according to where the
combustion of fuel takes place. In one class, the combustion of fuel takes place outside the cylinder, and such an engine is called an external combustion engine. The most common examples of this class are steam engines and steam turbines, where the working medium is steam. In an external combustion engine, the power is produced in two stages.
The energy in steam engines
The energy in steam engines is derived from the heat energy stored in steam. Steam engines are devices that convert the thermal energy of steam into mechanical work, which can then be used to perform various tasks, such as turning a crankshaft, driving machinery, or generating electricity. The basic principle involves the transformation of heat energy into kinetic energy and, subsequently, into mechanical work. Here’s a breakdown of how the energy in steam engines is utilized:
- Generation of Steam:
- The process begins with the generation of steam. Water is heated to its boiling point in a boiler, and the resulting steam is then used as the working fluid. The heat required for this phase change is supplied by burning a fuel (such as coal, oil, or natural gas) or through other heat sources, like nuclear reactions or geothermal heat.
- Expansion of Steam:
- The steam is directed into a cylinder in the case of a reciprocating engine or onto blades in the case of a steam turbine. As the steam expands, it pushes against a piston (in reciprocating engines) or impinges on the turbine blades (in turbines), causing these components to move. The expansion of steam is the key process where heat energy is converted into kinetic energy.
- Conversion to Mechanical Work:
- The kinetic energy of the moving components (piston or turbine blades) is then converted into mechanical work. In reciprocating engines, the linear motion of the piston is converted into rotary motion using a connecting rod and crankshaft. In steam turbines, the rotary motion of the turbine rotor is directly used for mechanical work.
- Rotary Motion and Power Generation:
- The rotary motion produced by the steam engine can be harnessed for various applications. In early steam engines, this rotary motion was often used to drive machinery in factories or to turn the wheels of locomotives. In power plants, steam engines (or more commonly, steam turbines) are connected to electrical generators to produce electricity.
- Efficiency Considerations:
- The efficiency of a steam engine is influenced by several factors, including the temperature and pressure of the steam, the design of the engine, and the heat losses in the system. Engineers strive to optimize these factors to maximize the conversion of heat energy into useful work and improve the overall efficiency of the system.
- Condensation and Return:
- After performing work, the spent steam is typically condensed back into water. This condensed water is then returned to the boiler for reheating, completing the closed-loop cycle. The condensation and return processes contribute to the efficiency and sustainability of steam-based systems.
In summary, the energy in steam engines is derived from the heat energy stored in steam. The process involves the generation of steam, its expansion to perform work, the conversion of kinetic energy into mechanical work, and, in some cases, the condensation and return of the spent steam for reuse. Steam engines have played a crucial role in various applications, especially during the Industrial Revolution, and continue to be utilized in specific industrial and historical contexts.
The energy released from the fuel in the furnace of the boiler is first utilized to evaporate water in a boiler and then the steam so produced is made to act on the piston of the steam engine or on the blades of the steam turbine producing power. When the combustion of fuel takes place inside the engine cylinder so that the products of combustion directly act on the piston, the engine is known as an internal combustion engine.
Diesel engines, gas engines, and petrol engines are common examples of this class where the working medium is the product of combustion. Steam engines were manufactured up to the year 1930 for use as stationary prime movers, particularly in the textile industry. They are still used for locomotives for railways and now slowly they are being replaced by Qiesel locomotives. In addition, they are used on ships where they are slowly being replaced by steam turbines and Diesel engines
Steam Engine Plant
A steam engine plant refers to a facility or installation that incorporates steam engines for power generation, industrial processes, or other applications. These plants can vary widely in scale, purpose, and technology, but they all share the common feature of using steam engines as a primary means of converting thermal energy into mechanical work. Here are key components and aspects associated with a steam engine plant:
- Boiler:
- The boiler is a central component of a steam engine plant where water is heated to produce steam. The heat can be derived from burning fossil fuels (coal, oil, natural gas), biomass, nuclear reactions, or other heat sources. The steam generated in the boiler is then used to drive the steam engine.
- Steam Engine (Reciprocating or Turbine):
- The steam engine is the core component that converts the energy stored in steam into mechanical work. There are two main types of steam engines: reciprocating engines and turbines. Reciprocating engines use pistons, while turbines use blades to extract energy from the expanding steam.
- Condenser:
- In many steam engine plants, a condenser is used to condense the spent steam back into water after it has performed work in the engine. Condensation increases the efficiency of the plant by creating a vacuum in the system, allowing for a more effective expansion of steam in the engine.
- Pumps:
- Pumps are used to circulate water within the steam engine plant. Feedwater pumps deliver water to the boiler, while condensate pumps return condensed water from the condenser to the boiler for reheating. Other pumps may be used for water treatment or to maintain pressure in the system.
- Heat Exchangers:
- Heat exchangers may be employed to transfer heat between different fluids in the plant. For example, a feedwater heater can preheat the water entering the boiler using steam extracted from the turbine.
- Control Systems:
- Steam engine plants are equipped with control systems to regulate various parameters, including steam pressure, temperature, and flow rates. These systems ensure the safe and efficient operation of the plant.
- Generator (for Power Plants):
- In power plants, a generator is connected to the steam engine to convert mechanical work into electrical energy. The rotating shaft of the steam engine turns the generator’s rotor, producing electricity.
- Turbogenerator (for Steam Turbines):
- In power plants that use steam turbines, a turbogenerator is employed. The steam turbine drives the generator directly to produce electricity.
- Auxiliary Systems:
- Various auxiliary systems are essential for the overall functioning of the plant. These may include systems for lubrication, cooling, fuel handling, and emissions control.
- Safety Systems:
- Steam engine plants incorporate safety systems to prevent accidents and mitigate risks. These may include pressure relief valves, emergency shutdown procedures, and monitoring systems to detect and address abnormal conditions.
- Cogeneration Systems:
- Some steam engine plants are designed for cogeneration, where the waste heat from the steam engine is captured and used for other purposes, such as heating buildings or industrial processes. This enhances the overall efficiency of the plant.
- Historical and Preserved Plants:
- Some steam engine plants are historical and preserved for educational or cultural purposes. These plants showcase the technology of a bygone era and may still operate in museums or heritage sites.
Steam engine plants have been historically significant, playing a pivotal role in the Industrial Revolution and early power generation. While newer technologies like gas turbines and internal combustion engines have become more prevalent in modern power generation, steam engine plants continue to operate in specific applications and are preserved for historical and educational purposes.
A steam engine plant consists essentially of three main units: Boiler, Engine, and Condenser. In many cases, particularly in locomotive steam engines, a separate condenser is not provided and the engine exhausts into the atmosphere. The steam from the boiler is admitted into a steam chest from where it enters the engine cylinder through a valve driven by an eccentric on the engine crankshaft.
After expansion in the engine cylinder and doing work on the piston, the steam is exhausted into a condenser where it is condensed and returned as feed water to the boiler, thus, completing the cycle. Nearly all reciprocating steam engines are double-acting, i.e. steam is admitted in turn to each side of the piston and two working strokes are produced during each revolution of the crankshaft. We here illustrate a simple form of a single-cylinder, horizontal, reciprocating steam engine. The figure shows the major principal parts of the engine.
Classification of the Steam Engines
Steam engines can be classified based on various factors, including their design, operation, and application. Here are some common classifications of steam engines:
- Based on Design:
- Reciprocating Steam Engines:
- These engines use pistons that move back and forth within cylinders. The linear motion of the piston is converted into rotary motion to perform mechanical work.
- Steam Turbines:
- Steam turbines use a rotary design with blades or vanes on a rotor. The steam’s kinetic energy rotates the rotor, producing rotary motion that can be used for power generation or mechanical work.
- Reciprocating Steam Engines:
- Based on Action:
- Single-Action Engines:
- In single-action engines, steam acts on one side of the piston, providing power during one direction of motion. The return stroke is typically powered by an external force, such as a flywheel.
- Double-Action Engines:
- Double-action engines use steam to provide power in both directions of the piston’s motion. This allows for continuous rotary motion without the need for an external force during the return stroke.
- Single-Action Engines:
- Based on Steam Expansion:
- Simple Expansion Engines:
- Simple expansion engines use steam at a single pressure level during the expansion phase. The steam is exhausted once it has performed work in the cylinder.
- Compound Expansion Engines:
- Compound engines have multiple cylinders with different pressure levels. Steam passes through high-pressure and low-pressure cylinders successively, allowing for more efficient use of steam and increased expansion.
- Simple Expansion Engines:
- Based on Valve Gear:
- Slide-Valve Engines:
- Slide valves are a common type of valve gear used to control the flow of steam in reciprocating engines. These engines are known for their simplicity.
- Piston Valve Engines:
- Piston valves are an alternative to slide valves, providing better control over steam admission and exhaust. They are often found in more advanced reciprocating engines.
- Slide-Valve Engines:
- Based on Application:
- Stationary Engines:
- Stationary steam engines are fixed in one location and were historically used in factories, mills, and power plants to drive machinery.
- Marine Engines:
- Steam engines designed for use on ships are called marine engines. They powered steamships during the 19th and early 20th centuries.
- Railway (Locomotive) Engines:
- Steam engines used in locomotives for railways played a crucial role in transportation during the 19th and early 20th centuries.
- Portable Engines:
- Portable steam engines were designed for mobility and could be transported to different locations for specific applications, such as agricultural use or construction.
- Stationary Engines:
- Based on Application of Steam:
- High-Pressure Engines:
- High-pressure engines operate with steam at elevated pressures, typically in the range of 100 to 500 psi.
- Low-Pressure Engines:
- Low-pressure engines operate with lower steam pressures, often in the range of 15 to 50 psi.
- High-Pressure Engines:
These classifications provide an overview of the diverse types of steam engines that have been developed over the years. Each type has its advantages and disadvantages, and the choice of a particular type depends on factors such as the intended application, efficiency requirements, and technological advancements.
Steam engines may be classified in the following ways :
- Position of the axis of the cylinder: Vertical, Inclined or Horizontal engine.
- According to the action of steam upon the piston: Single-acting or Double-acting engine.
- A number of cylinders used in which steam expands: Single-expansion or Simple engine (total expansion of steam in one cylinder), and Multiple-expansion or compound engine (total expansion of steam in more than one cylinder).
- Method of removal of exhaust steam: Condensing or Non-condensing engine
- The magnitude of rotative speed: Low, Medium, or High-speed engine.
- Type of valve used: Slide valve, Corliss valve, or Drop valve engine.
- Use or field of application: Stationary, Portable (movable), Locomotive, Marine engine.
Parts of the Steam Engine
A steam engine consists of various components that work together to convert thermal energy from steam into mechanical work. The specific design and configuration of these parts can vary depending on the type of steam engine (reciprocating engine or turbine) and its intended application. Here are the key parts of a steam engine:
- Boiler:
- The boiler is a vessel where water is heated to produce steam. It is a critical component in the steam engine system. Boilers can vary in design, including fire-tube boilers and water-tube boilers. The heat source, which can be a furnace or other heating element, raises the temperature of the water to its boiling point, producing steam.
- Steam Engine (Reciprocating Engine or Turbine):
- The steam engine is the core component that converts the energy stored in steam into mechanical work. There are two main types of steam engines:
- Reciprocating Engines: These engines use pistons that move back and forth within cylinders. The linear motion of the piston is converted into rotary motion to perform mechanical work.
- Steam Turbines: Turbines use a rotary design with blades or vanes on a rotor. The steam’s kinetic energy rotates the rotor, producing rotary motion that can be used for power generation or mechanical work.
- The steam engine is the core component that converts the energy stored in steam into mechanical work. There are two main types of steam engines:
- Cylinder (Reciprocating Engines):
- In reciprocating engines, the cylinder is a cylindrical chamber where the piston moves back and forth. Steam is admitted to one side of the piston, causing it to move and perform work.
- Piston (Reciprocating Engines):
- The piston is a cylindrical or disk-shaped component that moves within the cylinder. It is attached to a connecting rod, and the reciprocating motion of the piston is converted into rotary motion by a crankshaft.
- Crankshaft (Reciprocating Engines):
- The crankshaft is a rotating shaft that converts the reciprocating motion of the piston into rotary motion. It is connected to the piston via a connecting rod.
- Connecting Rod (Reciprocating Engines):
- The connecting rod connects the piston to the crankshaft. It transmits the linear motion of the piston to the rotary motion of the crankshaft.
- Governor:
- The governor is a device that regulates the speed of the steam engine by controlling the admission of steam. It adjusts the flow of steam to maintain a constant speed under varying loads.
- Valve Gear:
- The valve gear controls the flow of steam into and out of the cylinder. In reciprocating engines, this can include slide valves, piston valves, or other mechanisms that manage steam admission and exhaust.
- Condenser (Some Engines):
- In some steam engine systems, particularly those using steam turbines, a condenser is used to condense the spent steam back into water after it has performed work. Condensation enhances the efficiency of the engine.
- Feedwater Pump:
- The feedwater pump circulates water from the condenser or a separate reservoir to the boiler. This pump maintains the water level in the boiler.
- Steam Generator (For Power Plants):
- In power plants, a steam generator is used to produce steam from water. This is different from a boiler and is often used in conjunction with steam turbines.
These components work together to enable the steam engine to function, converting thermal energy from steam into useful mechanical work. The specific arrangement and design of these parts depend on the type of steam engine and its intended application.
The parts of the steam engine may be broadly divided into two groups, namely, stationary parts and moving parts.
- Stationary parts: Engine frame, Cylinder, Steam chest, Stuffing box, Crosshead guides, and Main bearings.
- Moving parts: Piston and piston rod. Crosshead, Connecting rod, Crankshaft, Flywheel, Slide valve and valve rod, Eccentric and eccentric rod, and Governor.
The function of the steam engine parts is as follows: The engine frame is a heavy casting that supports all the stationary as well as moving parts of the engine and holds in proper alignment. It may rest directly on the engine foundation or upon the engine bed plate fixed on the engine foundation.
The cylinder is a cast iron cylindrical hollow vessel in which the Slide valve of the piston moves to and fro under the pressure of the steam. Both the ends of the cylinder are closed by covers and made steam right. The steam chest is a closed chamber integral to the cylinder. It supplies steam to the cylinder with the movement of the slide valve
The stuffing box and gland are fitted on the crank end cover of the cylinder and their function is to prevent the leakage of steam past the piston rod which moves to arid fro. The piston is a cast iron cylindrical disc moving to and fro in the cylinder under the action of the steam pressure. Its function is to convert the heat energy of the steam into mechanical work.
Cast Iron Piston Rings of Steam Engines
Piston rings are critical components in reciprocating engines, including steam engines, as they help form a seal between the cylinder and the piston. The primary function of piston rings is to prevent the leakage of combustion gases from the combustion chamber, ensure efficient energy conversion, and aid in the lubrication of the cylinder walls. While piston rings can be made from various materials, including cast iron, their design and material selection depend on the specific requirements of the engine. Here’s a brief overview of cast iron piston rings in steam engines:
- Material Selection:
- Cast iron is a common material for piston rings due to its favorable properties, including good wear resistance, durability, and high-temperature stability. Cast iron piston rings can withstand the harsh conditions within an engine cylinder, where they are exposed to high temperatures, pressure, and sliding contact.
- Types of Cast Iron Used:
- There are different types of cast iron used for piston rings, each with specific characteristics:
- Gray Iron: This is a commonly used material for piston rings. Gray iron is known for its excellent wear resistance and damping properties, making it suitable for engine applications.
- Ductile Iron (Nodular Iron): Ductile iron, known for its increased strength and ductility compared to gray iron, is sometimes used for high-performance applications.
- There are different types of cast iron used for piston rings, each with specific characteristics:
- Design and Function:
- Piston rings are typically installed in grooves on the outer surface of the piston. They have a spring-like tension that helps them press against the cylinder walls. The rings create a seal that prevents the combustion gases from leaking into the crankcase and promotes efficient energy transfer from the expanding gases to the piston.
- Functions of Piston Rings:
- Sealing: The primary function is to create a seal between the cylinder and the piston, preventing gas leakage during the combustion process.
- Heat Transfer: Piston rings help dissipate heat from the piston to the cylinder walls, contributing to temperature regulation.
- Lubrication: The rings help distribute oil along the cylinder walls, ensuring proper lubrication and reducing friction.
- Coating and Surface Treatments:
- To enhance the performance of cast iron piston rings, various coatings and surface treatments may be applied. These can include chrome plating, nitriding, or other treatments that improve wear resistance and reduce friction.
- Maintenance and Replacement:
- Over time, piston rings may wear due to the harsh operating conditions in the engine. Regular maintenance involves inspecting and, if necessary, replacing worn or damaged piston rings to maintain engine efficiency.
It’s important to note that while cast iron piston rings are common, advances in materials science have led to the development of alternative materials, such as various alloys and coatings, to further improve performance, reduce friction, and enhance overall engine efficiency. The choice of piston ring material depends on factors like engine design, operating conditions, and performance requirements.
Cast iron piston rings make the piston steam tight in the cylinder and thereby prevent the leakage of steam past the piston. The CTosshead is a link between the piston rod and the connecting rod.’ It guides the motion of the piston rod and prevents it from bending. The connecting rod helps in converting the reciprocating motion of the piston into the rotary motion of the crank. Its one end is connected to the crosshead by means of a gudgeon pin or crosshead pin and another end is connected to the crank.
The crankshaft is the main shaft of the engine and carries on it the flywheel and the eccentric. It is supported on the main bearings of the engine and is free to rotate in them. It is made of mild steel. The crank formed on the crankshaft works on the lever principle and produces rotary motion of the crankshaft.
The Slide Valve
The slide valve is a crucial component in many reciprocating steam engines, playing a key role in controlling the flow of steam into and out of the cylinder. It is a type of valve mechanism that directs steam to either side of the piston, allowing for the reciprocating motion that drives the engine. The slide valve is commonly associated with early steam engines and has been used in various configurations. Here’s an overview of the slide valve and its function:
Components and Operation:
- Construction:
- The slide valve consists of a flat, rectangular plate that slides back and forth over openings in the cylinder walls called steam ports. The valve plate is typically made of cast iron, and it is attached to a rod or spindle.
- Mounting:
- The slide valve is mounted on the cylinder, and it moves with the reciprocating motion of the piston. The valve plate covers and uncovers the steam ports at the appropriate times in the engine’s operating cycle.
- Steam Ports:
- Steam ports are openings in the cylinder wall that connect to the steam chest. The steam chest is a space outside the cylinder where steam from the boiler is directed before entering the cylinder.
- Steam Chest:
- The steam chest is a chamber located on the side of the cylinder. It receives steam from the boiler and distributes it to the cylinder through the steam ports.
- Admission and Exhaust Phases:
- During the engine’s operating cycle, the slide valve controls the admission of steam to one side of the piston while allowing the exhaust of steam from the other side. This process occurs in a coordinated manner to drive the reciprocating motion of the piston.
- Valve Gear:
- The movement of the slide valve is controlled by the valve gear, which can include mechanisms like eccentrics, eccentric rods, and a rocker arm. The valve gear ensures that the slide valve moves in sync with the piston’s motion.
- Double-Port and Single-Port Slide Valves:
- There are variations of the slide valve design, including double-port and single-port configurations. In a double-port slide valve, there are two steam ports on each side of the valve, while a single-port slide valve has only one port on each side.
- Reversing Mechanism:
- Some slide valve systems include a reversing mechanism to change the direction of the engine’s rotation. This is achieved by altering the position of the slide valve, redirecting steam flow to the opposite side of the piston.
Advantages and Limitations:
- Advantages:
- Simple design.
- Effective in controlling steam admission and exhaust.
- Reliability in certain applications.
- Limitations:
- Limited speed control.
- May lead to steam leakage and inefficiencies at high speeds.
- Later developments, such as piston valves, were introduced to address limitations.
The slide valve was widely used during the early years of steam engine development, especially in stationary engines and early locomotives. While it has been largely replaced by more advanced valve mechanisms in modern steam engines, the slide valve played a significant role in the industrial revolution and the development of steam power.
The slide valve is situated in the steam chest and its function is to admit the steam from the steam chest to the cylinder and exhaust the steam from the cylinder at the proper moment. The valve gets to and fro motion from the eccentric fitted on the crankshaft. The eccentric is fitted on the crankshaft. The function of eccentric is to convert the rotary motion of the crankshaft into the reciprocating motion of the slide valve.
The main bearings support the engine crankshaft and are fitted on the engine frame. The part of the crankshaft which turns in the bearing is called a main bearing journal as shown in fig. 9-3. The flywheel is a heavy cast iron or cast steel wheel mounted on the crankshaft to prevent the fluctuation of engine speed throughout the stroke and to carry the crank smoothly over the dead centers. The steam engine governor is a device for keeping the speed of the engine more or less constant at all loads. For this, it controls either the quantity or pressure of the steam supplied to the engine according to the load on the engine.
Working with a Simple, Double-acting, Condensing Steam Engine
Working with a simple, double-acting, condensing steam engine involves understanding its components, operating principles, and the associated systems. Here’s a general overview of how such an engine works:
Components of a Simple, Double-acting, Condensing Steam Engine:
- Cylinder:
- The cylinder is a key component where the reciprocating motion of the piston takes place.
- Piston:
- The piston is a cylindrical component that moves back and forth within the cylinder. It is connected to a piston rod.
- Slide Valve:
- The slide valve controls the admission and exhaust of steam to and from the cylinder. It is mounted on the side of the cylinder and moves in coordination with the piston.
- Steam Chest:
- The steam chest is a chamber located outside the cylinder. It receives steam from the boiler and distributes it to the cylinder through the slide valve.
- Condenser:
- The condenser is a component that condenses the exhaust steam into water. It enhances the efficiency of the engine by creating a vacuum in the system.
- Boiler:
- The boiler is where water is heated to produce steam. The steam generated in the boiler is then sent to the steam chest.
- Pump (Condensate Pump):
- The pump is used to remove the condensed water (condensate) from the condenser and return it to the boiler.
- Connecting Rod and Crankshaft:
- The connecting rod connects the piston to the crankshaft. The linear motion of the piston is converted into rotary motion by the crankshaft.
Operating Principles:
- Admission Phase:
- Steam is admitted to one side of the piston through the slide valve. The pressure of the steam pushes the piston, causing it to move.
- Expansion Phase:
- As the piston moves, the steam expands and does work on the piston. This is the phase where mechanical work is performed.
- Exhaust Phase:
- The slide valve redirects the steam to the other side of the piston, allowing the exhaust steam to escape from the cylinder.
- Return Stroke:
- The piston now moves in the opposite direction as the steam is admitted to the other side. The cycle repeats.
- Condensation:
- After performing work, the exhaust steam is directed to the condenser, where it is condensed into water. This creates a vacuum in the cylinder, improving efficiency.
- Pump Action:
- The condensate pump removes the condensed water from the condenser and returns it to the boiler. This closed-loop system allows for the reuse of water.
Working Cycle:
- Admission of Steam:
- Steam is admitted to one side of the piston, pushing it and performing work.
- Expansion:
- Steam expands, and the piston moves, converting the energy into mechanical work.
- Exhaust:
- The slide valve redirects steam to the other side of the piston, and exhaust steam is released.
- Return Stroke:
- The piston moves in the opposite direction, and the cycle repeats.
- Condensation and Pumping:
- Exhaust steam is condensed in the condenser, and the condensate is pumped back to the boiler.
Understanding the cycle and components involved in a simple, double-acting, condensing steam engine provides insight into its operation and efficiency. Keep in mind that variations exist, and advancements in steam engine technology have led to more complex and efficient designs.
The function of a steam engine is to convert the heat energy of steam into
mechanical work. The pressure of the steam acts on the piston and moves it to and fro in the cylinder. It is necessary to have some method of converting this to-and-fro motion of the piston into a rotary motion since the rotary motion can be conveniently transmitted from the engine to any other driven machine.
This to-and-fro motion of the piston is converted into rotary motion with the help of connecting the rod and crank of the steam engine. This steam admitted to the cover end exerts pressure on the surface of the piston and pushes it to the crank end (right-hand side) of the cylinder. At the end of this stroke, fresh steam from the steam chest is again admitted by the D-slide valve to the crank end of the cylinder (when the admission steam port is opened), while the exhaust steam on the cover end of the cylinder passes at the same time into the condenser through the steam port and exhaust port.
The Crankshaft of the Steam Engine
The crankshaft is a crucial component in many types of engines, including steam engines. Its primary function is to convert the reciprocating motion of the piston into rotary motion, allowing for the efficient transfer of power from the engine to other mechanical devices or systems. Here’s how the crankshaft works in the context of a steam engine:
Components and Functionality:
- Connecting Rod:
- The connecting rod connects the piston to the crankshaft. It is typically a rigid rod that transmits the linear motion of the piston to the rotary motion of the crankshaft.
- Piston:
- The piston is a cylindrical component that moves back and forth within the cylinder of the steam engine. It is connected to the connecting rod.
- Crankshaft:
- The crankshaft is a rotating shaft with one or more crankpins offset from the axis of rotation. The crankpins are connected to the connecting rods. The crankshaft’s design and configuration depend on the engine type and its intended application.
Working Principle:
- Reciprocating Motion:
- As the steam engine operates, the piston moves back and forth within the cylinder in a reciprocating motion. This motion is a result of the expansion and contraction of steam in the cylinder, which pushes the piston.
- Connecting Rod:
- The connecting rod is attached to the piston at one end and to the crankshaft at the other end. As the piston moves, it imparts linear motion to the connecting rod.
- Crankshaft Rotation:
- The connecting rod is connected to the crankshaft at a point called the crankpin. The offset nature of the crankpin causes the crankshaft to rotate when the connecting rod moves. This rotation converts the reciprocating motion of the piston into rotary motion of the crankshaft.
- Rotary Motion Transfer:
- The rotary motion of the crankshaft can be further utilized to drive other mechanical components, such as gears, belts, or directly connected machinery. This rotary motion is more suitable for many applications, providing a continuous and smoother operation compared to the reciprocating motion of the piston.
Key Considerations:
- Number of Crankpins:
- The number of crankpins on a crankshaft depends on the engine’s design. Engines may have single-cylinder crankshafts or multi-cylinder crankshafts, where each cylinder has its own crankpin.
- Balance and Vibration:
- Proper balance is crucial for the smooth operation of the engine. Counterweights may be added to the crankshaft to balance the forces generated by the reciprocating components, reducing vibration and wear.
- Bearings:
- The crankshaft is supported by bearings to reduce friction and facilitate smooth rotation. Lubrication is essential to minimize wear on the bearings.
- Design Variations:
- Different engine designs may have variations in crankshaft configurations. For example, a steam engine with a double-acting piston may have two connecting rods and two crankpins, providing power during both the piston’s forward and backward strokes.
In summary, the crankshaft in a steam engine plays a crucial role in converting reciprocating motion into rotary motion, allowing the engine to efficiently transfer power to external devices or systems. The design and construction of the crankshaft are key factors in the overall performance and reliability of the steam engine.
Thus, the steam at the cover end exhausts while that at the crank end pushes the piston back to its original position. The D-slide valve gets to and fro motion from the eccentric fitted on the crankshaft. Thus, two working strokes are completed and the crankshaft turns by one revolution, i.e., the engine is double-acting. These operations are repeated. When the exhaust steam is exhausted into the atmosphere, the engine is known as a non-condensing engine.
The motion of the piston and piston rod moves the crosshead, connecting rod, crank, and crankshaft. The motion of the piston, piston rod, and crosshead is to and fro. This to-and-fro motion is converted into rotary motion with the help of the connecting rod, crank, and crank pin. The end of the connecting rod which is attached to the crosshead can only move in a straight line, while the other end attached to the crank pin can move only in a circle, since, the crank carrying the crank pin is free to turn the crankshaft.
The motion of the connecting rod is, thus, oscillating. Since the crank is fixed on the crankshaft, the crankshaft will rotate in its bearing. The flywheel is mounted on the crankshaft. The following terms are useful in understanding the working of the steam engine: The cylinder bore is the inside diameter of (the cylinder or the liner. The piston stroke is the distance traveled (or moved) by the piston from one end of the cylinder to the other end, while the crank is making half a revolution.
EMS Power Machines
We design, manufacture and assembly Power Machines such as – diesel generators, electric motors, vibration motors, pumps, steam engines and steam turbines
EMS Power Machines is a global power engineering company, one of the five world leaders in the industry in terms of installed equipment. The companies included in the company have been operating in the energy market for more than 60 years.
EMS Power Machines manufactures steam turbines, gas turbines, hydroelectric turbines, generators, and other power equipment for thermal, nuclear, and hydroelectric power plants, as well as for various industries, transport, and marine energy.
EMS Power Machines is a major player in the global power industry, and its equipment is used in power plants all over the world. The company has a strong track record of innovation, and it is constantly developing new and improved technologies.
Here are some examples of Power Machines’ products and services:
- Steam turbines for thermal and nuclear power plants
- Gas turbines for combined cycle power plants and industrial applications
- Hydroelectric turbines for hydroelectric power plants
- Generators for all types of power plants
- Boilers for thermal power plants
- Condensers for thermal power plants
- Reheaters for thermal power plants
- Air preheaters for thermal power plants
- Feedwater pumps for thermal power plants
- Control systems for power plants
- Maintenance and repair services for power plants
EMS Power Machines is committed to providing its customers with high-quality products and services. The company has a strong reputation for reliability and innovation. Power Machines is a leading provider of power equipment and services, and it plays a vital role in the global power industry.
EMS Power Machines, which began in 1961 as a small factory of electric motors, has become a leading global supplier of electronic products for different segments. The search for excellence has resulted in the diversification of the business, adding to the electric motors products which provide from power generation to more efficient means of use.